Apoptosis-inducing factor: a mitochondrial protein associated with metabolic diseases—a narrative review
Introduction
Background
Apoptosis-inducing factor (AIF) is a nuclear encoded mitochondrial inter-membrane space flavoprotein, regarded as an apoptotic effector molecule. AIF is encoded by the AIFM1 gene which has a total length of 36,525 bases and is located in the Xq26.1 region, with 17 exons and four variable shears (1). Accumulated research results on AIF have gradually enhanced our understanding of its structure and function. Most of the AIF-like proteins possess the GXGXXA and V/IXGXGXXG motifs, characterized by the FAD- and NAD(P)-binding domains. The N-termini of mammal AIF possesses mitochondrial leading sequence (MLS) and a stretch of hydrophobic amino acids (2). AIF is an evolutionarily highly conserved protein with significant homology in both vertebrates and invertebrates and is associated with the development of a variety of diseases, including X-linked recessive auditory neuropathy spectrum disorder (3), retinopathy (4,5), chronic kidney disease (6,7), diabetes (8,9), and malignancy (10,11).
Rationale and knowledge gap
AIF is widely believed to play a role in various diseases by regulating apoptosis. It transfers from mitochondria to the nucleus and induces apoptosis in a variety of cell death models, such as DNA damage reagent treatment (12), glutamate-induced excitotoxicity (13,14) or N-methyl-D-aspartic acid (NMDA) receptor (NMDAR) agonists (15), and hypoxic-ischemic reperfusion (16,17). Importantly, the overexpressed anti-apoptotic molecule, heat shock protein 70 (HSP70), can inhibit its transfer to the nucleus by binding to AIF, ultimately protecting the nerves of hypoxic or ischemic transgenic mice (18,19). Moreover, mitochondrial soluble 54–77 portion that stabilize human AIF at intermembrane mitochondrial space pH may produce a negative impact in overall human AIF stability, which provides guidance for developing human AIF bioactive compounds (20). These findings suggest that AIF is a potential drug development target.
In addition to being involved in the signaling pathway of lethal cells, AIF plays a key role in maintaining mitochondrial homeostasis (21,22). AIF is not only an important factor in maintaining the functional integrity of the mitochondrial respiratory chain but also participates in the regulation of the redox state of cells, such as by regulating the activity of nicotinamide adenine dinucleotide hydrogen (NADH) oxidase to affect reactive oxygen species (ROS) levels. Recent evidence indicates that AIF is necessary for the activity of coiled-coil-helix-coiled-coil-helix domain-containing 4 (CHCHD4), which is a critical intermembrane mitochondrial space protein involved in the oxidative folding of respiratory complexes subunits, suggesting the importance of AIF in respiratory chain biogenesis and/or maintenance (23,24). Also, AIF acts as a mitochondrial flavin adenine dinucleotide (FAD)-dependent oxidoreductase in normal cells, playing a key role in regulating oxidative phosphorylation (OXPHOS) and the oxygen reflux state equilibrium. Therefore, AIF is currently considered to be a bifunctional protein with an electron acceptor/donor (oxidoreductase) function (21,22).
The respiratory chain is a continuous reaction system comprising a series of hydrogen transfer and electron transmission reactions arranged in a certain order, which gives the paired hydrogen atoms taken off by metabolites to oxygen to make water, and adenosine triphosphate (ATP) is generated. The mitochondrial AIF protein is an important factor in maintaining the functional integrity of the respiratory chain in mammalian cells, while AIF deletion can lead to mitochondrial respiratory chain destruction through lowering the amount of respiratory chain complex OXPHOS (25) as well as the regulation of post-translational modification of the related subunits of mitochondrial respiratory chain complex I, III, IV (26,27). For instance, in vivo experiments revealed that mice with tissue conditional knockout AIF exhibited severe mitochondrial lesions (28). In fact, previous articles have reviewed the current knowledge around the critical role of AIF focusing on AIFM1-linked pathologies in mitochondrial diseases (21,29). Acting as the apoptosis inducer, a comprehensive understanding of AIF-mediated apoptosis and AIF-related metabolic diseases is essential.
Objective
With new findings on the role of AIF in metabolism being reported in some recent publications, it is necessary to carefully review and re-analyze the published literature on AIF-mediated apoptosis and AIF-related metabolic diseases. We present this article in accordance with the Narrative Review reporting checklist (available at https://cdt.amegroups.com/article/view/10.21037/cdt-23-123/rc).
Methods
We conducted a literature search of the PubMed database for papers published up to June 2022 on the topic of AIF in metabolic diseases using the following keywords: “apoptosis”, “metabolism” or “mitochondria” plus “apoptosis-inducing factor”. Table 1 shows our search formulas and strategies. The retrieved articles were further analyzed.
Table 1
Items | Specification |
---|---|
Date of search | 2022.6.20 |
Databases and other sources searched | PubMed |
Search terms used | “Apoptosis-inducing factor” |
“Apoptosis-inducing factor” AND “apoptosis” | |
“Apoptosis-inducing factor” AND “metabolism” | |
“Apoptosis-inducing factor” AND “mitochondria” | |
Timeframe | From 1996 to 2022 |
Inclusion and exclusion criteria | Inclusion criteria: papers mentioning or reporting on the biological functions of AIF and the role of AIF in apoptosis and metabolic diseases |
Exclusion criteria: non-English language papers | |
Selection process | The selection was independently conducted by L Zong and was discussed with Z Liang in the case of any disagreements |
AIF, apoptosis-inducing factor.
Discussion
Biochemistry, molecular characterization, and regulation of expression
The human AIFMI gene is localized to chromosome Xq26.1 and has five different isomers, namely AIF, AIF-exB, AIFsh, AIFsh2, and AIFsh3, which are formed in different selective shear modes. Among these, isomer 1 (AIF) is the most widely expressed in tissues and plays a major role, while the other isomers have their own tissue specificity and play different roles in tissues according to the functional domains they contain. The AIFsh2 and AIFsh3 proteins have a similar structure to the N-terminal portion of AIF; AIFsh2 has a weak expression in kidney and lung tissue and its messenger RNA (mRNA) cannot be detected in the brain, while AIFsh3 is only weakly expressed in kidney tissue (30). However, the structure of the AIFsh protein is similar to the C-terminal part of AIF, which can induce apoptosis under the stimulation of the apoptotic signal; however, owing to the lack of an NADH domain, it does not possess oxidoreductase activity, and also has weak expression in skeletal muscle and kidneys (31).
Since the AIF isomer 1 is the primary functional form of AIF, its full-length proteins, which are also known as AIF precursors, can be roughly divided into three domains: FAD binding domain (amino acids 122–262 and 400–477 positions), NADH binding domain (amino acids 263–399 position), and C-terminal domain (amino acids 478–610 position) (32). The crystal structure of mouse AIF exhibits a folding pattern, with the FAD binding domain and the NADH binding domain displaying the classic Rossman fold, while the C-terminal domain has five anti-parallel β-chains (amino acids 477–579 bits) and two α-helix (amino acids 580–610 bits) (32). Meanwhile, the crystal structure of human AIF is displayed by one FAD binding domain and two NADH molecules per protomer at NADH reduced state, and exhibits as the orthorhombic space group P212121 with two molecules per asymmetric unit in its oxidized form (23,33). The human AIF precursor protein contains 613 amino acids, two mitochondrial localization sequences, and two nuclear localization signals, and its mitochondrial localization sequences are amino acids 1–31 and 63–89, respectively, while the two nuclear localization sequences are amino acids 278–301 and amino acids 446–451, respectively (34). The AIF precursor protein has a molecular weight of about 67 kDa. AIF is first synthesized in the cytoplasm and then translocated into mitochondria based on the mitochondrial localization sequence (MLS) in its N-terminus, followed by processing into the mature mitochondrial AIF after mitochondrial processing peptidase (MPP) cleavage (35). Mature mitochondrial AIFs, with 55–613 amino acids and molecular weights of approximately 62 kDa, are anchored to the mitochondrial membrane by a second mitochondrial localization sequence, exposing the C-end to the inter-mitochondrial membrane cavity to perform the function of the mitochondrial oxidative respiratory chain complex I (26,27).
AIF-mediated apoptotic process is regulated by multiple genes, including p53, eukaryotic translation initiation factor 3 subunit p44 (eIF3g), and HSP70 (19,36,37). AIF is positively regulated by the p53 protein but it is regulated differently from most p53-targeted genes. Also, the basal expression level of AIF is regulated by p53, and the activated p53 gene does not lead to a further increase in AIF expression (36,38). There is a p53-binding element in the first intron of the AIF, and wild-type p53 can induce the degradation of large DNA fragments by producing activated AIFs and promote the programmed death of caspase-independent cells (39). The lack of functional p53 tends to result in decreased AIF expression in cells but the degree of reduction in AIF varies from cell to cell. For example, p53 deletions severely decrease AIF expression in MCF7, HCT116, and H1299 cells, while some other types of cells present only a slight reduction in AIF expression following p53 deletions (40). AIF can interact with eukaryotic translation initiation factor 3 subunit p44 (eIF3g) with sites of action in the C-terminal region of eIF3g N-terminus and AIF. The results of co-immunoprecipitation and confocal microscopy also confirm the interaction between AIF and eIF3g and demonstrate that eIF3g can release AIF inhibition of protein production (37). Moreover, the overexpression of mature AIF proteins can be further amplified by activating caspase-7 and affecting the cleavage of eIF3g (37). Furthermore, some other molecules, such as PARP1 (41), Harlequin (42), and PAK5 (43), have also been identified to influence AIF expression directly or indirectly.
Biological function
The biological function of AIF is mainly divided into two parts, that is, it regulates the redox reaction in normal cells and exerts a pro-apoptotic effect when stimulated by apoptotic factors. The specific mechanisms through which AIF exerts these two biological functions differ but also intersect.
Role of AIF in death
The apoptotic pathway is divided into an endogenous endoplasmic reticulum pathway, an endogenous mitochondrial pathway, and an exogenous death receptor pathway (Figure 1). AIF-mediated apoptosis belongs to the mitochondrial apoptotic pathway. AIF is localized on the mitochondrial membrane, and when stimulated by the apoptotic signal, 62 kDa AIF is hydrolyzed into a mature protein with a relative molecular mass of 57 kDa, which exhibits apoptotic potential (44,45). After stimulation by an apoptotic factor, AIF can be translocated from the mitochondria to the nucleus to induce apoptosis; although AIF does not possess the activity of endonuclease itself, recent evidence suggests that AIF can degrade DNA by its own and within a degradosome complex consisting of AIF and its association with proteins such as endonuclease CypA and histone H2AX, thereby directly leading to DNA breakage (46). In addition, AIF indirectly exerts its pro-apoptotic effect by acting on other proteins, such as EndoG (Figure 2).
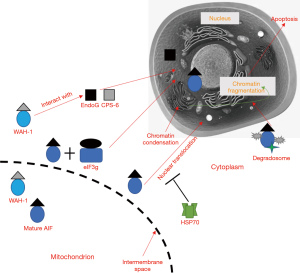
EndoG is an endonuclease that is capable of damaging both DNA and RNA; in mammalian cell apoptosis, EndoG can exert endonuclease activity, inducing DNA breaks. In Caenorhabditis elegans (C. elegans), Worm AIF Homolog (WAH-1) and CED-3 protease suppressor-6 (CPS-6) are homologs of the mammalian AIF and EndoG, respectively. WAH-1 is localized in the mitochondria of C. elegans and relies on the Bcl-2 homology domain 3 (BH3)-binding protein egg-laying defective-1 (EGL-1) to be released into the cytoplasm and nucleus in a caspase (CED-3)-dependent approach. Additionally, WAH-1 interacts with the mitochondrial digest CPS-6/EndoG to induce DNA fracture and apoptosis (47).
Mycobacterium bovine can induce bovine macrophage death via AIF-mediated non-caspase-dependent apoptosis, and the expression of AIF and EndoG proteins is increased, indicating that EndoG is involved in the apoptotic process of bovine macrophages with AIF involvement (48). The overexpression of HSP70 has been demonstrated to reduce apoptosis in multiple studies, and part of its mechanism is thought to be related to HSP70 preventing AIF release from mitochondria by inhibiting the activation of the BAX protein (49). With the assistance of nuclear transport receptors recognizing nuclear localization signal (NLS)1 and NLS2, AIF is translocated from the cytoplasm to the nucleus. eIF3g facilitates while HSP70 inhibits the cytonuclear translocation of AIF. After entering nucleus, AIF binds directly to DNA and causes peripheral chromatin condensation, which may lead to large-scale chromatin fragmentation.
At the same time, AIF can also promote apoptosis by modulating oxidoreductase activity. Some researchers believe that the oxidoreductase activity of AIF is not necessary to induce apoptosis, and that the oxidoreductase function and the induced apoptotic function of AIF can be completely separated, and that these two functions do not affect each other (50). In contrast, there are also studies showing that some redox regions of AIF affect its pro-apoptotic function, such as the presence of amino acids 509–559 polypeptide at the C-terminal of AIF, which is not only a redox-related sequence but also serves as a proteolytic signal and phosphorylation site, regulates the activity of calpain I, and affects the sensitivity of AIF to proteases as well as its ability to interact with DNA. This is critical to initiating caspase non-dependent apoptosis, and thus, the sequence function changes. Moreover, binding and dissociation with reduced NADH also affect its apoptosis function (51,52). Thus, there is a close relationship between the apoptotic and oxidoreductase activities of AIF; however, the specific transformation regulation mechanism of these two processes is still unclear.
In addition, AIF is critical for poly (ADP-ribose) polymerase-1 (PARP-1)-dependent cell death (parthanatos), which is a programmed necrotic cell death pathway characterized by PARP1 hyper-activation resulting in the transport of AIF from mitochondria to the nucleus, fragmenting DNA. Parthanatic cell death is a caspase-independent cell death, and does not induce apoptotic body formation or small DNA fragmentation, distinct from apoptosis, necrosis or other types of cell death (53,54). Parthanatos can be initiated by nitric oxide production, oxidative stress and extensive DNA damage. Widespread DNA damage causes overactivation of PARP1, which catalyzes the formation and accumulation of PAR polymer. The released PAR enters into cytosol where it binds AIF making it release from the mitochondria and translocate into the nucleus, inducing chromatin and nuclear condensation fragmentation and ultimately leading to parthanatic death cascade (55). AIF is regarded as the final executioner of parthanatos, and the AIF-mediated parthanatos has been broadly involved in the pathogenesis of various diseases, including nervous systems diseases, cancers and cardiovascular diseases (56-58).
Role of AIF in metabolism: molecular basis of its contribution to metabolic diseases
After AIF was identified, scientists focused on regulating chromatin coagulation and apoptosis. However, AIF alone as an apoptosis regulator does not explain many observations, such as AIF-deficient neurons exhibiting higher oxidative stress and increased bromodeoxyuridine accumulation (28,59). At this stage, the focus of researchers has shifted to the important role of AIF in maintaining mitochondrial energy metabolism as a mitochondrial oxidoreductase. Vahsen et al. observed a decrease in the rate of oxygen consumption in embryonic stem (ES) cells and increased production of lactate in AIF-knockout mice, and also found that AIF-deficient mice exhibited decreased OXPHOS in the retina and brain. This was associated with the decreased level of complex I subunits, neuronal defects, and retinal degeneration (60). In addition, recent studies have found that AIF deficiency in Drosophila larvae induces the decrease of complex I and complex IV activity, causing impaired OXPHOS and eventually leading to larval growth arrest and lethality (61,62). These findings suggest that AIF plays a significant pro-apoptotic role and also exerts an important function in the process of mitochondrial energy metabolism.
AIF also maintains mitochondrial structure and function. Given the ability of electron microscopy to examine neuronal cells lacking AIF with broken mitochondria and abnormal ridges, Cheung et al. speculated that AIF is involved in maintaining the structure of mitochondria (63). Although AIF is not a constituent of complex I, it can indirectly regulate the respiratory chain by recruiting and/or stabilizing the composition of the oxidative respiratory chain complex I and III (26,27). The absence of AIF affects the expression of cell complex I and its constituent elements. Human or mouse cells caused to not express AIF by homologous recombinant small interfering RNA (siRNA), have significantly weakened oxidative respiratory chain complex I activity and increased lactate and glycolysis-dependent ATP production, which can affect mitochondrial OXPHOS (28). Complete AIF knockout mice die before birth due to the absence of AIF to induce the loss of OXPHOS, thereby affecting the activity of NADH-dependent ferrocyanide reductase, so that the respiratory chain complex I function is insufficient resulting in considerable apoptosis (64). Zong et al. argued that the apoptosis of spiral ganglion neurons caused by abnormal expression of AIF is also associated with mitochondrial respiratory activity and impaired membrane potential (65).
In particular, in a physiological state without apoptotic stimulation, the down-regulation of AIF expression can inhibit aerobic respiration in mitochondria, prompting ATP production to shift to the glycolysis pathway, which is very similar to the “Warburg effect” of tumor metabolism (27). In addition, both hypoxia and hypoxia-inducible factor-1alpha (HIF-1α) overexpression can inhibit the transcriptional activity of the AIF through targeting hypoxia-response element (HRE). AIF is also a target gene for HIF-1α in tumor cells (66,67). In recent years, research on tumor metabolism has become more comprehensive, and researchers have focused more on the correlation between mitochondria and tumors. AIF, as a key molecule in mitochondria, plays a key role in maintaining mitochondrial homeostasis and ROS levels and is related to the development of tumors (43,68). Specific cases of AIF involvement in the development of tumors through metabolic regulation will be described in detail at a later stage.
AIF and metabolic diseases
With the development of a social economy and the improvement of human material living conditions, obesity, type 2 diabetes, dyslipidemia metabolism, and non-alcoholic fatty liver disease (NAFLD) have become common diseases worldwide, with high incidence rates that are increasing every year as well as a trend toward younger patients. In addition, cancer can alter the metabolism of cancer cells and normal tissues, and then, by combining with internal and external factors, affects systemic metabolism. As a mitochondrial protein, AIF is involved in regulating cellular energy metabolism by modulating mitochondrial structure and function, and is associated with metabolic abnormalities in a variety of metabolic diseases.
Diabetes: a metabolic disease that is regulated by AIF-mediated apoptosis
Diabetes mellitus is a metabolic disorder with a variety of predisposing factors. Its main clinical features are hyperglycemia, glucose, fat, and protein metabolism due to defect in insulin secretion or insulin action. Type 2 diabetes mellitus (T2DM), the most common type of diabetes in the clinic, is a complex metabolic disorder characterized by insulin resistance and islet β-cell dysfunction, which involves genetic, epigenetic, environmental, and lifestyle factors. Insulin resistance occurs in the early stages of T2DM, which, due to structural damage, gradually leads to a decrease in the ability of β cells to secrete insulin, eventually leading to glucose and lipid metabolism disorders (69). Mitochondrial oxidative stress is known to be a key factor in the dysfunction of insulin resistance and β cells. Excess ROS activate downstream apoptotic factors, including cytochrome C (Cyto-C) and AIF, and induce the apoptosis of β cells (70). Drugs targeting this mechanism can protect β cells in mouse models of T2DM from apoptotic death (9,71).
Diabetic complications are a direct threat to the lives of diabetic patients, including microangiopathies such as diabetic nephropathy and diabetic retinopathy. Apoptosis is the pathological basis of diabetic microangiopathy, while AIF-mediated apoptosis is the molecular basis leading to increased diabetic apoptosis. By detecting the expression of anti- and pro-apoptosis molecules in the retinas of diabetic and non-diabetic patients, it was found that AIF expression was increased in the retinas of diabetic patients. The enforced AIF can transport to the nucleus to cause peripheral chromatin condensation and large-scale fragmentation of DNA and to deteriorate apoptotic process in a caspase-independent manner. Thus, the inhibition of AIF nuclear translocation was the basic mechanism of the neuroprotective activity of pigment-epithelial-derived factors in rat models of retinal degeneration (72,73). In addition, in a study on the apoptosis of retinal pigment epithelial (RPE) cells induced by oxidative stress, the inhibition of AIF activity significantly reduced ROS production and RPE cell apoptosis (74). Therefore, inhibiting the highly expressed AIF in the retinas of diabetic patients may be a potential method of preventing diabetic retinopathy.
Mitochondrial diseases and AIFM1 gene mutation
Mitochondria, an organelle that exists in almost all eukaryotes, are the energy factories of cellular activity. Its main function is the OXPHOS synthesis of ATP, which is the site of the final oxidative release of substances such as sugars, fats, and amino acids. In general, the number of mitochondria in cells determines the level of metabolism; the more vigorously metabolized the cell, the more mitochondria there are. Therefore, mitochondrial function and structural abnormalities can cause metabolic diseases. Mitochondrial disease refers to a group of heterogeneous lesions induced by genetic defects that cause mitochondrial metabolic enzymes, resulting in ATP synthesis disorder and insufficient energy sources. Mitochondrial diseases usually involve multiple organs, and the organs which are highly ATP-dependent, such as the brain and skeletal muscle, are the most severely affected. They are clinically manifested as mitochondrial myopathy and mitochondrial encephalomyopathy.
In recent years, an increasing number of AIFM1 pathogenic mutations have been reported to be associated with mitochondrial diseases. The AIF1M1 pathogenic mutation was initially observed in male infants born from identical twin sisters (75). It was found that deletions of trinucleotides in exon 5 of the X-linked AIFM1 gene resulted in a FAD-binding domain. In addition, the AIF variant appeared to have folding features that were comparable to wild-type AIF; Nevertheless, AIF mutant polypeptides show weak FAD binding, which in turn leads to abnormal redox properties (76).
Subsequently, maternal heterogeneous mutations in the 9th exon of the AIFM1 gene result in AIF variants, which replace glycine (G308E) of 308 residues with glutamic acid, severely interfering with the AIF redox activity and NADH affinity (77). Another mistaken AIFM1 gene mutation was found in an American-Italian family where the substitution of glutamic acid-valine at residue 493 (E493V) led to hereditary Cowchock syndrome, which is a child-onset Charcot-Marie-Teeth disease (78). Recently, multiple clinical reports have described multiple pathogenic mutations of the AIFM1 gene, such as 12 mutant residues located in the two FAD-binding fragments [D237G (79), F210 L and F210S (80), V243 L (81), R201 del (75), T260A, G262S (82); R430C, R451Q, A472V, P475L, R422W and R422Q (3); Q479R (83)], four mutations within the NADH binding domain [G308E (77), G338E (84), L344F and G360R (3)], and three mutations located in the C-terminal region of the AIF protein [E493V (78), V498 M and I591M (3)]. In addition, it is notable that G3071 mutation can affect AIF at the molecular level, while E493V, DR201, and G308E mutations regulate human AIF stability; In particular, DR201 induces a population transfer to a less stable conformation, thereby remodeling the structure and dynamics of active sites (20,23). However, to date, little is known about the molecular mechanisms of OXPHOS, mitochondrial function, and apoptosis alterations caused by AIF mutations.
Cancer metabolism: AIF is deeply involved and potential drug targets
In the 1920s, Warburg observed that in vitro tumor tissue sections produced lactic acid using large amounts of glucose even in the presence of oxygen, a phenomenon defined as aerobic glycolysis or the Warburg effect (85,86). In recent decades, with the development and application of technology, researchers have not only revealed tumor heterogeneity and plasticity but have also discovered new metabolic pathways to maintain tumor growth. It has also been gradually established that tumorigenesis and development require the metabolic reprogramming of tumor cells. Tumor cells autonomously alter their fluxes through various metabolic pathways to meet increased bioenergy and biosynthetic needs and to alleviate the oxidative stress required for tumor cells to proliferate and survive. The depletion of certain nutrients may occur in the tumor microenvironment (TME), which maintains tumor cell proliferation by inducing nutrient clearance mechanisms, forcing tumor cells to adapt. In addition, tumor cell metastasis is the leading cause of death in cancer patients, and efforts have been made to explore how metastatic cells regulate metabolism. It is worth noting that AIF is abnormally expressed in multiple tumor tissues, such as lung cancer (27,68), breast cancer (43), pancreatic cancer (87), colorectal cancer (88), renal cell cancer (66), etc. At the same time, it has been demonstrated that down-regulating the expression of the AIF in the physiological state without apoptosis stimulation can inhibit aerobic respiration in mitochondria, prompting the ATP production to shift to the glycolysis pathway, which displays an effect that is consistent with the “Warburg effect” (27).
Hypoxia is a distinguishing feature of the TME, and we have previously described that both hypoxia and HIF-1α can have an impact on the transcriptional activity of AIF. Studies on colorectal cancer tissues and cell lines have shown that hypoxia can negatively regulate the expression of AIF, and AIF deletion can promote the inactivation of phosphatase and tensin homolog (PTEN)-induced OXPHOS and activate the protein kinase B (Akt)/glycogen synthase kinase 3 (GSK-3)-3/β-catenin signaling pathway, promoting tumor metabolic metabolism, and related signaling pathways to promote tumor epithelial interstitial transformation (66). Yang et al. found that overexpression of the CHCHD4 protein molecule increases the stability of HIF-1α under low-oxygen culture conditions, thereby regulating tumor cell growth and metastasis (89). It is worth mentioning that researchers have found that AIF and CHCHD4 can directly bind to and regulate the biosynthesis of mitochondrial respiratory chains, and play a critical role in the regulation of cellular energy metabolism processes (90). AIF is also an essential control factor for regulating the mitochondrial transport process of CHCHD4 translation coupling (24,91).
Furthermore, AIF, as an inducer of cell death, is necessary for specific stimuli-induced apoptosis pathways. Some studies have confirmed that it has anti-tumor activity (92); however, recent studies have shown that AIF has a survival-promoting effect, and high AIF expression has a certain pro-tumor activity. The overexpression of AIF protects malignantly transformed colorectal cancer cells from apoptosis under stress (93). This protective effect will lead to a modest increase in the level of oxidation in conventional cells, which is consistent with the role of AIF in regulating cellular ROS levels (94,95). Oxidative stress due to increased ROS is common in numerous types of tumorigenesis and models of tissue ischemia due to low blood flow (36,95). While high levels of oxidative stress inhibit tumor development, chronically increased oxidative stress continues to activate cellular stress pathways, resulting in malignant transformation or other biological behaviors in cells (96).
Moreover, ROS produced in multiple cell types acts as a signaling molecule to promote cell proliferation by activating relevant pathways (97). In developing tumor cells, ROS can affect the ingestion and consumption of glucose by tumor cells and the synthesis of cell macromolecules by modifying changes in the way cells are metabolized (98). Therefore, AIF overexpression can promote tumorigenesis by regulating oxidative stress and metabolic activity, and targeted AIF has the potential to treat malignancies (Table 2). For instance, attenuated Salmonella engineered with AIF eukaryotic expressing system triggers a decent anti-tumor effect in melanoma, providing a potential reagent for cancer therapy (99); Montelukast, a widely used drug for treating allergic asthma and rhinitis, was revealed to induce lung cancer cell death via the nuclear translocation of AIF, indicating a potential utility of montelukast in cancer prevention and treatment (100).
Table 2
Cancer type | Drugs and mechanism | Ref. |
---|---|---|
Melanoma | Salmonella engineered with an AIF eukaryotic expressing system | (99) |
Lung cancer | Montelukast: induces cell death via the nuclear translocation of AIF | (100) |
Bone morphogenetic protein inhibitors: induces apoptosis via AIF | (101) | |
Mitochondria targeting agents: induces apoptosis via AIF | (101) | |
Ovarian cancer | IFN-α2a: induces cell death via the nuclear translocation of AIF | (102) |
Diarylheptanoid hirsutenone: enhances chemotherapy sensitivity | (103) | |
Melanoma skin cancer | Sanggenol L: promotes apoptotic cell death via the activation of caspase cascades and AIF | (104) |
Colorectal cancer | Denbinobin: induces apoptosis by AIF release and DNA damage | (105) |
Breast cancer | Capsaicin: AIF-mediated mitochondrial dysfunction | (106) |
Dioscin: induces caspase-independent apoptosis through AIF activation | (107) | |
Vitamin C: induces apoptosis via AIF | (108) | |
Prostate cancer | Crude saponins: induces caspase-independent apoptosis via AIF activation | (109) |
The table is restricted to publications with the term “AIF” in the title. AIF, apoptosis-inducing factor; IFN, interferon.
Strengths and limitations
This study conducts a comprehensive review of the molecular mechanism of AIF in metabolic mediation and metabolic diseases, as well as the AIF-mediated apoptotic process, which helps us obtain a better understanding of AIF-mediated death and AIF-related metabolic diseases.
However, limitations still exist in this review as some mechanisms are still unclear and require further study, such as the relationship between AIF and ROS, whether AIF can clear ROS or induce ROS production, and whether these two contradictory phenomena are related to the localization of AIF or have cell specificity. Furthermore, the mechanism through which AIF within the nucleus induces chromosome condensation and DNA breakdown into large fragments, and the specific mechanisms by which AIF interacts with those factors in this process is also needs to be further investigated.
Conclusions
AIF exerts redox and pro-apoptotic activities, both of which ensure that it plays an important role in metabolic diseases. AIF, as an apoptotic-inducing factor, can promote apoptosis under the induction of apoptosis signals, which will become the pathological basis for some metabolic diseases, such as diabetes. Also, AIF affects the assembly of complex 1 as well as its stability and mitochondrial function. AIF mutations will directly induce mitochondrial dysfunction and lead to mitochondrial metabolic diseases, and its abnormal expression will also cause tumor cell metabolism changes by affecting the respiratory chain. However, AIF-mediated apoptosis or metabolic function influences each other. Interfering with the metabolic function can inadvertently cause apoptosis and interfering with the apoptosis function has the potential to alter metabolism in a pathologic manner. Hence, pathological mechanisms underlying AIF-influenced metabolic diseases are complicated and multifaced.
Acknowledgments
Funding: None.
Footnote
Reporting Checklist: The authors have completed the Narrative Review reporting checklist. Available at https://cdt.amegroups.com/article/view/10.21037/cdt-23-123/rc
Peer Review File: Available at https://cdt.amegroups.com/article/view/10.21037/cdt-23-123/prf
Conflicts of Interest: Both authors have completed the ICMJE uniform disclosure form (available at https://cdt.amegroups.com/article/view/10.21037/cdt-23-123/coif). The authors have no conflicts of interest to declare.
Ethical Statement: The authors are accountable for all aspects of the work in ensuring that questions related to the accuracy or integrity of any part of the work are appropriately investigated and resolved.
Open Access Statement: This is an Open Access article distributed in accordance with the Creative Commons Attribution-NonCommercial-NoDerivs 4.0 International License (CC BY-NC-ND 4.0), which permits the non-commercial replication and distribution of the article with the strict proviso that no changes or edits are made and the original work is properly cited (including links to both the formal publication through the relevant DOI and the license). See: https://creativecommons.org/licenses/by-nc-nd/4.0/.
References
- Susin SA, Zamzami N, Castedo M, et al. Bcl-2 inhibits the mitochondrial release of an apoptogenic protease. J Exp Med 1996;184:1331-41. [Crossref] [PubMed]
- Sevrioukova IF. Apoptosis-inducing factor: structure, function, and redox regulation. Antioxid Redox Signal 2011;14:2545-79. [Crossref] [PubMed]
- Zong L, Guan J, Ealy M, et al. Mutations in apoptosis-inducing factor cause X-linked recessive auditory neuropathy spectrum disorder. J Med Genet 2015;52:523-31. [Crossref] [PubMed]
- Mekala NK, Kurdys J, Depuydt MM, et al. Apoptosis inducing factor deficiency causes retinal photoreceptor degeneration. The protective role of the redox compound methylene blue. Redox Biol 2019;20:107-17. [Crossref] [PubMed]
- Suzuki Y, Yao T, Okumura K, et al. Elevation of the vitreous body concentrations of oxidative stress-responsive apoptosis-inducing protein (ORAIP) in proliferative diabetic retinopathy. Graefes Arch Clin Exp Ophthalmol 2019;257:1519-25. [Crossref] [PubMed]
- Koliaki C, Katsilambros N. Repositioning the Role of Tumor Necrosis Factor-Related Apoptosis-Inducing Ligand (TRAIL) on the TRAIL to the Development of Diabetes Mellitus: An Update of Experimental and Clinical Evidence. Int J Mol Sci 2022;23:3225. [Crossref] [PubMed]
- Yan D, Cai Y, Luo J, et al. FOXO1 contributes to diabetic cardiomyopathy via inducing imbalanced oxidative metabolism in type 1 diabetes. J Cell Mol Med 2020;24:7850-61. [Crossref] [PubMed]
- Kostic S, Hauke T, Ghahramani N, et al. Expression pattern of apoptosis-inducing factor in the kidneys of streptozotocin-induced diabetic rats. Acta Histochem 2020;122:151655. [Crossref] [PubMed]
- Liang T, Xu X, Ye D, et al. Caspase/AIF/apoptosis pathway: a new target of puerarin for diabetes mellitus therapy. Mol Biol Rep 2019;46:4787-97. [Crossref] [PubMed]
- Li X, Zhang G, Chen Q, et al. CD317 Promotes the survival of cancer cells through apoptosis-inducing factor. J Exp Clin Cancer Res 2016;35:117. [Crossref] [PubMed]
- Lewis EM, Wilkinson AS, Jackson JS, et al. The enzymatic activity of apoptosis-inducing factor supports energy metabolism benefiting the growth and invasiveness of advanced prostate cancer cells. J Biol Chem 2012;287:43862-75. [Crossref] [PubMed]
- Kadam AA, Jubin T, Mir HA, et al. Potential role of Apoptosis Inducing Factor in evolutionarily significant eukaryote, Dictyostelium discoideum survival. Biochim Biophys Acta Gen Subj 2017;1861:2942-55. [Crossref] [PubMed]
- Zhang Y, Gliyazova NS, Li PA, et al. Phenoxythiophene sulfonamide compound B355252 protects neuronal cells against glutamate-induced excitotoxicity by attenuating mitochondrial fission and the nuclear translocation of AIF. Exp Ther Med 2021;21:221. [Crossref] [PubMed]
- Cheung EC, Melanson-Drapeau L, Cregan SP, et al. Apoptosis-inducing factor is a key factor in neuronal cell death propagated by BAX-dependent and BAX-independent mechanisms. J Neurosci 2005;25:1324-34. [Crossref] [PubMed]
- Jacobs CM, Trinh MD, Rootwelt T, et al. Dexamethasone induces cell death which may be blocked by NMDA receptor antagonists but is insensitive to Mg2+ in cerebellar granule neurons. Brain Res 2006;1070:116-23. [Crossref] [PubMed]
- Rodriguez J, Li T, Xu Y, et al. Role of apoptosis-inducing factor in perinatal hypoxic-ischemic brain injury. Neural Regen Res 2021;16:205-13. [Crossref] [PubMed]
- Li T, Li K, Zhang S, et al. Overexpression of apoptosis inducing factor aggravates hypoxic-ischemic brain injury in neonatal mice. Cell Death Dis 2020;11:77. [Crossref] [PubMed]
- Ravagnan L, Gurbuxani S, Susin SA, et al. Heat-shock protein 70 antagonizes apoptosis-inducing factor. Nat Cell Biol 2001;3:839-43. [Crossref] [PubMed]
- Matsumori Y, Hong SM, Aoyama K, et al. Hsp70 overexpression sequesters AIF and reduces neonatal hypoxic/ischemic brain injury. J Cereb Blood Flow Metab 2005;25:899-910. [Crossref] [PubMed]
- Villanueva R, Romero-Tamayo S, Laplaza R, et al. Redox- and Ligand Binding-Dependent Conformational Ensembles in the Human Apoptosis-Inducing Factor Regulate Its Pro-Life and Cell Death Functions. Antioxid Redox Signal 2019;30:2013-29. [Crossref] [PubMed]
- Bano D, Prehn JHM. Apoptosis-Inducing Factor (AIF) in Physiology and Disease: The Tale of a Repented Natural Born Killer. EBioMedicine 2018;30:29-37. [Crossref] [PubMed]
- Coughlan MT, Higgins GC, Nguyen TV, et al. Deficiency in Apoptosis-Inducing Factor Recapitulates Chronic Kidney Disease via Aberrant Mitochondrial Homeostasis. Diabetes 2016;65:1085-98. [Crossref] [PubMed]
- Sorrentino L, Cossu F, Milani M, et al. Structural bases of the altered catalytic properties of a pathogenic variant of apoptosis inducing factor. Biochem Biophys Res Commun 2017;490:1011-7. [Crossref] [PubMed]
- Hangen E, Féraud O, Lachkar S, et al. Interaction between AIF and CHCHD4 Regulates Respiratory Chain Biogenesis. Mol Cell 2015;58:1001-14. [Crossref] [PubMed]
- Hevler JF, Zenezeni Chiozzi R, Cabrera-Orefice A, et al. Molecular characterization of a complex of apoptosis-inducing factor 1 with cytochrome c oxidase of the mitochondrial respiratory chain. Proc Natl Acad Sci U S A 2021;118:e2106950118. [Crossref] [PubMed]
- Hangen E, De Zio D, Bordi M, et al. A brain-specific isoform of mitochondrial apoptosis-inducing factor: AIF2. Cell Death Differ 2010;17:1155-66. [Crossref] [PubMed]
- Rao S, Mondragón L, Pranjic B, et al. AIF-regulated oxidative phosphorylation supports lung cancer development. Cell Res 2019;29:579-91. [Crossref] [PubMed]
- Klein JA, Longo-Guess CM, Rossmann MP, et al. The harlequin mouse mutation downregulates apoptosis-inducing factor. Nature 2002;419:367-74. [Crossref] [PubMed]
- Wischhof L, Scifo E, Ehninger D, et al. AIFM1 beyond cell death: An overview of this OXPHOS-inducing factor in mitochondrial diseases. EBioMedicine 2022;83:104231. [Crossref] [PubMed]
- Delettre C, Yuste VJ, Moubarak RS, et al. Identification and characterization of AIFsh2, a mitochondrial apoptosis-inducing factor (AIF) isoform with NADH oxidase activity. J Biol Chem 2006;281:18507-18. [Crossref] [PubMed]
- Delettre C, Yuste VJ, Moubarak RS, et al. AIFsh, a novel apoptosis-inducing factor (AIF) pro-apoptotic isoform with potential pathological relevance in human cancer. J Biol Chem 2006;281:6413-27. [Crossref] [PubMed]
- Maté MJ, Ortiz-Lombardía M, Boitel B, et al. The crystal structure of the mouse apoptosis-inducing factor AIF. Nat Struct Biol 2002;9:442-6. [Crossref] [PubMed]
- Ferreira P, Villanueva R, Martínez-Júlvez M, et al. Structural insights into the coenzyme mediated monomer-dimer transition of the pro-apoptotic apoptosis inducing factor. Biochemistry 2014;53:4204-15. [Crossref] [PubMed]
- Otera H, Ohsakaya S, Nagaura Z, et al. Export of mitochondrial AIF in response to proapoptotic stimuli depends on processing at the intermembrane space. EMBO J 2005;24:1375-86. [Crossref] [PubMed]
- Joza N, Pospisilik JA, Hangen E, et al. AIF: not just an apoptosis-inducing factor. Ann N Y Acad Sci 2009;1171:2-11. [Crossref] [PubMed]
- Ding L, Li J, Li W, et al. p53- and ROS-mediated AIF pathway involved in TGEV-induced apoptosis. J Vet Med Sci 2018;80:1775-81. [Crossref] [PubMed]
- Kim JT, Kim KD, Song EY, et al. Apoptosis-inducing factor (AIF) inhibits protein synthesis by interacting with the eukaryotic translation initiation factor 3 subunit p44 (eIF3g). FEBS Lett 2006;580:6375-83. [Crossref] [PubMed]
- Seth R, Yang C, Kaushal V, et al. p53-dependent caspase-2 activation in mitochondrial release of apoptosis-inducing factor and its role in renal tubular epithelial cell injury. J Biol Chem 2005;280:31230-9. [Crossref] [PubMed]
- Resnick-Silverman L, St Clair S, Maurer M, et al. Identification of a novel class of genomic DNA-binding sites suggests a mechanism for selectivity in target gene activation by the tumor suppressor protein p53. Genes Dev 1998;12:2102-7. [Crossref] [PubMed]
- Stambolsky P, Weisz L, Shats I, et al. Regulation of AIF expression by p53. Cell Death Differ 2006;13:2140-9. [Crossref] [PubMed]
- Regdon Z, Robaszkiewicz A, Kovács K, et al. LPS protects macrophages from AIF-independent parthanatos by downregulation of PARP1 expression, induction of SOD2 expression, and a metabolic shift to aerobic glycolysis. Free Radic Biol Med 2019;131:184-96. [Crossref] [PubMed]
- Hintze M, Griesing S, Michels M, et al. Alopecia in Harlequin mutant mice is associated with reduced AIF protein levels and expression of retroviral elements. Mamm Genome 2021;32:12-29. [Crossref] [PubMed]
- Xing Y, Li Y, Hu B, et al. PAK5-mediated AIF phosphorylation inhibits its nuclear translocation and promotes breast cancer tumorigenesis. Int J Biol Sci 2021;17:1315-27. [Crossref] [PubMed]
- Polster BM, Basañez G, Etxebarria A, et al. Calpain I induces cleavage and release of apoptosis-inducing factor from isolated mitochondria. J Biol Chem 2005;280:6447-54. [Crossref] [PubMed]
- Badugu R, Garcia M, Bondada V, et al. N terminus of calpain 1 is a mitochondrial targeting sequence. J Biol Chem 2008;283:3409-17. [Crossref] [PubMed]
- Novo N, Romero-Tamayo S, Marcuello C, et al. Beyond a platform protein for the degradosome assembly: The Apoptosis-Inducing Factor as an efficient nuclease involved in chromatinolysis. PNAS Nexus 2023;2:pgac312.
- Wang X, Yang C, Chai J, et al. Mechanisms of AIF-mediated apoptotic DNA degradation in Caenorhabditis elegans. Science 2002;298:1587-92. [Crossref] [PubMed]
- Benítez-Guzmán A, Arriaga-Pizano L, Morán J, et al. Endonuclease G takes part in AIF-mediated caspase-independent apoptosis in Mycobacterium bovis-infected bovine macrophages. Vet Res 2018;49:69. [Crossref] [PubMed]
- Kim JY, Han Y, Lee JE, et al. The 70-kDa heat shock protein (Hsp70) as a therapeutic target for stroke. Expert Opin Ther Targets 2018;22:191-9. [Crossref] [PubMed]
- Candé C, Cecconi F, Dessen P, et al. Apoptosis-inducing factor (AIF): key to the conserved caspase-independent pathways of cell death? J Cell Sci 2002;115:4727-34. [Crossref] [PubMed]
- Sevrioukova IF. Redox-linked conformational dynamics in apoptosis-inducing factor. J Mol Biol 2009;390:924-38. [Crossref] [PubMed]
- Churbanova IY, Sevrioukova IF. Redox-dependent changes in molecular properties of mitochondrial apoptosis-inducing factor. J Biol Chem 2008;283:5622-31. [Crossref] [PubMed]
- Wang Y, Dawson VL, Dawson TM. Poly(ADP-ribose) signals to mitochondrial AIF: a key event in parthanatos. Exp Neurol 2009;218:193-202. [Crossref] [PubMed]
- Liu L, Li J, Ke Y, et al. The key players of parthanatos: opportunities for targeting multiple levels in the therapy of parthanatos-based pathogenesis. Cell Mol Life Sci 2022;79:60. [Crossref] [PubMed]
- Park H, Kam TI, Dawson TM, et al. Poly (ADP-ribose) (PAR)-dependent cell death in neurodegenerative diseases. Int Rev Cell Mol Biol 2020;353:1-29. [Crossref] [PubMed]
- Wang X, Ge P. Parthanatos in the pathogenesis of nervous system diseases. Neuroscience 2020;449:241-50. [Crossref] [PubMed]
- Zhou Y, Liu L, Tao S, et al. Parthanatos and its associated components: Promising therapeutic targets for cancer. Pharmacol Res 2021;163:105299. [Crossref] [PubMed]
- Huang P, Chen G, Jin W, et al. Molecular Mechanisms of Parthanatos and Its Role in Diverse Diseases. Int J Mol Sci 2022;23:7292. [Crossref] [PubMed]
- Joza N, Susin SA, Daugas E, et al. Essential role of the mitochondrial apoptosis-inducing factor in programmed cell death. Nature 2001;410:549-54. [Crossref] [PubMed]
- Vahsen N, Candé C, Brière JJ, et al. AIF deficiency compromises oxidative phosphorylation. EMBO J 2004;23:4679-89. [Crossref] [PubMed]
- Joza N, Galindo K, Pospisilik JA, et al. The molecular archaeology of a mitochondrial death effector: AIF in Drosophila. Cell Death Differ 2008;15:1009-18. [Crossref] [PubMed]
- Troulinaki K, Büttner S, Marsal Cots A, et al. WAH-1/AIF regulates mitochondrial oxidative phosphorylation in the nematode Caenorhabditis elegans. Cell Death Discov 2018;4:2. [Crossref] [PubMed]
- Cheung EC, Joza N, Steenaart NA, et al. Dissociating the dual roles of apoptosis-inducing factor in maintaining mitochondrial structure and apoptosis. EMBO J 2006;25:4061-73. [Crossref] [PubMed]
- Brown D, Yu BD, Joza N, et al. Loss of Aif function causes cell death in the mouse embryo, but the temporal progression of patterning is normal. Proc Natl Acad Sci U S A 2006;103:9918-23. [Crossref] [PubMed]
- Zong L, Zhao J, Wu W, et al. AIF knockdown induce apoptosis and mitochondrial dysfunction in cochlear spiral ganglion neurons in vitro. Mol Med Rep 2020;21:1910-20. [Crossref] [PubMed]
- Xiong Z, Guo M, Yu Y, et al. Downregulation of AIF by HIF-1 contributes to hypoxia-induced epithelial-mesenchymal transition of colon cancer. Carcinogenesis 2016;37:1079-88. [Crossref] [PubMed]
- Luo SY, Wang JQ, Liu C, et al. Hif-1α/Hsf1/Hsp70 signaling pathway regulates redox homeostasis and apoptosis in large yellow croaker (Larimichthys crocea) under environmental hypoxia. Zool Res 2021;42:746-60. [Crossref] [PubMed]
- Faubert B, DeBerardinis RJ, Minna JD. AIF: an acquired metabolic liability in lung cancer. Cell Res 2019;29:607-8. [Crossref] [PubMed]
- Yang Y, Chan L. Monogenic Diabetes: What It Teaches Us on the Common Forms of Type 1 and Type 2 Diabetes. Endocr Rev 2016;37:190-222. [Crossref] [PubMed]
- Bruder-Nascimento T, da Silva MA, Tostes RC. The involvement of aldosterone on vascular insulin resistance: implications in obesity and type 2 diabetes. Diabetol Metab Syndr 2014;6:90. [Crossref] [PubMed]
- Fang X, Miao XL, Liu JL, et al. Berberine Induces Cell Apoptosis through Cytochrome C/Apoptotic Protease-Activating Factor 1/Caspase-3 and Apoptosis Inducing Factor Pathway in Mouse Insulinoma Cells. Chin J Integr Med 2019;25:853-60. [Crossref] [PubMed]
- Murakami Y, Ikeda Y, Yonemitsu Y, et al. Inhibition of nuclear translocation of apoptosis-inducing factor is an essential mechanism of the neuroprotective activity of pigment epithelium-derived factor in a rat model of retinal degeneration. Am J Pathol 2008;173:1326-38. [Crossref] [PubMed]
- Abu El-Asrar AM, Dralands L, Missotten L, et al. Expression of antiapoptotic and proapoptotic molecules in diabetic retinas. Eye (Lond) 2007;21:238-45. [Crossref] [PubMed]
- Fu Y, Tang M, Bai L, et al. The role of apoptosis inducing factor in the apoptosis of retinal pigment epithelium cells induced by oxidative stress. Cell Mol Biol (Noisy-le-grand) 2016;62:36-41. [PubMed]
- Ghezzi D, Sevrioukova I, Invernizzi F, et al. Severe X-linked mitochondrial encephalomyopathy associated with a mutation in apoptosis-inducing factor. Am J Hum Genet 2010;86:639-49. [Crossref] [PubMed]
- Sevrioukova IF. Structure/Function Relations in AIFM1 Variants Associated with Neurodegenerative Disorders. J Mol Biol 2016;428:3650-65. [Crossref] [PubMed]
- Berger I, Ben-Neriah Z, Dor-Wolman T, et al. Early prenatal ventriculomegaly due to an AIFM1 mutation identified by linkage analysis and whole exome sequencing. Mol Genet Metab 2011;104:517-20. [Crossref] [PubMed]
- Rinaldi C, Grunseich C, Sevrioukova IF, et al. Cowchock syndrome is associated with a mutation in apoptosis-inducing factor. Am J Hum Genet 2012;91:1095-102. [Crossref] [PubMed]
- Mierzewska H, Rydzanicz M, Biegański T, et al. Spondyloepimetaphyseal dysplasia with neurodegeneration associated with AIFM1 mutation - a novel phenotype of the mitochondrial disease. Clin Genet 2017;91:30-7. [Crossref] [PubMed]
- Hu B, Wang M, Castoro R, et al. A novel missense mutation in AIFM1 results in axonal polyneuropathy and misassembly of OXPHOS complexes. Eur J Neurol 2017;24:1499-506. [Crossref] [PubMed]
- Kettwig M, Schubach M, Zimmermann FA, et al. From ventriculomegaly to severe muscular atrophy: expansion of the clinical spectrum related to mutations in AIFM1. Mitochondrion 2015;21:12-8. [Crossref] [PubMed]
- Ardissone A, Piscosquito G, Legati A, et al. A slowly progressive mitochondrial encephalomyopathy widens the spectrum of AIFM1 disorders. Neurology 2015;84:2193-5. [Crossref] [PubMed]
- Morton SU, Prabhu SP, Lidov HGW, et al. AIFM1 mutation presenting with fatal encephalomyopathy and mitochondrial disease in an infant. Cold Spring Harb Mol Case Stud 2017;3:a001560. [Crossref] [PubMed]
- Diodato D, Tasca G, Verrigni D, et al. A novel AIFM1 mutation expands the phenotype to an infantile motor neuron disease. Eur J Hum Genet 2016;24:463-6. [Crossref] [PubMed]
- Warburg O, Minami S. Versuche an Überlebendem Carcinom-gewebe. Klinische Wochenschrift 1923;2:776-7. [Crossref]
- Warburg O. Über den Stoffwechsel der Carcinomzelle. Naturwissenschaften 1924;12:1131-7. [Crossref]
- Scott AJ, Wilkinson AS, Wilkinson JC. Basal metabolic state governs AIF-dependent growth support in pancreatic cancer cells. BMC Cancer 2016;16:286. [Crossref] [PubMed]
- Vadlamudi Y, Kang SC. Silencing ESRP1 expression promotes caspase-independent cell death via nuclear translocation of AIF in colon cancer cells. Cell Signal 2022;91:110237. [Crossref] [PubMed]
- Yang J, Staples O, Thomas LW, et al. Human CHCHD4 mitochondrial proteins regulate cellular oxygen consumption rate and metabolism and provide a critical role in hypoxia signaling and tumor progression. J Clin Invest 2012;122:600-11. [Crossref] [PubMed]
- Modjtahedi N, Hangen E, Gonin P, et al. Metabolic epistasis among apoptosis-inducing factor and the mitochondrial import factor CHCHD4. Cell Cycle 2015;14:2743-7. [Crossref] [PubMed]
- Modjtahedi N, Kroemer G. CHCHD4 links AIF to the biogenesis of respiratory chain complex I. Mol Cell Oncol 2015;3:e1074332. [Crossref] [PubMed]
- Agarwal E, Chaudhuri A, Leiphrakpam PD, et al. Akt inhibitor MK-2206 promotes anti-tumor activity and cell death by modulation of AIF and Ezrin in colorectal cancer. BMC Cancer 2014;14:145. [Crossref] [PubMed]
- Urbano A, Lakshmanan U, Choo PH, et al. AIF suppresses chemical stress-induced apoptosis and maintains the transformed state of tumor cells. EMBO J 2005;24:2815-26. [Crossref] [PubMed]
- Movsas B, Chapman JD, Horwitz EM, et al. Hypoxic regions exist in human prostate carcinoma. Urology 1999;53:11-8. [Crossref] [PubMed]
- Merelli A, Repetto M, Lazarowski A, et al. Hypoxia, Oxidative Stress, and Inflammation: Three Faces of Neurodegenerative Diseases. J Alzheimers Dis 2021;82:S109-26. [Crossref] [PubMed]
- Pinthus JH, Bryskin I, Trachtenberg J, et al. Androgen induces adaptation to oxidative stress in prostate cancer: implications for treatment with radiation therapy. Neoplasia 2007;9:68-80. [Crossref] [PubMed]
- Morgan MJ, Liu ZG. Crosstalk of reactive oxygen species and NF-κB signaling. Cell Res 2011;21:103-15. [Crossref] [PubMed]
- Xu H, Zhou S, Tang Q, et al. Cholesterol metabolism: New functions and therapeutic approaches in cancer. Biochim Biophys Acta Rev Cancer 2020;1874:188394. [Crossref] [PubMed]
- Wang H, Chen T, Wan L, et al. Attenuated Salmonella engineered with an apoptosis-inducing factor (AIF) eukaryotic expressing system enhances its anti-tumor effect in melanoma in vitro and in vivo. Appl Microbiol Biotechnol 2020;104:3517-28. [Crossref] [PubMed]
- Tsai MJ, Chang WA, Tsai PH, et al. Montelukast Induces Apoptosis-Inducing Factor-Mediated Cell Death of Lung Cancer Cells. Int J Mol Sci 2017;18:1353. [Crossref] [PubMed]
- Mondal A, Roberge J, Gilleran J, et al. Bone morphogenetic protein inhibitors and mitochondria targeting agents synergistically induce apoptosis-inducing factor (AIF) caspase-independent cell death in lung cancer cells. Cell Commun Signal 2022;20:99. [Crossref] [PubMed]
- Miyake K, Bekisz J, Zhao T, et al. Apoptosis-inducing factor (AIF) is targeted in IFN-α2a-induced Bid-mediated apoptosis through Bak activation in ovarian cancer cells. Biochim Biophys Acta 2012;1823:1378-88. [Crossref] [PubMed]
- Farrand L, Kim JY, Byun S, et al. The diarylheptanoid hirsutenone sensitizes chemoresistant ovarian cancer cells to cisplatin via modulation of apoptosis-inducing factor and X-linked inhibitor of apoptosis. J Biol Chem 2014;289:1723-31. [Crossref] [PubMed]
- Won YS, Seo KI. Sanggenol L promotes apoptotic cell death in melanoma skin cancer cells through activation of caspase cascades and apoptosis-inducing factor. Food Chem Toxicol 2020;138:111221. [Crossref] [PubMed]
- Chen TH, Pan SL, Guh JH, et al. Denbinobin induces apoptosis by apoptosis-inducing factor releasing and DNA damage in human colorectal cancer HCT-116 cells. Naunyn Schmiedebergs Arch Pharmacol 2008;378:447-57. [Crossref] [PubMed]
- Chang HC, Chen ST, Chien SY, et al. Capsaicin may induce breast cancer cell death through apoptosis-inducing factor involving mitochondrial dysfunction. Hum Exp Toxicol 2011;30:1657-65. [Crossref] [PubMed]
- Kim EA, Jang JH, Lee YH, et al. Dioscin induces caspase-independent apoptosis through activation of apoptosis-inducing factor in breast cancer cells. Apoptosis 2014;19:1165-75. [Crossref] [PubMed]
- Hong SW, Jin DH, Hahm ES, et al. Ascorbate (vitamin C) induces cell death through the apoptosis-inducing factor in human breast cancer cells. Oncol Rep 2007;18:811-5. [Crossref] [PubMed]
- Lee JH, Oh EK, Cho HD, et al. Crude saponins from Platycodon grandiflorum induce apoptotic cell death in RC-58T/h/SA#4 prostate cancer cells through the activation of caspase cascades and apoptosis-inducing factor. Oncol Rep 2013;29:1421-8. [Crossref] [PubMed]
(English Language Editor: A. Kassem)