Hemodynamic indices in pulmonary hypertension: a narrative review
Introduction
The pulmonary circulation starts in the right ventricle (RV), moves into the pulmonary arteries (PA), and extends through the pulmonary veins into the left atrium. It includes a network of arteries, veins, and lymphatics whose main function is gas exchange. The pulmonary circulation is a low pressure and low resistance system; however, in certain conditions the mean pulmonary artery pressure (mPAP) increases above 20 mmHg, leading to the diagnosis of pulmonary hypertension (PH). PH is divided into 5 groups based on similar pathophysiological mechanisms, clinical presentation, hemodynamic characteristics, and therapeutic management (1). Group 1 PH or pulmonary arterial hypertension (PAH) is characterized by precapillary PH [pulmonary artery wedge pressure (PAWP) ≤15 mmHg and pulmonary vascular resistance (PVR) ≥3 Wood units (WU)] that can lead to right heart failure and death (2). Group 2 PH or PH resulting from left heart disease (PH-LHD) is characterized by postcapillary PH (PAWP >15 mmHg) and can be divided into 2 hemodynamic subgroups, isolated postcapillary PH (IpcPH) in which the PVR is <3 WU and combined pre- and post-capillary PH (CpcPH) in which the PVR is ≥3 WU (3).
Great emphasis has been placed on risk stratifying patients to guide treatment. Commonly used tools include the French PH network registry assessment which incorporates data from up to six variables, and the US Registry to Evaluate Early and Long-term PAH Disease Management (REVEAL) 2.0 risk equation that includes up to 13 variables (4-6). These tools use limited hemodynamic information including right atrial pressure (RAP), cardiac index (CI), and PVR. A variety of other hemodynamic indices that provide information on different aspects of the pulmonary circulation have been explored in PH, but not yet incorporated into clinical practice.
Through this narrative review, we attempt to outline relevant hemodynamic indices studied in PH (Figure 1), including their description, normal values, use, and limitations as they apply to improving both the pathophysiological understanding and prognostic assessment in PH. We present the following article in accordance with the Narrative Review reporting checklist (available at https://cdt.amegroups.com/article/view/10.21037/cdt-22-244/rc).
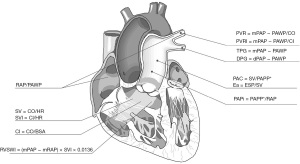
Methods
We performed a literature search on PubMed using the MeSH terms “pulmonary hypertension” with each of the indices outlined below. Additionally, we included only articles published in the English language between 1970 through 2021. References from these articles were also reviewed. The search methodology is also described in Table 1. A complete list of the indices described above can be found in Table 2. All the indices presented can be obtained during a regular right catheterization. We chose to include PA pressure-volume loops (PV loops) since there are methods to estimate the RV-arterial coupling using the hemodynamic data acquired during right heart catheterization (e.g., single beat method that uses a nonlinear extrapolation of early and late isovolumic portions of the RV pressure curve). The impact of provocative maneuvers (i.e., exhaled nitric oxide, fluids, and exercise) on these indices is beyond the scope of the manuscript.
Table 1
Items | Specification |
---|---|
Date of search | December 15th 2021 |
Databases and other sources searched | PubMed and references of selected articles |
Search terms used | Pulmonary hypertension and each of the indices outlined in the article |
Timeframe | 1970–2021 |
Inclusion and exclusion criteria | Articles in English language and human studies |
Selection process | Searched by 3 different authors independently after which a cross check was done to obtain consensus. Final check completed by faculty author |
Table 2
Index | Abbreviation | Formula | Parameter measured | Use in PH |
---|---|---|---|---|
Cardiac index | CI | CO/BSA | Right ventricular function (in patients with normal systolic and diastolic left ventricular function) | · Survival prognosis |
· Measurement of response to targeted therapy | ||||
Stroke volume | SV | CO/HR | Right ventricular function | · Prognosis of morbidity and mortality |
· Increases with exercise training | ||||
Stroke volume index | SVI | CI/HR | Right ventricular function | · Survival prognosis especially in SSc-PAH |
· Measurement of response to targeted therapy | ||||
Transpulmonary gradient | TPG | mPAP − PAWP | Pulmonary vascular constriction/remodeling | · Survival prognosis in PH-LHD |
· Survival prognosis in patients undergoing trans catheter aortic valve replacement | ||||
· Preoperative risk prognosticator of mortality post heart transplant | ||||
Diastolic pulmonary gradient | DPG | dPAP − PAWP | Pulmonary vascular constriction/remodeling | · Differentiate CpcPH and IpcPH in patients with PH-LHD |
Pulmonary vascular resistance | PVR | (mPAP − PAWP)/CO | Pulmonary vascular disease | · Differentiating CpcPH and IpcPH in patients with PH-LHD |
RV afterload (static) | · Survival prognosis | |||
· Measurement of response to targeted therapy | ||||
Pulmonary vascular resistance index | PVRI | (mPAP − PAWP)/CI | Pulmonary vascular disease | · Survival prognosis |
RV afterload (static) | · Diagnosis of precapillary PH in pediatric population | |||
Pulmonary artery compliance | PAC | SV/PAPP | Pulmonary artery distensibility | · Early diagnosis of PH in patients with normal PVR |
PAPP = sPAP − dPAP | RV afterload (dynamic) | · Survival prognosis | ||
· Predictor of mortality in PH-LHD (HFpEF and HFrEF) | ||||
· Response to therapy (IV and oral prostacyclin) in PAH | ||||
· Response to balloon angioplasty in CTEPH | ||||
Pulmonary effective arterial elastance | Ea | End systolic pressure/SV | RV afterload | · Prognostic marker in PH-LHD |
Ratio of right atrial pressure over pulmonary artery wedge pressure | RAP/PAWP | RAP/PAWP | Right ventricular failure | · Predictor of survival in PAH |
Pulmonary artery pulsatility index | PAPi | PAPP/RAP | Right ventricular function | · Survival prognosis |
· Predictor of RV failure in PAH | ||||
Right ventricular systolic work index | RVSWI | (mPAP − mRAP) × SVI × 0.0136 | Right ventricular work | · Survival prognosis in PAH and CTEPH |
PA pressure-volume loops | PV loops | End systolic elastance/Ea | RV-arterial coupling | · Early diagnosis of PAH and CTEPH |
· Evaluation of RV-PA coupling |
PH, pulmonary hypertension; CO, cardiac output; BSA, body surface area; HR, heart rate; CI, cardiac index; SSc-PAH, systemic sclerosis associated PAH; mPAP, mean pulmonary artery pressure; PAWP, pulmonary artery wedge pressure; LHD, left heart disease; dPAP, diastolic pulmonary artery pressure; CpcPH, combined pre- and post-capillary PH; IpcPH, isolated postcapillary PH; RV, right ventricle; SV, stroke volume; PAPP, pulmonary artery pulse pressure; sPAP, systolic pulmonary artery pressure; PVR, pulmonary vascular resistance; HFpEF, heart failure with preserved ejection fraction; HFrEF, heart failure with reduced ejection fraction; IV, intravenous; PAH, pulmonary artery hypertension; CTEPH, chronic thromboembolic pulmonary hypertension; mRAP, mean right atrial pressure; SVI, stroke volume index; PA, pulmonary artery.
Indices
CI
CI is the cardiac output (CO) normalized for body size and is obtained by dividing CO by body surface area (BSA) (7). CI is reflective of the global function of the RV (in patients with normal systolic and diastolic left ventricular function) and forms an integral component to assess the functionality of the cardiopulmonary unit. As PH worsens, the CI decreases, due to failure of the RV in the setting of a higher afterload. CI is a well-known predictor of outcomes in PH and the European Society of Cardiology/European Respiratory Society (ESC/ERS) guidelines assigned CI thresholds of ≥2.5 L/min/m2, 2–2.4 L/min/m2, and <2.0 L/min/m2 for patients at low (<5%), intermediate (5–10%) and high risk (>10%) of dying at 1-year (8).
Poor survival associated with a low CI (<2 L/min/m2) was initially described in patients enrolled in the Patient Registry for the Characterization of Primary Pulmonary Hypertension (9). Several other studies identified CI as a prognostic variable in PH (6,10-12). In addition, patients who improve CI with PAH-specific therapies have better survival (12,13). In a meta-analysis of trials studying the addition of prostacyclin analogues to targeted therapy for PAH, CI, and other prognostic markers such as New York Heart Association functional class and 6-minute walk distance (6MWD) were found to improve with the addition of prostacyclin analogues. However, no change in all-cause mortality was noted despite the improvement of CI (14). We described that mixed venous oxygen saturation was superior to thermodilution CI in predicting long-term mortality. A decrease in mixed venous oxygen saturation indicates that the CI (even if it is apparently adequate) is not sufficient to meet the tissue oxygen needs, hence there is an increase in oxygen extraction (15).
There are several limitations in using CI, particularly as it relates to the methodology used to measure it. The gold standard is the direct Fick method that requires the determination of resting oxygen consumption (VO2) and analysis of arterial and mixed venous blood to measure the oxygen saturation and hemoglobin, which are necessary for the calculation of oxygen content. The use of pulse oxygenation and prior hemoglobin determination introduces potential sources of error (16). Thermodilution is recommended by the 6th World Symposium on Pulmonary Hypertension (WSPH) when direct Fick methodology is not available because it can provide reliable measurements even in patients with low CO and/or severe tricuspid regurgitation (3); however, the wide limits of agreement (±1.96 SD of the differences) of up to 1 L/min/m2 between methods, impacts the risk group allocation proposed by ESC/ERS given the narrow CI band (2–2.5 L/min/m2) (17). Indirect Fick methodology, which estimatesVO2 based on a variety of formulae is not reliable and not recommended for routine CI determination (3,17). Current noninvasive methodologies to estimate CI, with the possible exception of acetylene and nitrous oxide rebreathing, are not precise enough to differentiate between ESC/ERS risk groups (18-23). Further technological developments are needed to precisely measure CI in a simple, non-invasive, and cost-effective manner in patients with PH (24).
Stroke volume (SV)
SV may be more accurate than CI in estimating RV function as it removes the compensatory heart rate (HR) response when CO is inappropriate to meet the body demands (SV = CO/HR). As PAH progresses the SV decreases; however, this is initially counterbalanced by an increase in HR; a compensatory mechanism that regulates the CI. Hence in the early stages, the CI may be numerically normal even when the SV is reduced.
In patients with idiopathic PAH (IPAH), SV at baseline and 1-year follow-up was a stronger predictor of mortality than CI (25). After a year of PH therapy, the increase in SV directly correlated with the 6MWD, and a 10 mL increase in SV was considered the minimal important difference (26). Treatment with epoprostenol increased the SV (41±11 mL) measured by cardiac magnetic resonance imaging with maximum improvement at 4 months after initiation of therapy, in the context of no significant changes in right ventricular end-diastolic volume and mass (27).
In patients with PAH, the SV correlates with World Health Organization functional class and its change during exercise was a predictor of morbidity and mortality (28-30). Additionally, a post hoc analysis of randomized control trials in patients with PAH and chronic thromboembolic pulmonary hypertension (CTEPH) showed that exercise training increased SV at 15 weeks by an average of 14.2 mL (31).
The accurate measurement of SV is challenging and not without pitfalls. The invasive measurement during right heart catheterization varies in accuracy depending on the methodology used (17). Fick methodology is the goal standard but requires metabolic cart determination, mixed venous and arterial blood gas oximetry (17). In addition, variations in the HR during CO determination may affect the calculation of the SV. Two-dimensional echocardiography has important limitations in assessing RV volumes because of its complex structure; a shortcoming that can be potentially overcome with continuous advances in three-dimensional echocardiography (32). Cardiac magnetic resonance imaging remains the gold standard methodology to assess SV since it can precisely determine diastolic and systolic RV volumes (25,33).
Stroke volume index (SVI)
SVI is calculated by dividing CI by HR or SV by BSA. The advantage of this index is the adjustment of SV by BSA since SV is influenced by body size, particularly fat-free body mass (34,35). SVI could allow for a more precise evaluation of the RV function in patients with PAH. In fact, an SVI, with <38 mL/m2 at first follow-up (3–4 months after starting PAH-specific therapy) was associated with significantly higher mortality in patients with PAH at intermediate risk by ESC/ERS guidelines (5,36).
SVI during vasoreactivity testing with inhaled nitric oxide in PAH could predict outcomes since patients with an SVI >39.9 mL/m2 had a 100% survival rate at 5 years (37). Some investigators have proposed using SVI rather than CI as a treatment target in PAH since a decrease in SVI was independently associated with death or lung transplantation (a drop of 10 mL/m2 in SVI led to a 28% increase) at the first follow-up right heart catheterization after initiation of PAH therapies (38).
SVI is a particularly useful prognostic indicator in patients with systemic sclerosis (SSc) associated PAH (SSc-PAH), in whom traditional hemodynamic indices like RAP, CI, and PVR showed limited utility in predicting survival in one study (39). Despite these results, the 6th WSPH recommends RAP and CI for prognostication in PAH, including patients with SSc-PAH. Patients with SSc-PAH have greater RV dysfunction and lower SV than patients with IPAH despite lower PA pressures and similar CI (40,41). In fact, SVI is a strong predictor of mortality in patients with SSc-PAH, since an SVI <30 mL/m2 carries a two-fold increased risk of death in these patients (42,43).
Transpulmonary gradient (TPG)
The TPG is calculated by subtracting the PAWP from the mPAP and higher values reflect pulmonary vascular constriction and/or remodeling. A TPG cutoff of >12 or ≤12 mmHg was used to distinguish IpcPH from CpcPH (44,45). As with mPAP, TPG is influenced by the same hemodynamic factors, including flow, resistance, and left heart filling pressures (46). Due to these limitations, TPG was removed, in favor of PVR (TPG/CO), as a tool to establish the different hemodynamic types of PH-LHD (3,8).
An elevated TPG has been linked with worse outcomes in patients with PH-LHD. A TPG ≥12 mmHg was associated with higher mortality and cardiac hospitalization in PH-LHD with an optimal cut-off of 16 mmHg (47). Other studies found an increase in the 30-day mortality in heart failure patients undergoing evaluation for heart transplantation with elevated TPG (≥12 or 15 mmHg, depending on the study), irrespectively of the PVR (48,49). An analysis using the United Network for Organ Sharing database also revealed longer hospital length of stay and higher mortality at 5 and 10 years post-heart transplantation in patients with preoperative TPG ≥15 mmHg (50). Given the increased morbidity-mortality of a high TPG (TPG ≥15 mmHg) or PVR ≥3 WU, heart transplantation guidelines recommend regular assessment of hemodynamics with pulmonary vasodilators (e.g., intravenous nitrates or inhaled nitric oxide) and inotropic agents (e.g., milrinone), to assess reversibility of the precapillary component in patients with CpcPH (51).
In patients with PH due to valvular heart disease, a higher TPG also predicted worse outcomes. Patients with high TPG (>12 mmHg) who underwent restrictive mitral annuloplasty for severe mitral regurgitation had worse outcomes (all-cause mortality and readmission for heart failure) (52). Similarly, patients with markers of precapillary PH (including an elevated TPG and PVR) had worse survival after transcatheter aortic valve replacement (53).
Diastolic pulmonary gradient (DPG)
DPG is calculated by subtracting PAWP from the diastolic pulmonary artery pressure (dPAP). At a constant SV, an increase of PAWP has a more pronounced effect on systolic pulmonary artery pressure (sPAP) and mPAP than dPAP, which might be explained by a lower sensitivity to vessel distensibility (45,46). Based on this assumption some authors advocate DPG in favor of TPG. Recent investigations demonstrated that both DPG and TPG depend on PAWP and CO (absolute increase in DPG with CO is 62% of the TPG increase with CO), blurring the potential advantages of one calculation versus the other (46,54). The fifth WSPH included DPG ≥7 mmHg along with PVR (45,55); however, the 6th WSPH eliminated DPG and only kept PVR for differentiating IpcPH from CpcPH (3).
DPG was initially used to differentiate cardiac versus pulmonary causes of acute respiratory failure (56). Initially, DPG was proposed as a good hemodynamic indicator to differentiate IpcPH from CpcPH (46). A DPG of ≥7 mmHg showed the best combination of sensitivity and specificity to be an independent predictor of survival in patients with PH-LHD (55). In addition, patients with DPG ≥7 mmHg had worse vascular medial hypertrophy, intimal and adventitial fibrosis, and more occluded vessels (55). A metanalysis revealed that PVR, pulmonary artery compliance (PAC), and DPG are associated with survival in patients with PH-LHD (57).
Nevertheless, the prognostic value of DPG in PH-LHD remains controversial and was not associated with survival in a relatively large retrospective multicenter study of patients with PH due to heart failure with reduced ejection fraction or heart failure with preserved ejection fraction (58-64). These negative results could in part be explained by the negative numeric values of DPG observed in some studies that reflect inconsistent pressure measurements across different parts of the respiratory cycle (e.g., dPAP averaged across the respiratory cycles while PAWP measured at end-expiration), a falsely lower dPAP by including a ringing artifact, or a higher PAWP due to under/over wedging or the inclusion of V waves in the measurement (65-67).
PVR
PVR is a static hemodynamic index based on Poiseuille’s law and is calculated as (mPAP − PAWP)/CO. PVR is essential for diagnosing pre-capillary PH as well as CpcPH (3). PVR has also been shown to provide prognostic information in different forms of PH and is a part of the US REVEAL 2.0 score for risk assessment (a PVR <5 WU reduces the score by one point) (6,68). A meta-regression analysis of 21 trials showed that changes in PVR were independently predictive of adverse clinical events, particularly total mortality (69).
While a PVR of ≥3 WU is currently used to define precapillary PH, this threshold is not based on evidence regarding the upper limit of normal which is <2 WU (70). In fact, a PVR >2.2 WU was associated with all-cause mortality in patients undergoing right heart catheterization; and a PVR between 2.2 and 3 WU may represent early pulmonary vascular disease (68,70,71). Patients with SSc demonstrated reduced long-term survival when the PVR was ≥2 WU (71). Interestingly, a retrospective study from Australia and New Zealand showed improvement in the 6MWD and New York Heart Association functional class in patients with PH (mPAP ≥25 mmHg and PAWP ≤15 mmHg) without a PVR >3 WU, who received PAH therapy (72).
Pulmonary vascular resistance index (PVRI)
The PVRI differs from PVR in that it takes into account the body habitus of the patient. It is calculated as follows: PVRI = (mPAP − PAWP)/CI or PVRI = PVR × BSA. Despite potential advantages, PVRI has not been routinely adopted in the adult population. A PVRI value of ≥30 WU·m2 (or mmHg/L/min/m2) was a predictor of 3-year survival in patients with PAH (73). A recent study by our group showed that both PVR and PVRI were associated with disease severity in PAH, and both indices were risk factors for first PAH hospitalization and death or death or lung transplant, without any apparent superiority between them (74). Interestingly, we noted an inverse correlation between PVR and body weight which can be explained by increased CO seen in obese individuals (75,76). The current recommendations by the 6th WSPH are to use the PVR for the diagnosis of PH in adults (77). However, the guidelines for PH in pediatric patients, recommend using PVRI given the wide variations in BSA in children (78,79).
Pulmonary arterial compliance (PAC)
Unlike the static index PVR, PAC measures arterial distensibility and therefore provides information about the pulsatile load on the RV. PAC and PVR exhibit an inverse hyperbolic relationship and in the early stages of the disease, the PAC might decrease even with minimal changes in PVR (80,81). A simplified approximation of PAC uses the ratio of SV and pulmonary artery pulse pressure (PAPP) [PAC = SV/(sPAP − dPAP)]. This simplified formula can overestimate PAC since the true increase in volume in the arterial system is difficult to measure, since the actual change in volume is lower than the SV (82,83). This determination can be improved by simultaneously acquiring invasive pressure measurements and magnetic resonance flow data (82).
PAC provides prognostic information in IPAH and SSc-PAH (42,84-86). In patients with IPAH, the 4-year survival was 100% in patients with PAC of >2 mL/mmHg and <40% in those with PAC of <0.81 mL/mmHg (84). Interestingly, adding other clinical or hemodynamic variables collected in the study did not provide further prognostic value than that provided by PAC (84). In patients with systemic lupus erythematosus associated PAH, a PAC <1.39 mL/mmHg was associated with all-cause mortality and clinical worsening (87). In patients with PH-LHD, a PAC of <1.1 mL/mmHg was a better predictor of mortality than DPG, TPG, or PVR (88). Furthermore, a PAC of <2.15 mL/mmHg was an important predictor of mortality in patients with PH-LHD, both in patients with IpcPH or CpcPH (89).
Treatment for PAH with prostacyclin analogues (intravenous or inhaled) was associated with an improvement in PAC that was strongly influenced by the change in PVR (90). Treatment with the oral prostacyclin treprostinil also led to improvements in PAC in patients with PAH, as the PAC increased from 1.5 to 1.9 mL/mmHg at 24 weeks of treatment (91). Balloon pulmonary angioplasty improved PAC in patients with CTEPH from 1.03 to 1.64 mL/mmHg, a change that may have contributed to the concomitant improvement in functional capacity (92).
Pulmonary effective arterial elastance (Ea)
Ea is computed by calculating the ratio of RV end-systolic pressure (ESP) to SV. It is a comprehensive hemodynamic parameter that incorporates resistive, pulsatile, and passive components of total RV afterload. PVR and PAC do not incorporate impedance which is related to the vascular storage and blood inertia of the proximal pulmonary vessels.
In PH-LHD with heart failure with reduced ejection fraction or heart failure with preserved ejection fraction, investigators used sPAP in place of right ventricular end systolic pressure (RVESP) to calculate the elastance (93,94). The median Ea was 1.036 mmHg/mL and patients with values above the median had worse mortality. Ea and PAC were more strongly associated with RV dysfunction and were consistently better predictors of mortality than TPG and PVR in patients with PH-LHD. Interestingly, Ea and PAC remained predictive of mortality in a subgroup of patients with normal resistive load (64).
RAP/PAWP
RAP/PAWP is another index that can serve to evaluate RV failure. As long as RV function is maintained, RAP remains lower than PAWP. When the RV starts failing, the RAP increases “out of proportion” to the PAWP, thus raising the RAP/PAWP ratio. Fluid overload and/or other organ dysfunction (left ventricular, kidney, and/or liver failure) would be expected to raise the RAP, but the RAP/PAWP ratio should remain <1 in the absence of RV failure.
In PAH, the RAP/PAWP ratio was a strong predictor of survival and performed better than RAP when not indexed for PAWP and other hemodynamic variables. A RAP/PAWP value of ≥1 provided the best combination of sensitivity and specificity (95). RAP/PAWP ratio is higher in precapillary PH and CpcPH as compared to IpcPH. A ratio of ≥1 was associated with smaller left atrial volume, decreased tricuspid annular plane systolic excursion (TAPSE), and a higher RV/left ventricle size ratio (96).
An increased RAP/PAWP ratio was associated with higher PVR, reduced RV function, and worse outcomes in patients with advanced systolic left heart failure (97). Similarly, a higher RAP/PAWP was associated with renal failure and mortality in acute decompensated systolic heart failure (98). In addition, RAP/PAWP ratio may help to identify patients at high risk of developing right ventricular failure and mortality after the implantation of a left ventricular assist device (99). RAP/PAWP ratio increased immediately following left ventricular assist device (LVAD) implantation, then decreased for a short period followed by a gradual increase in the long-term that may represent the change in the RV function over time (100).
Pulmonary artery pulsatility index (PAPi)
PAPi is an indirect measure of RV function and is defined as the ratio of PAPP to RAP [PAPi = (sPAP − dPAP)/RAP]. PAPi reflects the adaptive response of the RV to increased afterload (RV to pulmonary artery coupling) with implications for prognosis and survival (101,102). As previously described PAC = SV/(sPAP − dPAP); and by rearranging the terms, PAPP = SV/PAC. Based on this formula, the PAPP component in PAPi is affected by changes in SV and/or PVR (which has a hyperbolic relationship with PAC). In PAH, if the SV is maintained but the PVR is elevated, PAPP may not accurately reflect disease severity and hence PAPi is higher in PAH than in other conditions. As PAH progresses and the SV decreases, and RAP increases the PAPi would be lower.
PAPi was first described in 2012 in patients with acute right ventricular myocardial infarction and since then its use has expanded to advanced heart failure, cardiogenic shock, left ventricular assist device management, heart transplantation, and PAH (103-107). In PAH, a lower PAPi (<5.3) at diagnosis was associated with greater age, lower sPAP, higher mean RAP (mRAP), and higher mortality despite age stratification; an association predominantly driven by the mRAP (108). In another PAH study, patients with a lower PAPi (median 5.8) had worse survival in a multivariable model (101).
Right ventricular systolic work index (RVSWI)
RVSWI is used to quantify the amount of work required by the RV for ejecting blood in each cardiac cycle when adjusted for BSA. RVSWI is calculated as (mPAP − mRAP) × SVI × 0.0136. Hence, RVSWI takes into consideration both the pressure and SV of the RV. As the RV adapts to increased PA pressures in PH, while maintaining an adequate SVI, the RVSWI increases. In contrast, as the disease progresses, RV-arterial uncoupling occurs, and therefore the RVSWI decreases (109,110). However, a lower RVSWI may also indicate a reduction of pulmonary pressures through the effect of therapeutic agents that may lower the pulmonary pressures without pronounced increases in SVI, making the interpretation of the RVSWI challenging when used in isolation.
In patients with PAH and CTEPH, a lower RVSWI (<19.7 g·m/m2/beat) was associated with a higher incidence of heart failure related deaths or readmissions (111). RVSWI was a predictor of mortality in patients with idiopathic or heritable PAH (111). Interestingly, RVSWI was lower in heritable PAH than IPAH, suggesting a disproportionate RV dysfunction in the former group (112). RVSWI was lower (despite similar PVR) in patients with PAH associated with connective tissue disease (mostly SSc or mixed connective tissue disease) when compared with PAH due to other etiologies (mostly idiopathic or associated with congenital heart disease) (14.5 vs. 20.4 g·m/m2/beat), suggesting an intrinsic RV dysfunction in certain PAH etiologies that are linked to a lower survival (113). RVSWI of <5 g·m/m2/beat correlated with worse outcomes (death, ventricular assist device implantation, or heart transplantation) among patients with advanced heart failure undergoing evaluation for a heart transplant (114).
PV loops
The gold standard assessment of RV function is done by determining pressure-volume loops that allow a meticulous evaluation of the RV-pulmonary artery coupling. This procedure assesses how efficient the RV function is transferred as energy to the pulmonary vascular load (115,116). It is described by the ratio of end systolic elastance (Ees) over Ea (Ees/Ea). Ees is a measure of ventricular contractility that can be estimated by the ratio of end systolic pressure (ESP) to end systolic volume (ESV) (Ees = ESP/ESV). Pulmonary vascular load is estimated by the Ea derived from ESP divided by SV (Ea = ESP/SV). In PH, as the RV pressure rises throughout ejection with peaking at or near end-systole, the ESP is better approximated by systolic PAP and not mPAP. In the absence of direct ESP measurement, one can cautiously estimate it by the equation ESP = 1.65 × mPAP − 7.79 (117,118).
An efficient energy use or optimal RV-arterial coupling corresponds to an Ees/Ea ratio of 1.5–2 (109,119). Interestingly the shapes of the PV loops may provide information, while a rounder shape is normal, trapezoid and notched PV loops had the lowest Ees/Ea ratio (120,121). Different methods are used to determine the RV-arterial coupling including invasive methods (single beat or multiple beat measurements of Ees) as well as noninvasive methods (magnetic resonance imaging analysis) (122-125). The ratio of echocardiography-derived TAPSE and pulmonary arterial systolic pressure (PASP) may provide a noninvasive estimation of the RV-arterial coupling in PAH (126). A low TAPSE/PASP ratio (<0.19 mm/mmHg) is associated with overall mortality in patients with PAH, even when adjusted by clinical covariates and traditional echocardiographic and hemodynamic indicators (127).
Patients with PAH and CTEPH have decreased Ees/Ea ratios when compared with controls (123,124,128,129). The functional RV systolic adaptation was estimated by a simplified approach using SV/end-systolic volume ratio (“volume method”) and demonstrated to be an independent predictor of survival when controlled for traditional hemodynamic determinations (RAP, mPAP, SV) (95). Interestingly, during exercise, patients with PH had an increase in Ea without changes in Ees, leading to a decreased ratio when compared to controls (29). While RV-pulmonary artery uncoupling usually occurs at the later stages of the disease; exercise may help unmask abnormalities in the RV adaptation at an earlier stage (116,130). Hence, pressure-volume loops can provide a wealth of information, the challenge however lies in the measurement.
Conclusions
The hemodynamic assessment of PH is challenging because there are many factors at play between the RV and the PA circulation. A variety of indices measuring different aspects of the RV-PA system provide valuable information in patients with PH; nevertheless, more needs to be done to identify and validate comprehensive hemodynamic indices that allow a thorough evaluation of the different types of PH with the goal of making an earlier diagnosis, understanding the specific hemodynamic compromise, assessing treatment response, and providing reliable prognostic information.
Acknowledgments
Funding: None.
Footnote
Reporting Checklist: The authors have completed the Narrative Review reporting checklist. Available at https://cdt.amegroups.com/article/view/10.21037/cdt-22-244/rc
Peer Review File: Available at https://cdt.amegroups.com/article/view/10.21037/cdt-22-244/prf
Conflicts of Interest: All authors have completed the ICMJE uniform disclosure form (available at https://cdt.amegroups.com/article/view/10.21037/cdt-22-244/coif). ART serves as an unpaid editorial board member of Cardiovascular Diagnosis and Therapy from September 2021 to August 2023. The other authors have no conflicts of interest to declare.
Ethical Statement: The authors are accountable for all aspects of the work in ensuring that questions related to the accuracy or integrity of any part of the work are appropriately investigated and resolved.
Open Access Statement: This is an Open Access article distributed in accordance with the Creative Commons Attribution-NonCommercial-NoDerivs 4.0 International License (CC BY-NC-ND 4.0), which permits the non-commercial replication and distribution of the article with the strict proviso that no changes or edits are made and the original work is properly cited (including links to both the formal publication through the relevant DOI and the license). See: https://creativecommons.org/licenses/by-nc-nd/4.0/.
References
- Simonneau G, D'Armini AM, Ghofrani HA, et al. Predictors of long-term outcomes in patients treated with riociguat for chronic thromboembolic pulmonary hypertension: data from the CHEST-2 open-label, randomised, long-term extension trial. Lancet Respir Med 2016;4:372-80. [Crossref] [PubMed]
- Tonelli AR, Arelli V, Minai OA, et al. Causes and circumstances of death in pulmonary arterial hypertension. Am J Respir Crit Care Med 2013;188:365-9. [Crossref] [PubMed]
- Simonneau G, Montani D, Celermajer DS, et al. Haemodynamic definitions and updated clinical classification of pulmonary hypertension. Eur Respir J 2019;53:1801913. [Crossref] [PubMed]
- Galiè N, Channick RN, Frantz RP, et al. Risk stratification and medical therapy of pulmonary arterial hypertension. Eur Respir J 2019;53:1801889. [Crossref] [PubMed]
- Boucly A, Weatherald J, Savale L, et al. Risk assessment, prognosis and guideline implementation in pulmonary arterial hypertension. Eur Respir J 2017;50:1700889. [Crossref] [PubMed]
- Benza RL, Gomberg-Maitland M, Elliott CG, et al. Predicting Survival in Patients With Pulmonary Arterial Hypertension: The REVEAL Risk Score Calculator 2.0 and Comparison With ESC/ERS-Based Risk Assessment Strategies. Chest 2019;156:323-37. [Crossref] [PubMed]
- Reynolds HR, Hochman JS. Cardiogenic shock: current concepts and improving outcomes. Circulation 2008;117:686-97. [Crossref] [PubMed]
- Galiè N, Humbert M, Vachiery JL, et al. 2015 ESC/ERS Guidelines for the diagnosis and treatment of pulmonary hypertension: The Joint Task Force for the Diagnosis and Treatment of Pulmonary Hypertension of the European Society of Cardiology (ESC) and the European Respiratory Society (ERS): Endorsed by: Association for European Paediatric and Congenital Cardiology (AEPC), International Society for Heart and Lung Transplantation (ISHLT). Eur Respir J 2015;46:903-75. [Crossref] [PubMed]
- D'Alonzo GE, Barst RJ, Ayres SM, et al. Survival in patients with primary pulmonary hypertension. Results from a national prospective registry. Ann Intern Med 1991;115:343-9. [Crossref] [PubMed]
- McLaughlin VV, Archer SL, Badesch DB, et al. ACCF/AHA 2009 expert consensus document on pulmonary hypertension a report of the American College of Cardiology Foundation Task Force on Expert Consensus Documents and the American Heart Association developed in collaboration with the American College of Chest Physicians; American Thoracic Society, Inc.; and the Pulmonary Hypertension Association. J Am Coll Cardiol 2009;53:1573-619. [Crossref] [PubMed]
- Sandoval J, Bauerle O, Palomar A, et al. Survival in primary pulmonary hypertension. Validation of a prognostic equation. Circulation 1994;89:1733-44. [Crossref] [PubMed]
- McLaughlin VV, Shillington A, Rich S. Survival in primary pulmonary hypertension: the impact of epoprostenol therapy. Circulation 2002;106:1477-82. [Crossref] [PubMed]
- Higenbottam T, Butt AY, McMahon A, et al. Long-term intravenous prostaglandin (epoprostenol or iloprost) for treatment of severe pulmonary hypertension. Heart 1998;80:151-5. [Crossref] [PubMed]
- Wang P, Deng J, Zhang Q, et al. Additional Use of Prostacyclin Analogs in Patients With Pulmonary Arterial Hypertension: A Meta-Analysis. Front Pharmacol 2022;13:817119. [Crossref] [PubMed]
- Khirfan G, Almoushref A, Naal T, et al. Mixed Venous Oxygen Saturation Is a Better Prognosticator Than Cardiac Index in Pulmonary Arterial Hypertension. Chest 2020;158:2546-55. [Crossref] [PubMed]
- Ascha M, Bhattacharyya A, Ramos JA, et al. Pulse Oximetry and Arterial Oxygen Saturation during Cardiopulmonary Exercise Testing. Med Sci Sports Exerc 2018;50:1992-7. [Crossref] [PubMed]
- Khirfan G, Ahmed MK, Almaaitah S, et al. Comparison of Different Methods to Estimate Cardiac Index in Pulmonary Arterial Hypertension. Circulation 2019;140:705-7. [Crossref] [PubMed]
- Tonelli AR, Conci D, Tamarappoo BK, et al. Prognostic value of echocardiographic changes in patients with pulmonary arterial hypertension receiving parenteral prostacyclin therapy. J Am Soc Echocardiogr 2014;27:733-41.e2. [Crossref] [PubMed]
- Yung GL, Fedullo PF, Kinninger K, et al. Comparison of impedance cardiography to direct Fick and thermodilution cardiac output determination in pulmonary arterial hypertension. Congest Heart Fail 2004;10:7-10. [Crossref] [PubMed]
- Hoeper MM, Maier R, Tongers J, et al. Determination of cardiac output by the Fick method, thermodilution, and acetylene rebreathing in pulmonary hypertension. Am J Respir Crit Care Med 1999;160:535-41. [Crossref] [PubMed]
- Schwaiblmair M, Faul C, von Scheidt W, et al. Differences of cardiac output measurements by open-circuit acetylene uptake in pulmonary arterial hypertension and chronic thromboembolic pulmonary hypertension: a cohort study. Respir Res 2012;13:18. [Crossref] [PubMed]
- Tonelli AR, Alnuaimat H, Li N, et al. Value of impedance cardiography in patients studied for pulmonary hypertension. Lung 2011;189:369-75. [Crossref] [PubMed]
- Mayer L, Blumberg F, Wensel R, et al. Non-invasive cardiac output assessment in pulmonary hypertension. Eur Respir J 2014;44:2315.
- Rich JD, Archer SL, Rich S. Noninvasive cardiac output measurements in patients with pulmonary hypertension. Eur Respir J 2013;42:125-33. [Crossref] [PubMed]
- van Wolferen SA, Marcus JT, Boonstra A, et al. Prognostic value of right ventricular mass, volume, and function in idiopathic pulmonary arterial hypertension. Eur Heart J 2007;28:1250-7. [Crossref] [PubMed]
- van Wolferen SA, van de Veerdonk MC, Mauritz GJ, et al. Clinically significant change in stroke volume in pulmonary hypertension. Chest 2011;139:1003-9. [Crossref] [PubMed]
- Roeleveld RJ, Vonk-Noordegraaf A, Marcus JT, et al. Effects of epoprostenol on right ventricular hypertrophy and dilatation in pulmonary hypertension. Chest 2004;125:572-9. [Crossref] [PubMed]
- Nootens M, Wolfkiel CJ, Chomka EV, et al. Understanding right and left ventricular systolic function and interactions at rest and with exercise in primary pulmonary hypertension. Am J Cardiol 1995;75:374-7. [Crossref] [PubMed]
- Spruijt OA, de Man FS, Groepenhoff H, et al. The effects of exercise on right ventricular contractility and right ventricular-arterial coupling in pulmonary hypertension. Am J Respir Crit Care Med 2015;191:1050-7. [Crossref] [PubMed]
- Göransson C, Vejlstrup N, Carlsen J. Exercise cardiovascular magnetic resonance imaging allows differentiation of low-risk pulmonary arterial hypertension. J Heart Lung Transplant 2019;38:627-35. [Crossref] [PubMed]
- Nagel C, Benjamin N, Egenlauf B, et al. Effect of Supervised Training Therapy on Pulmonary Arterial Compliance and Stroke Volume in Severe Pulmonary Arterial Hypertension and Inoperable or Persistent Chronic Thromboembolic Pulmonary Hypertension. Respiration 2021;100:369-78. [Crossref] [PubMed]
- Freed BH, Patel AR, Lang RM. Redefining the role of cardiovascular imaging in patients with pulmonary arterial hypertension. Curr Cardiol Rep 2012;14:366-73. [Crossref] [PubMed]
- Göransson C, Vejlstrup N, Scheike T, et al. Implications of cardiac variability with cardiovascular magnetic resonance imaging for calculating trial sample size in pulmonary arterial hypertension. Int J Cardiol 2018;257:332-8. [Crossref] [PubMed]
- Jegier W, Sekelj P, Auld PA, et al. The relation between cardiac output and body size. Br Heart J 1963;25:425-30. [Crossref] [PubMed]
- Collis T, Devereux RB, Roman MJ, et al. Relations of stroke volume and cardiac output to body composition: the strong heart study. Circulation 2001;103:820-5. [Crossref] [PubMed]
- Dardi F, Gotti E, Palazzini M, et al. P4680Prognostic value of stroke volume index in patients with pulmonary arterial hypertension at intermediate risk. Eur Heart J 2019;40: [Crossref]
- Barańska-Pawełczak K, Wojciechowska C, Opara M, et al. Nitric Oxide Stroke Volume Index as a New Hemodynamic Prognostic Parameter for Patients with Pulmonary Arterial Hypertension. J Clin Med 2020;9:2939. [Crossref] [PubMed]
- Weatherald J, Boucly A, Chemla D, et al. Prognostic Value of Follow-Up Hemodynamic Variables After Initial Management in Pulmonary Arterial Hypertension. Circulation 2018;137:693-704. [Crossref] [PubMed]
- Condliffe R, Kiely DG, Peacock AJ, et al. Connective tissue disease-associated pulmonary arterial hypertension in the modern treatment era. Am J Respir Crit Care Med 2009;179:151-7. [Crossref] [PubMed]
- Fisher MR, Mathai SC, Champion HC, et al. Clinical differences between idiopathic and scleroderma-related pulmonary hypertension. Arthritis Rheum 2006;54:3043-50. [Crossref] [PubMed]
- Overbeek MJ, Lankhaar JW, Westerhof N, et al. Right ventricular contractility in systemic sclerosis-associated and idiopathic pulmonary arterial hypertension. Eur Respir J 2008;31:1160-6. [Crossref] [PubMed]
- Campo A, Mathai SC, Le Pavec J, et al. Hemodynamic predictors of survival in scleroderma-related pulmonary arterial hypertension. Am J Respir Crit Care Med 2010;182:252-60. [Crossref] [PubMed]
- Weatherald J, Boucly A, Launay D, et al. Haemodynamics and serial risk assessment in systemic sclerosis associated pulmonary arterial hypertension. Eur Respir J 2018;52:1800678. [Crossref] [PubMed]
- Dalen JE, Dexter L, Ockene IS, et al. Precapillary pulmonary hypertension; its relationship to pulmonary venous hypertension. Trans Am Clin Climatol Assoc 1975;86:207-18. [PubMed]
- Vachiéry JL, Adir Y, Barberà JA, et al. Pulmonary hypertension due to left heart diseases. J Am Coll Cardiol 2013;62:D100-8. [Crossref] [PubMed]
- Naeije R, Vachiery JL, Yerly P, et al. The transpulmonary pressure gradient for the diagnosis of pulmonary vascular disease. Eur Respir J 2013;41:217-23. [Crossref] [PubMed]
- Vanderpool RR, Saul M, Nouraie M, et al. Association Between Hemodynamic Markers of Pulmonary Hypertension and Outcomes in Heart Failure With Preserved Ejection Fraction. JAMA Cardiol 2018;3:298-306. [Crossref] [PubMed]
- Gorlitzer M, Ankersmit J, Fiegl N, et al. Is the transpulmonary pressure gradient a predictor for mortality after orthotopic cardiac transplantation? Transpl Int 2005;18:390-5. [Crossref] [PubMed]
- Murali S, Kormos RL, Uretsky BF, et al. Preoperative pulmonary hemodynamics and early mortality after orthotopic cardiac transplantation: the Pittsburgh experience. Am Heart J 1993;126:896-904. [Crossref] [PubMed]
- Jahanyar J, Liao JM, Butterfield RJ 3rd, et al. Transpulmonary Gradient as a Predictor of Outcomes after Heart Transplantation. J Heart Lung Transplant 2019;38:S390. [Crossref]
- Mehra MR, Canter CE, Hannan MM, et al. The 2016 International Society for Heart Lung Transplantation listing criteria for heart transplantation: A 10-year update. J Heart Lung Transplant 2016;35:1-23. [Crossref] [PubMed]
- Kainuma S, Toda K, Miyagawa S, et al. Detrimental effects of elevated transpulmonary gradient on outcomes following restrictive mitral annuloplasty in patients with pre-existing pulmonary hypertension. J Thorac Dis 2021;13:2746-57. [Crossref] [PubMed]
- Brunner NW, Yue SF, Stub D, et al. The prognostic importance of the diastolic pulmonary gradient, transpulmonary gradient, and pulmonary vascular resistance in patients undergoing transcatheter aortic valve replacement. Catheter Cardiovasc Interv 2017;90:1185-91. [Crossref] [PubMed]
- Handoko ML, De Man FS, Oosterveer FP, et al. A critical appraisal of transpulmonary and diastolic pressure gradients. Physiol Rep 2016;4:e12910. [Crossref] [PubMed]
- Gerges C, Gerges M, Lang MB, et al. Diastolic pulmonary vascular pressure gradient: a predictor of prognosis in "out-of-proportion" pulmonary hypertension. Chest 2013;143:758-66. [Crossref] [PubMed]
- Stevens PM. Assessment of acute respiratory failure: cardiac versus pulmonary causes. Chest 1975;67:1-2. [Crossref] [PubMed]
- Caravita S, Dewachter C, Soranna D, et al. Haemodynamics to predict outcome in pulmonary hypertension due to left heart disease: a meta-analysis. Eur Respir J 2018;51:1702427. [Crossref] [PubMed]
- Ibe T, Wada H, Sakakura K, et al. Pulmonary hypertension due to left heart disease: The prognostic implications of diastolic pulmonary vascular pressure gradient. J Cardiol 2016;67:555-9. [Crossref] [PubMed]
- Tedford RJ, Beaty CA, Mathai SC, et al. Prognostic value of the pre-transplant diastolic pulmonary artery pressure-to-pulmonary capillary wedge pressure gradient in cardiac transplant recipients with pulmonary hypertension. J Heart Lung Transplant 2014;33:289-97. [Crossref] [PubMed]
- Tampakakis E, Leary PJ, Selby VN, et al. The diastolic pulmonary gradient does not predict survival in patients with pulmonary hypertension due to left heart disease. JACC Heart Fail 2015;3:9-16. [Crossref] [PubMed]
- Rezaee ME, Nichols EL, Sidhu M, et al. Combined Post- and Precapillary Pulmonary Hypertension in Patients With Heart Failure. Clin Cardiol 2016;39:658-64. [Crossref] [PubMed]
- Palazzini M, Dardi F, Manes A, et al. Pulmonary hypertension due to left heart disease: analysis of survival according to the haemodynamic classification of the 2015 ESC/ERS guidelines and insights for future changes. Eur J Heart Fail 2018;20:248-55. [Crossref] [PubMed]
- Dragu R, Rispler S, Habib M, et al. Pulmonary arterial capacitance in patients with heart failure and reactive pulmonary hypertension. Eur J Heart Fail 2015;17:74-80. [Crossref] [PubMed]
- Tampakakis E, Shah SJ, Borlaug BA, et al. Pulmonary Effective Arterial Elastance as a Measure of Right Ventricular Afterload and Its Prognostic Value in Pulmonary Hypertension Due to Left Heart Disease. Circ Heart Fail 2018;11:e004436. [Crossref] [PubMed]
- Orabona P, D'Antonio A, De Rosa I, et al. Unusual clinical manifestation of metastatic pulmonary carcinoma. A case report and review of the literature. Arch Anat Cytol Pathol 1994;42:330-3. [PubMed]
- Halpern SD, Taichman DB. Misclassification of pulmonary hypertension due to reliance on pulmonary capillary wedge pressure rather than left ventricular end-diastolic pressure. Chest 2009;136:37-43. [Crossref] [PubMed]
- Nagy AI, Venkateshvaran A, Merkely B, et al. Determinants and prognostic implications of the negative diastolic pulmonary pressure gradient in patients with pulmonary hypertension due to left heart disease. Eur J Heart Fail 2017;19:88-97. [Crossref] [PubMed]
- Maron BA, Brittain EL, Hess E, et al. Pulmonary vascular resistance and clinical outcomes in patients with pulmonary hypertension: a retrospective cohort study. Lancet Respir Med 2020;8:873-84. [Crossref] [PubMed]
- Sung SH, Yeh WY, Chiang CE, et al. The prognostic significance of the alterations of pulmonary hemodynamics in patients with pulmonary arterial hypertension: a meta-regression analysis of randomized controlled trials. Syst Rev 2021;10:284. [Crossref] [PubMed]
- Kovacs G, Berghold A, Scheidl S, et al. Pulmonary arterial pressure during rest and exercise in healthy subjects: a systematic review. Eur Respir J 2009;34:888-94. [Crossref] [PubMed]
- Xanthouli P, Jordan S, Milde N, et al. Haemodynamic phenotypes and survival in patients with systemic sclerosis: the impact of the new definition of pulmonary arterial hypertension. Ann Rheum Dis 2020;79:370-8. [Crossref] [PubMed]
- Ratwatte S, Anderson J, Strange G, et al. Pulmonary arterial hypertension with below threshold pulmonary vascular resistance. Eur Respir J 2020;56:1901654. [Crossref] [PubMed]
- Benza RL, Gomberg-Maitland M, Naeije R, et al. Prognostic factors associated with increased survival in patients with pulmonary arterial hypertension treated with subcutaneous treprostinil in randomized, placebo-controlled trials. J Heart Lung Transplant 2011;30:982-9. [Crossref] [PubMed]
- Khirfan G, Li M, Wang X, et al. Is pulmonary vascular resistance index better than pulmonary vascular resistance in predicting outcomes in pulmonary arterial hypertension? J Heart Lung Transplant 2021;40:614-22. [Crossref] [PubMed]
- Alpert MA, Omran J, Bostick BP. Effects of Obesity on Cardiovascular Hemodynamics, Cardiac Morphology, and Ventricular Function. Curr Obes Rep 2016;5:424-34. [Crossref] [PubMed]
- Poirier P, Giles TD, Bray GA, et al. Obesity and cardiovascular disease: pathophysiology, evaluation, and effect of weight loss: an update of the 1997 American Heart Association Scientific Statement on Obesity and Heart Disease from the Obesity Committee of the Council on Nutrition, Physical Activity, and Metabolism. Circulation 2006;113:898-918. [Crossref] [PubMed]
- Galiè N, McLaughlin VV, Rubin LJ, et al. An overview of the 6th World Symposium on Pulmonary Hypertension. Eur Respir J 2019;53:1802148. [Crossref] [PubMed]
- Abman SH, Hansmann G, Archer SL, et al. Pediatric Pulmonary Hypertension: Guidelines From the American Heart Association and American Thoracic Society. Circulation 2015;132:2037-99. [Crossref] [PubMed]
- Rosenzweig EB, Abman SH, Adatia I, et al. Paediatric pulmonary arterial hypertension: updates on definition, classification, diagnostics and management. Eur Respir J 2019;53:1801916. [Crossref] [PubMed]
- Tedford RJ, Hassoun PM, Mathai SC, et al. Pulmonary capillary wedge pressure augments right ventricular pulsatile loading. Circulation 2012;125:289-97. [Crossref] [PubMed]
- Lankhaar JW, Westerhof N, Faes TJ, et al. Pulmonary vascular resistance and compliance stay inversely related during treatment of pulmonary hypertension. Eur Heart J 2008;29:1688-95. [Crossref] [PubMed]
- Muthurangu V, Atkinson D, Sermesant M, et al. Measurement of total pulmonary arterial compliance using invasive pressure monitoring and MR flow quantification during MR-guided cardiac catheterization. Am J Physiol Heart Circ Physiol 2005;289:H1301-6. [Crossref] [PubMed]
- Westerhof N, Lankhaar JW, Westerhof BE. The arterial Windkessel. Med Biol Eng Comput 2009;47:131-41. [Crossref] [PubMed]
- Mahapatra S, Nishimura RA, Sorajja P, et al. Relationship of pulmonary arterial capacitance and mortality in idiopathic pulmonary arterial hypertension. J Am Coll Cardiol 2006;47:799-803. [Crossref] [PubMed]
- Mahapatra S, Nishimura RA, Oh JK, et al. The prognostic value of pulmonary vascular capacitance determined by Doppler echocardiography in patients with pulmonary arterial hypertension. J Am Soc Echocardiogr 2006;19:1045-50. [Crossref] [PubMed]
- Gan CT, Lankhaar JW, Westerhof N, et al. Noninvasively assessed pulmonary artery stiffness predicts mortality in pulmonary arterial hypertension. Chest 2007;132:1906-12. [Crossref] [PubMed]
- Guo X, Lai J, Wang H, et al. Predictive Value of Pulmonary Arterial Compliance in Systemic Lupus Erythematosus Patients With Pulmonary Arterial Hypertension. Hypertension 2020;76:1161-8. [Crossref] [PubMed]
- Al-Naamani N, Preston IR, Paulus JK, et al. Pulmonary Arterial Capacitance Is an Important Predictor of Mortality in Heart Failure With a Preserved Ejection Fraction. JACC Heart Fail 2015;3:467-74. [Crossref] [PubMed]
- Pellegrini P, Rossi A, Pasotti M, et al. Prognostic relevance of pulmonary arterial compliance in patients with chronic heart failure. Chest 2014;145:1064-70. [Crossref] [PubMed]
- Brittain EL, Pugh ME, Wheeler LA, et al. Prostanoids but not oral therapies improve right ventricular function in pulmonary arterial hypertension. JACC Heart Fail 2013;1:300-7. [Crossref] [PubMed]
- Khan A, White RJ, Meyer G, et al. Oral treprostinil improves pulmonary vascular compliance in pulmonary arterial hypertension. Respir Med 2022;193:106744. [Crossref] [PubMed]
- Akaslan D, Ataş H, Aslanger E, et al. Change in pulmonary arterial compliance and pulmonary pulsatile stress after balloon pulmonary angioplasty. Anatol J Cardiol 2022;26:43-8. [Crossref] [PubMed]
- Metkus TS, Mullin CJ, Grandin EW, et al. Heart Rate Dependence of the Pulmonary Resistance x Compliance (RC) Time and Impact on Right Ventricular Load. PLoS One 2016;11:e0166463. [Crossref] [PubMed]
- Vanderpool RR, Pinsky MR, Naeije R, et al. RV-pulmonary arterial coupling predicts outcome in patients referred for pulmonary hypertension. Heart 2015;101:37-43. [Crossref] [PubMed]
- Fares WH, Bellumkonda L, Tonelli AR, et al. Right atrial pressure/pulmonary artery wedge pressure ratio: A more specific predictor of survival in pulmonary arterial hypertension. J Heart Lung Transplant 2016;35:760-7. [Crossref] [PubMed]
- Van A, Turlapati N, Jakkoju A, et al. Right Atrial to Pulmonary Artery Wedge Pressure (RA/PAWP) Ratio Across the Hemodynamic Spectrum in Pulmonary Hypertension. Am J Respir Crit Care Med 2019;199:A2807.
- Drazner MH, Velez-Martinez M, Ayers CR, et al. Relationship of right- to left-sided ventricular filling pressures in advanced heart failure: insights from the ESCAPE trial. Circ Heart Fail 2013;6:264-70. [Crossref] [PubMed]
- Grodin JL, Drazner MH, Dupont M, et al. A disproportionate elevation in right ventricular filling pressure, in relation to left ventricular filling pressure, is associated with renal impairment and increased mortality in advanced decompensated heart failure. Am Heart J 2015;169:806-12. [Crossref] [PubMed]
- Segev A, Nathanzon S, Fardman A, et al. Right atrium to pulmonary capillary wedge pressure ratio is associated with right ventricular failure and mortality after left ventricular assist device surgery. Eur Heart J 2020;41: [Crossref]
- Fujino T, Sayer A, Nitta D, et al. Longitudinal Trajectories of Hemodynamics Following Left Ventricular Assist Device Implantation. J Card Fail 2020;26:383-90. [Crossref] [PubMed]
- Mazimba S, Welch TS, Mwansa H, et al. Haemodynamically Derived Pulmonary Artery Pulsatility Index Predicts Mortality in Pulmonary Arterial Hypertension. Heart Lung Circ 2019;28:752-60. [Crossref] [PubMed]
- Bernardo R, Vanderpool R, Rischard F. The pulmonary artery pulsatility index correlates with ventriculo-vascular coupling and elevated filling pressures in patients with pulmonary arterial hypertension. Eur Respir J 2018;52:PA3314.
- Korabathina R, Heffernan KS, Paruchuri V, et al. The pulmonary artery pulsatility index identifies severe right ventricular dysfunction in acute inferior myocardial infarction. Catheter Cardiovasc Interv 2012;80:593-600. [Crossref] [PubMed]
- Kang G, Ha R, Banerjee D. Pulmonary artery pulsatility index predicts right ventricular failure after left ventricular assist device implantation. J Heart Lung Transplant 2016;35:67-73. Erratum in: J Heart Lung Transplant 2017;36:1272. [Crossref] [PubMed]
- Morine KJ, Kiernan MS, Pham DT, et al. Pulmonary Artery Pulsatility Index Is Associated With Right Ventricular Failure After Left Ventricular Assist Device Surgery. J Card Fail 2016;22:110-6. [Crossref] [PubMed]
- Kochav SM, Flores RJ, Truby LK, et al. Prognostic Impact of Pulmonary Artery Pulsatility Index (PAPi) in Patients With Advanced Heart Failure: Insights From the ESCAPE Trial. J Card Fail 2018;24:453-9. [Crossref] [PubMed]
- Guven G, Brankovic M, Constantinescu AA, et al. Preoperative right heart hemodynamics predict postoperative acute kidney injury after heart transplantation. Intensive Care Med 2018;44:588-97. [Crossref] [PubMed]
- Lim Y, Low TT, Chan SP, et al. Does pulmonary artery pulsatility index predict mortality in pulmonary arterial hypertension? ESC Heart Fail 2021;8:3835-44. [Crossref] [PubMed]
- Vonk-Noordegraaf A, Haddad F, Chin KM, et al. Right heart adaptation to pulmonary arterial hypertension: physiology and pathobiology. J Am Coll Cardiol 2013;62:D22-33. [Crossref] [PubMed]
- Stevens GR, Garcia-Alvarez A, Sahni S, et al. RV dysfunction in pulmonary hypertension is independently related to pulmonary artery stiffness. JACC Cardiovasc Imaging 2012;5:378-87. [Crossref] [PubMed]
- Ibe T, Wada H, Sakakura K, et al. Right Ventricular Stroke Work Index. Int Heart J 2018;59:1047-51. [Crossref] [PubMed]
- Brittain EL, Pugh ME, Wheeler LA, et al. Shorter survival in familial versus idiopathic pulmonary arterial hypertension is associated with hemodynamic markers of impaired right ventricular function. Pulm Circ 2013;3:589-98. [Crossref] [PubMed]
- Clapham KR, Highland KB, Rao Y, et al. Reduced RVSWI Is Associated With Increased Mortality in Connective Tissue Disease Associated Pulmonary Arterial Hypertension. Front Cardiovasc Med 2020;7:77. [Crossref] [PubMed]
- Kato TS, Stevens GR, Jiang J, et al. Risk stratification of ambulatory patients with advanced heart failure undergoing evaluation for heart transplantation. J Heart Lung Transplant 2013;32:333-40. [Crossref] [PubMed]
- Ren X, Johns RA, Gao WD. EXPRESS: Right Heart in Pulmonary Hypertension: From Adaptation to Failure. Pulm Circ 2019; Epub ahead of print. [Crossref] [PubMed]
- Vonk Noordegraaf A, Westerhof BE, Westerhof N. The Relationship Between the Right Ventricle and its Load in Pulmonary Hypertension. J Am Coll Cardiol 2017;69:236-43. [Crossref] [PubMed]
- Tedford RJ, Hsu S, Kass DA. Letter by Tedford et al Regarding Article, "Effective Arterial Elastance in the Pulmonary Arterial Circulation: Derivation, Assumptions, and Clinical Applications". Circ Heart Fail 2020;13:e007081. [Crossref] [PubMed]
- Tello K, Richter MJ, Axmann J, et al. More on Single-Beat Estimation of Right Ventriculoarterial Coupling in Pulmonary Arterial Hypertension. Am J Respir Crit Care Med 2018;198:816-8. [Crossref] [PubMed]
- Champion HC, Michelakis ED, Hassoun PM. Comprehensive invasive and noninvasive approach to the right ventricle-pulmonary circulation unit: state of the art and clinical and research implications. Circulation 2009;120:992-1007. [Crossref] [PubMed]
- Maughan WL, Shoukas AA, Sagawa K, et al. Instantaneous pressure-volume relationship of the canine right ventricle. Circ Res 1979;44:309-15. [Crossref] [PubMed]
- Richter MJ, Hsu S, Yogeswaran A, et al. Right ventricular pressure-volume loop shape and systolic pressure change in pulmonary hypertension. Am J Physiol Lung Cell Mol Physiol 2021;320:L715-25. [Crossref] [PubMed]
- Sanz J, García-Alvarez A, Fernández-Friera L, et al. Right ventriculo-arterial coupling in pulmonary hypertension: a magnetic resonance study. Heart 2012;98:238-43. [Crossref] [PubMed]
- Tedford RJ, Mudd JO, Girgis RE, et al. Right ventricular dysfunction in systemic sclerosis-associated pulmonary arterial hypertension. Circ Heart Fail 2013;6:953-63. [Crossref] [PubMed]
- McCabe C, White PA, Hoole SP, et al. Right ventricular dysfunction in chronic thromboembolic obstruction of the pulmonary artery: a pressure-volume study using the conductance catheter. J Appl Physiol (1985) 2014;116:355-63. [PubMed]
- Kuehne T, Yilmaz S, Steendijk P, et al. Magnetic resonance imaging analysis of right ventricular pressure-volume loops: in vivo validation and clinical application in patients with pulmonary hypertension. Circulation 2004;110:2010-6. [Crossref] [PubMed]
- Tello K, Wan J, Dalmer A, et al. Validation of the Tricuspid Annular Plane Systolic Excursion/Systolic Pulmonary Artery Pressure Ratio for the Assessment of Right Ventricular-Arterial Coupling in Severe Pulmonary Hypertension. Circ Cardiovasc Imaging 2019;12:e009047. [Crossref] [PubMed]
- Tello K, Axmann J, Ghofrani HA, et al. Relevance of the TAPSE/PASP ratio in pulmonary arterial hypertension. Int J Cardiol 2018;266:229-35. [Crossref] [PubMed]
- Hsu S, Houston BA, Tampakakis E, et al. Right Ventricular Functional Reserve in Pulmonary Arterial Hypertension. Circulation 2016;133:2413-22. [Crossref] [PubMed]
- Wauthy P, Naeije R, Brimioulle S. Left and right ventriculo-arterial coupling in a patient with congenitally corrected transposition. Cardiol Young 2005;15:647-9. [Crossref] [PubMed]
- Trip P, Rain S, Handoko ML, et al. Clinical relevance of right ventricular diastolic stiffness in pulmonary hypertension. Eur Respir J 2015;45:1603-12. [Crossref] [PubMed]