Pathology of coronary atherosclerosis and thrombosis
Introduction
Coronary heart disease is a leading cause of death in the Western world (1). The majority of the coronary heart disease is attributed to atherosclerosis where the development of thrombosis may cause fatal complications such as acute myocardial infarction and sudden cardiac death (2). Many researchers have attempted to find out novel biomarkers and obtain detailed plaque morphologies by imaging modalities to predict and prevent this critical complication. The process of atherosclerotic plaque progression is considered to be dynamic and complicated. An understanding of the histomorphological characteristics of coronary plaques is of particular importance for both basic and clinical researchers who investigate the pathogenesis of atherosclerotic coronary disease. In this review, we describe the histomorphological features of native coronary atherosclerosis and thrombosis based on the findings obtained from human autopsy cases including sudden coronary death victims.
Progression of coronary atherosclerosis
Coronary atherosclerosis begins with early intimal hyperplasia near branch points. These lesions can be observed from birth and may progress to pathologic intimal thickening (PIT). The infiltration of macrophages into the lipid pools of PIT is associated with the transition of lipid pools to the necrotic core to form fibroatheroma. The further expansion of the necrotic core along with the thinning of the fibrous cap lead to the development of thin-cap fibroatheroma (TCFA) which is considered to be the precursor lesion of plaque rupture. The process of plaque progression is generally accompanied by the progression of calcification, and the necrotic core and the surrounding tissue may be eventually calcified to form fibrocalcific plaques (Figure 1) (3).
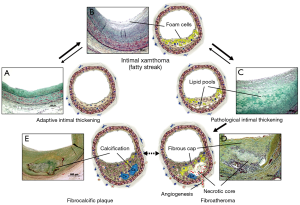
Intimal thickening and intimal xanthoma
Adaptive intimal thickening (AIT) and intimal xanthoma (or so called “fatty streaks”) are considered by some as the earliest manifestations of human atherosclerotic disease (Figure 1A,B) (4). These lesions may be precursors to symptomatic atherosclerotic disease, while others believe these to be non-atherosclerotic lesions secondary to blood flow (5). AIT occurs in children at similar locations as advanced plaques in adults, whereas intimal xanthomas may regress in later adult life (6,7). Histologically, AIT consists mainly of smooth muscle cells (SMCs) and proteoglycan matrix with little or no infiltrating inflammatory cells, while intimal xanthoma corresponds to the accumulation of predominantly macrophages within the intima (5).
PIT
PIT is considered by some to be the first of the progressive lesions, and is characterized by the presence of acellular lipid pools which is generally located close to the medial wall (Figure 1C) (4,5). The intima consists of SMCs in the extracellular matrix (ECM) and occasionally shows the presence of macrophages which are typically away from area of lipid pools. The presence of macrophages in PIT may indicate a more-advanced stage of atherogenesis as compared with the absence of macrophages (Figure 2) (8). The ECM primarily consists of hyaluronan and proteoglycan (biglycan, decorin, and versican), where the presence of proteoglycan is known to be associated with the retention of subendothelial lipid (9,10).
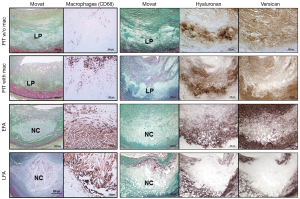
Fibroatheroma
The first of the advanced coronary lesions in concordance with the American Heart Association consensus classification is a fibroatheroma (5,11). This lesion is characterized by the presence of necrotic core which is generated by infiltration of macrophages into lipid pools and is encapsulated by surrounding fibrous tissue (Figure 1D). Macrophage apoptosis is considered to be of utmost importance in the necrotic core formation, while defective phagocytic clearance of apoptotic bodies may also play an important role in the development of the necrotic core (8,12).
Fibroatheroma can be further divided into early and late lesions. The early phase is characterized by the infiltration of macrophages into the lipid pool, together with the focal loss of proteoglycans and/or collagen (8). The accumulation of free cholesterol in early fibroatheromas is not extensive, while late fibroatheromas consist of discrete collections of cellular debris, increased free cholesterol, and near complete depletion of extracellular matrix (Figure 2) (8). It has been reported that the expression of hyaluronan and the proteoglycans (biglycan, decorin, and versican) is decreased in the necrotic core, probably due to active metalloproteinases (MMPs) and/or peptidases of the ADAMTS (A Disintegrin And Metalloproteinase with Thrombospondin Motifs) family (5,13).
TCFA (vulnerable plaque)
TCFA is essentially synonymous with vulnerable plaque and the concept of TCFA was developed from the observations of ruptured plaque (5,14). TCFA morphologically resembles plaque rupture but is different in terms of the absence of a luminal thrombus and a disrupted fibrous cap (Figure 3) (5,15). TCFAs generally contain a relatively large necrotic core with an overlying thin fibrous cap where varying degrees of macrophages and lymphocytes and paucity or absence of SMCs are observed (Figure 3A). The fibrous cap thickness of TCFA is pathologically defined as <65 µm, which is based on a finding from an autopsy study in sudden coronary death victims showing a mean cap thickness at the site of rupture of 23±19 µm, with 95% of the ruptured caps measuring <65 µm (14). TCFA is considered to be the precursor lesion of plaque rupture, where the thinning or weakening of the fibrous cap represents a common paradigm of disruption leading to plaque rupture (5,18).
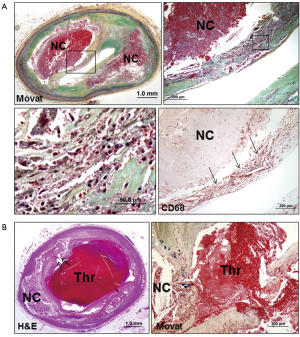
The prevalence of TCFAs in patients presenting with acute myocardial infarction has been reported to be greater in men with a mean of 3 per heart, as compared with women with half as many (19). The prevalence of TCFAs in sudden coronary death victims was also greater in men than women, although the difference was not statistically significant. TCFAs and plaque ruptures have shown a predilection for certain anatomic locations within the coronary trees. The majority of acute and healed ruptures and TCFAs are concentrated in the proximal and mid portion of the three major coronary arteries, and are rarely observed in the distal portion of these arteries (20). The most frequent location is the proximal portion of the left anterior descending coronary artery, with sites in the proximal left circumflex and mid to proximal right coronary arteries being half as common.
Coronary calcification and fibrocalcific plaque
Progression of coronary atherosclerosis is generally accompanied by an increase in calcification. PIT is known to be the earliest type of lesion exhibiting calcification. The intimal calcification may begin with SMC apoptosis and release of matrix vesicles in the lipid pools of PIT, which can be identified as “early microcalcification” (≥0.5 µm, and typically <15 µm) by von Kossa or Alizarin red staining (Figure 4A) (8,17,21-23). Microcalcification may also occur from macrophage apoptosis or matrix vesicles releasing from macrophages particularly in the necrotic core of fibroatheroma (17,24). Calcification from macrophage apoptosis likely appears as larger punctate and blocky appearance (“punctate microcalcification”, ≥15 µm) as compared with that from SMC apoptosis (Figure 4B) (17). A recent autopsy study involving 28 non-decalcified early coronary lesions demonstrated that 57% of PIT without macrophages exhibited “early microcalcification” but none of them had “punctate microcalcification”, whereas 44% of PIT with macrophages and 83% of early fibroatheromas showed “punctate microcalcification” (8).

Microcalcification may coalesce into larger masses and involve both the necrotic core and the surrounding extracellular matrix including collagen to form speckled or fragmented calcification. This pattern may also start in the deeper region of the necrotic core close to the media (Figure 4C). Further progression of calcification is usually observed as extension from the outer rim of the necrotic core into the surrounding collagenous matrix, and the central core may remain non-calcified or become fully calcified to form sheets or plates of calcification (Figure 4D). The confluent areas of calcification, i.e., fragmented or sheet calcification, are more frequently observed in late fibroatheroma as compared with early fibroatheroma, and are also the hallmark of stable or fibrocalcific plaques (Figure 1E). Calcified sheets may potentially fracture to form nodular calcification which is typically accompanied by fibrin deposition and often observed in heavily calcified arteries (Figure 4E).
Bone formation is rarely observed in calcified lesions (17). The prevalence of bone formation in peripheral arteries has been reported to be 19% in patients who underwent lower limb amputation (25), whereas that in coronary arteries has yet to be investigated to our knowledge, but is likely to be infrequent.
Coronary arterial remodeling
In the late 1980s, Glagov et al. elegantly showed that an increase in vessel size with increasing plaque burden allows the lumen to remain similar to normal adjacent artery segments as long as the plaque burden remains <40% (26). This phenomenon of vessel enlargement is called “arterial remodeling” (26,27). Postmortem and intravascular ultrasound studies have revealed that positive remodeling of the coronary arterial wall is associated with a greater lipid content and macrophage infiltration as well as the presence of unstable plaque features such as acute rupture, intraplaque hemorrhage, and TCFAs (28-30). Conversely, lesions of erosion, chronic total occlusion, and fibrocalcific plaques have been shown to exhibit negative remodeling (28). Motoyama et al. (31) in a computed tomographic (CT) angiography study showed that lesions with positive remodeling along with the low-attenuation plaque (lipidic plaques) were more likely to have future events at 1 to 2 years of follow-up than those without such features.
Coronary thrombosis
Pathologic studies in sudden coronary death victims have identified acute luminal thrombi in the majority (50–75%) of the cases whereas the remainder exhibit no thrombus but has severe atherosclerotic disease with >75% cross sectional luminal narrowing with or without a healed myocardial infarction (5). In case of acute coronary thrombosis, three distinct morphologic entities have been described as an etiology of thrombosis; plaque rupture, erosion, and calcified nodule (5). The frequency of the coronary lesions with thrombi has been reported to be 55–65% for ruptures, 30–35% for erosions, and 2–7% with calcified nodules in sudden coronary death victims (5,32).
Plaque rupture
Plaque rupture is characterized by the presence of a luminal thrombus overlying a thin disrupted fibrous cap which is often infiltrated by macrophages and T-lymphocytes, and there is an underlying large necrotic core (Figure 3B) (5,16,17). The disrupted fibrous cap mainly consists of type I collagen where SMCs are absent or sparse. It is generally a prevailing thought that rupture of the fibrous cap occurs at the weakest point, often near shoulder regions. However, previous autopsy studies demonstrated that plaque ruptures can occur at the mid-portion of the fibrous cap at the same frequency as shoulder regions in patients who died during exercise, whereas ruptures typically occur at shoulder regions when patients died at rest (33). Therefore, it is speculated that different processes may be involved in the final event of plaque rupture, where macrophages in the fibrous cap release matrix metalloproteinase (MMP) (34) to weaken the cap, and high shear stress may also contribute to the disruption of the cap (35). Microcalcification within a thin fibrous cap (typically 10 µm in diameter) may facilitate plaque rupture through local increase in stress that leads to interfacial debonding (17,22); however, it has been also reported that microcalcification <5 µm in diameter is seemingly less harmful while that >5 µm in diameter may predict plaque rupture (23).
Once the fibrous cap ruptures and the necrotic core contents are exposed to the flowing blood in the lumen, coagulation cascade is activated in response to the exposure of lipids and tissue factors (TF) which are present in the core. The luminal thrombus is rich in platelet (white thrombus) near the actual rupture site, while at sites proximal and distal to the rupture, there is propagation of the thrombus which is rich in erythrocytes that alternate with regions of fibrin and platelets (red thrombus). A study of aspirated thrombi from acute myocardial infarction patients examined by scanning electron microscopy confirmed a decrease in platelet content and an increase of fibrin and red cell content in accordance with ischemic time duration (36).
Plaque erosion
Plaque erosion is an acute thrombosis without rupture, where the luminal thrombus is in direct contact with the intima which is rich in SMCs in a proteoglycan matrix and there is an absence of endothelial lining (Figure 5) (15,37,38). The underlying plaque in erosions consists of PIT or fibroatheroma with a thick fibrous cap. The area in close proximity to the thrombus is rich in versican, hyaluronan and type III collagen unlike rupture or stable plaque which are rich in type I collagen and biglycan and decorin (37,38). The erosion lesions tend to be eccentric and are infrequently calcified with intact medial wall. Currently, no distinct morphological features suggestive of erosion-prone plaques have been identified.
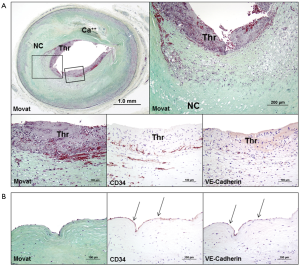
It is hypothesized that a selective accumulation of hyaluronan in eroded plaques may promote deendothelialization and platelet aggregation. Hyaluronan can directly promote the polymerization of fibrin, which may facilitate SMC migration and plaque progression. A previous autopsy study in sudden coronary death victims demonstrated that the majority of the eroded site shows minimal inflammation characterized by few or absent macrophages and lymphocytes (37), whereas another report showed that the site of erosion is always inflamed (39). It has been reported that eroded plaques have greater proportion of female, younger age, less % stenosis, less calcification, and less obstructive thrombus as compared with ruptured plaques (37). Plaque erosion accounts for over 80% of thrombi occurring in women <50 years of age.
In sudden coronary death victims, intramyocardial microemboli are more frequently observed in erosions (71%) as compared with plaque rupture (42%) (40). Moreover, autopsy studies showed that greater than 85% of coronary thrombi in erosions exhibited late stages of healing, whereas in ruptures only one-half of thrombi showed healing (41). Postmortem coronary thrombi superimposed on eroded plaques have been shown to contain a higher density of myeloperoxidase-positive cells than those superimposed on ruptured plaques (42). Also, circulation blood myeloperoxidase levels are elevated in patients with acute coronary syndromes attributed to plaque erosion as compared with those attributed to rupture, suggesting that elevations in selective inflammatory biomarkers may reflect specific acute coronary events.
Calcified nodule
Calcified nodules, the least frequent cause of coronary thrombi, is a lesion that occurs in heavily calcified arteries with areas of fragmented calcified sheets that form small amorphous nodules with surrounding fibrin and luminal thrombus. A recent autopsy study in 236 sudden coronary death victims with acute thrombi has reported that the prevalence of calcified nodules was 5% (32). Eruptive calcified nodules are usually observed in eccentric lesions where protrusion of the nodules into the lumen causes disruption of the overlying endothelium to form a platelet or fibrin-rich thrombus which is generally non-occlusive. Little is known about the precise mechanisms underlying the calcified nodule; however, one hypothesis is that mechanical stress on the heavily calcified coronary arteries might fragment sheets of calcium, resulting in small nodules surrounded by fibrin, which may eventually erupt through the plaque surface. Intraplaque fibrin is also observed in non-eruptive nodular calcification which does not have thrombus, potentially arising from surrounding leaky and damaged capillaries. Calcified nodules are more likely seen in older individuals and are preferentially found in the middle right coronary or left anterior descending coronary arteries that are tortuous.
Mechanisms of rapid lesion progression
The process of coronary plaque progression is generally characterized by the proliferation of SMCs and macrophages along with the accumulation of ECM and lipids. Nevertheless, rapid progression of coronary plaques is also likely attributed to other factors that include episodic thrombosis and intraplaque hemorrhage.
Healed plaque rupture
Silent luminal thrombi either from plaque rupture, erosion, or calcified nodule, are eventually repaired to form healed lesions, where the process contributes to a substantial increase in plaque burden and luminal narrowing (Figure 6). Healed plaque rupture are detected microscopically by the identification of breaks in the fibrous cap (type I collagen) with an overlying repair reaction consisting of SMCs in a proteoglycan-rich matrix and collagen type III (Figure 6A) (15,44). Similarly, in healed erosion, although there are no sites of rupture identified, there is a layered effect with type I collagen underlying the superficial region rich in proteoglycan and type III collagen with interspersed SMCs. Silent luminal thrombus is usually non-occlusive (Figure 6A), but in case of occlusive thrombosis, it results in the development of chronic total occlusion (Figure 6B) (43).
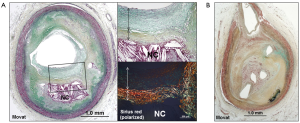
The prevalence of silent plaque rupture, erosion, or calcified nodule, in the clinical setting remains unknown. However, autopsy studies provide evidences of plaque progression from repeated thrombotic events (44,45). Histologic studies have shown that the frequency of healed plaque rupture correlates with the degree of luminal narrowing (45). Healed plaque rupture was identified in 16% of lesions with <20% diameter stenosis, 19% of lesions with 21% to 50% stenosis, and 73% of lesions with >50% stenosis (45). It has been also reported that 61% of hearts from sudden coronary death victims have healed plaque ruptures and the percentage of stenosis increases with the increasing number of healed plaque ruptures (44).
Intraplaque hemorrhage and expansion of the necrotic core
Advanced necrotic cores typically contain abundant free cholesterol which may be derived from apoptotic macrophages, but other sources should also contribute to the expansion of the necrotic core because cholesterol in macrophages is present in an esterified form (46). Considering that red blood cell membranes contain more free cholesterol than any other cells in the body (47), intraplaque hemorrhage is a logical origin of the free cholesterol seen in advanced necrotic cores. The assessment of prior hemorrhages by staining for glycophorin A, a specific marker for red blood cell membranes, as well as by iron staining for hemoglobin, demonstrated that intraplaque hemorrhage was more common in ruptured plaques, TCFAs, and fibroatheromas with late necrotic cores, as compared with early plaques in sudden coronary death victims (48). Moreover, the degree of glycophorin A immunostaining correlated with both the necrotic core size and the extent of macrophage infiltration (48). These observations indicate that collections of red blood cells may directly contribute to the necrotic core expansion, plaque enlargement, and lesion instability (Figure 3A,6A).
The origin of intraplaque hemorrhage is debated between those who believe that it is the influx of blood from the luminal side through plaque fissure or rupture, and others who propose that it is leakage from intraplaque vasa vasorum which extend into the intima from the adventitia. The latter hypothesis is supported by some reports that demonstrated dysmorphogenesis of the vasa vasorum with absence of mural cells and presence of poor endothelial cell junctions (49). It is possible that these immature or leaky vessels also allow diffusion of plasma molecules and diapedesis of leucocytes as well as erythrocytes (50), which may serve as the driving force of further centripetal angiogenesis from the adventitia (51).
Comparisons of morphologic features in advanced plaques
Previous autopsy studies have demonstrated the morphological comparisons between plaque ruptures, TCFAs, erosions, and stable plaques (i.e., severe coronary disease with >75% cross sectional area luminal narrowing without acute thrombi) in culprit lesions from sudden coronary death victims (Table 1) (52). Plaque rupture exhibited larger size of the necrotic core, greater number of cholesterol clefts within the necrotic core, greater infiltration of macrophages, and greater number of vasa vasorum and hemosiderin-laden macrophages, as compared with erosions or stable plaques. It has been also shown that plaque ruptures have relatively larger necrotic core, greater infiltration of macrophages within the fibrous cap, greater calcification and a greater accumulation of hemosiderin, as compared with TCFAs (20,52).
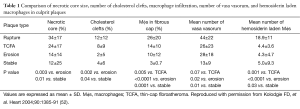
Full table
The extent of luminal narrowing varies in the plaque morphologies. TCFAs and fibroatheromas have been shown to exhibit the least luminal narrowing while lesions with plaque rupture, hemorrhage, or healed plaque rupture show the severest narrowing (52). The vast majority (approximately 80%) of TCFAs had <75% cross sectional area luminal narrowing (equivalent to <50% diameter stenosis), whereas 46% of healed plaque rupture and 43% of acute rupture showed the severe luminal narrowing (i.e., >75% cross-sectional area narrowing).
The mean necrotic core size in lesions from sudden coronary death victims was independent of luminal narrowing and was greatest in plaque ruptures, followed by TCFAs, erosion, and stable plaques (Table 1). In a detailed morphometric analysis of ruptured plaques, 80% of necrotic cores were larger than 1.0 mm2 and comprised >10% of plaque area in approximately 90% of the lesions. In 65% of plaque ruptures, the necrotic core occupied >25% of plaque area, whereas 75% of TCFAs had necrotic cores >10% of plaque area. The mean cross-sectional luminal narrowing in TCFAs was 71%, with necrotic core representing 10–25% of the lesion. On the other hand, the length of the necrotic core in ruptures and TCFAs was similar, varying from 2–22.5 mm, with a mean of 8 and 9 mm, respectively (53). In terms of the circumferential involvement of the necrotic core, 75% of TCFAs showed >120 degrees of the intima involved by a necrotic core (52).
Conclusions
The progression of early coronary plaques is characterized by the conversion of lipid pools in PIT into the necrotic core in fibroatheroma, which is accompanied by infiltration of macrophages and the focal loss of proteoglycans and collagen matrix. The expansion of the necrotic core is associated with the accumulation of free cholesterol which may be derived from intraplaque hemorrhage that can occur from leaky vasa vasorum within the intima or from luminal side through rupture or fissure. TCFA consists of a large necrotic core harbored by a thin (<65 µm) fibrous cap with abundant foamy macrophages, and is considered to be the prelude of plaque rupture. The majority of coronary thrombosis is attributed to plaque rupture, whereas other etiologies include plaque erosion and calcified nodule. Coronary thrombosis can occur asymptomatically to form healed lesions which contribute to an increase in plaque burden and luminal narrowing. The necrotic core and the surrounding tissue may be eventually calcified to develop calcified sheets that characterize stable and fibrocalcific plaques. We believe the description of histomorphological characteristics of coronary plaques in the current review would help understand the pathogenesis of atherosclerosis and thrombosis and facilitate the development of novel diagnostic tools and therapeutic strategies to eventually prevent fatal thrombotic complications.
Acknowledgements
Funding: Dr. Otsuka is supported by a Grant-in-Aid for Young Scientists (B) from the Japan Society for the Promotion of Science (15K19404).
Footnote
Conflicts of Interest: The authors have no conflict of interests to declare.
References
- Mozaffarian D, Benjamin EJ, Go AS, et al. Heart disease and stroke statistics--2015 update: a report from the American Heart Association. Circulation 2015;131:e29-322. [Crossref] [PubMed]
- Davies MJ, Thomas A. Thrombosis and acute coronary-artery lesions in sudden cardiac ischemic death. N Engl J Med 1984;310:1137-40. [Crossref] [PubMed]
- Bentzon JF, Otsuka F, Virmani R, et al. Mechanisms of plaque formation and rupture. Circ Res 2014;114:1852-66. [Crossref] [PubMed]
- Stary HC, Chandler AB, Glagov S, et al. A definition of initial, fatty streak, and intermediate lesions of atherosclerosis. A report from the committee on vascular lesions of the council on arteriosclerosis, american heart association. Circulation 1994;89:2462-78. [Crossref] [PubMed]
- Virmani R, Kolodgie FD, Burke AP, et al. Lessons from sudden coronary death: A comprehensive morphological classification scheme for atherosclerotic lesions. Arterioscler Thromb Vasc Biol 2000;20:1262-75. [Crossref] [PubMed]
- Velican C. A dissecting view on the role of the fatty streak in the pathogenesis of human atherosclerosis: Culprit or bystander? Med Interne 1981;19:321-37. [PubMed]
- Imakita M, Yutani C, Strong JP, et al. Second nation-wide study of atherosclerosis in infants, children and young adults in japan. Atherosclerosis 2001;155:487-97. [Crossref] [PubMed]
- Otsuka F, Kramer MC, Woudstra P, et al. Natural progression of atherosclerosis from pathologic intimal thickening to late fibroatheroma in human coronary arteries: A pathology study. Atherosclerosis 2015;241:772-82. [Crossref] [PubMed]
- Nakashima Y, Fujii H, Sumiyoshi S, et al. Early human atherosclerosis: Accumulation of lipid and proteoglycans in intimal thickenings followed by macrophage infiltration. Arterioscler Thromb Vasc Biol 2007;27:1159-65. [Crossref] [PubMed]
- Williams KJ. Interactions of lipoproteins with proteoglycans. Methods Mol Biol 2001;171:457-77. [PubMed]
- Stary HC, Chandler AB, Dinsmore RE, et al. A definition of advanced types of atherosclerotic lesions and a histological classification of atherosclerosis. A report from the committee on vascular lesions of the council on arteriosclerosis, american heart association. Circulation 1995;92:1355-74. [Crossref] [PubMed]
- Schrijvers DM, De Meyer GR, Herman AG, et al. Phagocytosis in atherosclerosis: Molecular mechanisms and implications for plaque progression and stability. Cardiovasc Res 2007;73:470-80. [Crossref] [PubMed]
- Stupka N, Kintakas C, White JD, et al. Versican processing by a disintegrin-like and metalloproteinase domain with thrombospondin-1 repeats proteinases-5 and -15 facilitates myoblast fusion. J Biol Chem 2013;288:1907-17. [Crossref] [PubMed]
- Burke AP, Farb A, Malcom GT, et al. Coronary risk factors and plaque morphology in men with coronary disease who died suddenly. N Engl J Med. 1997;336:1276-82. [Crossref] [PubMed]
- Otsuka F, Joner M, Prati F, et al. Clinical classification of plaque morphology in coronary disease. Nat Rev Cardiol 2014;11:379-89. [Crossref] [PubMed]
- Otsuka F, Finn AV, Virmani R. Do vulnerable and ruptured plaques hide in heavily calcified arteries? Atherosclerosis 2013;229:34-7. [Crossref] [PubMed]
- Otsuka F, Sakakura K, Yahagi K, et al. Has our understanding of calcification in human coronary atherosclerosis progressed? Arterioscler Thromb Vasc Biol 2014;34:724-36. [Crossref] [PubMed]
- Davies MJ, Thomas AC. Plaque fissuring--the cause of acute myocardial infarction, sudden ischaemic death, and crescendo angina. Br Heart J 1985;53:363-73. [Crossref] [PubMed]
- Burke AP, Virmani R, Galis Z, et al. 34th bethesda conference: Task force #2--what is the pathologic basis for new atherosclerosis imaging techniques? J Am Coll Cardiol 2003;41:1874-86. [Crossref] [PubMed]
- Kolodgie FD, Burke AP, Farb A, et al. The thin-cap fibroatheroma: A type of vulnerable plaque: The major precursor lesion to acute coronary syndromes. Curr Opin Cardiol 2001;16:285-92. [Crossref] [PubMed]
- Kockx MM, De Meyer GR, Muhring J, et al. Apoptosis and related proteins in different stages of human atherosclerotic plaques. Circulation 1998;97:2307-15. [Crossref] [PubMed]
- Vengrenyuk Y, Carlier S, Xanthos S, et al. A hypothesis for vulnerable plaque rupture due to stress-induced debonding around cellular microcalcifications in thin fibrous caps. Proc Natl Acad Sci U S A 2006;103:14678-83. [Crossref] [PubMed]
- Kelly-Arnold A, Maldonado N, Laudier D, et al. Revised microcalcification hypothesis for fibrous cap rupture in human coronary arteries. Proc Natl Acad Sci U S A 2013;110:10741-6. [Crossref] [PubMed]
- New SE, Goettsch C, Aikawa M, et al. Macrophage-derived matrix vesicles: An alternative novel mechanism for microcalcification in atherosclerotic plaques. Circ Res 2013;113:72-7. [Crossref] [PubMed]
- Soor GS, Vukin I, Leong SW, et al. Peripheral vascular disease: Who gets it and why? A histomorphological analysis of 261 arterial segments from 58 cases. Pathology 2008;40:385-91. [Crossref] [PubMed]
- Glagov S, Weisenberg E, Zarins CK, et al. Compensatory enlargement of human atherosclerotic coronary arteries. N Engl J Med 1987;316:1371-5. [Crossref] [PubMed]
- Clarkson TB, Prichard RW, Morgan TM, et al. Remodeling of coronary arteries in human and nonhuman primates. JAMA 1994;271:289-94. [Crossref] [PubMed]
- Burke AP, Kolodgie FD, Farb A, et al. Morphological predictors of arterial remodeling in coronary atherosclerosis. Circulation 2002;105:297-303. [Crossref] [PubMed]
- Varnava AM, Mills PG, Davies MJ. Relationship between coronary artery remodeling and plaque vulnerability. Circulation 2002;105:939-43. [Crossref] [PubMed]
- Schoenhagen P, Ziada KM, Kapadia SR, et al. Extent and direction of arterial remodeling in stable versus unstable coronary syndromes: An intravascular ultrasound study. Circulation 2000;101:598-603. [Crossref] [PubMed]
- Motoyama S, Sarai M, Harigaya H, et al. Computed tomographic angiography characteristics of atherosclerotic plaques subsequently resulting in acute coronary syndrome. J Am Coll Cardiol 2009;54:49-57. [Crossref] [PubMed]
- Yahagi K, Davis HR, Arbustini E, et al. Sex differences in coronary artery disease: Pathological observations. Atherosclerosis 2015;239:260-7. [Crossref] [PubMed]
- Burke AP, Farb A, Malcom GT, et al. Plaque rupture and sudden death related to exertion in men with coronary artery disease. JAMA 1999;281:921-6. [Crossref] [PubMed]
- Sukhova GK, Schonbeck U, Rabkin E, et al. Evidence for increased collagenolysis by interstitial collagenases-1 and -3 in vulnerable human atheromatous plaques. Circulation 1999;99:2503-9. [Crossref] [PubMed]
- Gijsen FJ, Wentzel JJ, Thury A, et al. Strain distribution over plaques in human coronary arteries relates to shear stress. Am J Physiol Heart Circ Physiol 2008;295:H1608-14. [Crossref] [PubMed]
- Silvain J, Collet JP, Nagaswami C, et al. Composition of coronary thrombus in acute myocardial infarction. J Am Coll Cardiol 2011;57:1359-67. [Crossref] [PubMed]
- Farb A, Burke AP, Tang AL, et al. Coronary plaque erosion without rupture into a lipid core. A frequent cause of coronary thrombosis in sudden coronary death. Circulation 1996;93:1354-63. [Crossref] [PubMed]
- Kolodgie FD, Burke AP, Farb A, et al. Differential accumulation of proteoglycans and hyaluronan in culprit lesions: Insights into plaque erosion. Arterioscler Thromb Vasc Biol 2002;22:1642-8. [Crossref] [PubMed]
- van der Wal AC, Becker AE, van der Loos CM, et al. Site of intimal rupture or erosion of thrombosed coronary atherosclerotic plaques is characterized by an inflammatory process irrespective of the dominant plaque morphology. Circulation 1994;89:36-44. [Crossref] [PubMed]
- Schwartz RS, Burke A, Farb A, et al. Microemboli and microvascular obstruction in acute coronary thrombosis and sudden coronary death: Relation to epicardial plaque histopathology. J Am Coll Cardiol 2009;54:2167-73. [Crossref] [PubMed]
- Kramer MC, Rittersma SZ, de Winter RJ, et al. Relationship of thrombus healing to underlying plaque morphology in sudden coronary death. J Am Coll Cardiol 2010;55:122-32. [Crossref] [PubMed]
- Ferrante G, Nakano M, Prati F, et al. High levels of systemic myeloperoxidase are associated with coronary plaque erosion in patients with acute coronary syndromes: A clinicopathological study. Circulation 2010;122:2505-13. [Crossref] [PubMed]
- Sakakura K, Nakano M, Otsuka F, et al. Comparison of pathology of chronic total occlusion with and without coronary artery bypass graft. Eur Heart J 2014;35:1683-93. [Crossref] [PubMed]
- Burke AP, Kolodgie FD, Farb A, et al. Healed plaque ruptures and sudden coronary death: Evidence that subclinical rupture has a role in plaque progression. Circulation 2001;103:934-40. [Crossref] [PubMed]
- Mann J, Davies MJ. Mechanisms of progression in native coronary artery disease: Role of healed plaque disruption. Heart 1999;82:265-8. [Crossref] [PubMed]
- Tabas I. Consequences of cellular cholesterol accumulation: Basic concepts and physiological implications. J Clin Invest 2002;110:905-11. [Crossref] [PubMed]
- Yeagle PL. Cholesterol and the cell membrane. Biochim Biophys Acta 1985;822:267-87. [Crossref] [PubMed]
- Kolodgie FD, Gold HK, Burke AP, et al. Intraplaque hemorrhage and progression of coronary atheroma. N Engl J Med 2003;349:2316-25. [Crossref] [PubMed]
- Sluimer JC, Kolodgie FD, Bijnens AP, et al. Thin-walled microvessels in human coronary atherosclerotic plaques show incomplete endothelial junctions relevance of compromised structural integrity for intraplaque microvascular leakage. J Am Coll Cardiol 2009;53:1517-27. [Crossref] [PubMed]
- Zhang Y, Cliff WJ, Schoefl GI, Higgins G. Plasma protein insudation as an index of early coronary atherogenesis. Am J Pathol 1993;143:496-506. [PubMed]
- Michel JB, Thaunat O, Houard X, Meilhac O, Caligiuri G, Nicoletti A. Topological determinants and consequences of adventitial responses to arterial wall injury. Arterioscler Thromb Vasc Biol. 2007;27:1259-68. [Crossref] [PubMed]
- Kolodgie FD, Virmani R, Burke AP, et al. Pathologic assessment of the vulnerable human coronary plaque. Heart. 2004;90:1385-91. [Crossref] [PubMed]
- Virmani R, Burke AP, Kolodgie FD, Farb A. Vulnerable plaque: The pathology of unstable coronary lesions. J Interv Cardiol. 2002;15:439-46. [Crossref] [PubMed]