FUNDC1: a key mediator of adenosine A2BR activation-induced inhibition of cardiac mitophagy under ischemia/reperfusion conditions
Highlight box
Key findings
• FUNDC1 is a key mediator of adenosine A2BR activation-induced inhibition of cardiac mitophagy under ischemia/reperfusion conditions.
What is known and what is new?
• Mitophagy is an essential factor in mitochondrial quality control against myocardial I/R injury.
• A2BR activation may decrease the binding of FUNDC1 to mitochondrial outer membrane by increasing FUNDC1 tyrosine phosphorylation via increasing Src tyrosine kinase activity.
What is the implication, and what should change now?
• It is helpful to fully understand the influence of adenosien receptor activation on mitochondrial structure and function which may promote the development of new methods against myocardial I/R injury.
Introduction
Background
Myocardial ischaemia/reperfusion (I/R) injury, which is caused by the massive production of reactive oxygen species (ROS), inflammatory cell infiltration, calcium overload and other factors, is a type of serious injury that occurs when the ischaemic myocardium is reperfused during the treatment of ischaemic heart disease (1). Improving the outcomes of ischaemia heart disease requires as little I/R injury as possible. Langendorff technology, which is relatively mature, and it is convenient to obtain the information about myocardial and coronary vessel status of a single heart, has been used to set myocardial I/R model for many years (2,3). Because mitochondria are the main source of adenosine triphosphate (ATP) and can trigger apoptosis or necrosis by generating ROS, the stability of mitochondrial morphology and function controls the life and death of the cell (4). ROS can open the mitochondrial permeability transition pore (mPTP) through various mechanisms, thereby causing the mitochondrial membrane to expand and rupture. Cytochrome C and other pro-apoptotic factors in mitochondria will enter the body, causing the death of cardiomyocytes (5). Therefore, it is important to inhibit the damage to the reperfused myocardium through preventing and reducing mitochondrial dysfunction.
Rationale and knowledge gap
The dysfunctional relationship between mitochondrial fission, mitochondrial fusion and mitophagy has been proven to be a key factor leading to myocardial I/R injury (6). Mitophagy, a kind of selective autophagy, exerts a key role in the quality control of mitochondria. Mitophagy not only selectively removes damaged mitochondria but also promotes the synthesis of new mitochondria, thereby ensuring mitochondrial quality (7). In 1987, adenosine was first reported to pronouncedly decrease infarct size and improve regional ventricular function in canine hearts under I/R conditions, and adenosine and its analogues have been considered to be the most promising drugs for treating myocardial I/R injury (8). Adenosine and its analogues induce a cardioprotective effect by activating different adenosine receptors (9). Studies on the effects of adenosine receptor activation on autophagy and mitophagy are insufficient, and the results are contradictory and complex (Table 1).
Table 1
AR subtypes | Model | The effect of AR activation on autophagy and mitophagy |
---|---|---|
AR (10) | Primary bovine PAECs | AR↑ |
Mitophagy↑ | ||
A1R (11) | HL-1/isolated adult rat cardiomyocytes simulated I/R | A1R↑ |
Autophagy↑ | ||
A1R (12) | Starvation model of human islets, human pancreatic neuroendocrine cell line BON-1 and in hepatocytes | Low A1R↑ |
Autophagy↑ | ||
Low A1R↑ | ||
Autophagy↓ | ||
A2R (13) | Isolated Wistar rat heart I/R model | A2R↑ |
Autophagy↓ | ||
A2AR (14) | Chondrocytes | A2AR↑ |
Autophagy↑ | ||
A2AR (15) | Neutrophils | A2AR↑ |
Autophagy↓ | ||
A2BR (16) | Mice AMI in vivo | A2BR↓ |
Autophagy↓ | ||
A3R (17) | Lung cancer cell lines (A549 and H1299) | A3R↑ |
Autophagy↑ | ||
A3A3R (18) | A375 melanoma cells | A3R↑ |
Autophagy↑ |
AR, adenosine receptor; PAEC, pulmonary artery endothelial cell; I/R, ischaemia/reperfusion; AMI, acute myocardial infarction.
Among the 4 well-known adenosine receptors, the adenosine 2B receptor (A2BR) subtype was proven to mediate the cardioprotective effects induced by both ischaemic preconditioning and postconditioning (19). Mitochondrial oxidative stress injury was alleviated by inhibiting mitochondrial complex I activity by activating Src tyrosine kinase via the activation of adenosine A2A receptor (A2AR) or A2BR (20). Meanwhile, Src tyrosine kinase regulates the activity of a variety of mitophagy-associated proteins (21). Although adenosine A2 receptor could decrease myocardial autophagy after reperfusion, the mechanism by which adenosine A2BR activates autophagy or mitophagy under I/R conditions is still unclear.
Objective
Our aim was to explore the effects of adenosine A2BR activation on cardiac autophagy and mitophagy under reperfusion conditions and the potential mechanism, to demonstrate that adenosine A2BR activation may inhibit myocardial autophagy and mitochondrial phagocytosis induced by I/R, and that Src tyrosine kinase may mediate adenosine A2BR activation to inhibit myocardial autophagy and mitosis induced by I/R by regulating FUNDC1. We present this article in accordance with the ARRIVE reporting checklist (available at https://cdt.amegroups.com/article/view/10.21037/cdt-22-468/rc).
Methods
Chemicals
BAY60-6583 (BAY, cat. No. 4472) and PP2 (cat. No. P0042) were purchased from Tocris Bioscience (Ellisville, MO, USA). Antibodies against LC3 (cat. No. 51520, RRID: AB_881429) and TOM20 (cat. No. 56783, RRID: AB_945896) were obtained from Abcam (San Francisco, CA, USA). The COX IV (cat. No. 4850S, RRID: AB_2085424) antibody was obtained from Cell Signing Technology (Beverly, MA, USA). Antibodies against Src (cat. No. 2109, AB_2106059) and FUNDC1 (cat. No. 251933) were purchased from ZEN BIO (Chengdu, China). β-Tubulin (cat. No. TDY043CTDY) was obtained from TDR Biotech (Qingdao, China). The fluorescent polymer microspheres were purchased from Duke Scientific Corp. (Fremont, CA, USA), and 2,3,5-triphenyltetrazolium chloride (TTC) was purchased from Sigma-Aldrich Chemical (St. Louis, MO, USA). MitoTracker Green (cat. No. C1048), LysoTracker Red (cat. No. C1046) and mCherry-GFP-LC3B (cat. No. C3011) were obtained from Beyotime Biotechnology (Shanghai, China).
Experimental animals
Adult male Wistar (7–10 w) weighing 250–350 g was purchased from the Laboratory Animal Research Center of North China University of Science and Technology. They were put into a cage (4 rats/cage) under 22±2 ℃ and 55%±5% humidity with a 12 h/12 h light/dark cycle. Supply standard laboratory food and drinking water. All of the experimental protocols were approved by the Ethics Committee of North China University of Science and Technology (application approval No. 2017023) and conducted under NIH Guidelines for the Care and Use of Laboratory Animals (NIH Publication No. 85-23, revised in 1996).
Regional I/R model of isolated rat hearts
The rat heart was operated according to the above method (22). Male Wistar rats (250–350 g) were anesthetized using sodium thiobarbital (100 mg/kg ip). Take out the heart quickly and wash it with cold Krebs-Henseleit buffer (pH 7.4), which contains 118.5 mM NaCl, 4.7 mM KCl, 1.2 mM MgSO4, 1.8 mM CaCl2, 24.8 mM NaHCO3, 1.2 mM KH2PO4, and 10 mM glucose. Then, after the aortic intubation, the heart was fixed on the Langendorff device, perfused with Krebs-Henseleit buffer, and inflated with 95% O2/5% CO2. The whole process took only 30 seconds. A latex balloon attached to a pressure sensor is inserted through the left atrium into the left ventricle. Using the physiological signal collection and processing system (Chengdu Instrument Manufactory, China) to continuously record various cardiac dynamic parameters including heart rate (HR) and left ventricular systolic pressure (LVSP). All hearts can be held steady for at least 20 min. After the blood pressure is stabilized, coronary flow (CF) is measured. Heart with a CF value of >28 or <10 mL/min will be excluded. Initially, left ventricular end-diastolic pressure (LVEDP) was recorded between 5 and 10 mmHg. A 5-0 suture is wound around the left coronary artery, with one end of the suture threaded through a small piece of soft vinyl tube to form a sleeve. The sleeve is pulled and fixed to the catheter with a small hemostat, causing ischemia (20). In the I/R group, the isolated myocardium was subjected to ischemia for 30 min, and then reperfused for 1 h. In the sham operation group, a silk thread was implanted, but there was no suture. BAY (1–1,000 nM) was injected for 65 min within 5 min of reperfusion. The Src tyrosine inhibitor PP2 (1 µM) was added for 70 min before reperfusion for 10 min.
Measurement of infarct size
The area at risk (AAR) and infarct area were assessed through fluorescent polymer microspheres and TTC staining as described previously (23). In order to achieve randomness, the reagents were prepared by another person and named by number every day. The person who did the animal experiments did not know what reagents were given. The number of groups has been added to each presentation of figures. In addition, 4 hearts were excluded from the study because of CF <10 mL/min, while 2 hearts were excluded from the study because of CF values >28 or 28 mL/min. Briefly, the coronary artery was reoccluded, and fluorescent polymer microspheres were infused. Then, the hearts were weighed, stored at −20 ℃ for 2 h, frozen and cut into 1 mm slices. Immerse the section in 10 mL of 10% formalin to increase its contrast, and squeeze it into the middle of a glass plate of only 1 mm. The myocardial ischemia and myocardial ischemia were qualitatively analyzed by using transparent acetate section. Background subtraction, brightness and contrast enhancement are performed on each image to improve sharpness and sharpness. The area was converted to volume by multiplying the section thickness, and the infarct size was expressed as a percentage (%) of AAR.
Western blotting
Apical heart tissues were prepared as protein lysates. The protein content on each swimming lane of Western Blot is 40 µg/mL, and the polyvinylidene fluoride (PVDF) membrane of 0.45 µm is used for all blocking membranes. All membranes were incubated overnight with primary antibodies (LC3, COX IV, TOM20, or FUNDC1) at 4 ℃, and each band was tested for the second antibody in an hour at room temperature. All thin films were visualized using enhanced chemiluminescence (ECL) method. The membrane was prepared again with anti-Tubulin antibody, and the equal load of the sample was determined. Use ImageJ to record the pictures in the computer and quantify them. All our Western Blot results are repeated 6 times.
Cell culture
H9c2 myocytes were obtained from the American Standard Culture Collection (ATCC, Manassas, VA, USA). Cells were cultured at 37 ℃ in 5% CO2/95% O2 air in Dulbecco’s Modified Eagle Medium (DMEM, Gibco, Carlsbad, CA, USA) containing 10% fetal bovine serum (FBS) (Gibco) and 100 U penicillin/streptomycin (24).
Hypoxia/reoxygenation (H/R) model in H9c2 cells
H9c2 cells were placed in a glucose-free Tyrode solution in a simulated ischemic environment (10 mM 2-deoxy-D-glucose and 10 mM sodium dithionite) for 30 min, followed by treatment with normal Tyrode reperfusion for 20 min (22). BAY treatment was performed within 5 min before reoxygenation. The selected Src tyrosine inhibitor PP2 (1 µM) was added 10 min before reoxygenation.
Immunofluorescence analysis
Cells were plated in a specific culture dish (50,000 cells/dish) for 36 h. After being treated according to the experimental protocol in 2.7, the cells were fixed in 4% formaldehyde for 1 h at room temperature. The cells were cleaned with phosphate buffered saline (PBS) three times. After the first cleaning, the cells were infiltrated with 0.1% TritonX-100 at room temperature for 15 min. The second time, the cells were closed with 5% BSA at room temperature for 1 h. Then the cells were rinsed with PBS 5 times, FITC or Cy3 secondary antibody was added, and incubated at 37 ℃ for 1 h. The confocal microscope was used for laser scanning. FITC fluorescence was excited by 488 nm helium-neon laser and imaged by 520 nm long path filter. The fluorescence of Cy3 was excited by a 543 nm helium-neon laser, and Cy3 was imaged by a 570 nm long path filter.
Colocalization of lysosomes and mitochondria in H9c2 cells
Cells were plated in specific culture dishes (50,000 cells/dish) for 36 h. The cells were washed 3 times using PBS and then treated with DMEM containing LysoTracker Red (1:20,000) and MitoTracker Green (1:10,000) for 30 min. After being incubated with fluorescence probes, the cells were treated as follows. After being washed with PBS, culture dishes were placed on the objective table and imaged with a laser scanning confocal microscope. The green fluorescence of MitoTracker is excited by a 488 nm He-Ne laser and processed by a 516 nm long path filter. LysoTracker Red light was studied with 543 nm He-Ne laser, and the image was processed with 590 nm long path filter.
Autophagy flow assay
Cells were seeded in particular culture dishes (50,00 cells/dish) for 24 h. After culturing to a certain density, Ad-mCherry-GFP-LC3B was included, reaching an ultimate concentration of 40 vp/mL. After 24 h of excitation, mCherry and GFP fluorescent spots were analyzed by confocal microscopy. A 488 nm He-Ne laser was used to excite GFP fluorescence, and a 516 nm long optical path filter was used for image processing. Under the He-Ne laser at 543 nm, mCherry fluorescence is excited, and then the image is processed with a 590 nm optical path filter (25).
Protein‒protein interaction (PPI) network construction
We used online databases (STRING, https://string-db.org/; INBIO, https://inbio-discover.com/) to construct the PPI network.
Mitochondrial isolation
According to the instructions of the manufacturer, mitochondria andcytoplasm were separated by standard homogenization, protease digestion, and differential centrifugation and so on, using tissue mitochondrial separation reagent (Beyotime Institute of Biotechnology, Shanghai, China). Simply put, the weighed heart sample was placed into a frozen PBS (v/v, 1/10) and manually cut using small scissors. The sliced tissue was separated from PBS at a speed of 600 rpm, and then cultured on ice using trypsin digest (v/v, 1/8) for 20 min. The digestion solution of trypsin was discarded and homogenized with a mixture of protease inhibitors in the mitochondrial separation buffer (solution A). Centrifuge the supernatant at 3,000 ×g for 10 min. The isolated mitochondrial particles were then suspended to the storage buffer (40 µL/100 mg tissue). Each process is carried out at 4 ℃.
Co-immunoprecipitation (CoIP)
Src tyrosine kinase antibody was cross-linked to magnetic beads according to Pierce Crosslink Magnetic IP/CoIP Kit (88805; Thermo Scientific, Rockford, IL, USA). One milligram mitochondrial protein was cross-linked with Src antibody with 1 mg Src antibody and incubated overnight at 4 ℃. The protein-Src antibody-bead complexes were washed with IP-lysis/wash buffer twice and then ultrapure water before eluting the bound antigen. The eluate was then immunoblotted.
Statistical analysis
Statistical analysis was conducted using the SigmaPlot 12.5 version software (Systat Software, Inc., San Jose, CA, U.S.A.). The data are expressed as the mean ± SEM and were obtained from 3 to 6 separate experiments. A comparison of two groups was assessed by Student’s t-test. One-way ANOVA followed by Tukey’s test was recruited to determine differences between more than two groups. A value of P<0.05 was considered statistically significant. A protocol was prepared before the study without registration.
Results
Adenosine A2BR activation inhibits I/R-induced myocardial autophagy and mitophagy
As shown in Table 2, no obvious differences were found in baseline cardiodynamic variables between the sham and I/R groups, CF and LVSP were decreased, while LVEDP was increased in the I/R group (Table 2). Moreover, infarct size was pronouncedly boosted in the I/R group (Figure 1A-1C). These results showed that our regional I/R model of isolated rat hearts was successfully established.
Table 2
Groups | CF | HR | LVSP | LVEDP | |||||||
---|---|---|---|---|---|---|---|---|---|---|---|
Baseline | End | Baseline | End | Baseline | End | Baseline | End | ||||
Sham | 15.50±1.08 | 13.13±0.75 | 205.00±2.00 | 200.00±10.00 | 109.85±4.56 | 93.53±4.30 | 6.54±1.77 | 8.72±0.33 | |||
I/R | 15.38±0.75* | 11.13±1.11* | 201.00±5.00 | 188.00±6.00 | 109.23±7.16* | 84.05±4.21* | 5.82±0.78* | 18.86±0.58* |
Values are means ± SEM of 4 experimental observations. *, P<0.05 vs. sham. CF, coronary flow; HR, heart rate; LVSP, left ventricular systolic pressure; LVEDP, left ventricular end-diastolic pressure; I/R, ischaemia/reperfusion; SEM, standard error of the mean.
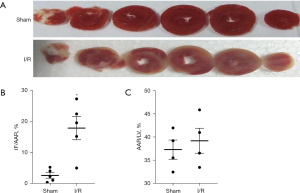
To determine the effects of adenosine A2BR activation on myocardial autophagy and mitophagy under I/R conditions, we measured the levels of autophagy and mitophagy marker protein expression. Western blotting showed that the ratio of LC3 II/β-Tubulin of I/R group (−0.39, 95% CI: −0.53 to −0.26; P=0.026), which is a major indicator of autophagy, was significantly increased, while the changes in mitophagy marker proteins (COX IV and TOM20) were not significant compared to those in the sham group (Figure 2A-2D). However, the selective adenosine A2BR agonist BAY reduced the ratio of LC3 II/β-Tubulin and increased COX IV and TOM20 expression in a dose-dependent manner (0.43, 95% CI: 0.15 to 0.71; P=0.011; −0.53, 95% CI: −0.74 to −0.31; P=0.009; 0.34, 95% CI: −0.05 to 0.73; P=0.032) (Figure 2A-2D). Moreover, confocal microscopy showed that the colocalization of TOM20 and LC3 was inhibited by BAY under H/R conditions (0.34, 95% CI: −0.05 to 0.73; 982.00, 95% CI: −156.05 to 2,120.05; P=0.004; 25.66, 95% CI: 17.80 to 35.52; P=0.004) (Figure 3A,3B). In addition, we found that after BAY was given under H/R conditions, it also inhibited co-localization of mitochondria and lysosomes (−46.79, 95% CI: −93.89 to 18.32; P=0.032) (Figure 3C,3D). The results showed that after BAY treatment, the number of yellow fluorescent dots and red fluorescent dots increased significantly, indicating that the number of autophagosomes and autophagolysosomes increased (25.50, 95% CI: 19.64 to 30.36; P<0.001; 25.50, 95% CI: 14.75 to 36.25; P<0.001) (Figure 4A,4B).
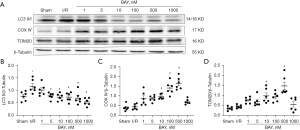
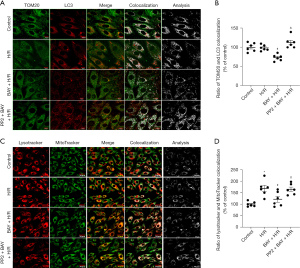
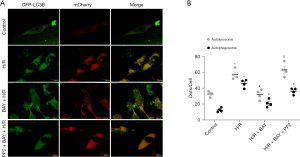
Src tyrosine kinase participates in the effects of adenosine A2BR activation against myocardial autophagy and mitophagy under I/R conditions
As shown in Figure 3A, the selective Src tyrosine kinase inhibitor PP2 inhibited the effect of BAY on TOM20 and LC3 colocalization. Moreover, Western blotting showed that the effects of A2BR activation on autophagy and mitophagy marker proteins, including LC3-II/β-Tubulin, COX IV and TOM20, were also inhibited by PP2 (0.04, 95% CI: −0.08 to 0.16; P=0.976) (Figure 5A-5D). In addition, comparing to the BAY group, the number of yellow fluorescent dots and red fluorescent dots reduced by PP2 treatment (Figure 4A,4B). These data demonstrated that adenosine A2BR activation could inhibit myocardial autophagy and mitophagy by activating Src tyrosine kinase under I/R conditions.
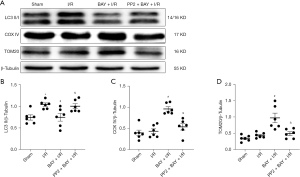
Adenosine A2BR activation inhibits myocardial autophagy and mitophagy under I/R conditions by regulating FUNDC1
The immunofluorescence results demonstrated that compared to that in the H/R group, the expression of mitochondrial FUNDC1 was reduced by BAY, but this effect was reversed by PP2 (Figure 6A,6B). To identify mitophagy-associated proteins that may play an important role in adenosine A2BR-induced mitophagy inhibition in I/R conditions, we used online databases (STRING, https://string-db.org/; INBIO, https://inbio-discover.com/) to analyse the interaction between Src tyrosine kinase and different mitophagy receptors and found that there was a strong correlation between Src tyrosine kinase and FUNDC1 (Figure 7A,7B). To further confirm the relationship between Src tyrosine kinase and FUNDC1, CoIP was performed. The results showed that mitochondrial FUNDC1 co-precipitated with Src tyrosine kinase after BAY was added (Figure 7C). Western blotting demonstrated that mitochondrial FUNDC1 expression was significantly decreased by BAY, and this effect was inhibited by PP2 (−0.07, 95% CI: −0.37 to −0.22; P=1.000) (Figure 7D). These results suggested that adenosine A2BR activation may inhibit myocardial autophagy and mitophagy by regulating mitochondrial FUNDC1 by activating Src tyrosine kinase.
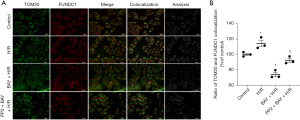
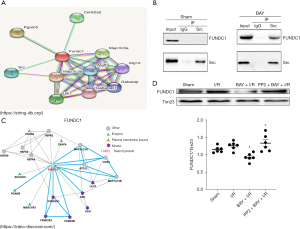
Discussion
Key findings
In the present study, adenosine A2BR activation may inhibit myocardial mitophagy by downregulating expression of the mitochondrial FUNDC1 by activating Src tyrosine kinase under reperfusion conditions and could increase the interaction between Src tyrosine kinase and FUNDC1.
Explanations of findings
The mitochondrion, which is called the “power factory” of the cell, is an important and unique organelle in eukaryotes. The main function of mitochondria is to synthesize ATP through the tricarboxylic acid cycle and oxidative phosphorylation. Therefore, mitochondria are the centre of energy conversion and output via the metabolic process in most cells. In addition, mitochondria also exert a critical role in the generation of ROS, fatty acid metabolism, calcium balance, and cell signal transduction (26). Mitochondrial dysfunction involves many cell activities, such as apoptosis, aging and disease. Mitochondrial damage is a key factor in cardiac ischemia reperfusion injury (27). It is widely accepted that autophagy is a double-edged sword in myocardial I/R injury (28). Increasing mitophagy can alleviate cardiac function injury and ventricular remodelling after coronary ligation, while decreasing mitophagy can exacerbate injury (29).
Adenosine A2BR is a kind of G-protein coupled receptor (GPCR) that is 332 amino acids in length with a molecular weight of approximately 36 kDa (30). In this study, because 10 nM BAY could induce stable and significant inhibitory effects on myocardial autophagy and mitophagy, this do was used in further experiments. Until recently, adenosine A2BR remained poorly characterized due to its low affinity for endogenous ligands. Several studies demonstrated that A2BR activation may reduce inflammation and promote tissue restitution by regulating immune cells alternative macrophage activation (31-33). According to previous studies, adenosine stimulates IL-10 through A2BR. In addition, adenosine receptor activation plays a role by interfering with the translation inhibition of IL-10 3'-UTR (34). In the study of sepsis, there are data proving that A2BR can prevent sepsis-induced death by inhibiting excessive inflammation (35). Not only that, but also study have pointed out that adenosine activation can enhance IL-10-mediated STAT3 signal transduction and M2c macrophage activation (36). A previous study showed that A2BR activation may induce strong protection against I/R injury by reducing oxidative stress by targeting mitochondria (20,37). Therefore, adenosine A2BR is an essential target in myocardial I/R injury (38). According to our research, Adenosine A2BR activation market is affected by myco-cardial autophagy and mitophagy under I/R conditions The mCherry-GFP-LC3B expression vector, A tandem fluorescent LC3, was used to further monitor the autophagy flow. Our study demonstrated that adenosine A2BR activation could reduce myocardial I/R injury by inhibiting mitochondrial complex I by activating Src tyrosine kinase (20). However, we showed that adenosine A2BR activation markedly inhibited myocardial autophagy and mitophagy, which may attenuate the protective effect of adenosine A2BR in I/R conditions.
Comparison with similar researches
Mitophagy-associated proteins are key factors in initiating mitophagy and determining selectivity, and Src tyrosine kinase regulates the activity of a variety of mitophagy-associated proteins by promoting tyrosine phosphorylation (21). This selectivity is achieved by identifying the LC3-interacting region (LIR). We demonstrated that adenosine A2BR activation could inhibit myocardial autophagy and mitophagy by activating Src tyrosine kinase under I/R conditions. In our study, the adenosine A2B receptor is activated through the selective adenosine A2B receptor agonist BAY60-6583, while in the literature, the adenosine A2B receptor is activated and suppressed through adenovirus injection, and the different activation methods may affect the final experimental results. Moreover, The animal models and animal species used in the research in the literature are different from our research (16). We use adult male Wistar rats to simulate the ischemia reperfusion model. In the article, C57 mice are used to establish the myocardial infarction model. Different models and animal strains have a significant impact on the experimental results. In the future, we can further use transgenic animals for verification.
Strengths and limitations
To date, FUNDC1, which is composed of 155 amino acids, is involved in mitophagy induced by hypoxia. FUNDC1 is a transmembrane protein that is located in the outer mitochondrial membrane and contains a typical sequence that interacts with the LC3 protein family. When tyrosine 18 and serine 13 of FUNDC1 are phosphorylated by Src tyrosine kinase or the protein kinase CK2, their activity is inhibited. When these sites are dephosphorylated, FUNDC1 is activated and binds to the LC3 protein family (39). Besides these phosphorylation sites, the effects of other FUNDC1 tyrosine sites are still not clear. In this study, only FUNDC1 has been discussed, but the Src tyrosine kinase regulates the content of FUNDC1 and thus affects autophagy. How to regulate FUNDC1 on the mitochondrial membrane and whether FUNDC1 has the binding site of the Src tyrosine kinase still need further study. In the current study, adenosine A2BR activation may inhibit myocardial autophagy and mitophagy by downregulating mitochondrial FUNDC1 by activating Src in I/R conditions. In this study, we found that adenosine A2BR may be activated by activating Src tyrosine kinase and decreasing FUNDC1 expression level, thus suppressing the autophagy of fungal mitochondria in I/R model. It betters the study on occurrence and regulation mechanism of mitochondrial autophagy, which is helpful to future targeted treatment of adenosine on mitochondrial damage through biochemical and pharmacological methods.
Implications and actions needed
We propose that the tyrosine phosphorylation of FUNDC1 may alter its spatial conformation and thus affect its binding to mitochondria. Moreover, the interaction between Src tyrosine kinase and FUNDC1 could be strengthened by adenosine A2BR activation. All the data show that A2BR activation may decrease the binding of FUNDC1 to mitochondrial outer membrane by increasing FUNDC1 tyrosine phosphorylation via increasing Src tyrosine kinase activity.
Conclusions
Adenosine A2BR activation may inhibit myocardial mitophagy by downregulating the mitochondrial FUNDC1 by activating Src tyrosine kinase under reperfusion conditions and could increase the interaction between Src tyrosine kinase and FUNDC1.
Acknowledgments
We thank Springer Nature Author Services for its linguistic assistance during the preparation of this manuscript.
Funding: This work was supported by grant 81700324 from the National Natural Science Foundation of China, grant 226Z7711G from the Hebei Provincial Special Fund for Central Guiding Local Science and Technology Development Plan and grant C20220354 from Hebei Provincial Financial Assistance for the Introduction of Overseas Researchers, and grant X2019173 from the NCST University Student Innovation Project.
Footnote
Reporting Checklist: The authors have completed the ARRIVE reporting checklist. Available at https://cdt.amegroups.com/article/view/10.21037/cdt-22-468/rc
Data Sharing Statement: Available at https://cdt.amegroups.com/article/view/10.21037/cdt-22-468/dss
Conflicts of Interest: All authors have completed the ICMJE uniform disclosure form (available at https://cdt.amegroups.com/article/view/10.21037/cdt-22-468/coif). All authors report that this work was supported by grant 81700324 from the National Natural Science Foundation of China, grant 226Z7711G from the Hebei Provincial Special Fund for Central Guiding Local Science and Technology Development Plan and grant C20220354 from Hebei Provincial Financial Assistance for the Introduction of Overseas Researchers, and grant X2019173 from the NCST University Student Innovation Project. The authors have no other conflicts of interest to declare.
Ethical Statement: The authors are accountable for all aspects of the work in ensuring that questions related to the accuracy or integrity of any part of the work are appropriately investigated and resolved. All of the experimental protocols were approved by the Ethics Committee of North China University of Science and Technology (application approval No. 2017023) and conducted under NIH Guidelines for the Care and Use of Laboratory Animals (NIH Publication No. 85-23, revised in 1996).
Open Access Statement: This is an Open Access article distributed in accordance with the Creative Commons Attribution-NonCommercial-NoDerivs 4.0 International License (CC BY-NC-ND 4.0), which permits the non-commercial replication and distribution of the article with the strict proviso that no changes or edits are made and the original work is properly cited (including links to both the formal publication through the relevant DOI and the license). See: https://creativecommons.org/licenses/by-nc-nd/4.0/.
References
- Jun JH, Shim JK, Oh JE, et al. Protective Effect of Ethyl Pyruvate against Myocardial Ischemia Reperfusion Injury through Regulations of ROS-Related NLRP3 Inflammasome Activation. Oxid Med Cell Longev 2019;2019:4264580. [Crossref] [PubMed]
- Rossello X, Hall AR, Bell RM, et al. Characterization of the Langendorff Perfused Isolated Mouse Heart Model of Global Ischemia-Reperfusion Injury: Impact of Ischemia and Reperfusion Length on Infarct Size and LDH Release. J Cardiovasc Pharmacol Ther 2016;21:286-95. [Crossref] [PubMed]
- Döring HJ. The isolated perfused heart according to Langendorff technique--function--application. Physiol Bohemoslov 1990;39:481-504. [PubMed]
- Wang J, Toan S, Zhou H. Mitochondrial quality control in cardiac microvascular ischemia-reperfusion injury: New insights into the mechanisms and therapeutic potentials. Pharmacol Res 2020;156:104771. [Crossref] [PubMed]
- Bugger H, Pfeil K. Mitochondrial ROS in myocardial ischemia reperfusion and remodeling. Biochim Biophys Acta Mol Basis Dis 2020;1866:165768. [Crossref] [PubMed]
- Mui D, Zhang Y. Mitochondrial scenario: roles of mitochondrial dynamics in acute myocardial ischemia/reperfusion injury. J Recept Signal Transduct Res 2021;41:1-5. [Crossref] [PubMed]
- Um JH, Yun J. Emerging role of mitophagy in human diseases and physiology. BMB Rep 2017;50:299-307. [Crossref] [PubMed]
- Olafsson B, Forman MB, Puett DW, et al. Reduction of reperfusion injury in the canine preparation by intracoronary adenosine: importance of the endothelium and the no-reflow phenomenon. Circulation 1987;76:1135-45. [Crossref] [PubMed]
- Ruan W, Ma X, Bang IH, et al. The Hypoxia-Adenosine Link during Myocardial Ischemia-Reperfusion Injury. Biomedicines 2022;10:1939. [Crossref] [PubMed]
- Ko J, Rounds S, Lu Q. Sustained adenosine exposure causes endothelial mitochondrial dysfunction via equilibrative nucleoside transporters. Pulm Circ 2020;10:2045894020924994. [Crossref] [PubMed]
- Yitzhaki S, Huang C, Liu W, et al. Autophagy is required for preconditioning by the adenosine A1 receptor-selective agonist CCPA. Basic Res Cardiol 2009;104:157-67. [Crossref] [PubMed]
- Israeli T, Riahi Y, Saada A, et al. Opposing effects of intracellular versus extracellular adenine nucleotides on autophagy: implications for β-cell function. J Cell Sci 2018;131:jcs212969. [Crossref] [PubMed]
- Jin Y, Wang H, Cui X, et al. Role of autophagy in myocardial reperfusion injury. Front Biosci (Elite Ed) 2010;2:1147-53. [PubMed]
- Friedman B, Corciulo C, Castro CM, et al. Adenosine A2A receptor signaling promotes FoxO associated autophagy in chondrocytes. Sci Rep 2021;11:968. [Crossref] [PubMed]
- Liu YW, Yang T, Zhao L, et al. Activation of Adenosine 2A receptor inhibits neutrophil apoptosis in an autophagy-dependent manner in mice with systemic inflammatory response syndrome. Sci Rep 2016;6:33614. [Crossref] [PubMed]
- Zhan E, Cao W, Fan X, et al. Decreased expression of adenosine receptor 2B confers cardiac protection against ischemia via restoring autophagic flux. Am J Transl Res 2020;12:7995-8006. [PubMed]
- Lu YC, Lee YR, Liao JD, et al. Reversine Induced Multinucleated Cells, Cell Apoptosis and Autophagy in Human Non-Small Cell Lung Cancer Cells. PLoS One 2016;11:e0158587. [Crossref] [PubMed]
- Soares AS, Costa VM, Diniz C, et al. Combination of Cl-IB-MECA with paclitaxel is a highly effective cytotoxic therapy causing mTOR-dependent autophagy and mitotic catastrophe on human melanoma cells. J Cancer Res Clin Oncol 2014;140:921-35. [Crossref] [PubMed]
- Gile J, Eckle T. ADORA2b Signaling in Cardioprotection. J Nat Sci 2016;2:e222. [PubMed]
- Xu J, Bian X, Liu Y, et al. Adenosine A(2) receptor activation ameliorates mitochondrial oxidative stress upon reperfusion through the posttranslational modification of NDUFV2 subunit of complex I in the heart. Free Radic Biol Med 2017;106:208-18. [Crossref] [PubMed]
- Hamacher-Brady A, Brady NR. Mitophagy programs: mechanisms and physiological implications of mitochondrial targeting by autophagy. Cell Mol Life Sci 2016;73:775-95. [Crossref] [PubMed]
- Xu J, Bian X, Zhao H, et al. Morphine Prevents Ischemia/Reperfusion-Induced Myocardial Mitochondrial Damage by Activating δ-opioid Receptor/EGFR/ROS Pathway. Cardiovasc Drugs Ther 2022;36:841-57. [Crossref] [PubMed]
- Yang Y, Zhao L, Ma J. Penehyclidine hydrochloride preconditioning provides cardiac protection in a rat model of myocardial ischemia/reperfusion injury via the mechanism of mitochondrial dynamics mechanism. Eur J Pharmacol 2017;813:130-9. [Crossref] [PubMed]
- Gao G, Chen W, Yan M, et al. Rapamycin regulates the balance between cardiomyocyte apoptosis and autophagy in chronic heart failure by inhibiting mTOR signaling. Int J Mol Med 2020;45:195-209. [PubMed]
- Geng MY, Wang L, Song YY, et al. Sidt2 is a key protein in the autophagy-lysosomal degradation pathway and is essential for the maintenance of kidney structure and filtration function. Cell Death Dis 2021;13:7. [Crossref] [PubMed]
- Zong WX, Rabinowitz JD, White E. Mitochondria and Cancer. Mol Cell 2016;61:667-76. [Crossref] [PubMed]
- Chouchani ET, Pell VR, James AM, et al. A Unifying Mechanism for Mitochondrial Superoxide Production during Ischemia-Reperfusion Injury. Cell Metab 2016;23:254-63. [Crossref] [PubMed]
- Scherz-Shouval R, Shvets E, Fass E, et al. Reactive oxygen species are essential for autophagy and specifically regulate the activity of Atg4. EMBO J 2019;38:e101812. [Crossref] [PubMed]
- Saito T, Sadoshima J. Molecular mechanisms of mitochondrial autophagy/mitophagy in the heart. Circ Res 2015;116:1477-90. [Crossref] [PubMed]
- Stehle JH, Rivkees SA, Lee JJ, et al. Molecular cloning and expression of the cDNA for a novel A2-adenosine receptor subtype. Mol Endocrinol 1992;6:384-93. [PubMed]
- Csóka B, Selmeczy Z, Koscsó B, et al. Adenosine promotes alternative macrophage activation via A2A and A2B receptors. FASEB J 2012;26:376-86. [Crossref] [PubMed]
- Koscsó B, Csóka B, Selmeczy Z, et al. Adenosine augments IL-10 production by microglial cells through an A2B adenosine receptor-mediated process. J Immunol 2012;188:445-53. [Crossref] [PubMed]
- Kreft JU, Plugge CM, Grimm V, et al. Mighty small: Observing and modeling individual microbes becomes big science. Proc Natl Acad Sci U S A 2013;110:18027-8. [Crossref] [PubMed]
- Németh ZH, Lutz CS, Csóka B, et al. Adenosine augments IL-10 production by macrophages through an A2B receptor-mediated posttranscriptional mechanism. J Immunol 2005;175:8260-70. [Crossref] [PubMed]
- Csóka B, Németh ZH, Rosenberger P, et al. A2B adenosine receptors protect against sepsis-induced mortality by dampening excessive inflammation. J Immunol 2010;185:542-50. [Crossref] [PubMed]
- Koscsó B, Csóka B, Kókai E, et al. Adenosine augments IL-10-induced STAT3 signaling in M2c macrophages. J Leukoc Biol 2013;94:1309-15. [Crossref] [PubMed]
- Fredholm BB, Arslan G, Halldner L, et al. Structure and function of adenosine receptors and their genes. Naunyn Schmiedebergs Arch Pharmacol 2000;362:364-74. [Crossref] [PubMed]
- Vecchio EA, White PJ, May LT. The adenosine A(2B) G protein-coupled receptor: Recent advances and therapeutic implications. Pharmacol Ther 2019;198:20-33. [Crossref] [PubMed]
- Lampert MA, Orogo AM, Najor RH, et al. BNIP3L/NIX and FUNDC1-mediated mitophagy is required for mitochondrial network remodeling during cardiac progenitor cell differentiation. Autophagy 2019;15:1182-98. [Crossref] [PubMed]