The number of myocardial infarction segments connected to papillary muscle is associated with the improvement in moderate ischemic mitral regurgitation
Introduction
For patients with coronary heart disease (CHD) with moderate ischemic mitral regurgitation (IMR), It is still controversial that (1) which patients can improve IMR from coronary artery bypass grafting (CABG) and avoid the additional injury caused by combined mitral valve surgery (2,3) and which patients cannot improve IMR from CABG and should be required simultaneous mitral valve surgery.
Previous studies (4,5) showed that a greater left ventricular ejection fraction (LVEF) and a greater posterior-inferior volume ratio are predictors of an improvement in IMR after isolated CABG. Conversely, left ventricular dysfunction (LVEF ≤40%), dyssynchronous movement between papillary muscles (PMs), and abnormal movement of the anterior wall of the left ventricle (LV) are risk factors for no IMR improvement after isolated CABG (6-8). These indicators are evaluated by echocardiography, which can be used to assess the structure and function of the LV, but not myocardial scarring.
Cardiac magnetic resonance (CMR) with late gadolinium enhancement (LGE) is the gold-standard imaging method for detecting myocardial scarring caused by myocardial infarction (MI) (9). IMR is the result of remodeling in the LV after MI (10); however, there are few studies on IMR and local remodeling of the LV, and the relationship between local remodeling of the LV after CABG and the change in IMR is not clear. We hypothesized that the severity of local LV remodeling was related to the improvement of IMR after CABG. Therefore, the purpose of this study was to evaluate the severity of infarction in myocardial segments connected to PM using LGE to predict whether IMR improves after isolated CABG and to optimize the surgical strategy and improve prognosis. We present the following article in accordance with the STARD reporting checklist (available at https://cdt.amegroups.com/article/view/10.21037/cdt-22-301/rc).
Methods
Patient enrollment
This is a single-center, retrospective, observational cohort study. Patients diagnosed with CHD complicated with moderate IMR who underwent an examination with LGE at Beijing Anzhen Hospital from January 2016 to December 2020 were selected continuously. The inclusion criteria were: (I) a diagnosis of moderate IMR by transthoracic echocardiography (11); (II) LGE and echocardiography within 10 days preoperatively; (III) accurate detection of myocardial scar tissue by LGE; (IV) complete anatomical revascularization (12); and (V) coronary computed tomography angiography (CTA) showing coronary bypass graft patency during the 1-year follow-up. The exclusion criteria were (I) organic mitral valve disease; (II) a history of mitral valve surgery; (III) coexistence of other heart valve diseases; (IV) long-term persistent atrial fibrillation and other arrhythmias; (V) preoperative emergency surgery for cardiogenic shock; and (VI) acute MI in the previous 3 months.
Study protocol
The patients’ baseline clinical characteristics, including demographic information, echocardiography, and LGE data, were retrospectively collected. Follow-up information was collected by telephone, WeChat, or outpatient visits. The patients were divided into two groups: the improved group (no or mild IMR) and the unimproved group (moderate or severe IMR) based on the severity of IMR, as assessed by echocardiography 1 year postoperatively. The baseline characteristics were compared between the two groups to identify the independent risk factors for moderate or severe IMR 1 year after isolated CABG. All patients provided written informed consent. The study was conducted in accordance with the Declaration of Helsinki (as revised in 2013). The study was approved by the Institutional Ethics Committee of Beijing Anzhen Hospital (No. 2016024) and informed consent was obtained from all the patients.
Echocardiography
All patients underwent transthoracic echocardiography at rest within 1 week preoperatively and 1 year postoperatively. Transthoracic echocardiography was performed using a Vivid 7 color Doppler echocardiography system (GE Healthcare, Chicago, IL, USA). The severity of IMR was quantified according to the standard quantitative proximal isovelocity surface area method recommended by the European Cardiovascular Association (13). IMR was divided (11) into no regurgitation, mild IMR [IMR area of <4 cm², effective regurgitation orifice area (EROA) of <0.2 cm²], moderate IMR (IMR area of 4–8 cm², EROA of 0.2–0.39 cm²), and severe IMR (IMR area of >8 cm², EROA of ≥0.4 cm²).
LV segmentation
According to the 17-segment heart zoning model proposed by the American Heart Association/College of Cardiology (14), the LV was divided into the basal segment, central segment, and apical segment along its long axis. The apex was too thin to be evaluated; thus, the apex segment was subtracted from the 17 segments, and the remaining 16 segments were analyzed. According to the anatomical characteristics of the PM and LV, the myocardium connected to PM is mainly located in the lower wall of the central segment [10], the proximal inferior lateral wall of the central segment [11], the proximal anterior lateral wall of the central segment [12], the lower wall of the apical segment [15], and the lateral wall of the apical segment [16] (Figure 1).
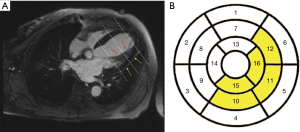
LGE examination and analysis
CMR with LGE was performed with a MAGNETOM Verio 3.0-T superconducting magnetic resonance system (Siemens Healthineers, Erlangen, Germany). Intravenous injection of gadopentetate dimeglumine contrast agent at 0.1 mmol/kg. Approximately, 10 minutes after LGE imaging was performed using phase-sensitive inversion recovery magnetic moment pre-preparation of a turbo fast low-angle shot stimulation (Turbo-FLASH) sequence.
When the LGE area was 0–50%, the segment was considered viable, and when the LGE area was 51–100%, the segment was considered as an MI segment. LGE of 50% was used as the optimal threshold to determine the viability of the myocardial segment and to predict whether the function of the segment improved after revascularization (15,16). If PM enhancement was seen on images with LGE, PM infarction (PMI) was considered. The degree of global myocardial remodeling of the LV was measured by the number of MI segments in the global LV, and the degree of local myocardial remodeling of the LV was measured by the number of MI segments connected to PM. Commercial cardiovascular post-processing software (cvi42; Circle Cardiovascular Imaging Inc., Calgary, Canada) was used for analysis. This was performed by a radiologist with 5 years of CMR experience who was unaware of the patients’ clinical data and grouping information.
Surgical techniques
A conventional sternal median incision was made. The internal mammary artery was obtained by ossification or pedicling, and the great saphenous vein was obtained using an open technique. Transplantation of the left internal mammary artery to the left anterior descending branch was the first-choice technique, and the great saphenous vein was sequentially anastomosed to other coronary arteries. Complete revascularization was performed in all patients (12). Off-pump or on-pump CABG was performed according to the patient’s condition and surgeon’s experience. Transit-time flow measurement was used to evaluate the quality of coronary bypass vessel anastomosis.
Postoperative management and medical therapy
The patients were returned to the intensive care unit with tracheal intubation and given ECG monitoring, ventilator assisted breathing, and hemodynamic monitoring. Arterial blood gas analysis was performed immediately after operation, on the first day after operation and before pulling out the endotracheal intubation, in order to timely adjust the water and electrolyte metabolism and acid-base balance disorders. Dual antiplatelet drugs were given on the first day after operation. Patients with heart failure and a reduced LVEF were treated with guideline-directed medical therapy, including angiotensin converting enzyme inhibitors (ACEIs), angiotensin-receptor blockers (ARBs), beta blockers, aldosterone antagonists, and/or sacubitril/valsartan (17).
Follow-up
The patients were followed up at 3 and 6 months postoperatively and every 6 months thereafter. Follow-up mainly included an assessment of whether IMR improved, New York Heart Association (NYHA) functional class, and the incidence of major cardiovascular and cerebrovascular events (MACCEs). An improvement in IMR was defined as no or mild MR, and no IMR improvement was defined as moderate or severe IMR by transthoracic echocardiography at 1 year after CABG. MACCEs included all-cause death, MI, heart failure, cerebrovascular events, and rehospitalization for heart disease. Coronary CTA results were also recorded. The patency of coronary bypass vessels was examined by coronary CTA and assessed according to the Fitzgibbon classification system (18). All patients’ data were obtained from our institution’s online database and collected using standardized data collection forms.
Statistical analysis
The Student’s t-test was used for normally distributed measurement data, which are expressed as mean ± standard deviation. The Mann-Whitney U test was used for non-normally distributed measurement data, which are expressed as median and interquartile range (P25, P75). The chi-square test or Fisher’s exact test was used for count data, which are expressed as frequency (rate). The Kaplan-Meier method was used to calculate the MACCEs-free survival curves of the two groups. The log-rank test was used to identify differences in the survival curves between the two groups. The univariate logistic regression analysis was used to assess the relationship between variables and no IMR improvement. Variables with a P value of <0.2 in the univariate logistic regression analysis and variables reported in previous studies, as well as variables considered clinically closely related to the endpoint events, were included in the multivariate logistic regression analysis using the forward stepwise method. The receiver operating characteristic (ROC) curve analysis was used to calculate the area under the curve (AUC), and the maximum Youden index (sensitivity + specificity − 1) determined the cut-off value for no IMR improvement after isolated CABG. All statistical tests were two-sided, and a P value of <0.05 was considered statistically significant. SPSS version 23.0 (IBM Corporation, Armonk, NY, USA) and R 4.0.2 (R Foundation for Statistical Computing, Vienna, Austria) were used for statistical analysis.
Results
A total of 126 patients diagnosed with CHD complicated with moderate IMR who underwent an examination with LGE at Beijing Anzhen Hospital from January 2016 to December 2020 were continuously enrolled. Of the 126 patients, 3 received medication, 48 underwent mitral valve plasty (MVP) during CABG, and 14 had other heart diseases and underwent cardiac surgery. Sixty-one patients underwent isolated CABG; of these patients, 2 died of low cardiac output syndrome and heart failure postoperatively, 2 were lost to follow-up 1 year postoperatively, and 3 developed coronary bypass vessel occlusion 1 year postoperatively. Therefore, 54 patients with CHD complicated with moderate IMR who underwent isolated CABG were included in this study (Figure 2).
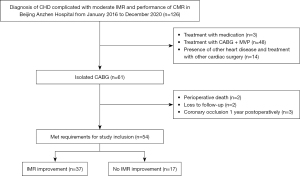
The mean age of the patients was 64.4±9.0 years (range, 43–84 years), and 72.2% of the patients (39/54) were male. IMR improved (no or mild IMR) in 37 patients (68.5%) at 1 year after CABG, while no improvement was noted in 17 patients (31.5%) at 1 year after CABG, including 15 patients (27.8%) with moderate IMR and 2 patients (3.7%) with severe IMR. There were no significant differences in age, sex, body mass index, and other demographic characteristics between the two groups (P>0.05) (Table 1).
Table 1
Variable | Improved (n=37) | Unimproved (n=17) | P values |
---|---|---|---|
Age (y) | 63.6±9.9 | 66.3±6.6 | 0.308 |
Age >65 years | 17 (45.9) | 8 (47.1) | 0.939 |
Male | 28 (75.7) | 11 (64.7) | 0.611 |
BMI (kg/m²) | 25.6±2.9 | 24.8±2.3 | 0.349 |
BSA (m²) | 1.79±0.2 | 1.75±0.2 | 0.381 |
Hypertension | 19 (51.4) | 10 (58.8) | 0.609 |
Diabetes | 11 (29.7) | 3 (17.6) | 0.544 |
Hyperlipidemia | 13 (35.1) | 9 (52.9) | 0.216 |
The history of PCI | 4 (10.8) | 2 (11.8) | 1.000 |
Smoking | 17 (45.9) | 6 (35.3) | 0.462 |
Drinking | 11 (29.7) | 4 (23.5) | 0.884 |
COPD | 2 (5.4) | 0 (0.0) | 1.000 |
Cerebral infarction | 6 (16.2) | 4 (23.5) | 0.791 |
PAD | 18 (48.6) | 9 (52.9) | 0.770 |
The data are presented as mean ± SD or n (%). BMI, body mass index; BSA, body surface area; PCI, percutaneous coronary intervention; COPD, chronic obstructive pulmonary disease; PAD, peripheral artery disease.
There were no significant differences in LVEF, LV size, and incidence of PMI between the two groups (all P>0.05). However, the number of MI segments in the global LV in the unimproved group was significantly higher than in the improved group [4 (3.0, 6.5) vs. 1.0 (0, 3.0); P=0.001], and the number of MI segments connected to PM in the unimproved group was higher [2.0 (2.0, 2.5) vs. 0 (0, 1.0); P<0.001] (Table 2). Figure 3 shows the CMR-LGE images, preoperative and postoperative echocardiography images of a patient whose IMR not improved at 1 year after isolated CABG. Figure 4 shows the CMR-LGE images, preoperative and postoperative echocardiography images of a patient whose IMR improvement at 1 year after isolated CABG.
Table 2
Variables | Improved (n=37) | Unimproved (n=17) | P values |
---|---|---|---|
Echocardiogram | |||
LVEF (%) | 48.9±10.6 | 44.4±11.1 | 0.156 |
LVEDD (mm) | 56.0±7.6 | 55.7±10.8 | 0.904 |
LVESD (mm) | 41.7±8.5 | 42.7±12.5 | 0.738 |
IMR area (cm²) | 5.6±1.1 | 5.5±1.3 | 0.646 |
EROA (cm²) | 0.30±0.07 | 0.34±0.11 | 0.264 |
LGE | |||
PMI | 25 (67.6) | 13 (76.5) | 0.506 |
Number of MI segments in global LV | 1.0 (0, 3.0) | 4.0 (3.0, 6.5) | 0.001 |
Number of MI segments connected to PM | 0 (0, 1.0) | 2.0 (2.0, 2.5) | <0.001 |
MI segments connected to PM ≥2 | 6 (16.2) | 14 (82.4) | <0.001 |
The data are presented as mean ± SD, n (%), or median (range). LGE, late gadolinium enhancement; LVEF, left ventricular ejection fraction; LVEDD, left ventricular end-diastolic diameter; LVESD, left ventricular end-systolic diameter; IMR, ischemic mitral regurgitation; EROA, effective regurgitation orifice area; PMI, papillary muscle infarction; MI, myocardial infarction; LV, left ventricle; PM, papillary muscle.
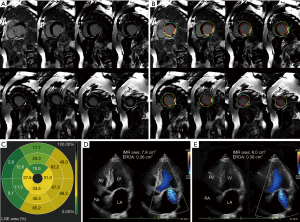
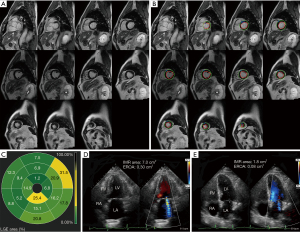
The clinical data of patients including LVEF, LV end-diastolic diameter (LVEDD), PMI, number of MI segments in the global LV, and number of MI segments connected to PM were included in the multivariate regression equation. The multivariate analysis showed that the number of MI segments connected to PM [odds ratio (OR) 4.39, 95% confidence interval (CI): 1.93–9.98; P<0.001] was associated with no IMR improvement after isolated CABG (Table 3). The ROC curve analysis showed that a cut-off value of ≥2 MI segments connected to PM was optimal for predicting an improvement in IMR (Figure 5), with a sensitivity of 82.4% and a specificity of 83.8%. The AUC was 0.86 (95% CI: 0.75–0.96). A cross-tabulation of preoperative LGE area and 1-year postoperative echocardiography is shown in Table 4.
Table 3
Variable | Univariate analysis | Multivariate analysis | |||
---|---|---|---|---|---|
OR (95% CI) | P values | OR (95% CI) | P values | ||
LVEF (%) | 0.96 (0.91–1.02) | 0.157 | – | – | |
LVEDD (mm) | 1.00 (0.93–1.07) | 0.902 | – | – | |
PMI | 1.56 (0.42–5.81) | 0.508 | – | – | |
Number of MI segments in global LV | 1.61 (1.20–2.16) | 0.001 | – | – | |
Number of MI segments connected to PM | 4.39 (1.93–9.98) | <0.001 | 4.39 (1.93–9.98) | <0.001 |
OR, odds ratio; CI, confidence interval; LVEF, left ventricular ejection fraction; LVEDD, left ventricular end-diastolic diameter; PMI, papillary muscle infarction; MI, myocardial infarction; LV, left ventricular; PM, papillary muscle.
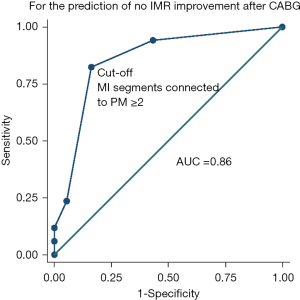
Table 4
Preoperative LGE area | 1-year postoperative echocardiography | Total | |
---|---|---|---|
No IMR improvement | IMR improvement | ||
No IMR improvement | 14 | 6 | 20 |
IMR improvement | 3 | 31 | 34 |
Total | 17 | 37 | 54 |
LGE, late gadolinium enhancement; IMR, ischemic mitral regurgitation.
Echocardiography showed no significant differences in LVEF, LVEDD, and LV end-systolic diameter between the improved group and the unimproved group at 1 year postoperatively (all P>0.05). However, IMR area and the EROA were significantly lower in the improved group than in the unimproved group (P<0.05). These patients were followed up for 1–5 years (median, 2.8 years), and the unimproved group had a significantly higher incidence of MACCEs than the improved group (P=0.041) (Figure 6). Among them, two patients in the improved group developed MACCEs (rehospitalization for heart disease), and four patients in the unimproved group developed MACCEs (two patients with all-cause death and two patients with rehospitalization for heart disease). The NYHA functional class was significantly higher in the unimproved group than in the improved group (P=0.026) (Table 5).
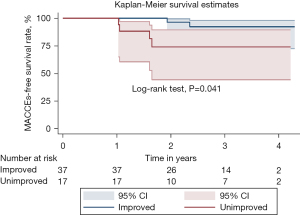
Table 5
Variable | Improved (n=37) | Unimproved (n=17) | P values |
---|---|---|---|
Echocardiogram | |||
LVEF (%) | 55.8±9.2 | 54.2±6.7 | 0.620 |
LVEDD (mm) | 51.2±5.9 | 52.5±6.7 | 0.561 |
LVESD (mm) | 36.2±7.4 | 38.2±7.1 | 0.481 |
IMR area (cm²) | 2.3±1.2 | 6.2±1.7 | <0.001 |
EROA (cm²) | 0.12±0.06 | 0.31±0.09 | <0.001 |
Results follow-up | |||
MACCEs | 2 (5.4) | 4 (23.5) | 0.041 |
NYHA classification | 0.026 | ||
Class I | 19 (51.4) | 4 (23.5) | |
Class II | 13 (35.1) | 7 (41.2) | |
Class III | 5 (13.5) | 5 (29.4) | |
Class IV | 0 (0.0) | 1 (5.9) | |
MI segments connected to PM ≥2 | 6 (16.2) | 14 (82.4) | <0.001 |
The data are presented as mean ± SD or n (%). LVEF, left ventricular ejection fraction; LVEDD, left ventricular end-diastolic diameter; LVESD, left ventricular end-systolic diameter; IMR, ischemic mitral regurgitation; EROA, effective regurgitation orifice area; MACCEs, major adverse cardiovascular and cerebrovascular events; NYHA, New York Heart Association.
Discussion
This study presents some important findings. First, among patients with moderate IMR, 31.5% demonstrated no IMR improvement at 1 year after isolated CABG. Second, the number of MI segments connected to PM was a predictor of no IMR improvement after isolated CABG. The ROC curve analysis showed that ≥2 MI segments connected to PM could predict no IMR improvement after isolated CABG, with good sensitivity and specificity. Third, patients with no IMR improvement had a poorer intermediate outcome and a higher NYHA functional class than patients with IMR improvement. Therefore, for patients with moderate IMR, LGE can be used to evaluate the number of MI segments connected to PM to predict whether IMR improves after isolated CABG, thus guiding the selection of surgical strategy.
The crux of the current controversy is that the intervention strategy for concurrent mitral valve surgery in patients with CHD complicated with moderate IMR is unclear (1). To date, the largest randomized controlled trial of the Cardiothoracic Surgery Trial Network study (2) showed that although CABG + MVP could more effectively improve mitral regurgitation, it did not improve postoperative survival or reduce the overall incidence of adverse events and hospital readmission, and it was associated with early nervous system events (cerebral infarction, transient ischemic attack, and metabolic encephalopathy) and supraventricular arrhythmia. Therefore, rather than determining what type of surgery is the best option for these patients, the surgeon might consider which patients are suitable for CABG versus CABG combined with mitral valve surgery. This study analyzed patients receiving isolated CABG and found that nearly 1/3 of the patients had no IMR improvement, and the incidence of MACCE and NYHA functional class were higher than in the improved group.
IMR is a result of local LV remodeling caused by inferior or posterior wall infarction, and the main mechanism of IMR is PM displacement in the apical and posterolateral directions, leading to stretching of the chordae tendineae (10). The present study showed that the ratio of LGE mass to overall LV mass was not a risk factor for unimproved IMR, whereas the myocardium connected to PM in the unimproved group contained more MI segments. The improvement in IMR may be due to the reversal of local LV remodeling after revascularization, the improvement in local LV wall motion, and the reduction in chordae tendineae stretching. If the degree of infarction in the myocardium connected to PM is severe, LV local remodeling cannot be reversed after revascularization, and IMR cannot be improved. Therefore, we believe that IMR is mainly caused by local remodeling of the LV, which is consistent with the results of a previous study (4).
Previous studies (4,5) showed that a greater LVEF and a greater posterior-inferior volume ratio are predictors of an improvement in IMR after isolated CABG. Conversely, LV dysfunction (LVEF ≤40%), dyssynchronous movement between PMs, and abnormal movement of the anterior wall of the LV are risk factors for no IMR improvement after isolated CABG (6-8). The main mechanism of IMR is ventricular remodeling induced by myocardial ischemia, which is closely related to myocardial scarring. Therefore, the degree of myocardial scarring is a key factor in predicting reverse myocardial remodeling and improving IMR after CABG. In this study, the evaluation of the degree and scope of myocardial scarring by LGE showed that the number of MI segments connected to PM was an independent risk factor for no IMR improvement after isolated CABG.
Several studies have revealed whether there is a correlation between IMR and PMI (19,20), showing that IMR is irrelevant to PMI. Similarly, Tanimoto et al. (21) concluded that IMR is caused by LV remodeling and that there is no evidence of a link to PMI. The results of the present study suggest that PMI is not associated with IMR improvement after isolated CABG. This is because the mitral valve-chordae tendineae-PM complex still exists after PMI. The function of the PM is not to contract to close the mitral valve, but to ensure that the mitral valve is in the proper position during ventricular contraction so that the mitral valve can be closed.
As described above, this study evaluated the number of MI segments connected to PM by CMR-LGE to predict whether IMR improved after isolated CABG in patients with CHD complicated with moderate IMR. The results showed that the number of MI segments connected to PM was an independent risk factor for no IMR improvement after isolated CABG. The results of this study are helpful to identify patients with improved IMR after isolated CABG, so as to make individualized diagnosis and treatment for such patients, reduce the incidence of MACCEs and improve the prognosis.
Limitations of the study
This study had several limitations. Firstly, this was a single-center, retrospective, observational cohort study with a small sample size. Thus, further verification in a multicenter, prospective, large-sample study is required. Secondly, the preoperative cardiac function of selected patients undergoing LGE was poor, which may have resulted in patient selection bias. Thirdly, the follow-up period was short; thus, a longer follow-up period is required to confirm our findings. Finally, because of the long LGE examination time at the postoperative outpatient department, some patients did not undergo postoperative LGE, preventing comparisons of the preoperative and postoperative imaging findings.
Conclusions
For patients with CHD complicated with moderate IMR, LGE can be used to evaluate the number of MI segments connected to PM to guide the choice of surgical strategy. The presence of <2 MI segments connected to PM is associated with an improvement in IMR after isolated CABG. For patients with ≥2 MI segments connected to PM, mitral valve surgery should be performed simultaneously with CABG.
Acknowledgments
The authors would like to thank Emily Woodhouse, PhD, and Angela Morben, DVM, ELS, from Liwen Bianji (Edanz) (www.liwenbianji.cn), for editing the English text of a draft of this manuscript, and Jumatay Biekan (Circle Cardiovascular Imaging, Calgary, Alberta, Canada) for his assistance in image post-processing.
Funding: This work was support by Beijing Science and Technology Project (Code: Z151100003915084). The Beijing Science and Technology Project is a fund project established by the Beijing municipal government to encourage innovation and development in science and technology.
Footnote
Reporting Checklist: The authors have completed the STARD reporting checklist. Available at https://cdt.amegroups.com/article/view/10.21037/cdt-22-301/rc
Data Sharing Statement: Available at https://cdt.amegroups.com/article/view/10.21037/cdt-22-301/dss
Peer Review File: Available at https://cdt.amegroups.com/article/view/10.21037/cdt-22-301/prf
Conflicts of Interest: All authors have completed the ICMJE uniform disclosure form (available at https://cdt.amegroups.com/article/view/10.21037/cdt-22-301/coif). The authors have no conflicts of interest to declare.
Ethical Statement: The authors are accountable for all aspects of the work in ensuring that questions related to the accuracy or integrity of any part of the work are appropriately investigated and resolved. The study was conducted in accordance with the Declaration of Helsinki (as revised in 2013). The study was approved by the Institutional Ethics Committee of Beijing Anzhen Hospital (No. 2016024) and informed consent was taken from all the patients.
Open Access Statement: This is an Open Access article distributed in accordance with the Creative Commons Attribution-NonCommercial-NoDerivs 4.0 International License (CC BY-NC-ND 4.0), which permits the non-commercial replication and distribution of the article with the strict proviso that no changes or edits are made and the original work is properly cited (including links to both the formal publication through the relevant DOI and the license). See: https://creativecommons.org/licenses/by-nc-nd/4.0/.
References
- Vahanian A, Beyersdorf F, Praz F, et al. 2021 ESC/EACTS Guidelines for the management of valvular heart disease. Eur Heart J 2022;43:561-632. [Crossref] [PubMed]
- Goldstein D, Moskowitz AJ, Gelijns AC, et al. Two-Year Outcomes of Surgical Treatment of Severe Ischemic Mitral Regurgitation. N Engl J Med 2016;374:344-53. [Crossref] [PubMed]
- Bakaeen FG, Gaudino M, Whitman G, et al. 2021: The American Association for Thoracic Surgery Expert Consensus Document: Coronary artery bypass grafting in patients with ischemic cardiomyopathy and heart failure. J Thorac Cardiovasc Surg 2021;162:829-850.e1. [Crossref] [PubMed]
- Sun X, Huang J, Shi M, et al. Predictors of moderate ischemic mitral regurgitation improvement after off-pump coronary artery bypass. J Thorac Cardiovasc Surg 2015;149:1606-12. [Crossref] [PubMed]
- Huang K, Wang Y, Huang J, et al. Nomogram Predicts Improvement of Ischemic Mitral Regurgitation After Coronary Artery Bypass Grafting. Ann Thorac Surg 2022;114:1656-63. [Crossref] [PubMed]
- Penicka M, Linkova H, Lang O, et al. Predictors of improvement of unrepaired moderate ischemic mitral regurgitation in patients undergoing elective isolated coronary artery bypass graft surgery. Circulation 2009;120:1474-81. [Crossref] [PubMed]
- Fattouch K, Sampognaro R, Speziale G, et al. Impact of moderate ischemic mitral regurgitation after isolated coronary artery bypass grafting. Ann Thorac Surg 2010;90:1187-94. [Crossref] [PubMed]
- Ji Q, Zhao Y, Shen J, et al. Risk Factors for Moderate or More Residual Regurgitation in Patients with Moderate Chronic Ischemic Mitral Regurgitation Undergoing Surgical Revascularization Alone. Int Heart J 2019;60:1268-75. [Crossref] [PubMed]
- Dhore-Patil AS, Aneja A. Role of Cardiovascular Magnetic Resonance in Ischemic Cardiomyopathy. Heart Fail Clin 2021;17:41-56. [Crossref] [PubMed]
- O'Gara PT, Mack MJ. Secondary Mitral Regurgitation. N Engl J Med 2020;383:1458-67. [Crossref] [PubMed]
- Bonow RO, O'Gara PT, Adams DH, et al. 2020 Focused Update of the 2017 ACC Expert Consensus Decision Pathway on the Management of Mitral Regurgitation: A Report of the American College of Cardiology Solution Set Oversight Committee. J Am Coll Cardiol 2020;75:2236-70. [Crossref] [PubMed]
- Neumann FJ, Sousa-Uva M, Ahlsson A, et al. 2018 ESC/EACTS Guidelines on myocardial revascularization. Eur Heart J 2019;40:87-165. [Crossref] [PubMed]
- Lancellotti P, Tribouilloy C, Hagendorff A, et al. Recommendations for the echocardiographic assessment of native valvular regurgitation: an executive summary from the European Association of Cardiovascular Imaging. Eur Heart J Cardiovasc Imaging 2013;14:611-44. [Crossref] [PubMed]
- Cerqueira MD, Weissman NJ, Dilsizian V, et al. Standardized myocardial segmentation and nomenclature for tomographic imaging of the heart. A statement for healthcare professionals from the Cardiac Imaging Committee of the Council on Clinical Cardiology of the American Heart Association. Circulation 2002;105:539-42. [Crossref] [PubMed]
- Garcia MJ, Kwong RY, Scherrer-Crosbie M, et al. State of the Art: Imaging for Myocardial Viability: A Scientific Statement From the American Heart Association. Circ Cardiovasc Imaging 2020;13:e000053. [Crossref] [PubMed]
- Cavalcante JL, Kusunose K, Obuchowski NA, et al. Prognostic Impact of Ischemic Mitral Regurgitation Severity and Myocardial Infarct Quantification by Cardiovascular Magnetic Resonance. JACC Cardiovasc Imaging 2020;13:1489-501. [Crossref] [PubMed]
- Otto CM, Nishimura RA, Bonow RO, et al. 2020 ACC/AHA Guideline for the Management of Patients With Valvular Heart Disease: Executive Summary: A Report of the American College of Cardiology/American Heart Association Joint Committee on Clinical Practice Guidelines. Circulation 2021;143:e35-71. [Crossref] [PubMed]
- Fitzgibbon GM, Kafka HP, Leach AJ, et al. Coronary bypass graft fate and patient outcome: angiographic follow-up of 5,065 grafts related to survival and reoperation in 1,388 patients during 25 years. J Am Coll Cardiol 1996;28:616-26. [Crossref] [PubMed]
- Bretschneider C, Heinrich HK, Seeger A, et al. Impact of Papillary Muscle Infarction on Ischemic Mitral Regurgitation Assessed by Magnetic Resonance Imaging. Rofo 2018;190:42-50. [Crossref] [PubMed]
- Bouma W, Willemsen HM, Lexis CP, et al. Chronic ischemic mitral regurgitation and papillary muscle infarction detected by late gadolinium-enhanced cardiac magnetic resonance imaging in patients with ST-segment elevation myocardial infarction. Clin Res Cardiol 2016;105:981-91. [Crossref] [PubMed]
- Tanimoto T, Imanishi T, Kitabata H, et al. Prevalence and clinical significance of papillary muscle infarction detected by late gadolinium-enhanced magnetic resonance imaging in patients with ST-segment elevation myocardial infarction. Circulation 2010;122:2281-7. [Crossref] [PubMed]