Angiographic quantitative flow ratio in acute coronary syndrome: beyond a tool to define ischemia-causing stenosis—a literature review
Introduction
Given improving clinical outcomes with physiology-guided percutaneous coronary intervention (PCI), international guidelines recommend the use of fractional flow reserve (FFR) or instantaneous wave-free ratio (iFR) for identifying ischemia-causing stenoses requiring revascularization (1,2). However, despite a large body of supporting evidence, the adoption of the conventional physiological assessment is limited in daily practice due to a series of concerns, including increased procedural time and cost, the need for invasive pressure wire advancement and the need to induce hyperemia with adenosine injection (3,4). To overcome these limitations, different physiology techniques derived from the computation of coronary angiography have been developed by multiple groups (5-8), potentially providing new opportunities for wide adoption in the catheterization laboratory. Among these angiography-based simulators to wire-based FFR, quantitative flow ratio (QFR) is currently the one with the largest amount of supporting clinical evidence (6,9-11).
Nevertheless, the well-established coronary physiological approaches of assessing lesion severity and for guiding revascularization in patients with chronic coronary syndrome (CCS) have not been extrapolated to the context of acute coronary syndrome (ACS) (2,12,13). An early PCI of the culprit vessel to restore myocardial perfusion is the current standard of care for patients presenting with ST-segment elevation myocardial infarction (STEMI) (13), or for those with high-risk non-ST-segment elevation acute coronary syndrome (NSTE-ACS) (14). Under these circumstances, it appears unnecessary for the functional assessment of the culprit lesion before interventional treatment, along with the evidence showing that hemodynamic severity of the infarcted-related artery (IRA) evaluated by FFR or QFR at the acute phase of myocardial infarction (MI) may be underestimated due to the presence of microvascular dysfunction (15,16). However, for culprit vessels after stent implantation in ACS, recent studies have revealed the prognostic value of QFR in predicting long-term adverse cardiac outcomes (16,17). In addition, around half of acute myocardial infarction (AMI) patients have multivessel disease (MVD), with angiographically significant stenoses located in non-culprit vessels (18). Multiple clinical trials robustly demonstrated that complete revascularization with PCI of non-culprit lesions (NCLs) under the guidance of coronary angiography or physiology improves outcomes in patients with STEMI and MVD (19-21). Therefore, further application of coronary physiology in ACS patients has been attempted, and angiography-derived QFR, due to its less-invasive nature, may extend the benefits of physiological assessment for this high-risk subset of CAD patients.
ACS represents a broad spectrum of CAD, including patients with STEMI as well as non-ST-segment elevation myocardial infarction (NSTEMI) and unstable angina. As more sensitive cardiac-specific troponin measurements have been widely used, the detection of MI appeared to increase, while the diagnosis of unstable angina showed a reciprocal decrease (14,22). Hence, this review article will summarize the available data of angiographic QFR in different clinical scenarios of ACS, mainly focusing on the acute phase of MI, providing insights toward diagnostic accuracy of QFR for the functional assessment of non-culprit vessels and clinical implications of QFR-derived physiological parameters. We present the following article in accordance with the Narrative Review reporting checklist (available at https://cdt.amegroups.com/article/view/10.21037/cdt-22-334/rc).
Methods
A literature search was performed on the electronic databases, including PubMed, Google Scholar and Web of Science covering publications up to May 2022. The relevant guidelines and original articles published in English language were included. We present the detail of our search strategy in Table 1.
Table 1
Items | Description |
---|---|
Date of search | June 2022 |
Databases searched | PubMed, Google Scholar and Web of Science |
Search terms used (including MeSH and free text search terms and filters) | fractional flow reserve, FFR, quantitative flow ratio, acute coronary syndrome, ACS, acute myocardial infarction, STEMI, myocardial infarction, index of microcirculatory resistance, pullback pressure gradient index, intravascular ultrasound, optical coherence tomography |
Timeframe | Up to May 2022 |
Inclusion and exclusion criteria | All relevant reviews and original articles with focus on the QFR in the setting of ACS and all articles had to be published in English language |
Selection process | The search was conducted independently by JC, HL and DY; data selection is the intersection of the search of these three authors |
FFR, fractional flow reserve; ACS, acute coronary syndrome; STEMI, ST-segment elevation myocardial infarction; QFR, quantitative flow ratio.
Diagnostic performance of QFR: from CCS to ACS
The concept and validation of QFR in CCS
The three key steps in computational estimation of coronary physiology are reconstruction of geometric models from coronary images, applying hemodynamic boundary conditions and selecting fluid dynamics solutions (23). QFR is a well-established approach enabling rapid computation of FFR based on coronary angiography. For QFR computation, geometric model of coronary tree is reconstructed in 3-dimensional (3D) from paired two angiographic projections at least 25° apart. The coronary flow is estimated by frame count-based flow velocity and the pressure drop is calculated based on fluid dynamic equations (6,9). The FAVOR (Functional Assessment by Various Flow Reconstructions) pilot study (6) was the first to show the feasibility and diagnostic accuracy of QFR in identifying flow-limiting stenosis in core-lab setting. Notably, QFR was calculated using 3 flow models based on the different hyperemic flow velocities in this study. Fixed-flow QFR (fQFR) is calculated based on the population-averaged fixed empiric hyperemic flow velocity of 0.35 m/s. Adenosine-flow QFR (aQFR) and contrast-flowed QFR (cQFR) are acquired using TIMI frame count on coronary angiography during the setting with and without drug-induced hyperemia, respectively. All three QFR approaches showed good diagnostic accuracy in identifying functionally significant lesions. Importantly, cQFR, which was computed without pharmacologically induced hyperemia, showed no significant difference in predicting FFR compared with aQFR, resulting in a wide adoption of the cQFR model in subsequent validation studies and current practice.
Similar results were observed in three major prospective validations (9-11), as summarized in Table 2 (6,9-11,24,25). Overall, in all three trials, QFR has a high area under of the curve (AUC) for predicting an FFR ≤0.80, ranging from 0.85 for WIFI II (Wire-Free Functional Imaging II) trial (11), to 0.92 and 0.96 for FAVOR II Europe-Japan (10) and FAVOR II China study (9), respectively. Furthermore, the measurement time of online QFR was significantly shorter than FFR [5.0 minutes (3.5–6.1) vs. 7.0 minutes (5.0–10.0)] (10). An individual patient-level meta-analysis, which included 819 patients with 969 analyzable vessels from the aforementioned four prospective trials, revealed that the diagnostic performance of QFR was 84% sensitivity, 88% specificity, a positive predictive value of 80%, and a negative predictive value of 95% (24) (Table 2). Notably, these early validation studies mainly focused on selected patients with stable clinical scenarios and intermediate lesions, and those who presented with MI at the acute phase were ineligible for inclusion (9-11). Although FAVOR II China and FAVOR II Europe-Japan studies included patients scheduled for secondary evaluation of NCLs after 72 hours of primary PCI, only 14 of the 308 patients and 6 of the 272 patients, respectively, presenting with AMI entered the final analysis, limiting its validity in ACS setting (9,10).
Table 2
Trial | FAVOR Pilot (6) | FAVOR II China (9) | FAVOR II E-J (10) | WIFI II (11) | Meta-analysis (24) | μQFR study (25) |
---|---|---|---|---|---|---|
Year | 2016 | 2017 | 2018 | 2018 | 2019 | 2021 |
No. of patients | 73 | 308 | 272 | 172 | 819 | 306 |
No. of vessels | 84 | 332 | 317 | 255 | 969 | 330 |
Population | Patients from FAVOR Piolt/II China/E-J and WIFI II | Patients from FAVOR II China | ||||
Stable CAD | 100% | 22.4% | 98% | 100% | ||
UA | 66.5% | NA | ||||
AMI | 4.5% | 2% | ||||
Diameter stenosis | 46.1±8.9% | 46.5±11.3% | 45±10% | 50±12% | 44.8±12.6% | NA |
Simulators | cQFR | cQFR | cQFR | cQFR | cQFR | μQFR |
Company | Medis Medical | Pulse Medical | Medis Medical | Medis Medical | Medis & Pulse | Pulse Medical |
Analysis time (min) | NA | 4.36±2.55 | 5.0 (3.5–6.1) | NA | NA | 1.1±0.4 |
Mean FFR | 0.84±0.08 | 0.82±0.12 | 0.83±0.09 | 0.82±0.11 | 0.83±0.10 | 0.82±0.12 |
FFR ≤0.80 | 32% | 34% | 33% | 36% | 35% | 36% |
AUC | 0.92 (0.85–0.97) | 0.96 (0.94–0.98) | 0.92 (0.89–0.96) | 0.86 (0.81–0.91) | 0.92 (0.90–0.95) | 0.97 (0.95–0.99) |
Accuracy, % | 86 [78–93] | 93 [89–95] | 87 [NA] | 83 [NA] | 87 [85–89] | 93 [90–96] |
Sensitivity, % | 74 [54–89] | 95 [89–98] | 87 [78–92] | 77 [66–85] | 84 [77–90] | 88 [80–93] |
Specificity, % | 91 [81–97] | 92 [87–95] | 87 [82–91] | 86 [79–91] | 88 [84–91] | 96 [93–98] |
PPV, % | 80 [59–93] | 86 [78–91] | 76 [68–84] | 75 [65–84] | 80 [76–85] | 93 [87–97] |
NPV, % | 88 [77–95] | 97 [94–99] | 93 [89–96] | 87 [80–92] | 95 [93–96] | 93 [89–96] |
Data are expressed as mean ± SD, median (25th, 75th percentiles), or n%. CAD, coronary artery disease; UA, unstable angina; AMI, acute myocardial infarction; AUC, area under of the curve; PPV, positive predictive value; NPV, negative predictive value; FFR, fractional flow reserve; QFR, quantitative flow ratio; cQFR, contrast-flow QFR; μQFR, Murray law-based QFR; NA, no acquired.
Validation studies of QFR in ACS
As mentioned previously, the use of invasive FFR in the acute setting of MI is inconvenient due to several inherent limitations. From a practical standpoint, angiography-derived QFR, which can be easily obtained during or after primary PCI with no requirement for additional invasive procedures or administration of coronary vasodilators that carry side effects, may offer an attractive alternative for this unmet clinical need. Furthermore, QFR, as a research tool, can be computed both offline and online, allowing clinicians to wildly review available angiograms from a functional perspective. With these advantages, accumulating studies have investigated the diagnostic value of QFR for the functional assessment of NCL in ACS (Table 3) (26-35).
Table 3
First author, year | Population | No. of patients [vessels] | Reference | Staged time* | AUC | Accuracy % | Sensitivity % | Specificity % | PPV % | NPV % |
---|---|---|---|---|---|---|---|---|---|---|
Acute coronary syndrome | ||||||||||
Spitaleri 2018 (26) | STEMI | 45 [49] | Index FFR | – | 0.96 | 94 | 88 | 97 | 94 | 94 |
Sejr-Hansen 2019 (27) | STEMI | NA [70] | Staged QFR | 13 days | NA | 93 | 92 | 94 | 94 | 94 |
Staged FFR | 13 days | 0.89 | 84 | 83 | 84 | 81 | 96 | |||
Staged iFR | 13 days | 0.81 | 74 | 73 | 74 | 69 | 78 | |||
Tebaldi 2020 (28) | NSTEMI | 116 [184] | Index FFR | – | 0.96 | 88 | 72 | 94 | 81 | 90 |
Erbay 2021 (29) | ACS | 321 [513] | Staged QFR | 49 days | NA | 94 | 95 | 94 | 91 | 97 |
Milzi 2021 (30) | AMI | 220 [280] | Staged FFR, stress CMR or SPECT | Within 6 months | 0.89 | NA | 84 | 86 | NA | NA |
All-comers | ||||||||||
Lauri 2019 (31) | STEMI | 82 [91] | Staged FFR | 8 days | 0.91 | 84 | 80 | 86 | 78 | 87 |
SA | 69 [91] | FFR | – | 0.94 | 89 | 87 | 90 | 90 | 90 | |
Choi 2020 (32) | All-comers | 452 [559] | FFR | – | 0.95 | 91 | 92 | 87 | 87 | 95 |
ACS | 117 [153] | Index FFR | – | 0.95 | 91 | 90 | 85 | 85 | 94 | |
Stable CAD | 335 [446] | FFR | – | 0.96 | 92 | 96 | 89 | 89 | 96 | |
Kirigaya 2021 (33) | STEMI | 50 [65] | Staged QFR | 14 days | 0.97 | 92 | 89 | 95 | 92 | 92 |
Stable CAD | 77 [95] | FFR | – | 0.88 | 85 | 80 | 91 | 91 | 80 | |
Lee 2021 (34) | All-comers | 915 [1,077] | FFR | – | 0.98 | 96 | 94 | 96 | 94 | 96 |
AMI | 103 [132] | FFR | NA | 0.97 | 92 | 93 | 92 | 93 | 92 | |
Angina | 812 [945] | FFR | – | 0.98 | 96 | 94 | 97 | 94 | 97 | |
Hwang 2019 (35) | AMI | 82 [105] | FFR | NA | 0.97 | 93 | 96 | 91 | 91 | 96 |
Stable CAD | 182 [253] | FFR | – | 0.95 | 90 | 90 | 90 | 83 | 94 |
*, staged time indicated the time between secondary procedures in nonculprit arteries and index procedures in culprit lesions. AUC, area under of the curve; PPV, positive predictive value; NPV, negative predictive value; STEMI, ST-segment elevation myocardial infarction; SA, stable angina; NSTEMI, non-ST-segment elevation myocardial infarction; CAD, coronary artery disease; ACS, acute coronary syndrome; AMI, acute myocardial infarction; FFR, fractional flow reserve; QFR, quantitative flow ratio; iFR, instantaneous wave-free ratio; CMR, cardiac magnetic resonance; SPECT, single-photon emission computed tomography; NA, no acquired.
Spitaleri et al. (26), for the first time, validated the role of QFR in identifying flow-limiting NCL in patients with STEMI and MVD. The researchers found, based on two independent cohorts consisting of 34 and 49 NCLs, that QFR values showed excellent reproducibility between the timing of index and staged procedures, and had a good diagnostic accuracy with FFR measurement as standard reference. This was further supported by multiple prospective trials (27,28) or retrospective observational studies (29,30). An iSTEMI substudy (27) compared the diagnostic performance of acute QFR in 70 NCLs with staged (median 13 days after primary PCI) QFR, iFR and FFR, and reported a moderate to excellent classification agreement, varying from 74% [95% confidence interval (CI): 65–83%] for staged iFR, 84% (95% CI: 76–90%) for staged FFR to 93% (95% CI: 87–99%) for staged QFR. In patients with NSTEMI undergoing primary PCI, QFR also displayed an excellent diagnostic correlation with FFR [AUC 0.964 (95% CI: 0.903–0.974)], which is equivalent to the accuracy of non-hyperemic pressure ratios (28). Recently, two large observational studies (29,30) with the inclusion of both STEMI and NSTE-ACS patients, further proved the feasibility and effectiveness of QFR analysis derived from acute angiograms to assess the hemodynamic relevance of NCLs, as judged by staged QFR (29) or planned standard ischemia testing (30) including invasive FFR, non-invasive stress cardiac magnetic resonance imaging (CMR) and single-photon emission computed tomography. Moreover, Dettori et al. (36) recently revealed that QFR in NCLs of patients with previous MI exhibited a good diagnostic value for determining not only the presence, but also the extent and severity of myocardial ischemia assessed by staged CMR.
Interestingly, the diagnostic accuracy and AUC of QFR derived in ACS patients from the studies above were numerically similar to those reported in studies focusing on stable patients (9,11). In order to directly compare the QFR diagnostic value in different clinical scenarios, Lauri et al. (31) retrospectively investigated 82 patients (91 NCLs) with STEMI and a propensity matched group of 69 stable angina patients (91 target vessels). It was shown that QFR applied to NCL during primary PCI had a comparable diagnostic performance compared with the stable CAD group [AUC 0.91 (0.85–0.97) vs. 0.94 (0.89–0.99), P=0.499], using staged FFR as the reference standard. Similar results were reported in several real-world studies among all-comer patients with CAD (32-34), showing that high diagnostic accuracy and agreement of QFR to predict FFR regardless of various clinical settings including acute STEMI (32-34), NSTE-ACS (32,34) or prior MI (32,37). In another study by Hwang et al. (35), QFR showed an excellent correlation (r=0.863 with FFR vs. 0.740 with iFR) and discrimination (AUC =0.953 with FFR vs. 0.880 with iFR) with respect to both FFR and iFR in patients with SCAD or AMI. However, the diagnostic performance of QFR was better when using FFR as the reference standard, probably because the simulated coronary flow model of QFR was originally proposed to mimic FFR.
Limitations and pitfalls of QFR measurement in ACS
Although accumulating favorable data have been released on the feasibility and effectiveness of QFR when applied to an ACS setting, several potential limitations and pitfalls should be noted in practice. First, the current evidence from the validation studies might not extrapolated to the overall group of ACS patients, i.e., the patients with hemodynamic instability requiring vasopressors or assist devices. In addition, not all the vessels were interrogated for the enrolled patients. QFR was not assessed in IRA because primary PCI of such vessels guided by angiography was still the standard treatment (13,14). As there is general agreement that coronary physiology should be applied to intermediate lesions with diameter stenosis (DS) of 30% to 90%, studies regarding QFR adoption in ACS applied similar criteria of lesion selection (27,35). Technically, the feasibility and diagnostic accuracy of QFR, as an angiography-derived method, depends on the quality of images. Despite using optimal images and projections, severe vessel overlapping, tortuosity or ostial disease in main vessels remains challenging for the QFR computations. Encouragingly, Murray law-based QFR (µQFR) (25), the next generation of QFR, was developed to calculate FFR from a single angiographic view, and showed a comparable diagnostic performance with the existing QFR required two angiographic projections (Table 1). This would, to some extent, optimize the process of QFR computation and enable wider accessibility of physiological assessment in some circumstances.
As the high incidence of severe coronary microvascular dysfunction (CMD) in the acute phase of MI, there has been concern about the utility of functional assessment in this clinical situation (38). Mejía-Rentería et al. (39) found that QFR showed lower diagnostic performance in patients with CMD, as assessed by index of microcirculatory resistance (IMR). In this study, the proportion of ACS was 30%, and NCLs were investigated. In the context of STEMI after PCI of culprit and non-culprit lesions, Tang et al. (16) found that post-PCI QFR value at index procedure was significantly decreased at the staged PCI in CMD group (0.940±0.088 vs. 0.928±0.094, P<0.001), while no statistical difference was found in patients without CMD (0.916±0.107 vs. 0.918±0.099, P=0.64). Future prospective trials are needed to explore the effect of microvascular circulation on the behavior of QFR accuracy using integrated physiological modalities, such as coronary flow reserve and CMR. Lastly, the diagnostic accuracy of NCL using QFR during ACS decreased close to the FFR cutoff of 0.80 (31), in line with the results observed in stable CAD patients (11). To maximize the accuracy of QFR, some researchers proposed a hybrid QFR-FFR approach, in which invasive FFR testing is only performed for lesions with QFR value within the “grey zone” (between 0.75 and 0.85) (29,31,40). Although the boundaries of QFR “grey zone” are not well defined, this hybrid strategy may provide useful information for clinical decision making with a substantial impact on patient’s comfort and cost-effectiveness.
Prognostic implications of QFR in ACS
The majority of the related evidence regarding the clinical significance of coronary physiology in ACS is derived from population presented with STEMI and MVD. A number of randomized controlled trials (RCTs) (19,41-43) and meta-analyses (44,45) have shown a reduction in major adverse cardiovascular events (MACE) with complete revascularization compared with the strategy of IRA-only PCI for STEMI patients. Accordingly, the latest American College of Cardiology and the American Heart Association guidelines on myocardial revascularization listed a class IA recommendation for PCI of NCLs, either guided by FFR or angiography, among STEMI patients with hemodynamically stable condition (2). Moreover, Strong evidence favoring the use of FFR in comparison to angiography revascularization has been gained from stable CAD (46,47). Of importance, intermediate stenoses, which may benefit from functional assessment, account for around 70% of coronary lesions located in non-culprit vessels in ACS (18,48). For these reasons, completeness of revascularization guided by coronary physiology in ACS patients has been attempted. However, the recent FLOWER-MI (Flow Evaluation to Guide Revascularization in Multi-Vessel ST-Elevation Myocardial Infarction) trial showed that FFR-guided PCI was not superior on both clinical and economic endpoints compared to angiography-guided PCI in STEMI, although the statistical power was inadequate and follow-up limited to a 1-year duration (21,49). Whether benefits can be achieved using physiological approaches among ACS patients remains controversial. In this section, we will discuss the clinical implications of QFR assessment before and after the procedure in ACS.
Prognostic value of QFR measurement in ACS
The recently published studies focusing on the prognostic value of QFR in patients with ACS are presented in Table 4 (16,17,32,50-57). A study by Choi et al. (32) enrolling 452 all-comer patients with CAD (25.9% with ACS) demonstrated that vessels with QFR ≤0.80 showed a significantly higher risk of vessel-related adverse events compared to those with QFR >0.80 at 2-year [hazard ratio (HR) 4.650, 95% CI: 1.254–17.240, P=0.022]. Similarly, a patient-level sub-analysis of randomized PANDA III (Comparison of BuMA eG Based BioDegradable Polymer Stent With EXCEL Biodegradable Polymer Sirolimus-eluting Stent in “Real-World” Practice) trial (50), evaluating QFR in 1,391 patients (82.7% with ACS) undergoing angiography-guided PCI, documented that patients receiving physiology-consistent PCI based on baseline QFR had a lower risk of 2-year MACE than patients with QFR-inconsistent treatment (8.4% vs. 14.7%, P<0.001). Notably, approximately 41% of patients were treated with PCI under the guidance of angiography, however, not in accordance with QFR-recommended revascularization (vessels with baseline QFR ≤0.80 were treated with PCI and vessels with baseline QFR >0.80 were deferred). In an acute STEMI setting, Bär et al. (51) conducted QFR analyses in 946 untreated nontarget vessels among 617 patients undergoing primary PCI and angiography-guided complete revascularization. The results showed QFR ≤0.80 in non-target vessel was associated with a higher risk of MACE at 5-year follow-up (HR 7.33, 95% CI: 4.54–11.83, P<0.001). Differences were mainly driven by spontaneous nontarget vessel MI and nontarget vessel revascularization. Of note, QFR was calculated for all eligible nontarget vessels regardless of the degree of DS, which leads to a relatively low proportion (3.8%) of vessels with QFR ≤0.80. Overall, these findings suggest the incremental prognostic value of QFR over angiography alone in NCL assessment in ACS patients.
Table 4
First author, year | Population | Primary endpoint | Comparison | No. of patients [vessels] | Result |
---|---|---|---|---|---|
Pre-interventional QFR | |||||
Erbay 2021 (17) | Patients with ACS after primary PCI | 2-yr POCE (death, nonfatal MI, IDR) | Nonculprit vessels with QFR ≤0.85 vs. >0.85 | 792 [1,231] | 23.0% vs. 2.2%, P<0.001 |
Choi 2021 (32) | All-comer patients with ≥1 intermediate stenoses (25.9% with ACS) | 2-yr VOCE (vessel-related cardiac death, MI, ischemia-driven TLR) | Vessels with QFR ≤0.80 vs. QFR >0.80 | 452 [599] | 4.2% vs. 0.9%, P=0.022 |
Zhang 2021 (50) | All-comer patients underwent angiography-guided PCI (82.7% with ACS) | 2-yr POCE (death, any MI, IDR) | QFR-consistent vs. QFR-inconsistent treatment group | 1391 [2,543] | 8.4% vs. 14.7%, P<0.001 |
Bär 2021 (51) | Patients with STEMI ≥1 untreated non-target vessel after angiography-guided CR | 5-yr POCE (cardiac death, spontaneous non-TV-MI, non-TVR) | Patients with QFR ≤0.80 vs. QFR >0.80 | 617 [946] | 62.9% vs. 12.5%, P<0.001 |
Zhang 2021 (52) | Patients with STEMI and multivessel disease | 1-yr POCE (death, nonfatal MI, IDR) | QFR-guided functional CR vs. IRA-only group | 229 [NA] | 9.6% vs. 20.1, P=0.025 |
Post-interventional QFR | |||||
Tang 2021 (16) | Patients with STEMI and multivessel disease | 2-yr VOCE (vessel-related cardiac death, MI, TVR) | Vessels with post-PCI QFR ≤0.91 vs. >0.91 | 186 [415] | 20.8% vs. 5.7%, P<0.001 |
Erbay 2021 (17) | Patients with ACS after primary PCI | 2-yr POCE (death, nonfatal MI, IDR) | Post-PCI culprit vessels with QFR ≤0.89 vs. >0.89 | 792 [792] | 25.6% vs. 4.8%, P<0.001 |
Kogame 2019 (53) | Stable CAD Patients with 3 vessel disease | 2-yr VOCE (vessel-related cardiac death, MI, TVR) | Vessels with post-PCI QFR <0.91 vs. ≥0.91 | 393 [771] | 12.0% vs. 3.7%, P<0.001 |
Biscaglia 2019 (54) | Patients with stable CAD or NSTE-ACS | 2-yr VOCE (vessel-related cardiac death, MI, IDR) | Vessels with post-PCI QFR ≤0.89 vs. >0.89 | 602 [751] | 25% vs. 3.5%, P<0.001 |
QFR-derived functional SYNTAX score | |||||
Spitaleri 2018 (26) | Patients with STEMI with ≥1 untreated non-culprit lesion | 5-yr POCE (death, any MI, any revascularization) | Functional IR vs. functional CR guided by QFR-derived residual FSS | 110 [NA] | 46% vs. 24%, P=0.01 |
Asano 2019 (55) | All-comer patients with de novo 3 vessel disease (excluded left main disease) | 2-yr POCE (death, any MI, any revascularization) | low-risk vs. intermediate-risk vs. high-risk group classified by QFR-derived FSS | 386 [836 lesions] | 3.7% vs. 11.0% vs. 19.0%, P=0.05 |
Zhang 2020 (56) | Patients with left main or multivessel disease (56.6% with UA and 28.3% with AMI) | 2-yr POCE (death, any MI, IDR) | low-risk vs. intermediate-risk vs. high-risk group classified by QFR-derived FSS | 607 [NA] | 9.1% vs. 13.5% vs. 22.3%, P=0.0004 |
Tang 2020 (57) | Patients with STEMI with ≥1 non-culprit lesion after successful PCI of culprit lesion | 2-yr POCE (death, any MI, IDR) | Functional IR vs. functional CR guided by QFR-derived residual FSS | 354 [NA] | 22.0% vs. 7.4%, P<0.001 |
ACS, acute coronary syndrome; PCI, percutaneous coronary intervention; QFR, quantitative flow ratio; POCE, patient-oriented composite endpoints; ACS, acute coronary syndrome; VOCE, vessel-oriented composite endpoints; MI, myocardial infarction; TLR, target lesion revascularization; IDR, ischemia-driven revascularization; STEMI, ST-segment elevation myocardial infarction; CR, complete revascularization; TVR, target vessel revascularization; IRA, infarcted-related artery; NSTE-ACS, non-ST-segment elevation acute coronary syndrome; CAD, coronary artery disease; AMI, acute myocardial infarction; UA, unstable angina; IR, incomplete revascularization; FSS, functional SYNTAX score; NA, no acquired.
Another important clinical scenario to perform physiological assessment is in the catheterization laboratory after the index procedure. Multiple studies have used FFR to ascertain the prognostic relevance of post-PCI functional assessment in patients with stable CAD and ACS (58-60). Accordingly, investigators attempted to explore the clinical value of post-procedural QFR in various clinical settings. Two post-hoc analyses of multicenter prospective studies—SYNTAX II and HAWKEYE (Angio-based Fractional Flow Reserve to Predict Adverse Events After Stent Implantation)—confirmed that post-PCI QFR was feasible and could offer prognostic information with procedural results and clinical outcomes (Table 4) (53,54). However, these studies mainly involved CCS patients, excluding patients in the acute phase of MI. Recently, Tang et al. (16) analysed QFR after stent implantation in 415 vessels included both culprit and nonculprit lesions from 186 STEMI patients, and found that post-PCI QFR <0.91 was associated with subsequent vessel-oriented cardiovascular events. Furthermore, Erbay et al. (17) evaluated the prognostic implication of pancoronary QFR assessment in a large cohort of 792 ACS patients and first reported post-PCI QFR ≤0.89 for culprit vessels as a best cut-off value to predict MACE at 2 years. Although the optimal post-PCI physiological thresholds of culprit and nonculprit vessels need to be further validated in future studies, QFR may provide a valuable tool for evaluating and optimizing PCI results, as the index can be easily obtained from routine coronary angiograms after PCI.
QFR-derived functional SYNTAX score and functional complete revascularization
The SYNTAX score (SS), which can objectively grade the anatomic complexity of CAD, was prospectively derived from the SYNTAX (Synergy Between Percutaneous Coronary Intervention With Taxus and Cardiac Surgery) trial and remains the most widely used and validated risk score to guide revascularization strategy in patients with MVD (61,62). Besides, it was reported that the functional SS (FSS), which was calculated by counting only flow-limiting stenosis with FFR ≤0.8, had a better discriminant ability in predicting clinical outcomes compared with anatomic SS (63). Building on this concept, the hypothesis that QFR-based FSS may improve risk classification and subsequently influence the treatment decision for patients with MVD was proved in subanalyses of the SYNTAX II and PANDA III trials (55,56) (Table 4). Due to the retrospective manner, many angiographic images were not suitable for QFR analysis in all 3 vessels, resulting in QFR-based FSS was acquired in only 28.2% and 53.7% of the entire population, respectively. Nevertheless, these studies both demonstrated the applicability of calculating a QFR-based FSS and validated its potential for predicting future cardiovascular events. Spitaleri et al. (26) further introduced, on the basis of a small cohort of 110 STEMI patients following successful primary PCI, a residual FSS guided by QFR (only NCL with QFR ≤0.80 and left untreated was taken into account in the SS calculation) to define functional complete revascularization (QFR-based residual FSS =0). The results revealed that, at 5-year follow-up, the risk of MACE (a combined incidence of death, MI or any revascularization) was significantly reduced in patients with functional complete revascularization (HR 2.3, 95% CI: 1.2–4.5, P=0.01). By applying this scoring system, Tang et al. (57) consistently confirmed, in a single-center study of 354 STEMI patients, the 2-year benefits in MACE of QFR-guided functional complete over incomplete revascularization (7.4% vs. 22.0%, P<0.001). Therefore, the improved scoring system combining anatomic and QFR-based physiological assessment might be a fast and feasible tool for risk stratification and PCI guidance in ACS patients with MVD.
Randomized clinical trial data
At present, neither of novel angiography-derived physiological technologies are recommended for routine use in clinical practice guidelines due to the absence of robust clinical trial evidence. More recently, the results of the FAVOR III China trial comparing outcomes of patients undergoing QFR-guided and angiography-guided PCI were reported, allowing QFR become the first angiographic flow measurement for PCI guidance with RCT data (64). A total of 3,825 patients with stable CAD or ACS [2,428 (63.5%) patients with ACS, including 207 patients with post-MI within 30 days] were randomly assigned to the QFR-guided or the angiography-guided group. At 1-year follow-up, the QFR-guided strategy was associated with a reduction in the primary endpoint MACE, a composite of death from any cause, MI, or ischemia-driven revascularization (IDR), compared with angiography-guidance alone (5.5% vs. 8.8%, HR 0.65, 95% CI: 0.51–0.83, P=0.004). The difference was driven by a substantial reduction in MI and IDR. Of importance, QFR assessment changed the treatment plan for 445 (23.3%) of 1,913 patients, mainly due to the treatment deferral of angiographically severe obstructive lesions originally intended for PCI, and thus reduce the number of stents implanted. In another prospective study, which randomized 229 patients with STEMI and MVD to QFR-guided complete revascularization vs. IRA-only treatment, showed QFR guidance was associated with a reduction in MACE at 1 year (9.6% vs. 20.1%, P=0.025) (52). These studies provide evidence that QFR may serve as a valuable tool for decision-making in the catheterization laboratory in addition to wire-based FFR and iFR.
QFR-based physiological assessment of CAD pattern
The mechanisms underlying suboptimal functional results after PCI are different, mainly due to the unsatisfactory stent deployment and the presence of residual diffuse disease (65). In recent years, there have been increasing efforts to characterize functional patterns of coronary atherosclerotic disease by using FFR or iFR pullback (66-68). A proof-of-concept study by Nijjer et al. (66) demonstrated that visual assessment of the iFR pullback tracing can physiologically map the entire coronary artery and can predict functional result after PCI in serial or diffuse lesions. Recently, Collet et al. (68) proposed the pullback pressure gradient (PPG) index derived from the motorized FFR pullback as a quantitative parameter to objectively discriminate between focal and diffuse disease. The PPG index is a continuous metric with higher values close to 1 indicate physiologically focal disease, whereas lower values close to 0 represent physiologically diffuse disease. As QFR software provides the virtual pullback curve by depicting pressure drop in each vessel segment, it is possible to calculate QFR-based PPG index without pressure wire. This concept has been recently validated by Biscaglia et al. (69) and Shin et al. (70), showing that the PPG index derived from QFR is feasible and reproducible in the discrimination of CAD patterns, and can predict post-PCI FFR or QFR results. Although preliminary and limited to a subset of CCS patients, these results, if further validated in various clinical settings, indicated that QFR-derived index of functional atherosclerotic pattern might be applicable for predicting PCI results and assisting lesions-specific treatment decision.
QFR-based physiological assessment of microcirculatory resistance
Coronary angiography as well as FFR and its simulators are tools to detect ischemic heart disease and myocardial ischemia attributed solely to epicardial stenoses. There is now greater recognition that CMD is emerging as another potential cause of angina (71). Therefore, various methods have been developed for microcirculatory assessment in the catheterization laboratory, and one of the most widely used indices is the IMR (72). However, similar to FFR, the adoption of IMR is extremely low in clinical practice because of the additional need for pressure-temperature sensor wire and hyperemic agents. With the recent development of functional coronary angiography techniques, researchers have proposed numerous wire-free parameters to estimate IMR using different methodologies (73-75). The series of subanalyses of the OxAMI (Oxford Acute Myocardial Infarction) study demonstrated the feasibility of calculating microcirculatory resistance based on the QFR system (labelled as IMRangio) and validated its diagnostic performance by comparison with wire-based IMR across patients with STEMI, NSTE-ACS and stable CAD (73,76). A later study from the same group, in 262 STEMI patients with primary PCI, found that non-hyperemic IMRangio of IRA with a cutoff value of 43U, independently predicted the long-term outcomes (77). These findings were further confirmed by Choi et al. (78) in a larger cohort with 10-year clinical follow-up data using another angiography-based method to derive IMR. Even if these data are preliminary, it seems that integrating angiography-derived QFR and IMR might be helpful for differentiating epicardial and microvascular disease, enabling us to acquire important information in a variety of scenarios, especially for the setting of MI with non-obstructive coronary artery disease.
QFR and intravascular imaging-defined plaque vulnerability
Intracoronary imaging techniques, such as intravascular ultrasound (IVUS) and optical coherence tomography (OCT), are well-established methods for the assessment of coronary plaque vulnerability that can increase the risk of future cardiovascular events (79-81). Several studies have revealed the clinical association between vulnerable plaque features and coronary physiological parameters, including wire-based FFR and angiographic QFR (82-84). Kanno et al. (84) found that QFR severity was associated with a higher prevalence of OCT-defined thin-cap fibroatheroma (TCFA) in stable patients. Similar findings were reported by Dan et al. (85) and Zuo et al. (86), showing that lower QFR value was related to IVUS or OCT-defined vulnerable plaque features (i.e., minimal lumen area, plaque burden and TCFA) in both stable angina and NSTEMI patients. However, in a recent study of 87 patients with CCS, QFR was not associated with features of plaque vulnerability such as fibrous cap thickness, although significant correlations were found between QFR and OCT-derived intraluminal stenosis parameters (87). Indeed, the complex interactions exist between coronary physiology and morphological plaque characteristics, which may differ in hyperemic, non-hyperemic or computational physiological indices (88). More recently, Zeng et al. (89) demonstrated that high lipid burden was associated with a numerical higher FFR value compared with OCT-derived computational FFR in assessing functional severity of coronary stenosis, which might be caused by larger luminal deformation of lipid-rich plaque.
Notably, the vulnerable plaque features were more commonly detected in the non-culprit vessels of ACS patients than the target vessels of patients with stable CAD, which partly led to a worse outcome for ACS patients after deferral of NCLs based on functional assessment (90,91). On the other hand, a post hoc analysis of the PROSPECT (Providing Regional Observations to Study Predictors of Events in the Coronary Tree) and IBIS4 (Intergraded Biomarkers Imaging Study 4) studies revealed that QFR could provide additional prognostic information in predicting 5-year MACE beyond virtual histology IVUS-based plaque characteristics (92). These findings suggest coronary physiology and plaque morphology have independent significances on clinical outcomes, and thus integrative evaluation of these 2 aspects of coronary plaque may provide a better understanding of the pathology of ACS. Recent advances in technology have enabled the fast computation of FFR from IVUS [denoted as ultrasonic flow ratio (UFR)] or OCT [denoted as optical flow ratio (OFR)], allowing assessment of both plaque morphology and coronary physiology without pressure wire and induced hyperemia. The accuracy of UFR and QFR were 92% and 90%, respectively, in the prediction of FFR ≤0.80 in the retrospective validation studies (93,94). The angiography-based QFR and intracoronary imaging-derived physiological indices are both computational approaches to determine the functional severity of the coronary stenosis. The fundamental difference between them is in the geometric reconstruction and the estimated hyperemic flow. A fixed hyperemic flow velocity was used for UFR/OFR computation because information on coronary flow can not be acquired from intracoronary images. However, IVUS or OCT images with high resolution provide more accurate lumen geometry than angiographic images. A recent study by Huang et al. (95) demonstrated that OFR was superior to QFR in the identification of flow-limiting coronary stenosis. Moreover, in the subset of patients with prior MI, the diagnostic performance of OFR was not influenced and significantly better than QFR. The recent published outcome study enrolling 604 patients with ACS showed that the presence of OFR ≤0.84 and lipid-to-cap ratio (a new morphologic parameter to define vulnerable plaque) >0.33 were both associated with an increased risk of nonculprit vessel-related MACE at 2 years. Importantly, the combination of lipid-to-cap ratio and OFR in NCLs significantly improved the prognostic performance in predicting future cardiovascular events (96). This indicates comprehensive morphofunctional profiling of coronary plaques may provide valuable information for clinician to aid decisions on revascularization in the catheterization laboratory.
Future perspectives
Although the diagnostic accuracy of QFR with FFR has been demonstrated in multiple studies, and its clinical value in comparison with angiography was shown in FAVOR III China trial, the clinical outcome data are still insufficient, particularly for high-risk ACS patients. The ongoing FAVOR III European-Japan randomized trial (NCT03729739) will directly compare the outcomes of QFR-guided and FFR-guided revascularization in 2000 patients presenting with both acute and chronic coronary syndromes. The QFR-STEMI (Quantitative Fractional Ratio-guided Revascularization in STEMI Patients With Multi-vessel Disease, NCT04259853) and QUOMODO (QUantitative Flow Ratio Or Angiography for the assessMent of nOn-culprit Lesions, NCT04808310) (97) trials will focus on the management of NCLs in STEMI.
In particular, various techniques derived from QFR have been proposed to comprehensively evaluate coronary circulation and atherosclerotic lesion characteristics, i.e., post-PCI QFR for assessing PCI results, QFR-based PPG index for determining physiological disease pattern and angiography-derived IMR for identifying microcirculatory dysfunction (Figure 1). Despite pilot studies showing promising clinical value of these new technologies, more studies are required for further validation. Intracoronary imaging modalities can provide information on the pathobiology of ACS. For this high-risk subset of CAD patients, the novel UFR or OFR, if further confirmed in prospective studies, might be a useful adjunctive tool for operators in the diagnosis and treatment of ACS during cardiac catheterization.
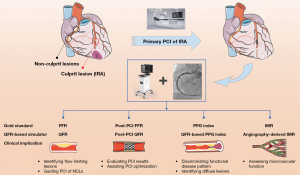
Conclusions
An emerging body of evidence indicates that angiography-derived QFR bears the potential of a wider adoption of coronary physiology assessment due to its nature of being less-invasive, time-saving and economy-friendly. In parallel, multiple technologies, i.e., QFR-based PPG index, angiography-derived IMR and intravascular imaging-based morphofunctional evaluation methods, have been proposed, allowing operators to easily obtained physiological data of micro and macro-circulation, together with atherosclerotic lesion characteristics in catheterization laboratories. Future studies are needed to further explore the benefits of these approaches on outcomes in ACS.
Acknowledgments
Funding: This work was supported by the Grant of Shanghai Science and Technology Committee (No.19XD1403300); and Shanghai Municipal Health Commission (No. 2019LJ10).
Footnote
Reporting Checklist: The authors have completed the Narrative Review reporting checklist. Available at https://cdt.amegroups.com/article/view/10.21037/cdt-22-334/rc
Conflicts of Interest: All authors have completed the ICMJE uniform disclosure form (available at https://cdt.amegroups.com/article/view/10.21037/cdt-22-334/coif). XL reports that he receives Grant of Shanghai Science and Technology Committee (No. 19XD1403300); and Shanghai Municipal Health Commission (No. 2019LJ10). The other authors have no conflicts of interest to declare.
Ethical Statement: The authors are accountable for all aspects of the work in ensuring that questions related to the accuracy or integrity of any part of the work are appropriately investigated and resolved.
Open Access Statement: This is an Open Access article distributed in accordance with the Creative Commons Attribution-NonCommercial-NoDerivs 4.0 International License (CC BY-NC-ND 4.0), which permits the non-commercial replication and distribution of the article with the strict proviso that no changes or edits are made and the original work is properly cited (including links to both the formal publication through the relevant DOI and the license). See: https://creativecommons.org/licenses/by-nc-nd/4.0/.
References
- Neumann FJ, Sousa-Uva M, Ahlsson A, et al. 2018 ESC/EACTS Guidelines on myocardial revascularization. Eur Heart J 2019;40:87-165. [Crossref] [PubMed]
- Writing Committee Members. 2021 ACC/AHA/SCAI Guideline for Coronary Artery Revascularization: A Report of the American College of Cardiology/American Heart Association Joint Committee on Clinical Practice Guidelines. J Am Coll Cardiol 2022;79:e21-e129. [Crossref] [PubMed]
- Dattilo PB, Prasad A, Honeycutt E, et al. Contemporary patterns of fractional flow reserve and intravascular ultrasound use among patients undergoing percutaneous coronary intervention in the United States: insights from the National Cardiovascular Data Registry. J Am Coll Cardiol 2012;60:2337-9. [Crossref] [PubMed]
- Parikh RV, Liu G, Plomondon ME, et al. Utilization and Outcomes of Measuring Fractional Flow Reserve in Patients With Stable Ischemic Heart Disease. J Am Coll Cardiol 2020;75:409-19. [Crossref] [PubMed]
- Kornowski R, Lavi I, Pellicano M, et al. Fractional Flow Reserve Derived From Routine Coronary Angiograms. J Am Coll Cardiol 2016;68:2235-7. [Crossref] [PubMed]
- Tu S, Westra J, Yang J, et al. Diagnostic Accuracy of Fast Computational Approaches to Derive Fractional Flow Reserve From Diagnostic Coronary Angiography: The International Multicenter FAVOR Pilot Study. JACC Cardiovasc Interv 2016;9:2024-35. [Crossref] [PubMed]
- Masdjedi K, van Zandvoort LJC, Balbi MM, et al. Validation of a three-dimensional quantitative coronary angiography-based software to calculate fractional flow reserve: the FAST study. EuroIntervention 2020;16:591-9. [Crossref] [PubMed]
- Li J, Gong Y, Wang W, et al. Accuracy of computational pressure-fluid dynamics applied to coronary angiography to derive fractional flow reserve: FLASH FFR. Cardiovasc Res 2020;116:1349-56. [Crossref] [PubMed]
- Xu B, Tu S, Qiao S, et al. Diagnostic Accuracy of Angiography-Based Quantitative Flow Ratio Measurements for Online Assessment of Coronary Stenosis. J Am Coll Cardiol 2017;70:3077-87. [Crossref] [PubMed]
- Westra J, Andersen BK, Campo G, et al. Diagnostic performance of in-procedure angiography-derived quantitative flow reserve compared to pressure-derived fractional flow reserve: the FAVOR II Europe-Japan study. J Am Heart Assoc 2018;7:e009603. [Crossref] [PubMed]
- Westra J, Tu S, Winther S, et al. Evaluation of Coronary Artery Stenosis by Quantitative Flow Ratio During Invasive Coronary Angiography: The WIFI II Study (Wire-Free Functional Imaging II). Circ Cardiovasc Imaging 2018;11:e007107. [Crossref] [PubMed]
- Knuuti J, Wijns W, Saraste A, et al. 2019 ESC Guidelines for the diagnosis and management of chronic coronary syndromes. Eur Heart J 2020;41:407-77. [Crossref] [PubMed]
- Ibanez B, James S, Agewall S, et al. 2017 ESC Guidelines for the management of acute myocardial infarction in patients presenting with ST-segment elevation: The Task Force for the management of acute myocardial infarction in patients presenting with ST-segment elevation of the European Society of Cardiology (ESC). Eur Heart J 2018;39:119-77. [Crossref] [PubMed]
- Collet JP, Thiele H, Barbato E, et al. 2020 ESC Guidelines for the management of acute coronary syndromes in patients presenting without persistent ST-segment elevation. Eur Heart J 2021;42:1289-367. [Crossref] [PubMed]
- Cuculi F, De Maria GL, Meier P, et al. Impact of microvascular obstruction on the assessment of coronary flow reserve, index of microcirculatory resistance, and fractional flow reserve after ST-segment elevation myocardial infarction. J Am Coll Cardiol 2014;64:1894-904. [Crossref] [PubMed]
- Tang J, Chu J, Hou H, et al. Clinical implication of QFR in patients with ST-segment elevation myocardial infarction after drug-eluting stent implantation. Int J Cardiovasc Imaging 2021;37:755-66. [Crossref] [PubMed]
- Erbay A, Penzel L, Abdelwahed YS, et al. Prognostic Impact of Pancoronary Quantitative Flow Ratio Assessment in Patients Undergoing Percutaneous Coronary Intervention for Acute Coronary Syndromes. Circ Cardiovasc Interv 2021;14:e010698. [Crossref] [PubMed]
- Park DW, Clare RM, Schulte PJ, et al. Extent, location, and clinical significance of non-infarct-related coronary artery disease among patients with ST-elevation myocardial infarction. JAMA 2014;312:2019-27. [Crossref] [PubMed]
- Wald DS, Morris JK, Wald NJ, et al. Randomized trial of preventive angioplasty in myocardial infarction. N Engl J Med 2013;369:1115-23. [Crossref] [PubMed]
- Smits PC, Abdel-Wahab M, Neumann FJ, et al. Fractional Flow Reserve-Guided Multivessel Angioplasty in Myocardial Infarction. N Engl J Med 2017;376:1234-44. [Crossref] [PubMed]
- Puymirat E, Cayla G, Simon T, et al. Multivessel PCI guided by FFR or angiography for myocardial infarction. N Engl J Med 2021;385:297-308. [Crossref] [PubMed]
- Giugliano RP, Braunwald E. The year in non-ST-segment elevation acute coronary syndrome. J Am Coll Cardiol 2012;60:2127-39. [Crossref] [PubMed]
- Tu S, Westra J, Adjedj J, et al. Fractional flow reserve in clinical practice: from wire-based invasive measurement to image-based computation. Eur Heart J 2020;41:3271-9. [Crossref] [PubMed]
- Westra J, Tu S, Campo G, et al. Diagnostic performance of quantitative flow ratio in prospectively enrolled patients: An individual patient-data meta-analysis. Catheter Cardiovasc Interv 2019;94:693-701. [Crossref] [PubMed]
- Tu S, Ding D, Chang Y, et al. Diagnostic accuracy of quantitative flow ratio for assessment of coronary stenosis significance from a single angiographic view: A novel method based on bifurcation fractal law. Catheter Cardiovasc Interv 2021;97:1040-7. [Crossref] [PubMed]
- Spitaleri G, Tebaldi M, Biscaglia S, et al. Quantitative Flow Ratio Identifies Nonculprit Coronary Lesions Requiring Revascularization in Patients With ST-Segment-Elevation Myocardial Infarction and Multivessel Disease. Circ Cardiovasc Interv 2018;11:e006023. [Crossref] [PubMed]
- Sejr-Hansen M, Westra J, Thim T, et al. Quantitative flow ratio for immediate assessment of nonculprit lesions in patients with ST-segment elevation myocardial infarction-An iSTEMI substudy. Catheter Cardiovasc Interv 2019;94:686-92. [Crossref] [PubMed]
- Tebaldi M, Biscaglia S, Erriquez A, et al. Comparison of quantitative flow ratio, Pd/Pa and diastolic hyperemia-free ratio versus fractional flow reserve in non-culprit lesion of patients with non ST-segment elevation myocardial infarction. Catheter Cardiovasc Interv 2021;98:1057-65. [Crossref] [PubMed]
- Erbay A, Penzel L, Abdelwahed YS, et al. Feasibility and diagnostic reliability of quantitative flow ratio in the assessment of non-culprit lesions in acute coronary syndrome. Int J Cardiovasc Imaging 2021;37:1815-23. [Crossref] [PubMed]
- Milzi A, Dettori R, Marx N, et al. Quantitative flow ratio (QFR) identifies functional relevance of non-culprit lesions in coronary angiographies of patients with acute myocardial infarction. Clin Res Cardiol 2021;110:1659-67. [Crossref] [PubMed]
- Lauri FM, Macaya F, Mejía-Rentería H, et al. Angiography-derived functional assessment of non-culprit coronary stenoses in primary percutaneous coronary intervention. EuroIntervention 2020;15:e1594-601. [Crossref] [PubMed]
- Choi KH, Lee SH, Lee JM, et al. Clinical relevance and prognostic implications of contrast quantitative flow ratio in patients with coronary artery disease. Int J Cardiol 2021;325:23-9. [Crossref] [PubMed]
- Kirigaya H, Okada K, Hibi K, et al. Diagnostic performance and limitation of quantitative flow ratio for functional assessment of intermediate coronary stenosis. J Cardiol 2021;77:492-9. [Crossref] [PubMed]
- Lee KY, Hwang BH, Kim MJ, et al. Influence of lesion and disease subsets on the diagnostic performance of the quantitative flow ratio in real-world patients. Sci Rep 2021;11:2995. [Crossref] [PubMed]
- Hwang D, Choi KH, Lee JM, et al. Diagnostic agreement of quantitative flow ratio with fractional flow reserve and instantaneous fave-Free ratio. J Am Heart Assoc 2019;8:e011605. [Crossref] [PubMed]
- Dettori R, Frick M, Burgmaier K, et al. Quantitative flow ratio is associated with extent and severity of ischemia in non-culprit lesions of patients with myocardial infarction. J Clin Med 2021;10:4535. [Crossref] [PubMed]
- Emori H, Kubo T, Kameyama T, et al. Diagnostic Accuracy of Quantitative Flow Ratio for Assessing Myocardial Ischemia in Prior Myocardial Infarction. Circ J 2018;82:807-14. [Crossref] [PubMed]
- van de Hoef TP, Nolte F. Impact of hyperaemic microvascular resistance on fractional flow reserve measurements in patients with stable coronary artery disease: insights from combined stenosis and microvascular resistance assessment. Heart 2014;100:951-9. [Crossref] [PubMed]
- Mejía-Rentería H, Lee JM, Lauri F, et al. Influence of Microcirculatory Dysfunction on Angiography-Based Functional Assessment of Coronary Stenoses. JACC Cardiovasc Interv 2018;11:741-53. [Crossref] [PubMed]
- Peper J, van Hamersvelt RW, Rensing BJWM, et al. Diagnostic performance and clinical implications for enhancing a hybrid quantitative flow ratio-FFR revascularization decision-making strategy. Sci Rep 2021;11:6425. [Crossref] [PubMed]
- Engstrøm T, Kelbæk H, Helqvist S, et al. Complete revascularisation versus treatment of the culprit lesion only in patients with ST-segment elevation myocardial infarction and multivessel disease (DANAMI-3—PRIMULTI): an open-label, randomised controlled trial. Lancet 2015;386:665-71. [Crossref] [PubMed]
- Kelbæk H, Høfsten DE, Køber L, et al. Deferred versus conventional stent implantation in patients with ST-segment elevation myocardial infarction (DANAMI 3-DEFER): an open-label, randomised controlled trial. Lancet 2016;387:2199-206. [Crossref] [PubMed]
- Mehta SR, Wood DA, Storey RF, et al. Complete Revascularization with Multivessel PCI for Myocardial Infarction. N Engl J Med 2019;381:1411-21. [Crossref] [PubMed]
- Pavasini R, Biscaglia S, Barbato E, et al. Complete revascularization reduces cardiovascular death in patients with ST-segment elevation myocardial infarction and multivessel disease: systematic review and meta-analysis of randomized clinical trials. Eur Heart J 2020;41:4103-10. [Crossref] [PubMed]
- Bainey KR, Engstrøm T, Smits PC, et al. Complete vs Culprit-Lesion-Only Revascularization for ST-Segment Elevation Myocardial Infarction: A Systematic Review and Meta-analysis. JAMA Cardiol 2020;5:881-8. [Crossref] [PubMed]
- Tonino PA, De Bruyne B, Pijls NH, et al. Fractional flow reserve versus angiography for guiding percutaneous coronary intervention. N Engl J Med 2009;360:213-24. [Crossref] [PubMed]
- Zimmermann FM, Ferrara A, Johnson NP, et al. Deferral vs. performance of percutaneous coronary intervention of functionally non-significant coronary stenosis: 15-year follow-up of the DEFER trial. Eur Heart J 2015;36:3182-8. [Crossref] [PubMed]
- Montone RA, Niccoli G, Crea F, et al. Management of non-culprit coronary plaques in patients with acute coronary syndrome. Eur Heart J 2020;41:3579-86. [Crossref] [PubMed]
- Le Bras A, Puymirat E, Rabetrano H, et al. Economic evaluation of fractional flow reserve-guided versus angiography-guided multivessel revascularisation in ST-segment elevation myocardial infarction patients in the FLOWER-MI randomised trial. EuroIntervention 2022;18:235-41. [Crossref] [PubMed]
- Zhang R, Dou K, Guan C, et al. Outcomes of quantitative flow ratio-based percutaneous coronary intervention in an all-comers study. EuroIntervention 2022;17:1240-51. [Crossref] [PubMed]
- Bär S, Kavaliauskaite R, Ueki Y, et al. Quantitative Flow Ratio to Predict Nontarget Vessel-Related Events at 5 Years in Patients With ST-Segment-Elevation Myocardial Infarction Undergoing Angiography-Guided Revascularization. J Am Heart Assoc 2021;10:e019052. [Crossref] [PubMed]
- Zhang J, Yao M, Jia X, et al. The efficacy and safety of quantitative flow ratio-guided complete revascularization in patients with ST-segment elevation myocardial infarction and multivessel disease: A pilot randomized controlled trial. Cardiol J 2021; Epub ahead of print. [Crossref] [PubMed]
- Kogame N, Takahashi K, Tomaniak M, et al. Clinical Implication of Quantitative Flow Ratio After Percutaneous Coronary Intervention for 3-Vessel Disease. JACC Cardiovasc Interv 2019;12:2064-75. [Crossref] [PubMed]
- Biscaglia S, Tebaldi M, Brugaletta S, et al. Prognostic Value of QFR Measured Immediately After Successful Stent Implantation: The International Multicenter Prospective HAWKEYE Study. JACC Cardiovasc Interv 2019;12:2079-88. [Crossref] [PubMed]
- Asano T, Katagiri Y, Chang CC, et al. Angiography-Derived Fractional Flow Reserve in the SYNTAX II Trial: Feasibility, Diagnostic Performance of Quantitative Flow Ratio, and Clinical Prognostic Value of Functional SYNTAX Score Derived From Quantitative Flow Ratio in Patients With 3-Vessel Disease. JACC Cardiovasc Interv 2019;12:259-70. [Crossref] [PubMed]
- Zhang R, Song C, Guan C, et al. Prognostic value of quantitative flow ratio based functional SYNTAX score in patients with left main or multivessel coronary artery sisease. Circ Cardiovasc Interv 2020;13:e009155. [Crossref] [PubMed]
- Tang J, Lai Y, Tu S, et al. Quantitative flow ratio guided residual functional SYNTAX score for risk assessment in patients with ST-segment elevation myocardial infarction undergoing percutaneous coronary intervention. EuroIntervention 2021;17:e287-93. [Crossref] [PubMed]
- Li SJ, Ge Z, Kan J, et al. Cutoff Value and Long-Term Prediction of Clinical Events by FFR Measured Immediately After Implantation of a Drug-Eluting Stent in Patients With Coronary Artery Disease: 1- to 3-Year Results From the DKCRUSH VII Registry Study. JACC Cardiovasc Interv 2017;10:986-95. [Crossref] [PubMed]
- Piroth Z, Toth GG, Tonino PAL, et al. Prognostic Value of Fractional Flow Reserve Measured Immediately After Drug-Eluting Stent Implantation. Circ Cardiovasc Interv 2017;10:e005233. [Crossref] [PubMed]
- Kasula S, Agarwal SK, Hacioglu Y, et al. Clinical and prognostic value of poststenting fractional flow reserve in acute coronary syndromes. Heart 2016;102:1988-94. [Crossref] [PubMed]
- Sianos G, Morel MA, Kappetein AP, et al. The SYNTAX Score: an angiographic tool grading the complexity of coronary artery disease. EuroIntervention 2005;1:219-27. [PubMed]
- Serruys PW, Chichareon P, Modolo R, et al. The SYNTAX score on its way out or … towards artificial intelligence: part I. EuroIntervention 2020;16:44-59. [Crossref] [PubMed]
- Nam CW, Mangiacapra F, Entjes R, et al. Functional SYNTAX score for risk assessment in multivessel coronary artery disease. J Am Coll Cardiol 2011;58:1211-8. [Crossref] [PubMed]
- Xu B, Tu S, Song L, et al. Angiographic quantitative flow ratio-guided coronary intervention (FAVOR III China): a multicentre, randomised, sham-controlled trial. Lancet 2021;398:2149-59. [Crossref] [PubMed]
- Ding D, Huang J, Westra J, et al. Immediate post-procedural functional assessment of percutaneous coronary intervention: current evidence and future directions. Eur Heart J 2021;42:2695-707. [Crossref] [PubMed]
- Nijjer SS, Sen S, Petraco R, et al. Pre-angioplasty instantaneous wave-free ratio pullback provides virtual intervention and predicts hemodynamic outcome for serial lesions and diffuse coronary artery disease. JACC Cardiovasc Interv 2014;7:1386-96. [Crossref] [PubMed]
- Kikuta Y, Cook CM, Sharp ASP, et al. Pre-Angioplasty Instantaneous Wave-Free Ratio Pullback Predicts Hemodynamic Outcome In Humans With Coronary Artery Disease: Primary Results of the International Multicenter iFR GRADIENT Registry. JACC Cardiovasc Interv 2018;11:757-67. [Crossref] [PubMed]
- Collet C, Sonck J, Vandeloo B, et al. Measurement of Hyperemic Pullback Pressure Gradients to Characterize Patterns of Coronary Atherosclerosis. J Am Coll Cardiol 2019;74:1772-84. [Crossref] [PubMed]
- Biscaglia S, Uretsky BF, Tebaldi M, et al. Angio-Based Fractional Flow Reserve, Functional Pattern of Coronary Artery Disease, and Prediction of Percutaneous Coronary Intervention Result: a Proof-of-Concept Study. Cardiovasc Drugs Ther 2022;36:645-53. [Crossref] [PubMed]
- Shin D, Dai N, Lee SH, et al. Physiological Distribution and Local Severity of Coronary Artery Disease and Outcomes After Percutaneous Coronary Intervention. JACC Cardiovasc Interv 2021;14:1771-85. [Crossref] [PubMed]
- Lindahl B, Baron T, Albertucci M, et al. Myocardial infarction with non-obstructive coronary artery disease. EuroIntervention 2021;17:e875-87. [Crossref] [PubMed]
- Fearon WF, Low AF, Yong AS, et al. Prognostic value of the Index of Microcirculatory Resistance measured after primary percutaneous coronary intervention. Circulation 2013;127:2436-41. [Crossref] [PubMed]
- De Maria GL, Scarsini R, Shanmuganathan M, et al. Angiography-derived index of microcirculatory resistance as a novel, pressure-wire-free tool to assess coronary microcirculation in ST elevation myocardial infarction. Int J Cardiovasc Imaging 2020;36:1395-406. [Crossref] [PubMed]
- Mejia-Renteria H, Lee JM, Choi KH, et al. Coronary microcirculation assessment using functional angiography: Development of a wire-free method applicable to conventional coronary angiograms. Catheter Cardiovasc Interv 2021;98:1027-37. [Crossref] [PubMed]
- Tebaldi M, Biscaglia S, Di Girolamo D, et al. Angio-Based Index of Microcirculatory Resistance for the Assessment of the Coronary Resistance: A Proof of Concept Study. J Interv Cardiol 2020;2020:8887369. [Crossref] [PubMed]
- Scarsini R, Shanmuganathan M, Kotronias RA, et al. Angiography-derived index of microcirculatory resistance (IMR(angio)) as a novel pressure-wire-free tool to assess coronary microvascular dysfunction in acute coronary syndromes and stable coronary artery disease. Int J Cardiovasc Imaging 2021;37:1801-13. [Crossref] [PubMed]
- Kotronias RA, Terentes-Printzios D, Shanmuganathan M, et al. Long-Term Clinical Outcomes in Patients With an Acute ST-Segment-Elevation Myocardial Infarction Stratified by Angiography-Derived Index of Microcirculatory Resistance. Front Cardiovasc Med 2021;8:717114. [Crossref] [PubMed]
- Choi KH, Dai N, Li Y, et al. Functional Coronary Angiography-Derived Index of Microcirculatory Resistance in Patients With ST-Segment Elevation Myocardial Infarction. JACC Cardiovasc Interv 2021;14:1670-84. [Crossref] [PubMed]
- Stone GW, Maehara A, Lansky AJ, et al. A prospective natural-history study of coronary atherosclerosis. N Engl J Med 2011;364:226-35. [Crossref] [PubMed]
- Erlinge D, Maehara A, Ben-Yehuda O, et al. Identification of vulnerable plaques and patients by intracoronary near-infrared spectroscopy and ultrasound (PROSPECT II): a prospective natural history study. Lancet 2021;397:985-95. [Crossref] [PubMed]
- Xing L, Higuma T, Wang Z, et al. Clinical Significance of Lipid-Rich Plaque Detected by Optical Coherence Tomography: A 4-Year Follow-Up Study. J Am Coll Cardiol 2017;69:2502-13. [Crossref] [PubMed]
- Usui E, Yonetsu T, Kanaji Y, et al. Optical Coherence Tomography-Defined Plaque Vulnerability in Relation to Functional Stenosis Severity and Microvascular Dysfunction. JACC Cardiovasc Interv 2018;11:2058-68. [Crossref] [PubMed]
- Ben-Dor I, Torguson R, Gaglia MA Jr, et al. Correlation between fractional flow reserve and intravascular ultrasound lumen area in intermediate coronary artery stenosis. EuroIntervention 2011;7:225-33. [Crossref] [PubMed]
- Kanno Y, Sugiyama T, Hoshino M, et al. Optical coherence tomography-defined plaque vulnerability in relation to functional stenosis severity stratified by fractional flow reserve and quantitative flow ratio. Catheter Cardiovasc Interv 2020;96:E238-47. [Crossref] [PubMed]
- Dan K, Garcia-Garcia HM, Yacob O, et al. Comparison of plaque distribution and wire-free functional assessment in patients with stable angina and non-ST elevation myocardial infarction: an optical coherence tomography and quantitative flow ratio study. Coron Artery Dis 2021;32:131-7. [Crossref] [PubMed]
- Zuo W, Sun R, Zhang X, et al. The Association Between Quantitative Flow Ratio and Intravascular Imaging-defined Vulnerable Plaque Characteristics in Patients With Stable Angina and Non-ST-segment Elevation Acute Coronary Syndrome. Front Cardiovasc Med 2021;8:690262. [Crossref] [PubMed]
- Milzi A, Dettori R, Burgmaier K, et al. Quantitative flow ratio is related to intraluminal coronary stenosis parameters as assessed with optical coherence tomography. J Clin Med 2021;10:1856. [Crossref] [PubMed]
- Yang S, Koo BK, Narula J. Interactions Between Morphological Plaque Characteristics and Coronary Physiology: From Pathophysiological Basis to Clinical Implications. JACC Cardiovasc Imaging 2022;15:1139-51. [Crossref] [PubMed]
- Zeng X, Holck EN, Westra J, et al. Impact of coronary plaque morphology on the precision of computational fractional flow reserve derived from optical coherence tomography imaging. Cardiovasc Diagn Ther 2022;12:155-65. [Crossref] [PubMed]
- Pinilla-Echeverri N, Mehta SR, Wang J, et al. Nonculprit Lesion Plaque Morphology in Patients With ST-Segment-Elevation Myocardial Infarction: Results From the COMPLETE Trial Optical Coherence Tomography Substudys. Circ Cardiovasc Interv 2020;13:e008768. [Crossref] [PubMed]
- Denormandie P, Simon T, Cayla G, et al. Compared Outcomes of ST-Segment-Elevation Myocardial Infarction Patients With Multivessel Disease Treated With Primary Percutaneous Coronary Intervention and Preserved Fractional Flow Reserve of Nonculprit Lesions Treated Conservatively and of Those With Low Fractional Flow Reserve Managed Invasively: Insights From the FLOWER-MI Trial. Circ Cardiovasc Interv 2021;14:e011314. [Crossref] [PubMed]
- Safi H, Bourantas CV, Ramasamy A, et al. Predictive value of the QFR in detecting vulnerable plaques in non-flow limiting lesions: a combined analysis of the PROSPECT and IBIS-4 study. Int J Cardiovasc Imaging 2020;36:993-1002. [Crossref] [PubMed]
- Yu W, Tanigaki T, Ding D, et al. Accuracy of Intravascular Ultrasound-Based Fractional Flow Reserve in Identifying Hemodynamic Significance of Coronary Stenosis. Circ Cardiovasc Interv 2021;14:e009840. [Crossref] [PubMed]
- Yu W, Huang J, Jia D, et al. Diagnostic accuracy of intracoronary optical coherence tomography-derived fractional flow reserve for assessment of coronary stenosis severity. EuroIntervention 2019;15:189-97. [Crossref] [PubMed]
- Huang J, Emori H, Ding D, et al. Diagnostic performance of intracoronary optical coherence tomography-based versus angiography-based fractional flow reserve for the evaluation of coronary lesions. EuroIntervention 2020;16:568-76. [Crossref] [PubMed]
- Hong H, Jia H, Zeng M, et al. Risk Stratification in Acute Coronary Syndrome by Comprehensive Morphofunctional Assessment With Optical Coherence Tomography. JACC Asia 2022;2:460-72. [Crossref] [PubMed]
- Ullrich H, Olschewski M, Belhadj KA, et al. Quantitative Flow Ratio or Angiography for the Assessment of Non-culprit Lesions in Acute Coronary Syndromes: Protocol of the Randomized Trial QUOMODO. Front Cardiovasc Med 2022;9:815434. [Crossref] [PubMed]