Sfrp2 regulates the WNT/β-catenin pathway to slow the development of aldosterone-producing adenoma
Highlight box
Key findings
• In this study, we learned that Sfrp2 can inhibit aldosterone secretion by suppressing β-catenin expression.
What is known and what is new?
• Previous studies have found that aldosterone oversecretion is related to overexpression of CYP11B2.
• In this study, we altered the expression of Sfrp2 to explore the secretion of aldosterone.
What is the implication, and what should change now?
• The study indicating that both the Sfrp2 and WNT pathways can be used as therapeutic targets for inhibiting aldosterone secretion and for the treatment of other diseases caused by increased aldosterone concentration. However, the mechanism between Sfrp2 and β-catenin still needs to be further explored.
Introduction
Primary aldosteronism (PA) is a clinical syndrome mainly characterized by water and sodium retention, hypertension, or hypokalemia due to the excessive secretion of aldosteronism by the adrenal cortex (1,2). PA is one of the most common causes of secondary hypertension, and the proportion of PA in hypertensive patients is about 10%, which can reach about 20% in patients with refractory hypertension (3,4). Aldosterone-producing adenoma (APA) is the most common subtype of PA. It usually occurs in the glomerulosa zone of the adrenal cortex and is a benign tumor that produces and secretes aldosterone. APA often causes severe hypertension, and commonly used antihypertensive drugs have poor efficacy (5). The commonly used APA treatment is APA resection, and after unilateral APA resection, the blood pressure of patients decreased to normal within 1 year, and more than 70% of other major symptoms disappeared (6). Before APA resection, drug therapy is often used to assist patients in adjusting their physical state. Meanwhile, when some patients cannot receive surgical treatment, drug therapy is also commonly used to reduce blood pressure or supplement potassium, so as to reduce the harm of APA (7).
Molecular study on the production of aldosterone have found that somatic mutations can contribute to the development of APA, and aldosterone synthase encoded by CYP11B2 (cytochrome P450 11B2) gene is an essential enzyme in the synthesis of aldosterone and participates in the pathogenesis of APA (8). Studies have found that the oversecretion of aldosterone is related to the overexpression of CYP11B2, and various factors may affect the expression and function of CYP11B2 in APA (9,10). Therefore, from the molecular perspective, targeted regulation of CYP11B2 expression has a therapeutic effect in APA, and CYP11B2 is an important therapeutic target of APA (11).
The Wnt/β-catenin signaling pathway, as a stem cell regulator, exists in numerous tissues and organs of the human body (12). In addition, due to the abnormal activation of the Wnt pathway in several tumors, there are many studies on the Wnt pathway gene as a therapeutic target, and it has been applied in the treatment of various tumors (13,14). Some researchers have tested the adrenocortical adenoma tissues of APA patients, and the results showed that CYP11B2 and activated β-catenin were also highly expressed in areas where a large number of mast cells existed (15). Berthon et al. also showed that the WNT/β-catenin signaling pathway is abnormally activated in APA, and WNT pathway signaling may be related to APA development (16).
Secreted frizzled-related protein 2 (Sfrp2) blocks the activation of the WNT/β-catenin signaling pathway by competing with frizzled for WNT ligands. Therefore, Sfrp2 is also known as an antagonist of WNT (17). Study have shown that Sfrp2 can stimulate tumor angiogenesis and can be used as an effective target for anti-tumor angiogenesis therapy (18). Sfrp2 may also be involved in regulating physiological activities such as heart development and tumorigenesis by blocking the WNT pathway (19). Moreover, Sfrp2 participates in the physiological activities of tumor cells by regulating the activity of the WNT pathway. It has also been shown that low Sfrp2 expression can lead to abnormal development of APA, and Sfrp2 gene deletion can promote increased aldosterone secretion in mice (20).
The present study focused on the WNT pathway and explored the effect of Sfrp2 expression on the WNT/β-catenin signaling pathway and APA through in vitro cell experiments and the establishment of a mouse APA model. We present this article in accordance with the ARRIVE and MDAR reporting checklists (available at https://cdt.amegroups.com/article/view/10.21037/cdt-23-105/rc).
Methods
Cell culture
Tissue samples from 16 APA patients and 11 normal adrenal tissue samples (NA) were collected from The Second Affiliated Hospital of Anhui Medical University. Normal adrenal tissue samples were obtained from surgically removed tissue samples of patients with adrenal cysts, renal clear cell carcinoma, etc. Human adrenal cortical adenocarcinoma cells (NCI-H295R) were purchased from Ningbo Mingzhou Biotech Co., Ltd. (Zhejiang Province, China). All cells were cultured in dulbecco’s modified eagle medium/Ham’s F 12 nutrient medium (DMEM/F12 medium) containing 1% Insulin-Transferrin-Selenium (ITS), 1% penicillin/streptomycin, 0.01% gentamicin, and 10% fetal calf serum (FCS) at 37 ℃ and 5% carbon dioxide (CO2). Cells in the Wnt-inhibited group were treated with 10 µM WNT pathway inhibitor KYA1797K (Selleck, Shanghai) and cultured in an incubator. The study was conducted in accordance with the Declaration of Helsinki (as revised in 2013). Informed consent was taken from all the patients. The study was approved by the Ethics Committee of The Second Affiliated Hospital of Anhui Medical University (No. SL-YX 2023-SZR 090).
Reverse transcription-polymerase chain reaction (RT-PCR)
Patient tissue samples were collected and cells were lysed at room temperature with TRIzol reagent (Sigma-Aldrich, Shanghai). The extracted total RNA was reversely transcribed into complementary DNA (cDNA) using a reverse transcription kit (TAKARA, Beijing). PCR was performed on the extracted cDNA using a fluorescent quantitative PCR instrument (Jinan Olaibo Scientific Instrument Co., Ltd., Shandong, China). Using GAPDH as an internal reference, the 2-∆∆Ct method was employed for data collection and analysis. The reaction system was as follows: pre-denaturation at 95 ℃ for 2 min; 95 ℃ reaction 15 s, 60 ℃ reaction 30 s, 72 ℃ reaction 30 s, for a total of 35 cycles. The primer sequence of Sfrp2 was as follows: 5'-ATCGGTGCTGTGGCTCAA-3' (sense), 5'-CATCAGGATGCCGGGACT-3' (antisense). The primer sequence of wnt5a was as follows: 5'-TGCCACTTGTATCAGGACCA-3' (sense), 5'-CTGTGCTGCAGTTCCATCTC-3' (antisense). The primer sequence of GAPDH was as follows: 5'-GGGAAACTGTGGCGTGAT-3' (sense), 5'-AAAGGTGGAGGAGTGGGT-3' (antisense). The primer sequence of CYP11B2 was as follows: 5'-ACCATGGATGTCCAGCAA-3' (sense), 5'-GAGAGCTGCCGAGTCTGA-3' (antisense). The primer sequence of β-catenin was as follows: 5'-GATTACTGCTCTGGCTCCTAGC-3' (sense), 5'-GACTCATCGTACTCCTGCTTGC-3' (antisense).
Immunohistochemistry
The collected tissue samples were embedded in paraffin and then cut into thin slices and mounted on glass slides. The paraffin sections were dewaxed and then treated with 3% hydrogen peroxide for 15 min. Next, the sections were cleaned with a Phosphate Buffer Saline (PBS) solution and then treated with 10% goat serum and sealed at room temperature for 30 min. The paraffin sections were subsequently cleaned with a PBS solution, and a diluted primary antibody solution, such as rat anti-β-catenin antibody (1:200, FineTest®, Wuhan, China) or rabbit anti-CYP11B2 antibody (1:2,000, Abcam, Cambridge, UK), was added to the sections and incubated at 4 ℃ overnight.
On the second day, horse radish peroxidase (HRP)-labeled goat anti-rabbit immunoglobulin G (IgG) secondary antibody solution (1:1,000, Solarbio, Beijing) or goat anti-rat IgG secondary antibody solution (1:1,000, Amyjet Scientific, Wuhan) was added to the slides and incubated at 37 ℃ for 30 min. The paraffin sections then were cleaned, and HRP avidin was added to the sections for 3 h. Diaminobenzidine (DAB) solution was added to the slices for 15 min to develop color, and the slices were sealed with neutral gum. Next, tissue staining was observed with a microscope (Precise Instrument Co., Ltd., Beijing).
Western blot analysis
NCI-H295R cells and APA tissues were collected, lysed with lysate (containing protease inhibitors), and seeded in culture plates for cell culture. The proteins were then collected and separated by electrophoresis using sodium dodecyl sulfate polyacrylamide gel electrophoresis (SDS-PAGE), and then transferred to polyvinylidene fluoride (PVDF) membranes. After washing the membrane, 5% skim milk was added and it was sealed at room temperature for 1–2 h. The membrane was then placed in a primary antibody diluent and incubated overnight at 4 ℃. Next, the membranes were washed with a tris buffered saline tween (TBST) solution and added with HRP-labeled goat anti-rabbit IgG diluent (1:10,000, Solarbio) and incubated at room temperature for 2 h. After washing the membrane, the enhanced chemiluminescence (ECL) chemiluminescence method (Solarbio life science, Beijing) was utilized to detect the bands on the membrane. Image J software (National Institutes of Health, USA) was used to determine the absorbance value of the protein bands.
The primary antibodies used in the experiment included the following: rabbit anti-Sfrp2 antibody (1:500, Beyotime, Beijing), rabbit anti-Wnt5a (1:600, Beyotime, Shanghai), rabbit anti-LEF (1:1,000, Abcam), rabbit anti-TCF (1:1,000, Abcam), and rabbit anti-CYP11B2 (1:2,000, Abcam).
Cell transfection
First, Sfrp2-targeting RNA interference plasmid vectors (Sfrp2 siRNA) and Sfrp2-overexpressed lentivirus vectors (Sfrp2 mimics) were constructed, and blank vectors were used as a negative control. NCI-H295R cells were then collected and inoculated into 24-well plates at a density of 2×105 cells/well, and cultured until 95% cells grew adherent to the wall. 20 pmol of a gene carrier solution and 1 µL of Lipofectamine™ 2000 (Thermo Fisher Scientific, US) were respectively added to an Opti-MEM (Minimum Essential Medium) serum-free medium for dilution. The two diluents were then mixed together and let stand at room temperature for 5 min. When the cell density in the culture plate reached 90%, the culture medium in the culture plate was removed. An Opti-MEM serum-free medium containing transfection genes and transfection reagents was then added to the culture plate, and replaced with a serum-containing medium after 4–6 h of culturing.
Methyl thiazolyl tetrazolium (MTT) analysis
The NCI-H295R cells received drug induction or transfection culture as required, and were inoculated into 96-well plates after the cell growth became stable. The cells were cultured in the culture plate until the growth was stable, and then cell activity was determined by MTT. 200 µL of a 10% MTT solution (Procell, Wuhan) was added to each well of the culture plate, and the culture plate was cultured for 4 h in the dark. The culture medium in the culture plate was discarded, 100 µL of a dimethylsulfoxide (DMSO) solution was added to each well, and then the culture plate was placed on the shaking table for 10 min. The absorbance of each group of cell solutions at a wavelength of 450 nm was assessed using a microplate reader (Safe heart Technology Co. Ltd., Beijing), and the data were collected and processed. Each group had five multiple holes, and the blank control group was set. The experiment was repeated three times.
Establishment of a mouse APA model
The 20 C57BL/6 mice used in this study were purchased from cyagen Biotechnology, Inc (Guangzhou, China). The mice were fed in a single cage, fed and drank freely, and the room temperature was maintained between 20 and 25 ℃. Subcutaneous tumor formation was performed on mice using NCI-H295R cells, when the mice grew to the fourth week, all mice were anesthetized by intraperitoneal injection of 20% urethane (0.5 mL/100 g). Then, a mini-osmotic pump was inserted into their scapular region, through which aldosterone (1 µg/h) was pumped into the mice (21,22). The mice were continuously injected with aldosterone for 4 weeks, during which the tail artery systolic blood pressure was measured once a week. Experiments were performed under a project license granted by the Ethics Committee of Anhui Medical University (No. LLSC20231760), in compliance with guidelines of Institute of Cancer and Basic Medicine (IBMC) and the Chinese Academy of Sciences for the care and use of animals. A protocol was prepared before the study without registration.
10 mice implanted with a mini-osmotic pump were divided into two groups: an Sfrp2 overexpression group and a control group. Mice in the Sfrp2 overexpression group were intravenously injected with Sfrp2 lentivirus vector solution every 3 days, 500 µL each time, a total of 6 injections; while mice in the negative control group were intravenously injected with lentivirus vector solution without the target gene. When the mice grew to 8 weeks of age, venous blood was collected and plasma aldosterone concentration (PAC) was determined by radioimmunoassay.
In addition, 10 mice implanted with a micro-osmotic pump were divided into two groups: a Wnt inhibition group and a control group. Mice in the Wnt-inhibition group were intraperitoneally injected with KYA1797K (25 mg/kg, Selleck), and mice in the control group were intraperitoneally injected with the same dose of normal saline. The mice were killed when they grew to 8 weeks old. The adrenal tissue of mice was resected, and the zona glomerulosa tissue was taken for follow-up detection.
Statistical analysis
SPSS 20.0 software (Chicago, USA) was used for statistical analysis. The mean value and standard deviation of a large amount of data from the same group were calculated. Data differences between the two groups were statistically analyzed using the t-test. P<0.05 was considered statistically significant, and GraphPad Prism 8.0 software (California, USA) was used for mapping. All experiments in this paper were repeated three times.
Results
Abnormal activation of the WNT/β-catenin pathway in adrenal aldosterone adenoma
Previous studies have reported abnormal activation of the WNT/β-catenin pathway in APA. Therefore, this study collected adrenal tissue samples from APA and non-APA patients and employed immunohistochemistry to detect the expression of β-catenin in the tissues. As shown in Figure 1A, β-catenin was expressed in large quantities in APA tissues. Of all the APA tissue samples, 12 cases (75%) showed high β-catenin expression, and the remaining 4 cases showed low expression or no significant difference. β-catenin exhibited low expression in NA tissue. Subsequently, the expression levels of Sfrp2 and Wnt5a in the zona glomerulosa of the two groups of tissue samples were further assessed. Compared with NA group, Sfrp2 expression in APA tissues decreased by about 30%, while Wnt5a was up-regulated in APA tissues (Figure 1B). Western Blot detection also showed that Sfrp2 was highly expressed in NA tissues, while the Wnt pathway proteins Wnt5a, LEF1, TCF, and aldosterone synthase CYP11B2 were highly expressed in APA tissues (Figure 1C).
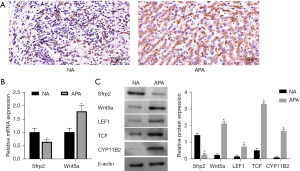
The WNT/β-catenin pathway regulates aldosterone secretion and influences the development of adrenal aldosterone adenoma
To verify the effect of WNT/β-catenin on aldosterone secretion, we used a WNT pathway inhibitor (KYA1797K) to inhibit β-catenin expression in NCI-H295R cells. Following induction culture with KYA1797K, the expression of β-catenin messenger RNA (mRNA) in H295R cells was decreased, and signal transduction of the WNT pathway was inhibited (Figure 2A). The expressions of the LEF1, TCF, and CYP11B2 proteins decreased, as did the secretion of aldosterone (Figure 2B). Next, the proliferative ability of APA cells was assessed. The proliferative ability of cultured cells induced by KYA1797K was significantly decreased, and the activity of cells in the inhibition group was lower than that in the control group (Figure 2C).
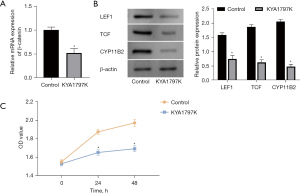
Change in the expression of Sfrp2, the regulation of Wnt pathway signaling, and the secretion of aldosterone
We further explored the regulatory role of Sfrp2 expression on the Wnt pathway and aldosterone secretion. An Sfrp2 silencing-RNA vector (Sfrp2 siRNA) and overexpression vector (Sfrp2 mimics) were transfected into NCI-H295R cells to detect the Wnt pathway activity in NCI-H295R cells. RT-PCR was used to test the transfection efficiency. The expression of Sfrp2 mRNA was decreased and β-catenin mRNA was increased in the siRNA-transfected cells. Following transfection with the overexpression vector, the expression of Sfrp2 mRNA was increased, while that of β-catenin mRNA was decreased.
Cell transfection was successful (Figure 3A). The expression of Wnt pathway-related proteins was assessed, and it was found that β-catenin, LEF1, TCF, and CYP11B2 protein expression were increased in the Sfrp2 siRNA group. The protein expression of β-catenin, LEF1, TCF, and CYP11B2 decreased in the Sfrp2 overexpression group (Figure 3B). The cell proliferation tests showed that suppressing the expression of Sfrp2 could promote the proliferative activity of APA cells, while increasing the expression of Sfrp2 could inhibit this activity (Figure 3C). These results showed that Sfrp2 acted as an inhibitor of the WNT pathway and negatively regulated the signal transduction of this pathway.
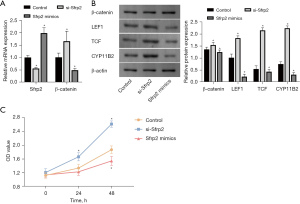
Increased expression of Sfrp2 in mice, inhibition of the activity of the WNT/β-catenin pathway, and secretion of aldosterone
We also established an APA mouse model. Sfrp2 gene expression in mice was increased, and the venous blood of mice was collected to detect the expression changes of β-catenin and CYP11B2 in plasma. Compared with the control mice, the plasma β-catenin and CYP11B2 expressions of Sfrp2-overexpressed mice were decreased (Figure 4A). Subsequent tests showed that increased Sfrp2 expression in APA mice could reduce the caudal arterial pressure and PAC (Figure 4B,4C). Also, increased Sfrp2 gene expression in mice could inhibit WNT/β-catenin signaling, aldosterone secretion, and APA development.
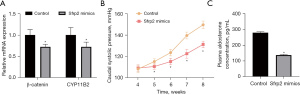
Inhibition of WNT/β-catenin pathway activity in mice and slowing the growth of adrenal aldosterone adenoma
The APA mice were intraperitoneally injected with a WNT/β-catenin inhibitor, KYA1797K. Compared with the inhibitor control group, the mRNA expression of β-catenin and arterial pressure in mice in the Wnt-inhibited group were decreased (Figure 5A,5B). The mice were killed and tumor tissues were collected for further detection. In the KYA1797K inhibition group, cell proliferation activity decreased, the tumor tissue was small, the growth rate was slow, and tumor growth was inhibited (Figure 5C,5D). Therefore, inhibiting the activity of the WNT/β-catenin pathway may help to slow the growth rate of adrenal aldosterone adenoma.
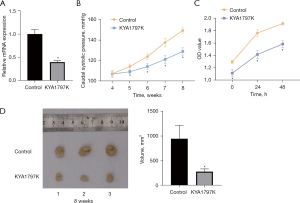
Discussion
PA is considered to be the leading cause of secondary endocrine hypertension. Compared with essential hypertension, PA-induced hypertension causes more serious organ damage and is more likely to result in cardiovascular diseases (23,24). Therefore, early treatment of PA can effectively reduce the morbidity and mortality of cardiovascular and cerebrovascular diseases. For some patients with special conditions, such as PA during pregnancy and elderly patients, drug therapy is more appropriate (25,26). Study have shown that in tumor cells, the WNT pathway is overactivated often due to gene mutations, and a large number of β-catenin proteins enter the nucleus for TCF/LEF transcriptional regulation (27). Study have shown that mutations in β-catenin genes in APA lead to abnormal activation of the WNT/β-catenin signaling pathway (28). Therefore, studying the WNT/β-catenin signaling pathway as a therapeutic target is a novel approach.
In this study, we assessed high expression of β-catenin in tissue samples from APA patients. Meanwhile, WNT pathway-related proteins (wnt5a, LEF1, and TCF) were highly expressed in APA samples. The WNT/β-catenin signaling pathway is abnormally activated in APA. As an antagonist of the WNT gene, Sfrp2 plays a role in various tumors by inhibiting the signal transduction of the WNT pathway (29,30). This experiment also found that in APA, Sfrp2 expression was low, while CYP11B2 expression and aldosterone secretion were high. It is speculated that abnormal activation of the WNT/β-catenin signaling pathway may be related to the secretion of aldosterone, and Sfrp2 can regulate aldosterone secretion by inhibiting the WNT/β-catenin signaling pathway.
KYA1797K is a WNT/β-catenin inhibitor that can promote the degradation of β-catenin by binding Axin and has been widely used in cancer stem cell studies (31,32). In this study, KYA1797K was applied to culture cells and inhibit the activity of the WNT/β-catenin signaling pathway. The results showed that inhibition of WNT/β-catenin signal transduction reduced the expression of CYP11B2 and the proliferative activity of APA cells. Thus, inhibition of the WNT/β-catenin signaling pathway can reduce aldosterone secretion and slow down APA development.
After regulating the expression of Sfrp2, we found that Sfrp2 could inhibit the expression of WNT/β-catenin pathway proteins and inhibit the secretion of aldosterone. In addition, the higher the expression of Sfrp2, the stronger the inhibition, and Sfrp2 acts as a WNT/β-catenin inhibitor. A mouse APA model was then established for in vivo experimentation. According to the literature, the dose of aldosterone used in this study was 1 µg/h, which was consistent with the dose used in common mouse APA models (33). The up-regulation of Sfrp2 gene expression in APA mice significantly reduced β-catenin expression and aldosterone concentration, and reduced arterial pressure in mice. After intravenous injection of KYA1797K in APA mice, the expression of β-catenin was inhibited, the arterial pressure of mice decreased, and the growth rate of adenoma slowed.
In this study, in vivo and in vitro experiments demonstrated that Sfrp2 inhibits the WNT/β-catenin signaling pathway by inhibiting the expression of β-catenin, thereby suppressing the secretion of aldosterone in mice. Increasing the expression of Sfrp2 in APA patients can effectively reduce the concentration of aldosterone and control patients' blood pressure, while simultaneously slowing the growth of APA tissues and delaying disease progression. Gene therapy with Sfrp2 is an effective treatment for patients undergoing conservative treatment. Increased aldosterone concentration in the human body will lead to a series of secondary diseases such as hypertension, hypokalemia, and hyperaldosteronemia; therefore, reducing aldosterone secretion has a significant effect on a variety of diseases.
Conclusions
In this study, we learned that Sfrp2 can inhibit aldosterone secretion by suppressing β-catenin expression, indicating that both the Sfrp2 and WNT pathways can be used as therapeutic targets for inhibiting aldosterone secretion and for the treatment of other diseases caused by increased aldosterone concentration. However, the mechanism between Sfrp2 and β-catenin still needs to be further explored.
Acknowledgments
Funding: None.
Footnote
Reporting Checklist: The authors have completed the ARRIVE and MDAR reporting checklists. Available at https://cdt.amegroups.com/article/view/10.21037/cdt-23-105/rc
Data Sharing Statement: Available at https://cdt.amegroups.com/article/view/10.21037/cdt-23-105/dss
Peer Review File: Available at https://cdt.amegroups.com/article/view/10.21037/cdt-23-105/prf
Conflicts of Interest: All authors have completed the ICMJE uniform disclosure form (available at https://cdt.amegroups.com/article/view/10.21037/cdt-23-105/coif). The authors have no conflicts of interest to declare.
Ethical Statement: The authors are accountable for all aspects of the work in ensuring that questions related to the accuracy or integrity of any part of the work are appropriately investigated and resolved. The study was conducted in accordance with the Declaration of Helsinki (as revised in 2013). Informed consent was taken from all the patients. The study was approved by the Ethics Committee of The Second Affiliated Hospital of Anhui Medical University (No. SL-YX 2023-SZR 090). This experiment was performed under a project license granted by the Ethics Committee of Anhui Medical University (No. LLSC20231760), in compliance with guidelines of Institute of Cancer and Basic Medicine (IBMC) and the Chinese Academy of Sciences for the care and use of animals.
Open Access Statement: This is an Open Access article distributed in accordance with the Creative Commons Attribution-NonCommercial-NoDerivs 4.0 International License (CC BY-NC-ND 4.0), which permits the non-commercial replication and distribution of the article with the strict proviso that no changes or edits are made and the original work is properly cited (including links to both the formal publication through the relevant DOI and the license). See: https://creativecommons.org/licenses/by-nc-nd/4.0/.
References
- Hundemer GL, Vaidya A. Primary Aldosteronism Diagnosis and Management: A Clinical Approach. Endocrinol Metab Clin North Am 2019;48:681-700. [Crossref] [PubMed]
- Reincke M, Bancos I, Mulatero P, et al. Diagnosis and treatment of primary aldosteronism. Lancet Diabetes Endocrinol 2021;9:876-92. [Crossref] [PubMed]
- Oki K, Gomez-Sanchez CE. The landscape of molecular mechanism for aldosterone production in aldosterone-producing adenoma. Endocr J 2020;67:989-95. [Crossref] [PubMed]
- Stavropoulos K, Imprialos K, Papademetriou V, et al. Primary Aldosteronism: Novel Insights. Curr Hypertens Rev 2020;16:19-23. [Crossref] [PubMed]
- Hellman P, Björklund P, Åkerström T. Aldosterone-Producing Adenomas. Vitam Horm 2019;109:407-31. [Crossref] [PubMed]
- Amar L, Plouin PF, Steichen O. Aldosterone-producing adenoma and other surgically correctable forms of primary aldosteronism. Orphanet J Rare Dis 2010;5:9. [Crossref] [PubMed]
- Amar L, Lorthioir A, Azizi M, et al. Progress in primary aldosteronism. Mineralocorticoid antagonist treatment for aldosterone-producing adenoma. Eur J Endocrinol 2015;172:R125-9. [Crossref] [PubMed]
- Nakamura Y, Yamazaki Y, Tezuka Y, et al. Expression of CYP11B2 in Aldosterone-Producing Adrenocortical Adenoma: Regulatory Mechanisms and Clinical Significance. Tohoku J Exp Med 2016;240:183-90. [Crossref] [PubMed]
- Gomez-Sanchez CE, Kuppusamy M, Reincke M, et al. Disordered CYP11B2 Expression in Primary Aldosteronism. Horm Metab Res 2017;49:957-62. [Crossref] [PubMed]
- Zennaro MC, Boulkroun S, Fernandes-Rosa FL. Pathogenesis and treatment of primary aldosteronism. Nat Rev Endocrinol 2020;16:578-89. [Crossref] [PubMed]
- Zhang G, Zou X, Liu Q, et al. MiR-193a-3p functions as a tumour suppressor in human aldosterone-producing adrenocortical adenoma by down-regulating CYP11B2. Int J Exp Pathol 2018;99:77-86. [Crossref] [PubMed]
- Clevers H, Nusse R. Wnt/β-catenin signaling and disease. Cell 2012;149:1192-205. [Crossref] [PubMed]
- Perugorria MJ, Olaizola P, Labiano I, et al. Wnt-β-catenin signalling in liver development, health and disease. Nat Rev Gastroenterol Hepatol 2019;16:121-36. [Crossref] [PubMed]
- Chen J, Rajasekaran M, Hui KM. Atypical regulators of Wnt/β-catenin signaling as potential therapeutic targets in Hepatocellular Carcinoma. Exp Biol Med (Maywood) 2017;242:1142-9. [Crossref] [PubMed]
- De Sousa K, Abdellatif AB, Giscos-Douriez I, et al. Colocalization of Wnt/β-catenin and ACTH signaling pathways and paracrine regulation in aldosterone producing adenoma. J Clin Endocrinol Metab 2022;107:419-34. [Crossref] [PubMed]
- Berthon A, Drelon C, Ragazzon B, et al. WNT/β-catenin signalling is activated in aldosterone-producing adenomas and controls aldosterone production. Hum Mol Genet 2014;23:889-905. [Crossref] [PubMed]
- Wang JC, Li YQ, Feng DY, et al. Loss of Sfrp2 contributes to the neurological disorders related with morphine withdrawal via Wnt/β-catenin signaling. Behav Brain Res 2019;359:609-18. [Crossref] [PubMed]
- van Loon K, Huijbers EJM, Griffioen AW. Secreted frizzled-related protein 2: a key player in noncanonical Wnt signaling and tumor angiogenesis. Cancer Metastasis Rev 2021;40:191-203. [Crossref] [PubMed]
- Wu Y, Liu X, Zheng H, et al. Multiple Roles of sFRP2 in Cardiac Development and Cardiovascular Disease. Int J Biol Sci 2020;16:730-8. [Crossref] [PubMed]
- Zhou T, Luo P, Wang L, et al. CTNNB1 Knockdown Inhibits Cell Proliferation and Aldosterone Secretion Through Inhibiting Wnt/β-Catenin Signaling in H295R Cells. Technol Cancer Res Treat 2020;19:1533033820979685. [Crossref] [PubMed]
- Aragao-Santiago L, Gomez-Sanchez CE, Mulatero P, et al. Mouse Models of Primary Aldosteronism: From Physiology to Pathophysiology. Endocrinology 2017;158:4129-4138. [Crossref] [PubMed]
- Leccia F, Batisse-Lignier M, Sahut-Barnola I, Val P, Lefrançois-Martinez AM, Martinez A. Mouse Models Recapitulating Human Adrenocortical Tumors: What Is Lacking? Front Endocrinol (Lausanne) 2016;7:93. [Crossref] [PubMed]
- Chambaz IC, Pechère-Bertschi A. Primary hyperaldosteronism. Rev Med Suisse 2011;7:1736-8, 1740-2. [PubMed]
- Funder JW, Carey RM. Primary Aldosteronism: Where Are We Now? Where to From Here? Hypertension 2022;79:726-35. [Crossref] [PubMed]
- Araujo-Castro M. Treatment of primary hyperaldosteronism. Med Clin (Barc) 2020;155:302-8. [Crossref] [PubMed]
- Ito H, Sasaoka A, Takao T, et al. Aldosterone-producing adrenocortical adenoma complicated by chronic renal failure. Case report and review of the literature. Am J Nephrol 1998;18:541-6. [Crossref] [PubMed]
- Nusse R, Clevers H. Wnt/β-Catenin Signaling, Disease, and Emerging Therapeutic Modalities. Cell 2017;169:985-99. [Crossref] [PubMed]
- Åkerström T, Maharjan R, Sven Willenberg H, et al. Activating mutations in CTNNB1 in aldosterone producing adenomas. Sci Rep 2016;6:19546. [Crossref] [PubMed]
- Kaur A, Webster MR, Marchbank K, et al. sFRP2 in the aged microenvironment drives melanoma metastasis and therapy resistance. Nature 2016;532:250-4. [Crossref] [PubMed]
- van Andel H, Kocemba KA, Spaargaren M, et al. Aberrant Wnt signaling in multiple myeloma: molecular mechanisms and targeting options. Leukemia 2019;33:1063-75. [Crossref] [PubMed]
- Cho YH, Ro EJ, Yoon JS, et al. Small molecule-induced simultaneous destabilization of β-catenin and RAS is an effective molecular strategy to suppress stemness of colorectal cancer cells. Cell Commun Signal 2020;18:38. [Crossref] [PubMed]
- Ruan Z, Liang M, Lai M, et al. KYA1797K down-regulates PD-L1 in colon cancer stem cells to block immune evasion by suppressing the β-catenin/STT3 signaling pathway. Int Immunopharmacol 2020;78:106003. [Crossref] [PubMed]
- Fernandes-Rosa FL, Boulkroun S, Zennaro MC. Genetic and Genomic Mechanisms of Primary Aldosteronism. Trends Mol Med 2020;26:819-32. [Crossref] [PubMed]
(English Language Editor: A. Kassem)