Echocardiographic guidance for diagnostic and therapeutic percutaneous procedures
Introduction
Echocardiography is beneficial for guiding numerous percutan-eous cardiovascular procedures of the pericardium, myocardium, and cardiac valves. It also plays an important role in guiding vascular access. The advantages of echocardiographyinclude mobility and real time imaging, which allow for assessment before, during, and after procedures. Ultrasound imaging is widely available, portable, and offers clear images to help evaluate the severity of valvular disease, surrounding structures, and the location of catheters and devices. These benefits enhance the efficiency and safety of diagnostic and therapeutic procedures. This article will address the advantages and the role of ultrasound imaging in percutaneous procedures, namely, transvenous and transarterial access, pericardiocentesis, endomyocardial biopsy, pulmonary valve repair, transcatheter aortic valve implantation, and mitral valve repair.
Transvenous access
Physicians place millions of central venous catheters (CVC) each year in the United States. Central venous access is commonly indicated for hemodynamic monitoring, hemodia-lysis, and administration of fluids, medications and nutrition. Unfortunately, the complication rate associated with the procedure exceeds 15% (1). Types of complications include infection, thrombosis, and mechanical, all of which depend on several factors such as operator experience, patient anatomy, and procedure characteristics (2,3). Common mechanical complications are arterial puncture, pneumothorax, hematoma, and hemothorax; these are reported to occur in 5 to 19% of patients (1,4,5). Complications may compromise patient safety and are expensive to treat, thus minimizing adverse events is beneficial. Ultrasound (US) guidance for the insertion of central lines, particularly through the internal jugular vein (IJ), has been shown to reduce mechanical complications, improve success rates, reduce number of attempts, and time required for insertion when compared to the technique of using external anatomic landmarks (6-9).
The internal jugular, subclavian, and femoral veins are common sites for central venous access. Successful catheteriz-ation using the landmark technique relies on a thorough understanding of anatomic landmarks at these sites. However, there is significant variability in anatomic structures in relation to the target veins (10,11). Thus, real-time (RT) US guidance allows operators to clarify the relative position of the needle, the desired vein, and the surrounding anatomy prior to and during CVC insertions (2,3). In a meta-analysis of studies comparing RT US guidance method with the landmark method for central line placement, Randolph et al. observed that US guidance decreased complications during attempted CVC placements (relative risk 0.22, confidence interval [CI] 0.10-0.45), corresponding to a relative risk reduction of 78% and reduced placement failures (relative risk 0.32, 95% CI: 0.18-0.55) when compared to the landmark method (12). In addition, the mean number of attempted venipunctures was significantly reduced with US guidance (relative risk 0.60, 95% CI: 0.45-0.79), corresponding to a relative risk reduction of 40%. In a recent meta-analysis of studies comparing two-dimensional (2D) US guidance or Doppler US guidance method with the landmark method, Hind et al. also observed similar results. For IJ insertions, 2D US guidance reduced complications (relative risk 0.43, 95% CI: 0.22-0.87), and decreased placement failure (relative risk 0.14, 95% CI: 0.06-0.33), when compared to the landmark method (2). They also observed that fewer attempts were required for successful placement and less time needed with 2D US guidance.
Other subsequent studies also suggest that US guided IJ catheterization was associated with a higher success rate and a lower complication rate. In a randomized, prospective study of RT US guided IJ catheterization in the emergency department (ED), Leung et al. observed that IJ cannulation was successful in 93.9% with US guidance method compared to 78.5% with the landmark method, a significant difference of 15.4% (P=0.009, 95% CI: 3.8% to 27.0%). Of the successful US guided catheterizations, 82.0% were inserted on the first attempt compared to 70.6% of the successful landmark catheters. The complication rate was observed in 4.6% in the US guidance method compared to 16.9% in the landmark method, a difference of 12.3% (95% CI 1.9% to 22.8%) (13). In a recent randomized, prospective study, Turker et al. also observed similar results with the US guided method having fewer number of attempts for successful insertion and a lower complication rate when compare to the landmark method(P<0.05 and P<0.01, respectively) (14).
The operator is able to visualize the relative position of the needle, the vein, and its surrounding structures, in addition to assessing the variant anatomy and patency of a target vein with the use of US guidance. During US guidance, the carotid artery is often visualized and it is pulsatile. Conversely, the IJ is weakly pulsatile and readily compressed by pressing against the vein with the US transducer, Figure 1A,B. With these advantages, patients are less likely to experience a prolonged, possibly uncomfortable, and sometimes unsuccessful catheterization (2,3). Because the use of RT US guidance has been shown to be beneficial for CVC placement, the Agency for Healthcare Research and Quality has recommended US as one of their 11 practices to improve patient care (3). The National Institute of Clinical Excellence in the United Kingdom also supports the use of US guidance for CVC placement (15).
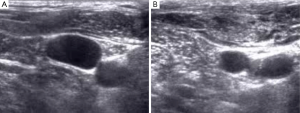
Transfemoral arterial access
The majority of diagnostic and interventional catheterizations are performed via percutaneous femoral arterial access. Annually, approximately up to 10 million arterial accesses are done worldwide (16,17). The incidence of vascular complication-sassociated with this procedure occurs in 1.8% to 4% of patients (18). Types of complications include hematomas (2-8%), pseudoaneurysm (1-2%), and arteriovenous fistulas (0.2-5%) (19-21). Risk factors for vascular complications include age, female gender, weight, previous arteriotomy at the same site, anticoagulation, renal failure, and location of arteriotomy (22). Vascular complications have been associated with increased length of hospitalization, morbidity, mortality, and healthcare costs (21,23-25). Fewer complications have been associated with arteriotomy site in the common femoral artery (CFA). In comparison, retroperitoneal hemorrhage is associated with cannulation above the inguinal ligament while pseudoaneurysm and arteriovenous fistula formation are associated with cannulation below the CFA bifurcation (26-29). Thus visualization of the vasculature and surrounding landmarks for the appropriate location of arteriotomy is important to reduce the risks and complications of femoral arterial access.
In most cases, a palpable arterial pulse is used as a landmark for access site. This is a well established, safe, and quick method for CFA catheterization (30,31). However, with significant variations in femoral vessel anatomy and if pulses are weak or nonpalpable, it may be challenging to obtain access at the appropriate site. The femoral head has been shown to be a reliable landmark for identifying the CFA and is visible with fluoroscopy. Therefore, fluoroscopy with arterial cannulation has been recommended to potentially reduce complications (32,33). The CFA courses over the femoral head in 92% of cases and the bifurcation of the CFA occurs below the middle of the femoral head 99% of the time (34). By using fluoroscopy to locate the femoral head, the chances of successful CFA puncture in the correct location are high. However, recent randomized trials did not demonstrate improvement with the use of fluoroscopy in the rate of CFA cannulations or reduction of complications compared to the palpation method alone (35-37). Furthermore, the wide spread use of fluoroscopy to obtain arterial access vary. Reasons for the variation are the high success rates with palpation method alone, the additional time and radiation exposure associated with the use of fluoroscopy, and the lack of data regarding benefit (35).
As discussed above, US guidance has been widely used for CVC access and now has become the standard of care. In a recent multicenter, randomized, controlled trial comparing fluoroscopic guidance versus US guidance for femoral arterial access, Seto et al. observed that US guidance reduced the risk of vascular access complications by 59%, improved first pass success rate to 82.7%, greatly decreased the number of accidental venipunctures, and reduced the time required for access (38). Table 1 lists the intraprocedural outcomes of this study. Ultrasound guidance improved CFA cannulation rates in patients with high bifurcations by 31%; however, it did not improve the rate of CFA cannulation in the overall population. Figure 2A illustrates a transducer with a needle guide and the underlying target artery. In Figure 2B, US guidance readily visualize the femoral artery and vein, and as the transducer is moved superiorly, the common femoral artery is clearly seen, Figure 2C. The benefits of US guidance method over fluoroscopy may improve patient comfort by shortening the time required for access and decreasing tissue and vessel trauma due to multiple venipunctures. Ultrasound would also be beneficial when CFA cannulation is essential, especially for high-risk patients with difficult access. It was also observed to be helpful in obese patients and patients with weak arterial pulses (31).
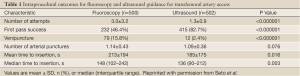
Full table
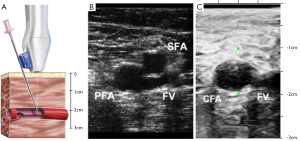
Pericardiocentesis
For patients suffering from pericardial tamponade (39), pericardio-centesis is a lifesaving procedure. However, until the advent of echocardiographic guidance, this procedure was essentially performed “blind”. Prior to US guidance, pericardiocentesis was associated with relatively high mortality and complication rates (6% and 20-50%, respectively) (40-42). Types of complications included pneumothorax, damage to surrounding vital organs, cardiac wall punctures, and death (39,43,44). Over the past 30 years, the incidence of pericardial effusions, as a complication of cardiothoracic surgery and perforations from catheter-based procedures, have increased the need for pericardiocentesis. Ultrasound guidance has improved the safety of this procedure in the past few decades, lowering both mortality and complication rates with direct visualization of the heart and surrounding organs.
Ultrasound guidance may be used to locate the optimal site of puncture for pericardiocentesis as well as visualize the depth of the effusion, distance from the chest wall to the effusion, and the surrounding vital organs (44). As shown in Figure 3, the optimal site of puncture is identified as the site where the largest fluid collection is closest to the body surface and from which the needle trajectory will avoid vital structures (40,44) The two most common entry sites for the needle are apical areas and the subxiphoid, but a parasternal location can also be used though it is used less frequently. The pericardial sac and effusion can be entered with a needle or with a polytef-sheathed needle. Continuous US guidance is generally not needed for the procedure, as it is often difficult to keep visualization of the needle tip. However, an injection of agitated saline can be used to confirm the location of the needle if bloody fluid has been aspirated or the position of the catheter is unknown (44). Figure 4A demonstrates a catheter within the pericardial effusion, however the fluid is bloody. In Figure 4B, a saline contrast study was done and it demonstrated that the contrast only appeared in the pericardial space, confirming that the catheter was within the pericardium and not within the heart. If the needle, guidewire, or sheath is not in the pericardial sac, it should be withdrawn, repositioned or another attempt should be used to enter the pericardial space. Figure 5 shows a case example in which a guidewire was inadvertently inserted into the LV but then detected by echo to not be in the pericardial space (but in the LV). Consequently the guidewire was removed and a repeat pericardiocentesis was performed. The subcostal, apical, or parasternal views can be used to monitor the procedure to ensure that the effusion is decreasing in size during the pericardiocentesis (39). For optimal drainage, we insert a pigtail catheter, into the pericardial sac. The catheter is then attached to a Jackson-Pratt drain, and left in place for 24 hours to several days once the drainage has significantly decreased to less than 100 mL in 24 hours (45) This minimizes the chance of reoccurrence of the effusion to <20% at one year (45). The omission of extended catheter drainage has been associated with recurrence rates up to 55% (41,45). Limited repeat echocardiograms should be used to assess the residual fluid daily. If there is a change in the appearance of the fluid aspirated, chest pain, sudden increase in fluid drained, or change in vitals, the patient should be reassessed (40).
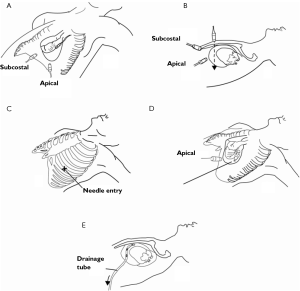
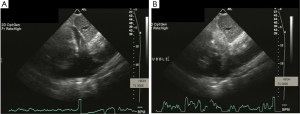
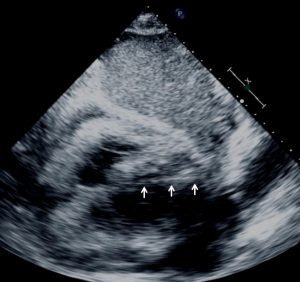
A decrease in both mortality and complication rates have been observed with US guided pericardiocentesis, as shown in Table 2. In 1,127 cases of US guided pericardiocentesis performed at the Mayo Clinic between 1979 and 2001, 97% of the cases were successful and 89% only needed a single attempt to gain access (41). Major complications, including chamber lacerations, pneumothorax with chest tube re-expansion, tachycardia, and death, only occurred in 1.2% of patients and minor complications, including minor pneumothorax, pleural pericardial fistula, and vasovagal responses, were observed in 3.5% of patients. With US guidance, patients have a better chance of survival and significantly lower complication rates (43). The Yonsei Clinic also achieved similar success rates. The 11-year study had an astounding overall success rate of 99% with a major complication rate of only 0.7% and 0 deaths (46). In addition, the safety of US guided pericardiocentesis has been shown in pediatric patients, cardiac perforated patients that were hemodynamically unstable, postoperative patients, and patients with malignancies (42,47,48).
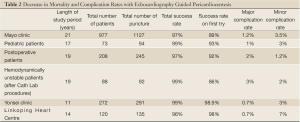
Full table
With the help of US guidance, the operator can directly visualize the pericardial effusion, the surrounding structures, the wall chambers, and most importantly the best approach to access the effusion. This helps reduce the number of failed attempts, chamber and organ punctures, and other major complications. With echocardiographic guidance, this procedure can be performed safely, relatively quickly, and efficiently at the bedside in the ICU, in the catheterization laboratory, or as outpatient in the echocardiography laboratory (41). In addition, US imaging reduces the need for surgery and exposure to radiation compared to fluoroscopy. Ultrasound guidance increases safety and efficacy; thus, it has become the standard method for pericardiocentesis.
Endomyocardial biopsy
First introduced in 1962, endomyocardial biopsies have help eddiagnose a wide variety of myocardial disorders, such as infiltrative cardiomyopathy and heart transplant rejection (44,49). This procedure is usually done under fluoroscopic guidance; however, echocardiography have been used to supplement or replace fluoroscopic guidance (44,50,51). Under echocardiographic guidance, there is a wider choice of biopsy sites, including ventricular septum, RV apex, and free wall (52). Since 2D TTE provides spatial orientation and anatomic definition, the likelihood of complications are greatly reduced, such as perforation and damage to the tricuspid valve (53). Most importantly, US guidance can assure that specimens are obtained from the septum and not the right ventricular (RV) free wall, especially since perforation of the RV or LV remains the most serious risks of the procedure (52,53). Ultrasound guidance also offers precise visualization, thus serial biopsies may be performed thereby allowing a quick procedure lasting 10-15 minutes.
Most myocardial biopsies may be performed in the catheterization laboratory with standard echocardiographic machines. A sheath is placed percutaneously in the right femoral or jugular vein (under US guidance) and the bioptome is advanced under X-ray guidance until it enters the right atrium, at which point, US guidance is continuously used (44,52,54). Once the forceps enter the right atrium, the subcostal or apical four-chamber view can be used to image the biopsy catheter (44,50,52). Ultrasound guidance helps the operating physician guide the forceps across the tricuspid and into the right ventricle. In Figure 6, echo can readily be used to identify the tip of the bioptome and determine its location. With US guidance, the bioptome may be repositioned along the intraventricular septum until its location is satisfactory, Figure 7. The position of the tip can be optimally viewed with both the apical and subcostal four-chamber views (44,52). Then the jaws of the forceps are open and the sample is excised, after which the site becomes a bit rigid. The procedure is performed repeatedly but the physician must take care to avoid performing a second biopsy at the same site. The most serious complication during this procedure is perforation of the right ventricle, a complication that has a high risk of death or morbidity (53). These complications are more likely to occur in patients with hypertrophic cardiomyopathy, endomyocardial fibrosis, or cardiac tumors (52,53). For these patients, US guidance gives optimal visualization to distinguish the septum from the RV free wall, as seen in Figure 8. In addition, US guidance helps to alert the physician when a complication occurs during the procedure and facilitates follow-up care with immediate detection of complications, especially pericardial effusions.
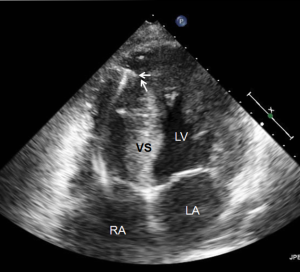
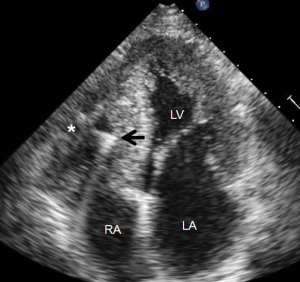
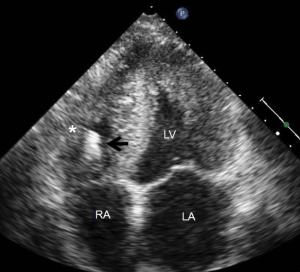
Many different centers and physicians have had success with US guided endomyocardial biopsies. Pierard et al. performed 22 biopsies on 18 patients (5-6 samples each patient on average) and had a success rate of 100% with no major complications (52). Minor complications included chest discomfort, premature ventricular contractions and atrial fibrillation. They concluded that US guidance is safe and simple technique to performing biopsies. In a study by Han et al., 90 patients underwent endomyocardial biopsies (55). The major complication rate was 5.6% and included myocardial perforation, right bundle branch block, and hemodynamically unstable tachycardia. Also, there were three perforations but none that required pericardiocentesis and there were no procedure related mortalities. Blomstrom-Lundqvist et al. performed 231 endomyocardial biopsies in 74 patients (50); sixty biopsies were performed under X-ray guidance while 171 were performed with US guidance with a complication rate of 4.8% (no deaths). In five of the procedures performed, all of which were under X-ray guidance, the specimens were considered inadequate. In addition they found that the incidence of cardiac perforations was higher in X-ray guided procedures than US guided procedures. They also discovered that more epicardial and pericardial tissue in X-ray guided biopsy specimens (5.8%) than those with US guidance (0.7%). Thus, the study concluded that US guidance improves safety and efficacy compared to X-ray guidance.
US guidance can assist in the safe and accurate performance of endomyocardial biopsies. X-ray guidance is another option, however, procedural complications and safety issues are a factor. Transesophageal echocardiography and intracardiac echocardiography may offer more optimal imaging, but have more risks and costs with patient sedation and required personnel (44).
Pulmonic Valve Implantation
Pulmonic valve disease is often associated with congenital heart diseases. Surgical intervention for these conditions consists of placing prosthetic valved or valveless conduits to establish continuity between the right ventricle (RV) and the pulmonary artery (PA) (56). Overtime, these prosthetic conduits are susceptible to obstruction or regurgitation leading to symptoms of exercise intolerance, arrhythmias, RV dysfunction, and an increased risk for sudden death (57-59). Patients with postoperative RV outflow tract (RVOT) dysfunction, whether from pulmonary regurgitation (PR), obstruction, or both, will need surgical intervention (60). Often, patients may undergo multiple open-heart surgeries over a lifetime to treat conduit dysfunction (61,62). Percutaneous pulmonary valve implantation (PPVI) appears to be a promising alternative to surgical pulmonary valve replacement (PVR) (63-65). Studies have shown that PPVI reduced RVOT obstruction, provided a competent pulmonary valve, improved functional status, and peak exercise parameters (56,60,65,66).
Numerous imaging modalities are used prior to, during, and post procedure of PPVI. It is important to have a full understanding of the outflow tract anatomy because candidates for PPVI must fulfill anatomic requirements for safe anchoring of the valve (RVOT >14 mm × 14 mm and < 22mm × 22 mm) (63). Patients also undergo cardiac magnetic resonance imaging (cMRI), with 3D reconstruction, prior to the procedure to determine the maximal and minimal dimensions of the RVOT. If the results of cMRI are doubtful, then balloon sizing of the RVOT is performed at the time of cardiac catheterization. Angiography of the coronary arteries is performed to assess the risk of compression from the valve implantation. If the risk of compression remains unclear, then coronary angiography is done with simultaneous inflation of angioplasty balloon at the implantation site (65,67,68). The site for implantation is determined from angiographic studies and hemodynamic assessment of RVOT to identify the position and extent of obstruction and regurgitation (56,63,69).
Percutaneous pulmonary valve implantation is currently done under fluoroscopy guidance. Transthoracic echocardiography is used mostly to assess the conduit mean gradients, RV size and function, and severity of PR prior to, during, and post procedure in PPVI. Figure 9 shows a 2D TEE view of a stented pulmonary artery with severe PR by 2D and 3D color flow Doppler. A catheter is well seen in the stent graft in Figure 10. Pre-deployment valve echo is used to identify the zone after implantation as seen in Figure 11A. Figure 11B shows a successfully placed pulmonic valve color flow Doppler. Two dimensional and 3D are used to further assess for PR. In this care, there was no residual PR.
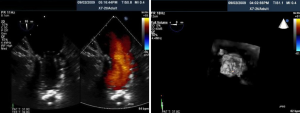
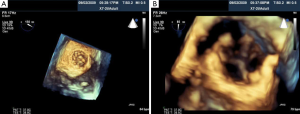
As illustrated above, intra-procedural 2D and 3D transesoph-ageal echocardiography may be beneficial during PPVI by providing interventionalists and echocardiographers the ability to fully assess the size and anatomy of the RVOT, PA, and surrounding structures. This may aid in selecting candidates with the appropriate anatomical requirements and avoid the potential risk of coronary artery compression. Other advantages may include localizing and identifying of guidewires, catheters, and balloons for precise position of valve deployment to prevent procedural complications.
Transcatheter Aortic Valve Implantation
Aortic stenosis is a progressive disease. After the onset of symptoms, untreated patients are at a high risk for death. The prevalence of aortic stenosis increases with age. It is present in 2% of the population over 50 years old, 3% over 75 years old, and 4% over 85 years old (70-72). The progression rate of aortic stenosis averages 0.1 cm2/year. Severe aortic stenosis is defined as having a maximum Doppler velocity across the aortic valve greater than 4 m/s, a valve area less than 1 cm2, and a mean aortic valve gradient greater than 40 mmHg (73). Indications for surgical intervention include the development of symptoms such as chest pain, syncope, shortness of breath, or other symptoms associated with heart failure, or if there is evidence of LV dysfunction (LVEF <50%). Approximately one third of elderly patients with symptomatic aortic stenosis are denied aortic valve replacement (AVR) due to significant co-morbidities (73-75). For patients who are high-risk surgical candidates, transcatheter aortic valve implantation (TAVI) is a less invasive, promising alternative in the treatment of aortic stenosis.
The first TAVI was performed in 2002, and since then, more than 25,000 Sapien Edwards aortic valves have been implanted worldwide (76). Figure 12 shows the 2 TAVI devices currently used in clinical trials in the United States or commercially used internationally, the MedTronicCoreValve (Minneapolis, Minnesota) and the Edwards Sapien Valve (Edwards Life Sciences, Irvine, California). The TAVI procedure consists of passing a catheter from the femoral artery up to the aorta and crossing the aortic valve. A standard balloon aortic valvuloplasty is performed to pre-dilate the stenotic aortic valve. The bioprosthetic heart valve, crimped onto a balloon catheter, is then advanced across the native aortic valve and deployed during rapid right ventricular pacing (77). TEE is used to size the aortic annulus. Concomitant TEE and fluoroscopy are used to position and deploy the valve. Successful TAVI requires selecting the appropriate prosthetic valve size and precise placement of the valve prosthesis. Intra-procedural 2D and/or 3D TEE provide interventional cardiologists and echocardiologists the ability to fully evaluate the anatomy of the aortic valve, annulus, and surrounding structures in patients undergoing TAVI. Table 3 lists the role of TEE guidance in TAVI.
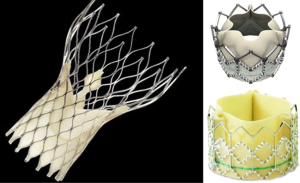
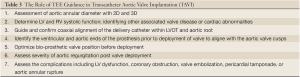
Full table
Two-dimensional and 3D TEE can visualize the aortic annulus, valve cusps, coronary arteries, and shape of the LVOT. In Figure 13, a 2D TEE pre-procedural X-plane image visualizes the aortic valve in cross section, in addition to the long axis view, in a patient undergoing TAVI. As shown in Figure 14A-D. 2D, 3D TEE in the short axis view at the aortic level (30°-60°) often visualizes the left main coronary artery and the long axis view (110°-140°) clearly shows the right coronary artery ostium. Annular remodeling in aortic stenosis has been reported to cause a decrease in the distance between the aortic annulus and coronary ostia. With X-plane 3D TEE mode, the ostia of the coronary arteries are distinctly visualized allowing evaluation of their distance from the native and prosthetic valve. This allows safer delivery of the prosthesis by avoiding occlusion of the coronary ostia. Other complications may arise if the prosthetic valve is not deployed in the correct position. The preferred position is for the prosthetic valve to be coaxial to the native valve and the ventricular edge of the aortic valve should be 2-4 mm below the aortic cusps allowing the post deployment position of the prosthesis to be 1-2 mm below the insertion points of the native valve cusps. Deployment of the valve too low in the LVOT may result in impingement of the anterior mitral leaflet. However, if the valve is deployed too high, then the prosthetic valve may occlude the coronary ostia or embolize into the aorta.
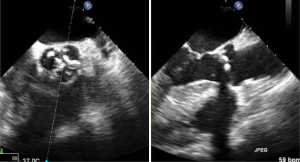
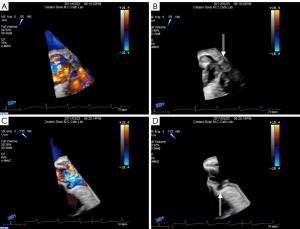
Pre-procedural TTE and intra-procedural TEE are used to assess the diameter and size of the aortic annulus. CT is often used to measure the aortic annulus prior to the procedure. During TAVI, TEE is used to corroborate the procedural measurements. For the Edwards Sapien valve, if the aortic annulus is 18-23 mm, then a 23 mm prosthesis is used for implantation, whereas, a 26 mm prosthesis is selected if the annulus is 23-26 mm. The annulus diameter is measured at the lowest portion of the hinge point of the aortic valve cusps.
However, the annular diameter measurements may vary because of the shape of the annulus is often oval rather than circular (78), depending on whether measurements were taken from a coronal or a sagittal plane. As shown in Figure 15A. X-plane imaging from a 3D TEE system allows for measuring the aortic valve annulus in orthogonal views. In Figure 16, 3D TEE imaging reveals that the aortic annulus is not circular but oval in this patient. Of note, 3D TEE has less underestimation of annular size and narrower limits of agreement then 2D TEE. Correct determination of aortic annular size is necessary to avoid complications such as post-procedural paravalvular aortic regurgitation if the prosthesis is too small or aortic annulus rupture if the prosthesis is too large.
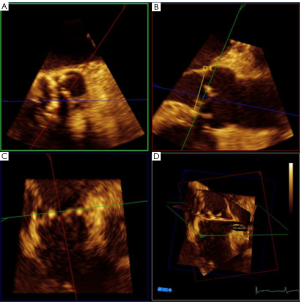
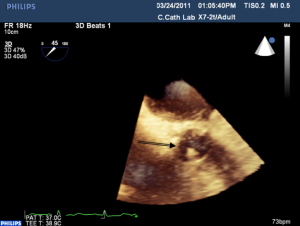
Aortic annulus may also vary in size depending on which imaging modality is used. Several studies have compared the measurements of the aortic annulus obtained by TTE, TEE, and cardiac CT in patients undergoing TAVI. Differences in measurements observed between the modalities include underestimation of annular size by TTE (79), disparity by approximately 1.4 mm between TTE and TEE (80), and underestimation of annular diameter by 2D TEE when compared between 3D TEE and MDCT (81).
During the TAVI procedure, both 2D and 3D TEE help identify intracardiac catheters, the valvuloplasty balloon, and the bioprosthetic valve. As shown in Figure 17, 3D TEE clearly shows the catheter across the calcific aortic valve in the aorta and LV. During predilation with balloon valvuloplasty, the balloon can be clearly visualized as seen in Figure 18A. After balloon deflation, echo is used to assess for complications. Figure 18B demonstrates that 3D color is also useful to assess the severity of aortic regurgitation post valvuloplasty. Prior to deployment of the bioprosthetic valve, the valve should be coaxically aligned in the LVOT and at the right level to avoid implanting the valve at an angle, which may result in paravalvular regurgitation. To achieve proper alignment, the ventricular edge of the valve should be approximately 4 mm below the aortic cusps, as shown in Figure 19A-C. In panel 19B, the bioprosthetic valve is placed too far into the aorta relative to the aortic cusps, whereas in panel 19 C after adjustment, the bioprosthetic valve is in the appropriate position across the native aortic valve between the LVOT and aorta. Figure 20 was obtained during rapid RV pacing during inflation of the balloon and deployment of the bioprosthetic valve with RT 3D TEE. Three-dimensional imaging illustrates how well the valve is seated as shown in Figure 21A. Color-flow 3D TEE permits assessment of both the severity and precise origin of the aortic regurgitation, whether paravalvular or central, and if the regurgitation is coming from multiple sites, as seen in Figure 21B.
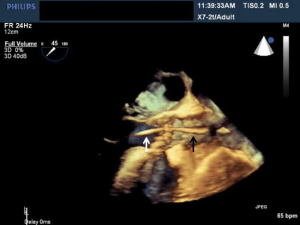
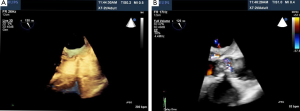
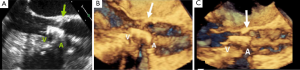
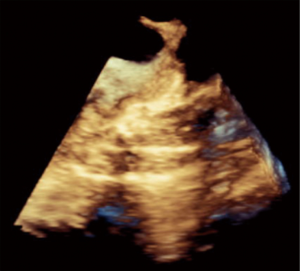
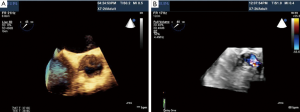
Aortic valve regurgitation is common after TAVI, occurring in 88% of cases (80). It is usually paravalvular regurgitation, which can be trivial to severe, and is generally along the posterior aspect of the prosthetic valve. Less commonly, the regurgitation can be transvalvular and often diminishes over time. In a recent study, moderate or severe paravalvular aortic regurgitation was present in 11.8% of the patients in the TAVI group at 30 days and in 10.5% at 1 year (82). The presence of moderate to severe paravalvular regurgitation after TAVI has been reported to have a 3.79-fold higher risk of 1-year post-procedural mortality (83). Paravalvular regurgitation should always be assessed by color flow Doppler interrogation of the aortic annulus immediately post procedure. Figure 22 shows an example of post-procedural transvalvular and paravalvular aortic regurgitation that is moderate in severity. Other possible complications are listed in Table 4.
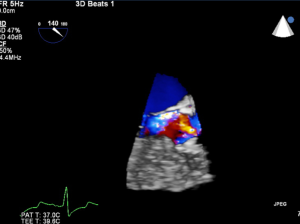
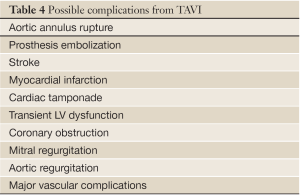
Full table
The benefits of 2D and 3D echocardiography for percutaneous AVR include a more accurate assessment of aortic annulus shape and size, visualization of catheters during the procedure, and efficacy of the procedure. Echocardiography is also used to evaluate post procedure complications. These benefits contribute to operator confidence during the procedure and successful implantation of the bioprosthetic valve.
Mitral Valve Repair with MitraClip
Mitral regurgitation (MR) is a progressive disease associated with left ventricular dysfunction and congestive heart failure. Patients with severe MR have a 5-year survival rate of 40%. If untreated, symptomatic patients may have a yearly death rate of greater than 5% (84). Surgery is recommended for symptomatic patients with severe MR or asymptomatic patients with evidence of left ventricular dysfunction (73). Mortality rates for mitral valve (MV) surgery have been reported to range from 1-2% for low-risk, young patients to 25% for high-risk, elderly patients undergoing MV replacement (85,86). In 2001, Alfieri et al. developed a modified, surgical MV repair technique which involves suturing the middle scallops of the anterior and posterior MV leaflets at the origin of the regurgitant jet to create a double-orifice MV (87). This was mainly for degenerative MR and the procedure is usually performed with an annuloplasty ring. In 2003, percutaneous MV repair was introduced with the first MitraClip implantation (88). This is an endovascular approach that approximates the anterior and posterior MV leaflets creating a double-orifice MV with a mechanical implant, the MitraClip (MitraClip System, Abbott Vascular Structural Heart, Menlo Park, CA). A recent study observed that the procedure was associated with increased safety, had similar improvements in clinical outcomes, and similar rates of reduction in MR at 12 and 24 months when compared with conventional surgery (89). The MitraClip implant may benefit patients who have met several important inclusion and exclusion criteria prior to clip placement (Table 5 and Figure 23A-D).
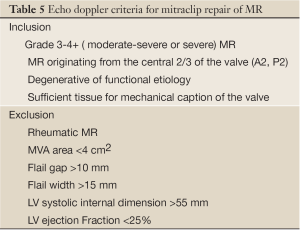
Full table
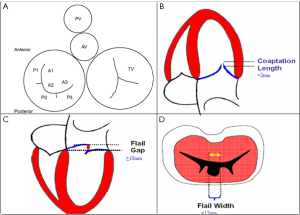
To optimize communication regarding MV morphology and anatomy among echocardiologists and interventional cardiologists, we use the classification of Carpentier (87). As shown in Figure 23A, we use the Carpentier nomenclature to describe the MV in the surgical view when using 3D TEE imaging. Although 2D TEE is helpful to assess MV morphology, 3D provides important additional information and details regarding morphology. For successful MV clip repair, the structural abnormalities and MR needs to be central. With degenerative MR due to prolapse or flail, the abnormality has to be limited to the central portion of the valve at the anterior leaflet (A2) and posterior leaflet (P2). Three-dimensional TEE, which can be done in live 3D TEE, Zoom mode or full volume imaging, provides an accurate en face anatomic definition of the MV. As demonstrated in Figure 24A, 2D, TEE can be misleading as to which scallop is prolapsed. In this 5-chamber view, the 2D TEE appears to show prolapse of the posterior lateral scallop or P1 prolapse, whereas on 3D TEE, the prolapse is central (P2), as seen in Figure 24B.
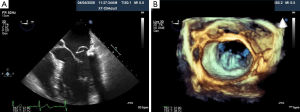
We have found that 3D TEE clarifies the site of MV prolapse in cases that are ambiguous by 2D TEE. To date, we have screened more than 900 patients for MitraClip, and currently, 125 patients have had MV clip repair at our institution (Cedars-Sinai Medical Center, Los Angeles, CA). Insufficient MR severity is the most frequent cause of exclusion from the trial. However, detection of lateral (A1 or P1) or medial (A3 or P3) prolapse on the screening 3D TEE may occur that was not clearly seen by 2D TEE. As shown in Figure 25A-D, the flail scallop appears to be the middle scallop (P2) on 2D TEE, but on 3D TEE, the flail is actually in both the middle and medial scallops (P2 and P3, respectively). Thus, this patient did not meet the criteria for MitraClip as the MR was due to a non-central MV leaflet prolapse and flail.
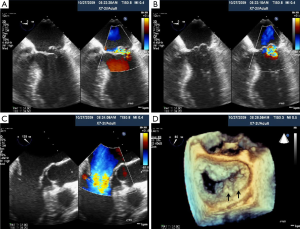
The MitraClip System is a catheter-based device that is inserted percutaneously via the femoral vein to the right atrium. Table 6 summarizes the steps of the procedure. As demonstrated in Figure 26A-C. X-plane view is used for trans-septal punctures to identify simultaneously the site of puncture from a bicaval and aortic short axis view. This allows confirmation of the puncture site relative to the fossa ovalis, in addition to its relationship to the aorta. Before the trans-septal puncture, the distance of the trans-septal needle in relation to the plane of the mitral valve is assessed with a 4-chamber view. The puncture site should be more superior along the intra-atrial septum so that there is more distance between the trans-septal catheter and the plane of the MV annulus; this is preferred for MV prolapse, especially bi-leaflet prolapse. After the MitraClip-guided catheter enters the left atrium, the guide catheter should be oriented towards the mitral valve apparatus,as shown in Figure 27A,B. Real-time 3D TEE imaging helps to orient the catheter in a way not possible by 2D TEE. The alignment and directionality of the catheter can be evaluated in the three dimensions of the heart. Three-dimensional TEE can also identify the locations of the guide catheter, the device, mitral valve, and left atrial appendage. Two-dimensional TEE can visualize the advancement of the MitraClip towards the MV; however, clip orientation within the LA can be problematic. The MitraClip should be located at the mid-portion of the MV (A2 and P2 segments) and oriented perpendicularly to the line of MV coaptation prior to the entry into the LV. With 3D guidance, as shown in Figure 28A-D, the clip can be directed to the mid-portion of the MV and adjusted perpendicularly to the line of MV coaptation. Once in the ideal position, the MitraClip is advanced into the LV. Two-dimensional TEE trans-gastric view can adequately assess the MitraClip orientation perpendicular to the line of MV coaptation within the LV, however this can be difficult, as shown in Figure 29A. However, 3D MitraClip orientation in the LV can often be obtained, as seen in Figure 29B.
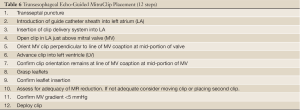
Full table
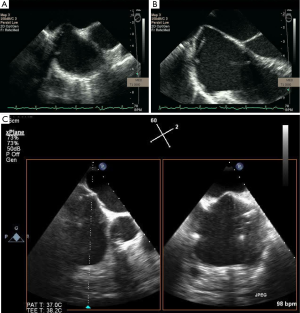
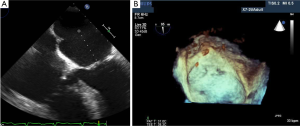
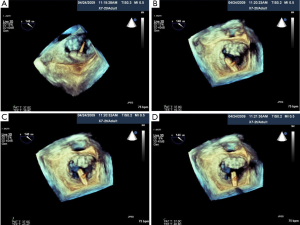
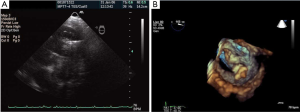
Next, the operator is set to capture the MV in the mid-portion of the MV leaflets. This step is generally guided by 2D TEE from the long axis view that shows the A2 P2 segments (angle 110°-140°) or combining with a 3D system X-plane view where the operator has a long axis view and a 2D bi-commissural view (60°-90°). This enables the operator to image P1-3 segments as well as A2 (Figure 30A,B). After MV leaflet capture, 2D TEE is used to assess leaflet insertion with multiple views including 4 chamber, bicommissural (2 chamber), and long axis (3 chamber) views. Three-dimensional TEE provides important adjunctive information regarding MR, such as identifying if there is more than 1 MR jet, which can sometimes be missed by 2D imaging. More interrogation with 2D color and spectral Doppler is performed if a second or third regurgitant jet is found. If a second clip is needed, 3D TEE color Doppler is helpful in identifying the site of the largest jet as well as the jet origin. After successful leaflet capture and satisfactory reduction of MR, Doppler is used to assess residual gradient across the MV to ensure the absence of mitral stenosis. A gradient of 5mmHg would be considered unacceptable and a gradient of 3 or 4 mmHg might preclude from using a second MitraClip. As Figure 31 shows, 3D TEE is useful after the deployment of MitraClip to evaluate the size, shape, and symmetry of the dual mitral orifices.
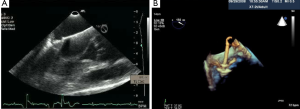
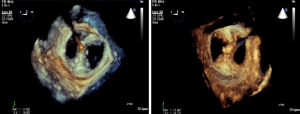
To date, over 3,000 MitraClip procedures have been performed worldwide and no intra-procedural mortality has been reported. Of the 128 cases performed in our institution, the post-procedure complications include 1 cardiac tamponade, 1 hemothorax, and 1 large right to left shunt. Both the pericardial tamponade and hemothorax cases were drained without complications and each had successful MitraClip placement. The case with severe right to left shunting across the atrial septum after the trans-septal puncture was associated with severe tricuspid regurgitation. An ASD closure device (Amplatzer) was used to close the shunt. Thus, if a patient has severe tricuspid regurgitation, especially in the setting of pulmonary hypertension, there may be a higher risk of developing a substantial right to left shunt across the atrial puncture site.
Percutaneous mitral valve repair with the MitraClip System has shown to be a promising method for a noninvasive treatment of severe MR. Real-time 3D TEE has been shown to be beneficial and is useful for optimal guidance of the MitraClip procedure (90). The addition of 3D TEE with 2D TEE has been reported to improve confidence of the echo interpretation (P<0.001) (91). In our experience, 3D enhances the confidence for the assessment of mitral valve morphology, localization of guiding catheters, and MitraClip placement. In addition, RT 3D TEE was associated with reduction in procedure time by 40 minutes (P=0.35).
Conclusion
In conclusion, echocardiographic guidance and monitoring of interventional procedures has transformed cardiovascular therapeutics as demonstrated in this article. Echo is not only useful but increases the safety of percutaneous access procedures for arterial and venous access. It has been found to enhance the safety of both pericardiocentesis and myocardial biopsy by allowing the operator to identify the location of catheters and avoiding unnecessary complications. Recent advances in cardiovascular catheter technology have seen the development of percutaneous valves, as well as valve repair devices such as the MitraClip. Current data demonstrates echo guidance of transcatheter procedures of valve interventions improves device delivery and patient outcomes.
Acknowledgements
Disclosure: The authors declare no conflict of interest.
References
- McGee DC, Gould MK. Preventing complications of central venous catheterization. N Engl J Med 2003;348:1123-33. [PubMed]
- Hind D, Calvert N, McWilliams R, et al. Ultrasonic locating devices for central venous cannulation: meta-analysis. BMJ 2003;327:361. [PubMed]
- Shojania KG, Duncan BW, McDonald KM, et al. Making health care safer: a critical analysis of patient safety practices. Evid Rep Technol Assess (Summ) 2001;i-x,1-668. [PubMed]
- Merrer J, De Jonghe B, Golliot F, et al. Complications of femoral and subclavian venous catheterization in critically ill patients: a randomized controlled trial. JAMA 2001;286:700-7. [PubMed]
- Mansfield PF, Hohn DC, Fornage BD, et al. Complications and failures of subclavian-vein catheterization. N Engl J Med 1994;331:1735-8. [PubMed]
- Troianos CA, Jobes DR, Ellison N. Ultrasound-guided cannulation of the internal jugular vein. A prospective, randomized study. Anesth Analg 1991;72:823-6. [PubMed]
- Mallory DL, McGee WT, Shawker TH, et al. Ultrasound guidance improves the success rate of internal jugular vein cannulation. A prospective, randomized trial. Chest 1990;98:157-60. [PubMed]
- Denys BG, Uretsky BF, Reddy PS. Ultrasound-assisted cannulation of the internal jugular vein. A prospective comparison to the external landmark-guided technique. Circulation 1993;87:1557-62. [PubMed]
- Oguzkurt L, Tercan F, Kara G, et al. US-guided placement of temporary internal jugular vein catheters: immediate technical success and complications in normal and high-risk patients. Eur J Radiol 2005;55:125-9. [PubMed]
- Land RE. The relationship of the left subclavian vein to the clavicle: practical considerations pertinent to the percutaneous catheterization of the subclavian vein. J Thorac Cardiovasc Surg 1972;63:564-8. [PubMed]
- Denys BG, Uretsky BF. Anatomical variations of internal jugular vein location: impact on central venous access. Crit Care Med 1991;19:1516-9. [PubMed]
- Randolph AG, Cook DJ, Gonzales CA, et al. Ultrasound guidance for placement of central venous catheters: a meta-analysis of the literature. Crit Care Med 1996;24:2053-8. [PubMed]
- Leung J, Duffy M, Finckh A. Real-time ultrasonographically-guided internal jugular vein catheterization in the emergency department increases success rates and reduces complications: a randomized, prospective study. Ann Emerg Med 2006;48:540-7. [PubMed]
- Turker G, Kaya FN, Gurbet A, et al. Internal jugular vein cannulation: an ultrasound-guided technique versus a landmark-guided technique. Clinics (Sao Paulo) 2009;64:989-92. [PubMed]
- Calvert N, Hind D, McWilliams RG, et al. The effectiveness and cost-effectiveness of ultrasound locating devices for central venous access: a systematic review and economic evaluation. Health Technol Assess 2003;7:1-84. [PubMed]
- Turi ZG. An evidence-based approach to femoral arterial access and closure. Rev Cardiovasc Med 2008;9:7-18. [PubMed]
- Hamel WJ. Femoral artery closure after cardiac catheterization. Crit Care Nurse 2009;29:39-46; quiz 47. [PubMed]
- Chandrasekar B, Doucet S, Bilodeau L, et al. Complications of cardiac catheterization in the current era: a single-center experience. Catheter Cardiovasc Interv 2001;52:289-95. [PubMed]
- Babu SC, Piccorelli GO, Shah PM, et al. Incidence and results of arterial complications among 16,350 patients undergoing cardiac catheterization. J Vasc Surg 1989;10:113-6. [PubMed]
- Johnston KW. Femoral and popliteal arteries: reanalysis of results of balloon angioplasty. Radiology 1992;183:767-71. [PubMed]
- Kinnaird TD, Stabile E, Mintz GS, et al. Incidence, predictors, and prognostic implications of bleeding and blood transfusion following percutaneous coronary interventions. Am J Cardiol 2003;92:930-5. [PubMed]
- Sherev DA, Shaw RE, Brent BN. Angiographic predictors of femoral access site complications: implication for planned percutaneous coronary intervention. Catheter Cardiovasc Interv 2005;65:196-202. [PubMed]
- Resnic FS, Arora N, Matheny M, et al. A cost-minimization analysis of the angio-seal vascular closure device following percutaneous coronary intervention. Am J Cardiol 2007;99:766-70. [PubMed]
- Ndrepepa G, Berger PB, Mehilli J, et al. Periprocedural bleeding and 1-year outcome after percutaneous coronary interventions: appropriateness of including bleeding as a component of a quadruple end point. J Am Coll Cardiol 2008;51:690-7. [PubMed]
- Fuchs S, Kornowski R, Teplitsky I, et al. Major bleeding complicating contemporary primary percutaneous coronary interventions-incidence, predictors, and prognostic implications. Cardiovasc Revasc Med 2009;10:88-93. [PubMed]
- Farouque HM, Tremmel JA, Raissi Shabari F, et al. Risk factors for the development of retroperitoneal hematoma after percutaneous coronary intervention in the era of glycoprotein IIb/IIIa inhibitors and vascular closure devices. J Am Coll Cardiol 2005;45:363-8. [PubMed]
- Ellis SG, Bhatt D, Kapadia S, et al. Correlates and outcomes of retroperitoneal hemorrhage complicating percutaneous coronary intervention. Catheter Cardiovasc Interv 2006;67:541-5. [PubMed]
- Tiroch KA, Arora N, Matheny ME, et al. Risk predictors of retroperitoneal hemorrhage following percutaneous coronary intervention. Am J Cardiol 2008;102:1473-6. [PubMed]
- Kim D, Orron DE, Skillman JJ, et al. Role of superficial femoral artery puncture in the development of pseudoaneurysm and arteriovenous fistula complicating percutaneous transfemoral cardiac catheterization. Cathet Cardiovasc Diagn 1992;25:91-7. [PubMed]
- Wacker F, Wolf KJ, Fobbe F. Percutaneous vascular access guided by color duplex sonography. Eur Radiol 1997;7:1501-4. [PubMed]
- Dudeck O, Teichgraeber U, Podrabsky P, et al. A randomized trial assessing the value of ultrasound-guided puncture of the femoral artery for interventional investigations. Int J Cardiovasc Imaging 2004;20:363-8. [PubMed]
- Schnyder G, Sawhney N, Whisenant B, et al. Common femoral artery anatomy is influenced by demographics and comorbidity: implications for cardiac and peripheral invasive studies. Catheter Cardiovasc Interv 2001;53:289-95. [PubMed]
- Fitts J, Ver Lee P, Hofmaster P, et al. Fluoroscopy-guided femoral artery puncture reduces the risk of PCI-related vascular complications. J Interv Cardiol 2008;21:273-8. [PubMed]
- Garrett PD, Eckart RE, Bauch TD, et al. Fluoroscopic localization of the femoral head as a landmark for common femoral artery cannulation. Catheter Cardiovasc Interv 2005;65:205-7. [PubMed]
- Huggins CE, Gillespie MJ, Tan WA, et al. A prospective randomized clinical trial of the use of fluoroscopy in obtaining femoral arterial access. J Invasive Cardiol 2009;21:105-9. [PubMed]
- Abu-Fadel MS, Sparling JM, Zacharias SJ, et al. Fluoroscopy vs. traditional guided femoral arterial access and the use of closure devices: a randomized controlled trial. Catheter Cardiovasc Interv 2009;74:533-9. [PubMed]
- Jacobi JA, Schussler JM, Johnson KB. Routine femoral head fluoroscopy to reduce complications in coronary catheterization. Proc (Bayl Univ Med Cent) 2009;22:7-8. [PubMed]
- Seto AH, Abu-Fadel MS, Sparling JM, et al. Real-time ultrasound guidance facilitates femoral arterial access and reduces vascular complications: FAUST (Femoral Arterial Access With Ultrasound Trial). JACC Cardiovasc Interv 2010;3:751-8. [PubMed]
- Oh JK, Seward JB, Tajik AJ. The Echo Manual. 3 ed. PA: Lippincott, Williams & Wilkins;2006.
- Tsang TS, Freeman WK, Sinak LJ, et al. Echocardiographically guided pericardiocentesis: evolution and state-of-the-art technique. Mayo Clin Proc 1998;73:647-52. [PubMed]
- Tsang TS, Enriquez-Sarano M, Freeman WK, et al. Consecutive 1127 therapeutic echocardiographically guided pericardiocenteses: clinical profile, practice patterns, and outcomes spanning 21 years. Mayo Clin Proc 2002;77:429-36. [PubMed]
- Tsang TS, Seward JB, Barnes ME, et al. Outcomes of primary and secondary treatment of pericardial effusion in patients with malignancy. Mayo Clin Proc 2000;75:248-53. [PubMed]
- Tsang TS, Barnes ME, Hayes SN, et al. Clinical and echocardiographic characteristics of significant pericardial effusions following cardiothoracic surgery and outcomes of echo-guided pericardiocentesis for management: Mayo Clinic experience, 1979-1998. Chest 1999;116:322-31. [PubMed]
- Silvestry FE, Kerber RE, Brook MM, et al. Echocardiography-guided interventions. J Am Soc Echocardiogr 2009;22:213-31; quiz 316-7. [PubMed]
- Rafique AM, Patel N, Biner S, et al. Frequency of Recurrence of Pericardial Tamponade in Patients With Extended Versus Nonextended Pericardial Catheter Drainage. Am J Cardiol 2011;108:1820-5. [PubMed]
- Cho BC, Kang SM, Kim DH, et al. Clinical and echocardiographic characteristics of pericardial effusion in patients who underwent echocardiographically guided pericardiocentesis: Yonsei Cardiovascular Center experience, 1993-2003. Yonsei Med J 2004;45:462-8. [PubMed]
- Tsang TS, El-Najdawi EK, Seward JB, et al. Percutaneous echocardiographically guided pericardiocentesis in pediatric patients: evaluation of safety and efficacy. J Am Soc Echocardiogr 1998;11:1072-7. [PubMed]
- Tsang TS, Freeman WK, Barnes ME, et al. Rescue echocardiographically guided pericardiocentesis for cardiac perforation complicating catheter-based procedures. The Mayo Clinic experience. J Am Coll Cardiol 1998;32:1345-50. [PubMed]
- Williams RL, Clark T, Blume CD. Clinical experience with a new combination formulation of triamterene and hydrochlorothiazide (Maxzide) in patients with mild to moderate hypertension. Am J Med 1984;77:62-6. [PubMed]
- Blomström-Lundqvist C, Noor AM, Eskilsson J, et al. Safety of transvenous right ventricular endomyocardial biopsy guided by two-dimensional echocardiography. Clin Cardiol 1993;16:487-92. [PubMed]
- Strachovsky G, Zeldis SM, Katz S, et al. Two-dimensional echocardiographic monitoring during percutaneous endomyocardial biopsy. J Am Coll Cardiol 1985;6:609-11. [PubMed]
- Piérard L, El Allaf D, D’Orio V, et al. Two-dimensional echocardiographic guiding of endomyocardial biopsy. Chest 1984;85:759-62. [PubMed]
- Fiorelli AI, Coelho GB, Santos RH, et al. Successful endomyocardial biopsy guided by transthoracic two-dimensional echocardiography. Transplant Proc 2011;43:225-8. [PubMed]
- Williams GA, Kaintz RP, Habermehl KK, et al. Clinical experience with two-dimensional echocardiography to guide endomyocardial biopsy. Clin Cardiol 1985;8:137-40. [PubMed]
- Han J, Park Y, Lee H, et al. Complications of 2-D echocardiography guided transfemoral right ventricular endomyocardial biopsy. J Korean Med Sci 2006;21:989-94. [PubMed]
- Bonhoeffer P, Boudjemline Y, Qureshi SA, et al. Percutaneous insertion of the pulmonary valve. J Am Coll Cardiol 2002;39:1664-9. [PubMed]
- Frigiola A, Redington AN, Cullen S, et al. Pulmonary regurgitation is an important determinant of right ventricular contractile dysfunction in patients with surgically repaired tetralogy of Fallot. Circulation 2004;110:II153-7. [PubMed]
- Gatzoulis MA, Balaji S, Webber SA, et al. Risk factors for arrhythmia and sudden cardiac death late after repair of tetralogy of Fallot: a multicentre study. Lancet 2000;356:975-81. [PubMed]
- Carvalho JS, Shinebourne EA, Busst C, et al. Exercise capacity after complete repair of tetralogy of Fallot: deleterious effects of residual pulmonary regurgitation. Br Heart J 1992;67:470-3. [PubMed]
- McElhinney DB, Hellenbrand WE, Zahn EM, et al. Short- and medium-term outcomes after transcatheter pulmonary valve placement in the expanded multicenter US melody valve trial. Circulation 2010;122:507-16. [PubMed]
- Stark J, Bull C, Stajevic M, et al. Fate of subpulmonary homograft conduits: determinants of late homograft failure. J Thorac Cardiovasc Surg 1998;115:506-14; discussion 514-6. [PubMed]
- Gerestein CG, Takkenberg JJ, Oei FB, et al. Right ventricular outflow tract reconstruction with an allograft conduit. Ann Thorac Surg 2001;71:911-7; discussion 917-8. [PubMed]
- Lurz P, Gaudin R, Taylor AM, et al. Percutaneous pulmonary valve implantation. Semin Thorac Cardiovasc Surg Pediatr Card Surg Annu 2009;112-7. [PubMed]
- Momenah TS, El Oakley R, Al Najashi K, et al. Extended application of percutaneous pulmonary valve implantation. J Am Coll Cardiol 2009;53:1859-63. [PubMed]
- Zahn EM, Hellenbrand WE, Lock JE, et al. Implantation of the melody transcatheter pulmonary valve in patients with a dysfunctional right ventricular outflow tract conduit early results from the u.s. Clinical trial. J Am Coll Cardiol 2009;54:1722-9. [PubMed]
- Asoh K, Walsh M, Hickey E, et al. Percutaneous pulmonary valve implantation within bioprosthetic valves. Eur Heart J 2010;31:1404-9. [PubMed]
- Lurz P, Bonhoeffer P, Taylor AM. Percutaneous pulmonary valve implantation: an update. Expert Rev Cardiovasc Ther 2009;7:823-33. [PubMed]
- Sridharan S, Coats L, Khambadkone S, et al. Images in cardiovascular medicine. Transcatheter right ventricular outflow tract intervention: the risk to the coronary circulation. Circulation 2006;113:e934-5. [PubMed]
- Bonhoeffer P, Boudjemline Y, Saliba Z, et al. Percutaneous replacement of pulmonary valve in a right-ventricle to pulmonary-artery prosthetic conduit with valve dysfunction. Lancet 2000;356:1403-5. [PubMed]
- Lindroos M, Kupari M, Heikkilä J, et al. Prevalence of aortic valve abnormalities in the elderly: an echocardiographic study of a random population sample. J Am Coll Cardiol 1993;21:1220-5. [PubMed]
- Faggiano P, Antonini-Canterin F, Baldessin F, et al. Epidemiology and cardiovascular risk factors of aortic stenosis. Cardiovasc Ultrasound 2006;4:27. [PubMed]
- Faggiano P, Aurigemma GP, Rusconi C, et al. Progression of valvular aortic stenosis in adults: literature review and clinical implications. Am Heart J 1996;132:408-17. [PubMed]
- Bonow RO, Carabello BA, Chatterjee K, et al. 2008 focused update incorporated into the ACC/AHA 2006 guidelines for the management of patients with valvular heart disease: a report of the American College of Cardiology/American Heart Association Task Force on Practice Guidelines (Writing Committee to revise the 1998 guidelines for the management of patients with valvular heart disease). Endorsed by the Society of Cardiovascular Anesthesiologists, Society for Cardiovascular Angiography and Interventions, and Society of Thoracic Surgeons. J Am Coll Cardiol 2008;52:e1-142. [PubMed]
- Iung B, Cachier A, Baron G, et al. Decision-making in elderly patients with severe aortic stenosis: why are so many denied surgery? Eur Heart J 2005;26:2714-20. [PubMed]
- Biner S, Rafique AM, Goykhman P, et al. Prognostic value of E/E’ ratio in patients with unoperated severe aortic stenosis. JACC Cardiovasc Imaging 2010;3:899-907. [PubMed]
- Cribier A, Eltchaninoff H, Bash A, et al. Percutaneous transcatheter implantation of an aortic valve prosthesis for calcific aortic stenosis: first human case description. Circulation 2002;106:3006-8. [PubMed]
- Leon MB, Smith CR, Mack M, et al. Transcatheter aortic-valve implantation for aortic stenosis in patients who cannot undergo surgery. N Engl J Med 2010;363:1597-607. [PubMed]
- Poh KK, Levine RA, Solis J, et al. Assessing aortic valve area in aortic stenosis by continuity equation: a novel approach using real-time three-dimensional echocardiography. Eur Heart J 2008;29:2526-35. [PubMed]
- Messika-Zeitoun D, Serfaty JM, Brochet E, et al. Multimodal assessment of the aortic annulus diameter: implications for transcatheter aortic valve implantation. J Am Coll Cardiol 2010;55:186-94. [PubMed]
- Moss RR, Ivens E, Pasupati S, et al. Role of echocardiography in percutaneous aortic valve implantation. JACC Cardiovasc Imaging 2008;1:15-24. [PubMed]
- Otani K, Takeuchi M, Kaku K, et al. Assessment of the aortic root using real-time 3D transesophageal echocardiography. Circ J 2010;74:2649-57. [PubMed]
- Smith CR, Leon MB, Mack MJ, et al. Transcatheter versus surgical aortic-valve replacement in high-risk patients. N Engl J Med 2011;364:2187-98. [PubMed]
- Tamburino C, Capodanno D, Ramondo A, et al. Incidence and predictors of early and late mortality after transcatheter aortic valve implantation in 663 patients with severe aortic stenosis. Circulation 2011;123:299-308. [PubMed]
- Trichon BH, Felker GM, Shaw LK, et al. Relation of frequency and severity of mitral regurgitation to survival among patients with left ventricular systolic dysfunction and heart failure. Am J Cardiol 2003;91:538-43. [PubMed]
- Mehta RH, Eagle KA, Coombs LP, et al. Influence of age on outcomes in patients undergoing mitral valve replacement. Ann Thorac Surg 2002;74:1459-67. [PubMed]
- Nowicki ER, Birkmeyer NJ, Weintraub RW, et al. Multivariable prediction of in-hospital mortality associated with aortic and mitral valve surgery in Northern New England. Ann Thorac Surg 2004;77:1966-77. [PubMed]
- Alfieri O, Maisano F, De Bonis M, et al. The double-orifice technique in mitral valve repair: a simple solution for complex problems. J Thorac Cardiovasc Surg 2001;122:674-81. [PubMed]
- Condado JA, Vélez-Gimón M. Catheter-based approach to mitral regurgitation. J Interv Cardiol 2003;16:523-34. [PubMed]
- Feldman T, Foster E, Glower DD, et al. Percutaneous repair or surgery for mitral regurgitation. N Engl J Med 2011;364:1395-406. [PubMed]
- Altiok E, Becker M, Hamada S, et al. Real-time 3D TEE allows optimized guidance of percutaneous edge-to-edge repair of the mitral valve. JACC Cardiovasc Imaging 2010;3:1196-8. [PubMed]
- Biner S, Perk G, Kar S, et al. Utility of combined two-dimensional and three-dimensional transesophageal imaging for catheter-based mitral valve clip repair of mitral regurgitation. J Am Soc Echocardiogr 2011;24:611-7. [PubMed]