Opportunistic screening at chest computed tomography: literature review of cardiovascular significance of incidental findings
Introduction
Chest computed tomography (CT) is performed for a variety of clinical indications in the lungs, mediastinum, pleura and chest wall. In addition to providing answers to specific clinical questions, a chest CT also contains several incidental cardiovascular findings. One study showed that an incidental cardiac abnormality is seen in 78% of routine chest CT scans (1). Some of these findings are more evident than before due to advances in multidetector CT technology. These incidental cardiovascular findings are often overlooked and typically not included in the radiology report. Choy et al. showed that 22.3% of pertinent cardiac findings were not included in chest CT report including coronary artery, aortic valve, and mitral annular calcifications (1). Sverzellati et al. showed 63.2% of potentially clinically significant cardiovascular findings were unreported in routine chest CT reports at four academic centers (2). Kuetting et al. reported that only 37% of cardiac findings were reported in routine chest CTs in ICU patients (3). However, these findings have an impact on patient management and have prognostic implications.
In this narrative review, we aim to summarize the current evidence-based literature on the clinical significance of these incidental cardiovascular abnormalities in a routine chest CT. This article provides a unique, comprehensive discussion and comparison of the current available data regarding incidental cardiovascular findings with their prognostic and clinical significance. With the increased emphasis placed on the quality and value of radiologist’ report over quantity, it is important to understand the clinical significance of these findings to improve the healthcare and outcomes of patients. We present this article in accordance with the Narrative Review reporting checklist (available at https://cdt.amegroups.com/article/view/10.21037/cdt-23-79/rc).
Methods
PubMed and Web of Science databases were searched for this narrative review from origin to February 2023. The search strategy is summarized in Table 1. Original research, review articles and guidelines/expert consensus in English that involved an adult population were included in this review.
Table 1
Items | Specification |
---|---|
Date of search | 02/03/2023 |
Database(s) | PubMed, Web of Science |
Timeframe | No time limit |
Search terms used | (Chest computed tomography) AND (incidental) AND (cardiac OR cardiovascular) |
Inclusion criteria | Original articles, reviews, guidelines and expert consensus about incidental cardiovascular findings on non-gated chest CT; articles in English; articles with adult cohort |
Exclusion criteria | No incidental cardiovascular findings; findings on CTs other than non-gated chest CT; articles not in English |
Discussion
Coronary artery calcification (CAC)
CAC is a well-known indicator of coronary artery disease (CAD) and an important prognostic marker as shown by numerous studies (4-8). CAC is typically evaluated using a dedicated prospectively ECG-triggered cardiac CT, from which the calcium is quantified (9,10). In addition to the total CAC score, other parameters including the number of involved vessels, location and patterns of calcification have also been shown to have prognostic value. Worse prognosis has been reported with higher burden of calcification, higher number of calcific foci, calcification in the left main coronary artery, and spotty pattern of calcification (11,12).
CAC can be detected in up to 53% of the patients without a history of CAD in CT scan for noncardiac indications (13). Incidental CAC in a routine chest CT can be visually categorized as mild (isolated flecks of calcification), moderate (between mild and severe), and severe (continuous calcification) (14) (Figure 1). An ordinal scoring has also been described with a score of 1 for CAC involving less than 1/3rd of the length of a coronary artery, score of 2 for CAC involving 1/3–2/3rd of the artery and a score of 3 for CAC involving more than 2/3rd of the artery. The individual vessel scores are added, which provides 3 categories of increasing severity: 0, 1–3, and 4–12 (14,15). Additionally, segment involvement score (SIS) can be used for scoring of CAC. It is based on assigning 1 to each coronary artery segment with coronary calcification (16). Multiple studies have shown high concordance between CAC score in a routine chest CT and ECG-gated cardiac CT (17-19). The sensitivity and specificity of non-gated routine chest CT for CAC score were reported as 96.8% and 100% respectively (19).
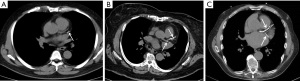
CAC score on a routine chest CT is not only associated with CAD, but also with other cardiovascular risk factors like type 2 diabetes mellitus (DM), dyslipidemia, hypertension, and Framingham Risk score (FRS) (20,21). CAC score in a routine chest CT has prognostic value and can predict major adverse cardiovascular events (MACE) (22-25). The ordinal CAC score was found to be associated with increased CV deaths with an estimated hazard ratio (HR) of 1.21 (26). CAC score has hazard ratios of 1.54 and 1.41 for coronary and cardiovascular events respectively, even after adjusting for age, sex, clinical indication, image quality and type of medical center (27). The incidence of any cardiovascular event increases by 25% for every 1 standard deviation (SD) increase in the CAC score and incidence of any coronary event increases by 42% for every 1 SD increase (27). Visible CAC on chest CT in patients without known CAD is associated with an increased rate of MACE (HR =6.0) at median follow-up of 3.5 years (28). Similarly, increase in CAC is strongly associated with increased risk of cardiac death (15,24,29). Hughes et al. reported that the odds ratio (OR) for death increased by 50% for each SD increase in calcium score detected by routine chest CT, after adjustment of other traditional cardiovascular risk factors (24). In a large study of 4,953 scans, CAC on routine chest CT was found to be associated with increased myocardial infarction and revascularization (24).
Low-dose CT (LDCT) examinations for lung cancer screening in smokers provides an opportunity to additionally screen for CAC in this cohort which has a higher risk of CAD as well (15,30). A meta-analysis of four randomized controlled trials of LDCT demonstrated that cardiovascular events are strongly associated with the presence of CAC, regardless of sex with a relative risk of 2.85. Also, higher CAC score is associated with increased cardiovascular events with a relative risk of 3.47 (30). CAC can also be detected on contrast-enhanced chest CT studies, although visual evaluation underestimates calcium due to high attenuation of contrast media. Contrast-enhanced chest CT has a sensitivity of 83% and specificity of 100% for the detection of CAC (31). In a study of 116 patients, only 13 contrast-enhanced studies were mislabeled as negative CAC, with all these patients having Agatston score of <30 (31). CAC on contrast-enhanced CT was associated with MACE with a hazard ratio of 4.5 (31). CAC detected on CT pulmonary angiography has been shown to be an independent predictor of short-term adverse outcomes in patients without known cardiac disease (32).
The 2016 SCCT/STR guidelines recommended the routine interpretation of the presence and severity (mild, moderate, severe) of CAC in all routine chest CT scans irrespective of the indications of the scans (33). Moreover, the recent expert consensus document of SCCT recommends reporting CAC Data and Reporting System (CAC-DRS) category based on either Agatston or visual scoring of CAC on all non-gated non-contrast chest CT scans regardless of the indication (34).
Aortic valve calcification (AVC)
AVC is a sequela of fibrocalcific changes of the valve leaflets. Significant AVC can lead to valvular stenosis. AVC could be qualitatively graded as (I) mild—separate, small, calcified foci; (II) moderate—multiple, larger calcified foci; or (III) severe—extensive, confluent calcification (Figure 2). AVC could also be quantified using Agatston scoring technique, which has excellent agreement with the qualitative score (35-37). In clinical practice, dedicated AVC score is used to quantify the degree of aortic stenosis in patients with discordant echocardiographic findings, especially with low flow, low gradient aortic stenosis (38). In such patients, the aortic valve area and gradients (mean and peak) correlate with AVC scores. Sex-specific thresholds have been established for severity of aortic stenosis, i.e., ≥1,274 AU in women and ≥2,065 AU in men (39-42).
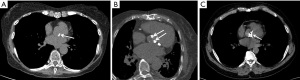
AVC is also a marker of atherosclerosis that can be easily identified in a routine chest CT. AVC is associated with other CVD risk factors including diabetes, hypertension, obesity, and metabolic syndrome, even after adjustment for age and gender (43,44). AVC also correlates with CAD and is a marker of subclinical CAD. A meta-analysis of 13 studies showed that AVC is associated with a higher risk of CAD than controls (45). AVC is associated with higher prevalence and severity of CAD, with one study showing that AVC is associated with a CAC score of 441±802, whereas patients without AVC had a lower CAC of 265±566 (44). Another study showed that the prevalence ratio of AVC increased from 1.83 in patients with mild CAC (<100) to 3.36 in patients with higher CAC (>400) (46).
A study of 10,410 patients showed that AVC is an independent predictor of MACE, independent of the severity of CAD (47). The HR of MACE was 2.03 in the presence of calcification of two or three leaflets compared to no calcification (47). Owens et al. showed that AVC is predictive of cardiovascular events (HR =1.5), coronary events (HR =1.72) and cardiovascular mortality (HR =2.5), after adjusting for cardiovascular risk factors and inflammatory markers (48). The negative predictive value of AVC for overall mortality is 98.8 % (45).
Mitral annular calcification (MAC)
MAC could be diffuse or localized to the anterior or posterior annulus. It can occasionally resemble a mass or may present with milk of calcium, which has a central lower attenuation than the periphery (49). MAC is commonly encountered on non-gated chest CTs with an approximate 8% prevalence (50). Visual or quantitative assessment of MAC has been proposed on dedicated cardiac CT studies. Visual scoring classifies MAC into three groups based on circumferential involvement: (I) mild— < one third of the annulus involved; (II) moderate-between one third and half of the annulus involved; and (III) severe— > half of the mitral annulus involved (51) (Figure 3). Another visual scoring system of MAC included circumferential involvement of mitral annulus as well as calcium thickness and distribution/extent, involvement of trigone and valve leaflets (52). Quantification of MAC can also be done on non-contrast cardiac CT like Agatston CAC scoring (51). To our knowledge, there is no study in the literature investigating the feasibility of using similar scorings systems on non-gated chest CTs.
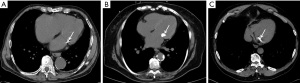
MAC is an atherosclerotic marker as it is an independent predictor of the presence and severity of CAD (53-55). MAC scores correlate with CAC scores, even after adjusting for traditional cardiovascular risk factors and inflammatory markers. As the CAC score increased from 100–399 to ≥400, the relative risk (RR) of MAC increased from 2.09 to 2.58 (55). MAC is also associated with AVC and thoracic aortic calcifications (TAC) (56). Additionally, the combined presence of AVC and MAC was found to be associated with the presence (OR =9.36), extent (beta-estimate of 1.86) and vulnerable characteristics of coronary plaques (OR =4.87). Hence, they can be seen as markers of CAD disease severity (57).
MAC has been shown to predict adverse cardiovascular events. Patients with MAC had a hazard ratio of 1.53 for cardiovascular events (47). Similarly, MAC was associated with an increased risk of myocardial infarction (MI) (HR =1.75) and vascular death (HR =1.53), after adjusting for other cardiovascular risk factors. The impact of the MAC was shown to be related to the thickness, with a MAC of >4 mm being a strong independent predictor of MI and ventricular dysfunction (58). The risk of cardiovascular event also increases with the number of mitral leaflets affected, with one-leaflet involvement having 1.83-fold higher risk and two leaflet involvement having 2.08-fold higher risk than no mitral calcifications (47). A recent meta-analysis of 26 studies including 35,070 subjects reported that MAC is associated with higher all-cause death and cardiovascular mortality (59).
In contrast to these studies, Bhatt et al. did not find significant association between MAC and moderate or severe coronary artery stenosis and number of obstructive vessels (60). Although it might not provide independent and incremental information as much as the CAC, MAC still provides significant information for cardiovascular risk assessment and valvular interventions. Therefore, reporting of the presence and severity (mild, moderate, severe) of the MAC was recommended by SCCT in CAC-DRS (34).
Thoracic aortic calcification (TAC)
Atherosclerotic TAC is more commonly seen in the aortic arch and descending thoracic aorta than the ascending aorta. TAC is a marker of CAD and is significantly associated with CAC (23,61). Like CAC, TAC can be classified visually or quantified using Agatston method (62). One visual scoring system classifies TAC into three groups: (I) mild—≤3 focal calcifications; (II) moderate—4–5 focal calcifications or one calcification extending over more than 3 slices of 5 mm thickness); and (III) severe—>5 focal calcifications or >1 calcification extending more than 3 slices (22) (Figure 4).
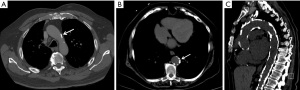
Along with CAC, increasing TAC risk categories detected on non-gated chest CTs were associated with cardiovascular events and all-cause mortality, although CAC is a stronger predictor than TAC (HR for all-cause mortality 9.13 vs. 5.45; HR for cardiovascular events 4.46 vs. 2.25) (23). The association between TAC and cardiovascular events is higher for descending aortic (HR =1.49) and arch (HR =1.43) calcifications than ascending aorta calcifications (HR =1.26) (26). Descending aortic calcifications on routine chest CT have been shown to be an indicator of cardiovascular risk (HR =1.45) and included in a CT-based prediction model (23). Additionally, TAC is an independent risk factor for all-cause mortality with HR of 1.61 (63). A recent analysis of CAC Consortium data by Han et al. showed that the severity of TAC was important for cardiovascular risk assessment. TAC score >300 (by Agatston method) was strongly associated with higher cardiovascular events compared to patients with TAC <300 even after adjusting for clinical risk factors and CAC (HR =4.72) (62). A study of 1,164 patients showed that risk scores based on TACs, plaques, irregularities, and elongation were all predictive of MACE complementing other established risk scores (64).
A few other studies reported contradictory results. The MESA and Framingham Heart Study (FHS) showed no significant correlation between TAC and cardiac events, independent of CAC (65,66). Similarly, results of Heinz Nixdorf Recall (HNR) and Early Identification of Subclinical Atherosclerosis by Noninvasive Imaging Research (EISNER) studies showed that TAC was not a predictor of cardiac events after adjustment of CAC and FHS (67,68). Despite limited prognostic role of TAC beyond CAC, the reporting of TAC on all non-contrast chest CT and calcium scoring scans even without CAC is recommended by 2016 SCCT/STR guideline (Class IIb recommendation) and subsequent Coronary Artery Calcium Data and Reporting System, respectively (33,34).
Epicardial fat
The fat (adipose) tissue surrounding the heart is categorized into two types: epicardial adipose tissue (EAT), which is situated between the myocardium and visceral pericardial layer, and paracardiac adipose tissue (PAT), which is located outside the parietal pericardium (69) (Figure 5). The part of EAT that surrounds the coronary arteries is called as peri-coronary adipose tissue (PCAT). Additionally, small deposits of fat surrounding the thoracic aorta represents peri-aortic fat (70). Total thoracic adipose tissue (TAT) includes both epicardial and paracardial adipose tissue. Finally, subcutaneous adipose tissue (SAT) represents the fat volume outside of the sternum and vertebra. Epicardial fat has been shown to be metabolically active and produces inflammatory mediators such as cytokines that can result in coronary arterial inflammation and atherosclerosis, since there is no fascial barrier between these structures (70-73). Hence epicardial fat has become an important marker of coronary atherosclerosis.
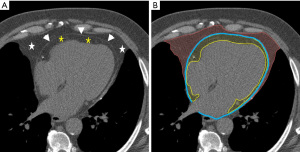
EAT correlates with whole body fat mass, abdominal adiposity, waist circumference, insulin resistance and triglyceridemia (73). EAT correlates with atherosclerosis including CAD, CAC score, non-calcific plaque, coronary artery stenosis grade, and carotid intima-media thickness (74-78). In women, greater amounts of epicardial adipose tissue (EAT) have been correlated with a decrease in coronary flow reserve (79). Increased EAT has been found to be connected to increased risk of coronary atherosclerosis and cardiovascular disease in individuals with HIV infection who are undergoing antiretroviral therapy (ART), as well as in patients with rheumatologic disorders (79).
Higher pericardial fat volume (combination of epicardial and paracardial fat) (Figure 6) was associated with poorer CVD prognosis, hard atherosclerotic CVD (ASCVD), HF and adverse LV remodeling. This association was not dependent of hepatic fat severity, inflammation, or insulin resistance (80). Epicardial fat volume (EFV) is a strong predictor of myocardial ischemia, has an independent role in predicting CAD and is associated with MACE, even after adjustment for FRS, CAC score, and BMI (81-83). In women, the risk of HF was found to be higher in association with an increase in pericardial fat compared to men (HR: 1.44 vs. 1.13) (84). A recent meta-analysis demonstrated that EFV was connected with obstructive coronary stenosis (OR =1.055), significant coronary stenosis (OR =1.514), myocardial ischemia (OR =1.062), and MACE (HR =1.040). However, the association with CAC was only marginally significant (OR =1.007). Furthermore, EFV was identified as an independent predictor of non-calcified obstructive or vulnerable coronary plaques in patients with low-to-intermediate-risk (85).
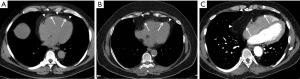
Epicardial fat is typically quantified using dedicated software algorithms from an ECG-gated cardiac CT. Although no visual scoring of EAT is present, manual measurement of EAT thickness on imaging has been attempted to avoid additional software use. However, EAT volumetry is calculated by dedicated software correlates better with CAD extent than manual bidimensional measurements of EAT thickness and is more reproducible (86). Similar quantification of EAT in a routine chest CT has excellent correlation with ECG-gated cardiac CT. This EAT from a routine chest CT has equivalent CAD predictive value as an ECG-gated CCTA (87). Along with CAC, extent of pericardial fat from routine chest CT is related to worse survival and is an independent predictor of all-cause death above and beyond age, sex, and CT determined hepatic fat, abdominal subcutaneous adipose tissue, aortic or valve calcification (25).
TAT has also been shown to be associated with CAC (88,89). Both TAT and SAT were demonstrated associations with the presence and severity of CAC and identified as an independent predictor, even after accounting for factors such as age, gender, BMI, and cardiovascular risk. In comparison to PAT and SAT, EAT showed a stronger correlation with the presence and severity of CAC, as well as with the FRS (88). Thoracic fat has been reported to be associated with metabolic syndrome (OR =5.7) (78). Periaortic fat is independently associated with CAC and increased prevalence of cardiovascular disease regardless of visceral adipose tissue volume (90). Besides, an association between periaortic adipose tissue and peripheral arterial disease has been observed (91).
Myocardial calcification and fat
Following MI, ventricular remodeling can result in myocardial deposition of calcium or fat. Myocardial calcification, especially in thinned myocardium indicates chronic MI, seen in 8% of such patients (92) (Figure 7). Calcification can also be seen as sequela of previous surgery or trauma. Myocardial fatty metaplasia can be seen in 60% of healed MI (93). This fat has mean attenuation between −10 and −20 HU, which is higher than that of pericardial fat, which typically has an attenuation of −100 HU (94) (Figure 8). CT has high accuracy in detecting myocardial fat and has excellent agreement with myocardial perfusion imaging in detecting chronic MI (95,96). Myocardial fat can also be seen in other abnormalities such as tuberous sclerosis, lipoma, dilated cardiomyopathy, and muscular dystrophy and occasionally without any specific etiology (93). Compared to non-MI controls, patients with MI had significantly higher prevalence of left ventricle (LV) myocardial fat as observed on CT. In the MI group the percentage of individuals with LV fat deposition was 62%, whereas in control group, it was only 3% (96). In a small study of MI patients with LV fat deposition on CT, the fat deposition was located in the segments supplied by the infarct-related artery, predominantly involving the subendocardium (94%) (96). Batal et al. found that 10% of patients with prior MI have subendocardial fat deposition on chest CT (97). Furthermore, the mean duration since the occurrence of the infarct was significantly higher in patients with LV fat deposition (8.2±4.4 years) compared to those without (2.2±2.6 years) (96). In patients with MI older than 3 years, increased percentage of fat was seen in infarcted LV at all 3 levels (base, midventricular, apical) (98). Adipose tissue in MI also increases with age, in males, and in those with CABG surgery (99).

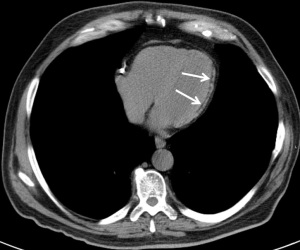
The clinical significance of incidental detected MI is that such unrecognized MI accounts for as much as one-fourth of all MI and carries a similar prognosis as that of recognized MI (100,101). Fatty metaplasia may be the only indicator of a silent MI and hence it important to recognize this abnormality (102). Direct evidence on the link between myocardial fat and prognosis is uncertain. Bader et al. showed that myocardial fat in non-contrast chest CTs was associated with improved survival in patients regardless of history of MI (103), whereas Hata et al. demonstrated its association with accelerated LV dysfunction (104). However, Batal et al. did not find any significant association between myocardial fat and LV ejection fraction or survival (97). Another study showed that fatty metaplasia is more often seen in older infarcts, in whom cardiac risk factors and medications did not have a significant influence (105).
Left ventricular size
Enlargement of LV could be seen in ischemic heart disease, nonischemic cardiomyopathies or valvular dysfunction. Since these diseases could be clinically silent, incidental detection of LV enlargement in routine chest CT might be the only indicator leading to further investigation and diagnosis of the underlying pathology. Although, cardiac MRI is the gold standard for quantification of LV size and function (106), a dedicated retrospective-gated cardiac CT could also be useful to detect LV enlargement with reasonable accuracy compared to MRI (107,108). Routine chest CT is also a reliable technique to detect LV enlargement with a high specificity (ranges 88–100%) and negative predictive values (range, 91–93%) (109-111) (Figure 9). LV is considered enlarged if the transverse diameter of LV on axial chest CT images is ≥55 mm in women and ≥60 mm in men (112). Due to the known correlation between LV enlargement and cardiovascular events, this information is very valuable from a chest CT.
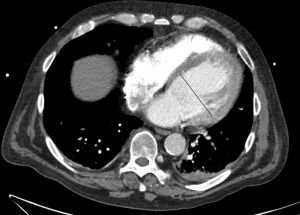
Hepatic fat
Hepatic fat or steatosis (HS) is seen in CT as diffuse hypoattenuation of the liver parenchyma of <40 HU or the ratio of liver/spleen attenuation of <1 (Figure 10). In severe steatosis, the attenuation of liver is lower than that of intrahepatic blood vessels (113). Causes of HS include obesity, dyslipidemia, diabetes, and metabolic syndrome (114). Nonalcoholic fatty liver disease (NAFLD) is linked to metabolic syndrome (73). HS by CT has been shown to be an independent predictor for the development of type 2 DM (115). Patients with HS also had higher values for BMI, visceral fat, diastolic blood pressure, and triglycerides concentration (116). HS is associated with markers of atherosclerosis including carotid intima medial thickness (CIMT), carotid plaque burden and CAC. Patients with HS had 20% greater CIMT than those without HS. Furthermore, NAFLD showed a notable elevation in CIMT when compared to matched (age, sex and BMI) healthy controls. Additionally, individuals with NASH exhibited even higher CIMT compared to those with simple steatosis (117). Prevalence of CAC was higher in HS (52%) than those without it (37%) (118).
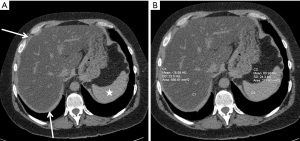
HS was independently associated with CAC, even after adjusting for CAD risk factors and liver enzymes. The presence of HS was also linked to a higher prevalence of atherosclerotic risk factors as well as an elevated 10-year total CHD risk based on FRS (118). In diabetic patients, increased hepatic fat has been shown to be associated with a higher risk of CAD (119). Hepatic fat is associated with increased cardiovascular disease risk independent of other prognostic risk factors (120). Additionally, NFALD was a predictor of cardiovascular disease independent of conventional risk factors (OR =4.12) (121). However, another study found that HS is associated with higher prevalence of cardiovascular risk factors, but it is not significantly associated with poor cardiovascular outcome/prognosis (80).
Bone density
Osteoporosis is characterized by the decreased bone density with increased risk of bone fractures. Dual energy X-ray absorptiometry (DEXA) of lumbar spine and femoral necks is the most commonly used and reliable method of measuring bone mineral density (BMD) and detecting osteoporosis (122). Osteoporosis can also be diagnosed from a chest CT when there is osteopenia with coarse and prominent vertical trabeculations in the vertebra. This has important implication since the risk of fracture can be potentially decreased by early treatment of osteoporosis. Combining sagittal reconstruction of the thoracic vertebrae from a standard chest CT image with spinal CT attenuation proves to be valuable in predicting bone mineral density (BMD) in populations at high risk (Figure 11). BMD is quantified by placing a region of interest in the vertebral body and is expressed in Hounsfield unit. It can be calculated from either thoracic or lumbar vertebra which correlates closely independent of age, sex, and race (123). In a particular study, it was observed that the mean BMDs in the thoracic spine were found to be 20.7% higher for female subjects and 17.0% higher for male subjects compared to the lumbar spine (124). A CT attenuation of >141 HU at T10-L3 vertebral bodies demonstrated a sensitivity of 93.5% and specificity of 86.1% in detecting normal trabecular BMD. Conversely, a threshold of <102.4 HU showed a specificity of 96.9% and sensitivity of 82.1% in differentiating osteoporosis from osteopenia, and normal BMD. The CT attenuation values were lower for vertebrae with compression than normal morphology (108.9±20.6 vs. 136.8±32.2 HU) (125).
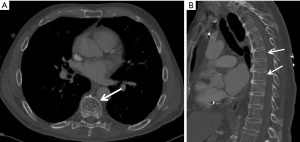
Osteoporosis has been shown to correlate with cardiovascular risk factors and cardiovascular diseases (126,127). Osteoporosis on CT correlates with hypertension, abnormal lipids, atherosclerosis, vascular calcification, and congestive heart failure. CT-derived volumetric BMD is inversely associated with CAC (P=0.05) (128). The inverse association of BMD with CAC is stronger in women without dyslipidemia (129). BMD has been inversely associated with subclinical and clinical CVD and MACE, even after adjusting for potential confounding factors (130). Furthermore, Tankó et al. showed that postmenopausal women with osteoporosis are at an increased risk for cardiovascular events (3.9-fold risk), that is proportional to the severity of osteoporosis (131).
Breast artery calcification
Breast arterial calcification (BAC) is a result of calcium deposition in the media of the arteries. Although this calcium deposition occurs in a different layer than the intimal deposition of CAC, both these calcifications increase the arterial wall stiffness and associated with higher morbidity and mortality (132-135). BAC has been shown to correlate with the risk of CAD and the actual CAC score (136) (Figure 12). A study of 2,100 asymptomatic patients showed that the presence and severity of BAC is a strong indicator of coronary artery plaque and CAC score with odds ratio of 3.02 and 3.54 respectively (127). A meta-analysis of 10 studies with 3,952 patients demonstrated the association of BAC with higher risk of CAD with an odds ratio of 3.86 (137). A more recent systematic review and meta-analysis of 31 studies in 35,583 patients demonstrated that BAC is associated with CAD (OR =2.61) as well as diabetes mellitus (OR =2.17) and hypertension (OR =1.80) (138). BAC is potentially a stronger predictor of CAD than the traditional risk factors such as family history, hypertension, and hyperlipidemia (137,139,140). A meta-analysis of 19 studies with 33,583 women showed that BAC did not only have high specificity for the presence of CAD (84.5–93%), but it also had high specificity for CAD morbidity and mortality (88–97%) (141). BAC is also associated with aortic calcification (142).
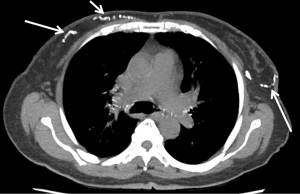
Note that most of the abovementioned studies used mammography for evaluation of BAC. A CT scan study also showed a similar significant association between BAC and both CVD and all-cause mortality independent of the other cardiovascular risk factors (all cause HR =5.67; 95% CI, 1.50–21.41) (143). This association was not seen with splenic and iliac arterial calcification (143). Since BAC is a strong predictor of CAC, CAD and CAD mortality, some authors indicate that BAC should be reported in chest CT (144).
Previous studies on chest CT
Multiple studies have demonstrated that reporting the abovementioned incidental cardiac findings helps to establish the diagnosis, alter management, and provide prognostic information (22,27,47,145,146). Mendoza et al. showed that detection of CAC on non-gated chest CT resulted in a change in management in 20.5% of the patients, which was more common in patients without an established diagnosis of CAD compared to those with CAD. The most common change was alteration in the medication regimen (73.5%). 2.6% of the patients needed percutaneous coronary intervention while 1.9% had surgery (145).
Additionally, a few dedicated studies have evaluated the cardiovascular prognostic significance of chest CT findings. A meta-analysis on 34,028 asymptomatic subjects showed higher cardiovascular risk in those with CAC in a routine chest CT. On a follow up of 45 months, 160 cardiovascular deaths (2.5%) and 570 cardiovascular events (4.5%) were seen in those with positive CAC, whereas only 47 cardiovascular deaths (0.55%) and 72 cardiovascular events (1.3%) were seen in those with negative CAC (147). Jacobs et al. showed that both CAC and TAC detected on routine chest CT were significantly and independently associated with increased risk of CVD events (27). Presence of severe CAC and TAC are associated with 3.7 (95% CI, 2.7–5.2) and 2.7 times (95% CI, 2.0–3.7) higher risk of CVD event respectively compared to patients with no calcium (27).
Gondrie et al. showed that incidentally detected AVC, MVC and MAC are independent predictors of CVD events (47). Severe AVC, MVC and MAC were associated with 2.03 (1.48–2.78), 2.08 (1.04–4.19) and 1.53 (1.13–2.08) increased risks of future CVD events respectively in comparison to patients with no calcification (47). Another study on smokers with lung cancer screening chest CT showed that CAC volume (1.45) and AVC volume (1.1) were predictive of 3-year cardiovascular events, along with cardiovascular history (1.89) and smoking history >10 years (1.24). A secondary model that did not include CV history also showed a significant C-statistic of 0.73 (147). A CT-based prediction model for detection of patients at risk for CVD including patient’s age, sex, left ascending artery and mitral valve calcification, cardiac size and descending aorta calcification was developed by Jairam et al. This model allowed accurate risk stratification and identification of high-risk patients (C statistic of 0.71) (22).
The importance of these incidental findings has now been recognized and incorporated into guideline documents endorsed by several different societies. SCCT/STR 2016 guidelines for calcium scoring and subsequent Coronary Artery Calcium Data and Reporting System recommends reporting calcium scoring, mitral annulus calcification, TAC in all routine non-gated chest CT scans (33,34). Similarly, a consensus statement from the British Society of Cardiovascular Imaging/British Society of Cardiac Computed Tomography (BSCI/BSCCT) and British Society of Thoracic Imaging (BSTI) recommends reporting of incidental coronary artery, aortic valve, mitral, myocardial and pericardial calcifications in conjunction with further management recommendations (148). Finally, American College of Radiology recommends reporting of incidental CAC on routine chest CT scans by using either Agatston or visual scoring methods (149).
Future directions
Advances in artificial intelligence (AI) brings the opportunity of using AI models for opportunistic screening in routine non-gated chest CTs. Deep learning convolutional neuronal networks (CNN) can evaluate multiple parameters at rapid pace without involvement of humans at comparable accuracies (150). New AI models provides automated quantification of calcium scores from chest CTs which has a good correlation with manual calcium scoring (151). A study of 14,959 patients with low-dose chest CT on the NLST database showed that automated score obtained from low-dose chest CT is a strong predictor of cardiovascular events, and demonstrates high correlation with manual quantification (152) Similarly, a deep learning algorithm for automated coronary and thoracic aortic calcium scoring on various types thoracic CTs that included the heart (CAC scoring CT, routine chest CT, PET-CT, radiation therapy treatment planning CT, and low-dose chest CT) showed interclass agreement (ICC) of 0.79–0.97 for CAC and 0.66–0.98 for TAC across the range of different types of CT examinations. Despite the variations in scan protocols and types, the automated scoring of CAC and TAC by deep learning algorithm were found to be robust (153).
Strengths and limitations
The main strength of this narrative review is providing a comprehensive analysis of clinical and prognostic implications of incidental cardiovascular abnormalities detected on non-gated routine chest CT scans. Additionally, this review incorporates a substantial number of studies which allows more diverse range of evidence, increases robustness of the findings, and strengthens the overall conclusions drawn from the review.
This review also has some limitations. As for any systematic review, there is a possibility of selection bias since the studies included in this review were selected based on certain criteria. Additionally, as studies with nonsignificant findings are prone not to be published, a publication bias may also exist. Besides, included studies may vary significantly in terms of study population, study design, and methodology resulting in significant heterogeneity within the data which potentially may impact the overall strength of the conclusions.
Conclusions
A routine chest CT has valuable information on cardiovascular disease, which is currently underutilized. There are several incidental findings that might help with diagnosis, cardiovascular risk stratification, appropriate referral and subsequent management. It is important for the chest radiologist to be cognizant of the clinical significance of incidentally encountered cardiovascular findings in chest CT.
Acknowledgments
Funding: None.
Footnote
Reporting Checklist: The authors have completed the Narrative Review reporting checklist. Available at https://cdt.amegroups.com/article/view/10.21037/cdt-23-79/rc
Peer Review File: Available at https://cdt.amegroups.com/article/view/10.21037/cdt-23-79/prf
Conflicts of Interest: All authors have completed the ICMJE uniform disclosure form (available at https://cdt.amegroups.com/article/view/10.21037/cdt-23-79/coif). AC and PSR report that they received royalties from Elsevier, unrelated to the content of this manuscript. PSR serves as an unpaid editorial board member of Cardiovascular Diagnosis and Therapy from September 2021 to August 2023. The other authors have no conflicts of interest to declare.
Ethical Statement: The authors are accountable for all aspects of the work in ensuring that questions related to the accuracy or integrity of any part of the work are appropriately investigated and resolved.
Open Access Statement: This is an Open Access article distributed in accordance with the Creative Commons Attribution-NonCommercial-NoDerivs 4.0 International License (CC BY-NC-ND 4.0), which permits the non-commercial replication and distribution of the article with the strict proviso that no changes or edits are made and the original work is properly cited (including links to both the formal publication through the relevant DOI and the license). See: https://creativecommons.org/licenses/by-nc-nd/4.0/.
References
- Choy G, Kröpil P, Scherer A, et al. Pertinent reportable incidental cardiac findings on chest CT without electrocardiography gating: review of 268 consecutive cases. Acta Radiol 2013;54:396-400. [Crossref] [PubMed]
- Sverzellati N, Arcadi T, Salvolini L, et al. Under-reporting of cardiovascular findings on chest CT. Radiol Med 2016;121:190-9. [Crossref] [PubMed]
- Kuetting D, Müller A, Feisst A, et al. Incidental Cardiac Findings in Non-Electrocardiogram-gated Thoracic Computed Tomography of Intensive Care Unit Patients: Assessment of Prevalence and Underreporting. J Thorac Imaging 2018;33:168-75. [Crossref] [PubMed]
- Obisesan OH, Osei AD, Uddin SMI, et al. An Update on Coronary Artery Calcium Interpretation at Chest and Cardiac CT. Radiol Cardiothorac Imaging 2021;3:e200484. [Crossref] [PubMed]
- LaMonte MJ, FitzGerald SJ, Church TS, et al. Coronary artery calcium score and coronary heart disease events in a large cohort of asymptomatic men and women. Am J Epidemiol 2005;162:421-9. [Crossref] [PubMed]
- Polonsky TS, McClelland RL, Jorgensen NW, et al. Coronary artery calcium score and risk classification for coronary heart disease prediction. JAMA 2010;303:1610-6. [Crossref] [PubMed]
- Budoff MJ, Young R, Burke G, et al. Ten-year association of coronary artery calcium with atherosclerotic cardiovascular disease (ASCVD) events: the multi-ethnic study of atherosclerosis (MESA). Eur Heart J 2018;39:2401-8. [Crossref] [PubMed]
- Greenland P, Blaha MJ, Budoff MJ, et al. Coronary Calcium Score and Cardiovascular Risk. J Am Coll Cardiol 2018;72:434-47. [Crossref] [PubMed]
- Agatston AS, Janowitz WR, Hildner FJ, et al. Quantification of coronary artery calcium using ultrafast computed tomography. J Am Coll Cardiol 1990;15:827-32. [Crossref] [PubMed]
- Alluri K, Joshi PH, Henry TS, et al. Scoring of coronary artery calcium scans: history, assumptions, current limitations, and future directions. Atherosclerosis 2015;239:109-17. [Crossref] [PubMed]
- Williams M, Shaw LJ, Raggi P, et al. Prognostic value of number and site of calcified coronary lesions compared with the total score. JACC Cardiovasc Imaging 2008;1:61-9. [Crossref] [PubMed]
- Ehara S, Kobayashi Y, Yoshiyama M, et al. Spotty calcification typifies the culprit plaque in patients with acute myocardial infarction: an intravascular ultrasound study. Circulation 2004;110:3424-9. [Crossref] [PubMed]
- Uretsky S, Chokshi N, Kobrinski T, et al. The interplay of physician awareness and reporting of incidentally found coronary artery calcium on the clinical management of patients who underwent noncontrast chest computed tomography. Am J Cardiol 2015;115:1513-7. [Crossref] [PubMed]
- Chiles C, Duan F, Gladish GW, et al. Association of Coronary Artery Calcification and Mortality in the National Lung Screening Trial: A Comparison of Three Scoring Methods. Radiology 2015;276:82-90. [Crossref] [PubMed]
- Shemesh J, Henschke CI, Shaham D, et al. Ordinal scoring of coronary artery calcifications on low-dose CT scans of the chest is predictive of death from cardiovascular disease. Radiology 2010;257:541-8. [Crossref] [PubMed]
- Ayoub C, Erthal F, Abdelsalam MA, et al. Prognostic value of segment involvement score compared to other measures of coronary atherosclerosis by computed tomography: A systematic review and meta-analysis. J Cardiovasc Comput Tomogr 2017;11:258-67. [Crossref] [PubMed]
- Kim YK, Sung YM, Cho SH, et al. Reliability analysis of visual ranking of coronary artery calcification on low-dose CT of the thorax for lung cancer screening: comparison with ECG-gated calcium scoring CT. Int J Cardiovasc Imaging 2014;30:81-7. [Crossref] [PubMed]
- Hutt A, Duhamel A, Deken V, et al. Coronary calcium screening with dual-source CT: reliability of ungated, high-pitch chest CT in comparison with dedicated calcium-scoring CT. Eur Radiol 2016;26:1521-8. [Crossref] [PubMed]
- Chen Y, Hu Z, Li M, et al. Comparison of Nongated Chest CT and Dedicated Calcium Scoring CT for Coronary Calcium Quantification Using a 256-Dector Row CT Scanner. Acad Radiol 2019;26:e267-74. [Crossref] [PubMed]
- Brown ER, Kronmal RA, Bluemke DA, et al. Coronary calcium coverage score: determination, correlates, and predictive accuracy in the Multi-Ethnic Study of Atherosclerosis. Radiology 2008;247:669-75. [Crossref] [PubMed]
- Lichtenstein G, Perlman A, Shpitzen S, et al. Correlation between coronary artery calcification by non-cardiac CT and Framingham score in young patients. PLoS One 2018;13:e0195061. [Crossref] [PubMed]
- Jairam PM, Gondrie MJ, Grobbee DE, et al. Incidental imaging findings from routine chest CT used to identify subjects at high risk of future cardiovascular events. Radiology 2014;272:700-8. [Crossref] [PubMed]
- Jacobs PC, Prokop M, van der Graaf Y, et al. Comparing coronary artery calcium and thoracic aorta calcium for prediction of all-cause mortality and cardiovascular events on low-dose non-gated computed tomography in a high-risk population of heavy smokers. Atherosclerosis 2010;209:455-62. [Crossref] [PubMed]
- Hughes-Austin JM, Dominguez A 3rd, Allison MA, et al. Relationship of Coronary Calcium on Standard Chest CT Scans With Mortality. JACC Cardiovasc Imaging 2016;9:152-9. [Crossref] [PubMed]
- Rodríguez-Granillo GA, Reynoso E, Capuñay C, et al. Prognostic Value of Vascular Calcifications and Regional Fat Depots Derived From Conventional Chest Computed Tomography. J Thorac Imaging 2019;34:33-40. [Crossref] [PubMed]
- Vehmas T. Visually scored calcifications in thoracic arteries predict death: follow-up study after lung cancer CT screening. Acta Radiol 2012;53:643-7. [Crossref] [PubMed]
- Jacobs PC, Gondrie MJ, Mali WP, et al. Unrequested information from routine diagnostic chest CT predicts future cardiovascular events. Eur Radiol 2011;21:1577-85. [Crossref] [PubMed]
- Yu C, Ng ACC, Ridley L, et al. Incidentally identified coronary artery calcium on non-contrast CT scan of the chest predicts major adverse cardiac events among hospital inpatients. Open Heart 2021;8:e001695. [Crossref] [PubMed]
- Haller C, Vandehei A, Fisher R, et al. Incidence and Implication of Coronary Artery Calcium on Non-gated Chest Computed Tomography Scans: A Large Observational Cohort. Cureus 2019;11:e6218. [Crossref] [PubMed]
- Fan L, Fan K. Lung cancer screening CT-based coronary artery calcification in predicting cardiovascular events: A systematic review and meta-analysis. Medicine (Baltimore) 2018;97:e10461. [Crossref] [PubMed]
- Fresno CU, Tijmes FS, Thavendiranathan P, et al. Visual Ordinal Scoring of Coronary Artery Calcium on Contrast-Enhanced and Noncontrast Chest CT: A Retrospective Study of Diagnostic Performance and Prognostic Utility. AJR Am J Roentgenol 2022;219:569-78. [Crossref] [PubMed]
- Vakil P, Wen Z, Lima AS, et al. Predictive Value of Coronary Artery Calcium in Patients Receiving Computed Tomography Pulmonary Angiography for Suspected Pulmonary Embolism in the Emergency Department. J Thorac Imaging 2022;37:279-84. [Crossref] [PubMed]
- Hecht HS, Cronin P, Blaha MJ, et al. 2016 SCCT/STR guidelines for coronary artery calcium scoring of noncontrast noncardiac chest CT scans: A report of the Society of Cardiovascular Computed Tomography and Society of Thoracic Radiology. J Thorac Imaging 2017;32:W54-66. [Crossref] [PubMed]
- Hecht HS, Blaha MJ, Kazerooni EA, et al. CAC-DRS: Coronary Artery Calcium Data and Reporting System. An expert consensus document of the Society of Cardiovascular Computed Tomography (SCCT). J Cardiovasc Comput Tomogr 2018;12:185-91. [Crossref] [PubMed]
- Williams MC, Massera D, Moss AJ, et al. Presence And Quantification Of Valvular Heart Disease In The SCOT-HEART Trial. J Cardiovasc Comput Tomogr 2019;13:S11. [Crossref]
- Zhu Y, Wang Y, Gioia WE, et al. Visual scoring of aortic valve calcifications on low-dose CT in lung cancer screening. Eur Radiol 2020;30:2658-68. [Crossref] [PubMed]
- Willmann JK, Weishaupt D, Lachat M, et al. Electrocardiographically gated multi-detector row CT for assessment of valvular morphology and calcification in aortic stenosis. Radiology 2002;225:120-8. [Crossref] [PubMed]
- Baumgartner H. Recommendations on the echocardiographic assessment of aortic valve stenosis: a focused update from the European Association of Cardiovascular Imaging and the American Society of Echocardiography. Eur Heart J Cardiovasc Imaging 2017;18:254-75. [Crossref] [PubMed]
- Pawade T, Clavel MA, Tribouilloy C, et al. Computed Tomography Aortic Valve Calcium Scoring in Patients With Aortic Stenosis. Circ Cardiovasc Imaging 2018;11:e007146. [Crossref] [PubMed]
- Kaden JJ, Freyer S, Weisser G, et al. Correlation of degree of aortic valve stenosis by Doppler echocardiogram to quantity of calcium in the valve by electron beam tomography. Am J Cardiol 2002;90:554-7. [Crossref] [PubMed]
- Liu F, Coursey CA, Grahame-Clarke C, et al. Aortic valve calcification as an incidental finding at CT of the elderly: severity and location as predictors of aortic stenosis. AJR Am J Roentgenol 2006;186:342-9. [Crossref] [PubMed]
- Christensen JL, Tan S, Chung HE, et al. Aortic valve calcification predicts all-cause mortality independent of coronary calcification and severe stenosis. Atherosclerosis 2020;307:16-20. [Crossref] [PubMed]
- Katz R, Budoff MJ, Takasu J, et al. Relationship of metabolic syndrome with incident aortic valve calcium and aortic valve calcium progression: the Multi-Ethnic Study of Atherosclerosis (MESA). Diabetes 2009;58:813-9. [Crossref] [PubMed]
- Messika-Zeitoun D, Bielak LF, Peyser PA, et al. Aortic valve calcification: determinants and progression in the population. Arterioscler Thromb Vasc Biol 2007;27:642-8. [Crossref] [PubMed]
- Di Minno MND, Di Minno A, Ambrosino P, et al. Cardiovascular morbidity and mortality in patients with aortic valve sclerosis: A systematic review and meta-analysis. Int J Cardiol 2018;260:138-144. [Crossref] [PubMed]
- Nasir K, Katz R, Al-Mallah M, et al. Relationship of aortic valve calcification with coronary artery calcium severity: the Multi-Ethnic Study of Atherosclerosis (MESA). J Cardiovasc Comput Tomogr 2010;4:41-6. [Crossref] [PubMed]
- Gondrie MJ, van der Graaf Y, Jacobs PC, et al. The association of incidentally detected heart valve calcification with future cardiovascular events. Eur Radiol 2011;21:963-73. [Crossref] [PubMed]
- Owens DS, Budoff MJ, Katz R, et al. Aortic valve calcium independently predicts coronary and cardiovascular events in a primary prevention population. JACC Cardiovasc Imaging 2012;5:619-25. [Crossref] [PubMed]
- Adler Y, Fink N, Spector D, et al. Mitral annulus calcification--a window to diffuse atherosclerosis of the vascular system. Atherosclerosis 2001;155:1-8. [Crossref] [PubMed]
- Mahnken AH, Mühlenbruch G, Das M, et al. MDCT detection of mitral valve calcification: prevalence and clinical relevance compared with echocardiography. AJR Am J Roentgenol 2007;188:1264-9. [Crossref] [PubMed]
- Eberhard M, Schönenberger ALN, Hinzpeter R, et al. Mitral annular calcification in the elderly - Quantitative assessment. J Cardiovasc Comput Tomogr 2021;15:161-6. [Crossref] [PubMed]
- Guerrero M, Wang DD, Pursnani A, et al. A Cardiac Computed Tomography-Based Score to Categorize Mitral Annular Calcification Severity and Predict Valve Embolization. JACC Cardiovasc Imaging 2020;13:1945-57. [Crossref] [PubMed]
- Cavalcanti LRP, Sá MPBO, Perazzo ÁM, et al. Mitral Annular Calcification: Association with Atherosclerosis and Clinical Implications. Curr Atheroscler Rep 2020;22:9. [Crossref] [PubMed]
- Adler Y, Herz I, Vaturi M, et al. Mitral annular calcium detected by transthoracic echocardiography is a marker for high prevalence and severity of coronary artery disease in patients undergoing coronary angiography. Am J Cardiol 1998;82:1183-6. [Crossref] [PubMed]
- Hamirani YS, Nasir K, Blumenthal RS, et al. Relation of mitral annular calcium and coronary calcium (from the Multi-Ethnic Study of Atherosclerosis [MESA]). Am J Cardiol 2011;107:1291-4. [Crossref] [PubMed]
- Allison MA, Cheung P, Criqui MH, et al. Mitral and aortic annular calcification are highly associated with systemic calcified atherosclerosis. Circulation 2006;113:861-6. [Crossref] [PubMed]
- Utsunomiya H, Yamamoto H, Kunita E, et al. Combined presence of aortic valve calcification and mitral annular calcification as a marker of the extent and vulnerable characteristics of coronary artery plaque assessed by 64-multidetector computed tomography. Atherosclerosis 2010;213:166-72. [Crossref] [PubMed]
- Kohsaka S, Jin Z, Rundek T, et al. Impact of mitral annular calcification on cardiovascular events in a multiethnic community: the Northern Manhattan Study. JACC Cardiovasc Imaging 2008;1:617-23. [Crossref] [PubMed]
- Wang TKM, Griffin BP, Xu B, et al. Relationships between mitral annular calcification and cardiovascular events: A meta-analysis. Echocardiography 2020;37:1723-31. [Crossref] [PubMed]
- Bhatt H, Sanghani D, Julliard K, et al. Is Mitral Annular Calcification Associated With Atherosclerotic Risk Factors and Severity and Complexity of Coronary Artery Disease? Angiology 2015;66:659-66. [Crossref] [PubMed]
- Adler Y, Fisman EZ, Shemesh J, et al. Spiral computed tomography evidence of close correlation between coronary and thoracic aorta calcifications. Atherosclerosis 2004;176:133-8. [Crossref] [PubMed]
- Han D, Kuronuma K, Rozanski A, et al. Implication of thoracic aortic calcification over coronary calcium score regarding the 2018 ACC/AHA Multisociety cholesterol guideline: results from the CAC Consortium. Am J Prev Cardiol 2021;8:100232. [Crossref] [PubMed]
- Santos RD, Rumberger JA, Budoff MJ, et al. Thoracic aorta calcification detected by electron beam tomography predicts all-cause mortality. Atherosclerosis 2010;209:131-5. [Crossref] [PubMed]
- Gondrie MJ, Mali WP, Jacobs PC, et al. Cardiovascular disease: prediction with ancillary aortic findings on chest CT scans in routine practice. Radiology 2010;257:549-59. [Crossref] [PubMed]
- Budoff MJ, Nasir K, Katz R, et al. Thoracic aortic calcification and coronary heart disease events: the multi-ethnic study of atherosclerosis (MESA). Atherosclerosis 2011;215:196-202. [Crossref] [PubMed]
- Hoffmann U, Massaro JM, D'Agostino RB Sr, et al. Cardiovascular Event Prediction and Risk Reclassification by Coronary, Aortic, and Valvular Calcification in the Framingham Heart Study. J Am Heart Assoc 2016;5:e003144. [Crossref] [PubMed]
- Kälsch H, Mahabadi AA, Moebus S, et al. Association of progressive thoracic aortic calcification with future cardiovascular events and all-cause mortality: ability to improve risk prediction? Results of the Heinz Nixdorf Recall (HNR) study. Eur Heart J Cardiovasc Imaging 2019;20:709-17. [Crossref] [PubMed]
- Wong ND, Gransar H, Shaw L, et al. Thoracic aortic calcium versus coronary artery calcium for the prediction of coronary heart disease and cardiovascular disease events. JACC Cardiovasc Imaging 2009;2:319-26. [Crossref] [PubMed]
- Guglielmo M, Lin A, Dey D, et al. Epicardial fat and coronary artery disease: Role of cardiac imaging. Atherosclerosis 2021;321:30-8. [Crossref] [PubMed]
- Alexopoulos N, Katritsis D, Raggi P. Visceral adipose tissue as a source of inflammation and promoter of atherosclerosis. Atherosclerosis 2014;233:104-12. [Crossref] [PubMed]
- Iacobellis G, Corradi D, Sharma AM. Epicardial adipose tissue: anatomic, biomolecular and clinical relationships with the heart. Nat Clin Pract Cardiovasc Med 2005;2:536-43. [Crossref] [PubMed]
- Sacks HS, Fain JN. Human epicardial adipose tissue: a review. Am Heart J 2007;153:907-17. [Crossref] [PubMed]
- Cikim AS, Topal E, Harputluoglu M, et al. Epicardial adipose tissue, hepatic steatosis and obesity. J Endocrinol Invest 2007;30:459-64. [Crossref] [PubMed]
- Abazid RM, Smettei OA, Kattea MO, et al. Relation Between Epicardial Fat and Subclinical Atherosclerosis in Asymptomatic Individuals. J Thorac Imaging 2017;32:378-82. [Crossref] [PubMed]
- Franssens BT, Nathoe HM, Visseren FL, et al. Relation of Epicardial Adipose Tissue Radiodensity to Coronary Artery Calcium on Cardiac Computed Tomography in Patients at High Risk for Cardiovascular Disease. Am J Cardiol 2017;119:1359-65. [Crossref] [PubMed]
- Alexopoulos N, McLean DS, Janik M, et al. Epicardial adipose tissue and coronary artery plaque characteristics. Atherosclerosis 2010;210:150-4. [Crossref] [PubMed]
- Gorter PM, de Vos AM, van der Graaf Y, et al. Relation of epicardial and pericoronary fat to coronary atherosclerosis and coronary artery calcium in patients undergoing coronary angiography. Am J Cardiol 2008;102:380-5. [Crossref] [PubMed]
- Dey D, Wong ND, Tamarappoo B, et al. Computer-aided non-contrast CT-based quantification of pericardial and thoracic fat and their associations with coronary calcium and Metabolic Syndrome. Atherosclerosis 2010;209:136-41. [Crossref] [PubMed]
- Sade LE, Eroglu S, Bozbaş H, et al. Relation between epicardial fat thickness and coronary flow reserve in women with chest pain and angiographically normal coronary arteries. Atherosclerosis 2009;204:580-5. [Crossref] [PubMed]
- Shah RV, Anderson A, Ding J, et al. Pericardial, But Not Hepatic, Fat by CT Is Associated With CV Outcomes and Structure: The Multi-Ethnic Study of Atherosclerosis. JACC Cardiovasc Imaging 2017;10:1016-27. [Crossref] [PubMed]
- Cheng VY, Dey D, Tamarappoo B, et al. Pericardial fat burden on ECG-gated noncontrast CT in asymptomatic patients who subsequently experience adverse cardiovascular events. JACC Cardiovasc Imaging 2010;3:352-60. [Crossref] [PubMed]
- Ding J, Hsu FC, Harris TB, et al. The association of pericardial fat with incident coronary heart disease: the Multi-Ethnic Study of Atherosclerosis (MESA). Am J Clin Nutr 2009;90:499-504. [Crossref] [PubMed]
- Tamarappoo B, Dey D, Shmilovich H, et al. Increased pericardial fat volume measured from noncontrast CT predicts myocardial ischemia by SPECT. JACC Cardiovasc Imaging 2010;3:1104-12. [Crossref] [PubMed]
- Kenchaiah S, Ding J, Carr JJ, et al. Pericardial Fat and the Risk of Heart Failure. J Am Coll Cardiol 2021;77:2638-52. [Crossref] [PubMed]
- Mancio J, Azevedo D, Saraiva F, et al. Epicardial adipose tissue volume assessed by computed tomography and coronary artery disease: a systematic review and meta-analysis. Eur Heart J Cardiovasc Imaging 2018;19:490-7. [Crossref] [PubMed]
- Bastarrika G, Broncano J, Schoepf UJ, et al. Relationship between coronary artery disease and epicardial adipose tissue quantification at cardiac CT: comparison between automatic volumetric measurement and manual bidimensional estimation. Acad Radiol 2010;17:727-34. [Crossref] [PubMed]
- Nagayama Y, Nakamura N, Itatani R, et al. Epicardial fat volume measured on nongated chest CT is a predictor of coronary artery disease. Eur Radiol 2019;29:3638-46. [Crossref] [PubMed]
- Ahmadi N, Nabavi V, Yang E, et al. Increased epicardial, pericardial, and subcutaneous adipose tissue is associated with the presence and severity of coronary artery calcium. Acad Radiol 2010;17:1518-24. [Crossref] [PubMed]
- Ditomasso D, Carnethon MR, Wright CM, et al. The associations between visceral fat and calcified atherosclerosis are stronger in women than men. Atherosclerosis 2010;208:531-6. [Crossref] [PubMed]
- Lehman SJ, Massaro JM, Schlett CL, et al. Peri-aortic fat, cardiovascular disease risk factors, and aortic calcification: the Framingham Heart Study. Atherosclerosis 2010;210:656-61. [Crossref] [PubMed]
- Fox CS, Massaro JM, Schlett CL, et al. Periaortic fat deposition is associated with peripheral arterial disease: the Framingham heart study. Circ Cardiovasc Imaging 2010;3:515-9. [Crossref] [PubMed]
- Gowda RM, Boxt LM. Calcifications of the heart. Radiol Clin North Am 2004;42:603-17. vi-vii. [Crossref] [PubMed]
- Kimura F, Matsuo Y, Nakajima T, et al. Myocardial fat at cardiac imaging: how can we differentiate pathologic from physiologic fatty infiltration? Radiographics 2010;30:1587-602. [Crossref] [PubMed]
- Rodríguez-Granillo GA, Rosales MA, Renes P, et al. Chronic myocardial infarction detection and characterization during coronary artery calcium scoring acquisitions. J Cardiovasc Comput Tomogr 2010;4:99-107. [Crossref] [PubMed]
- Gupta M, Kadakia J, Hacioglu Y, et al. Non-contrast cardiac computed tomography can accurately detect chronic myocardial infarction: Validation study. J Nucl Cardiol 2011;18:96-103. [Crossref] [PubMed]
- Ichikawa Y, Kitagawa K, Chino S, et al. Adipose tissue detected by multislice computed tomography in patients after myocardial infarction. JACC Cardiovasc Imaging 2009;2:548-55. [Crossref] [PubMed]
- Batal O, Frick W, Dardari Z. Ct Subendocardial Fat in Patients with Prior Myocardial Infarction. J Cardiovasc Comput Tomogr 2021;15:S8. [Crossref]
- Raney AR, Saremi F, Kenchaiah S, et al. Multidetector computed tomography shows intramyocardial fat deposition. J Cardiovasc Comput Tomogr 2008;2:152-63. [Crossref] [PubMed]
- Su L, Siegel JE, Fishbein MC. Adipose tissue in myocardial infarction. Cardiovasc Pathol 2004;13:98-102. [Crossref] [PubMed]
- Sheifer SE, Manolio TA, Gersh BJ. Unrecognized myocardial infarction. Ann Intern Med 2001;135:801-11. [Crossref] [PubMed]
- Kannel WB, Abbott RD. Incidence and prognosis of unrecognized myocardial infarction. An update on the Framingham study. N Engl J Med 1984;311:1144-7. [Crossref] [PubMed]
- Jacobi AH, Gohari A, Zalta B, et al. Ventricular myocardial fat: CT findings and clinical correlates. J Thorac Imaging 2007;22:130-5. [Crossref] [PubMed]
- Bader AS, Levsky JM, Zalta BA, et al. Ventricular Myocardial Fat: An Unexpected Biomarker for Long-term Survival? Eur Radiol 2019;29:241-50. [Crossref] [PubMed]
- Hata Y, Koike Y, Kimura N, et al. Longitudinal effect of myocardial fat deposition on left ventricular diastolic function: a retrospective cohort study. Int J Cardiovasc Imaging 2021; Epub ahead of print. [Crossref] [PubMed]
- Lücke C, Schindler K, Lehmkuhl L, et al. Prevalence and functional impact of lipomatous metaplasia in scar tissue following myocardial infarction evaluated by MRI. Eur Radiol 2010;20:2074-83. [Crossref] [PubMed]
- Maceira AM, Prasad SK, Khan M, et al. Reference right ventricular systolic and diastolic function normalized to age, gender and body surface area from steady-state free precession cardiovascular magnetic resonance. Eur Heart J 2006;27:2879-88. [Crossref] [PubMed]
- Nasir K, Katz R, Mao S, et al. Comparison of left ventricular size by computed tomography with magnetic resonance imaging measures of left ventricle mass and volumes: the multi-ethnic study of atherosclerosis. J Cardiovasc Comput Tomogr 2008;2:141-8. [Crossref] [PubMed]
- Daniel KR, Bertoni AG, Ding J, et al. Comparison of methods to measure heart size using noncontrast-enhanced computed tomography: correlation with left ventricular mass. J Comput Assist Tomogr 2008;32:934-41. [Crossref] [PubMed]
- Kathiria NN, Devcic Z, Chen JS, et al. Assessment of Left Ventricular Enlargement at Multidetector Computed Tomography. J Comput Assist Tomogr 2015;39:794-6. [Crossref] [PubMed]
- Murphy DJ, Lavelle LP, Gibney B, et al. Diagnostic accuracy of standard axial 64-slice chest CT compared to cardiac MRI for the detection of cardiomyopathies. Br J Radiol 2016;89:20150810. [Crossref] [PubMed]
- Eifer DA, Nguyen ET, Thavendiranathan P, et al. Diagnostic Accuracy of Sex-Specific Chest CT Measurements Compared With Cardiac MRI Findings in the Assessment of Cardiac Chamber Enlargement. AJR Am J Roentgenol 2018;211:993-9. [Crossref] [PubMed]
- Hota P, Simpson S. Going beyond Cardiomegaly: Evaluation of Cardiac Chamber Enlargement at Non-Electrocardiographically Gated Multidetector CT: Current Techniques, Limitations, and Clinical Implications. Radiol Cardiothorac Imaging 2019;1:e180024. [Crossref] [PubMed]
- Hamer OW, Aguirre DA, Casola G, et al. Fatty liver: imaging patterns and pitfalls. Radiographics 2006;26:1637-53. [Crossref] [PubMed]
- Kang H, Greenson JK, Omo JT, et al. Metabolic syndrome is associated with greater histologic severity, higher carbohydrate, and lower fat diet in patients with NAFLD. Am J Gastroenterol 2006;101:2247-53. [Crossref] [PubMed]
- Ding J, Hsu FC, Szklo M, et al. Fatty liver and development of type 2 diabetes: the Multi-Ethnic Study of Atherosclerosis (MESA). Paper presented at: Obesity Society’s 2010 Annual Scientific Meeting; October 2010; San Diego, CA.
- Targher G, Bertolini L, Padovani R, et al. Relation of nonalcoholic hepatic steatosis to early carotid atherosclerosis in healthy men: role of visceral fat accumulation. Diabetes Care 2004;27:2498-500. [Crossref] [PubMed]
- Targher G, Bertolini L, Padovani R, et al. Relations between carotid artery wall thickness and liver histology in subjects with nonalcoholic fatty liver disease. Diabetes Care 2006;29:1325-30. [Crossref] [PubMed]
- Santos RD, Nasir K, Conceição RD, et al. Hepatic steatosis is associated with a greater prevalence of coronary artery calcification in asymptomatic men. Atherosclerosis 2007;194:517-9. [Crossref] [PubMed]
- Madaj P, Budoff MJ. Risk stratification of non-contrast CT beyond the coronary calcium scan. J Cardiovasc Comput Tomogr 2012;6:301-7. [Crossref] [PubMed]
- Targher G, Bertolini L, Poli F, et al. Nonalcoholic fatty liver disease and risk of future cardiovascular events among type 2 diabetic patients. Diabetes 2005;54:3541-6. [Crossref] [PubMed]
- Hamaguchi M, Kojima T, Takeda N, et al. Nonalcoholic fatty liver disease is a novel predictor of cardiovascular disease. World J Gastroenterol 2007;13:1579-84. [Crossref] [PubMed]
- Anil G, Guglielmi G, Peh WC. Radiology of osteoporosis. Radiol Clin North Am 2010;48:497-518. [Crossref] [PubMed]
- Li D, Mao SS, Khazai B, et al. Noncontrast cardiac computed tomography image-based vertebral bone mineral density: the Multi-Ethnic Study of Atherosclerosis (MESA). Acad Radiol 2013;20:621-7. [Crossref] [PubMed]
- Budoff MJ, Hamirani YS, Gao YL, et al. Measurement of thoracic bone mineral density with quantitative CT. Radiology 2010;257:434-40. [Crossref] [PubMed]
- Wang P, She W, Mao Z, et al. Use of routine computed tomography scans for detecting osteoporosis in thoracolumbar vertebral bodies. Skeletal Radiol 2021;50:371-9. [Crossref] [PubMed]
- Lian XL, Zhang YP, Li X, et al. Exploration on the relationship between the elderly osteoporosis and cardiovascular disease risk factors. Eur Rev Med Pharmacol Sci 2017;21:4386-90. [PubMed]
- Yoon YE, Kim KM, Han JS, et al. Prediction of Subclinical Coronary Artery Disease With Breast Arterial Calcification and Low Bone Mass in Asymptomatic Women: Registry for the Women Health Cohort for the BBC Study. JACC Cardiovasc Imaging 2019;12:1202-11. [Crossref] [PubMed]
- Hyder JA, Allison MA, Wong N, et al. Association of coronary artery and aortic calcium with lumbar bone density: the MESA Abdominal Aortic Calcium Study. Am J Epidemiol 2009;169:186-94. [Crossref] [PubMed]
- Jensky NE, Hyder JA, Allison MA, et al. The association of bone density and calcified atherosclerosis is stronger in women without dyslipidemia: the multi-ethnic study of atherosclerosis. J Bone Miner Res 2011;26:2702-9. [Crossref] [PubMed]
- Szulc P, Foesser D, Chapurlat R. High Cardiovascular Risk in Older Men with Poor Bone Microarchitecture-The Prospective STRAMBO Study. J Bone Miner Res 2021;36:879-91. [Crossref] [PubMed]
- Tankó LB, Christiansen C, Cox DA, et al. Relationship between osteoporosis and cardiovascular disease in postmenopausal women. J Bone Miner Res 2005;20:1912-20. [Crossref] [PubMed]
- Kim H, Greenberg JS, Javitt MC. Breast calcifications due to Mönckeberg medial calcific sclerosis. Radiographics 1999;19:1401-3. [Crossref] [PubMed]
- Lanzer P, Boehm M, Sorribas V, et al. Medial vascular calcification revisited: review and perspectives. Eur Heart J 2014;35:1515-25. [Crossref] [PubMed]
- Kelly BS, Scanl ON E, Heneghan H, et al. Breast Arterial Calcification on screening mammography can predict significant Coronary Artery Disease in women. Clin Imaging 2018;49:48-53. [Crossref] [PubMed]
- Proudfoot D, Shanahan CM. Biology of calcification in vascular cells: intima versus media. Herz 2001;26:245-51. [Crossref] [PubMed]
- Ryan AJ, Choi AD, Choi BG, et al. Breast arterial calcification association with coronary artery calcium scoring and implications for cardiovascular risk assessment in women. Clin Cardiol 2017;40:648-53. [Crossref] [PubMed]
- Jiang X, Clark M, Singh RK, et al. Association of breast arterial calcification with stroke and angiographically proven coronary artery disease: a meta-analysis. Menopause 2015;22:136-43. [Crossref] [PubMed]
- Lee SC, Phillips M, Bellinge J, et al. Is breast arterial calcification associated with coronary artery disease?-A systematic review and meta-analysis. PLoS One 2020;15:e0236598. [Crossref] [PubMed]
- Hendriks EJ, de Jong PA, van der Graaf Y, et al. Breast arterial calcifications: a systematic review and meta-analysis of their determinants and their association with cardiovascular events. Atherosclerosis 2015;239:11-20. [Crossref] [PubMed]
- Schnatz PF, Marakovits KA, O'Sullivan DM. The association of breast arterial calcification and coronary heart disease. Obstet Gynecol 2011;117:233-41. [Crossref] [PubMed]
- Shah N, Chainani V, Delafontaine P, et al. Mammographically detectable breast arterial calcification and atherosclerosis. Cardiol Rev 2014;22:69-78. [Crossref] [PubMed]
- Soylu Aİ, Soylu K, Aydın R, et al. Calcification of breast artery as detected by mammography: association with coronary and aortic calcification. Turk J Med Sci 2019;49:190-7. [Crossref] [PubMed]
- Hendriks EJ, Beulens JW, de Jong PA, et al. Calcification of the splenic, iliac, and breast arteries and risk of all-cause and cardiovascular mortality. Atherosclerosis 2017;259:120-7. [Crossref] [PubMed]
- Bui QM, Daniels LB. A Review of the Role of Breast Arterial Calcification for Cardiovascular Risk Stratification in Women. Circulation 2019;139:1094-101. [Crossref] [PubMed]
- Mendoza DP, Kako B, Digumarthy SR, et al. Impact of Significant Coronary Artery Calcification Reported on Low-Dose Computed Tomography Lung Cancer Screening. J Thorac Imaging 2020;35:129-135. [Crossref] [PubMed]
- Mets OM, Vliegenthart R, Gondrie MJ, et al. Lung cancer screening CT-based prediction of cardiovascular events. JACC Cardiovasc Imaging 2013;6:899-907. [Crossref] [PubMed]
- Xie X, Zhao Y, de Bock GH, et al. Validation and prognosis of coronary artery calcium scoring in nontriggered thoracic computed tomography: systematic review and meta-analysis. Circ Cardiovasc Imaging 2013;6:514-21. [Crossref] [PubMed]
- Williams MC, Abbas A, Tirr E, et al. Reporting incidental coronary, aortic valve and cardiac calcification on non-gated thoracic computed tomography, a consensus statement from the BSCI/BSCCT and BSTI. Br J Radiol 2021;94:20200894. [Crossref] [PubMed]
- Munden RF, Carter BW, Chiles C, et al. Managing Incidental Findings on Thoracic CT: Mediastinal and Cardiovascular Findings. A White Paper of the ACR Incidental Findings Committee. J Am Coll Radiol 2018;15:1087-96. [Crossref] [PubMed]
- Seetharam K, Brito D, Farjo PD, et al. The Role of Artificial Intelligence in Cardiovascular Imaging: State of the Art Review. Front Cardiovasc Med 2020;7:618849. [Crossref] [PubMed]
- Eng D, Chute C, Khandwala N, et al. Automated coronary calcium scoring using deep learning with multicenter external validation. NPJ Digit Med 2021;4:88. [Crossref] [PubMed]
- Zeleznik R, Foldyna B, Eslami P, et al. Deep convolutional neural networks to predict cardiovascular risk from computed tomography. Nat Commun 2021;12:715. [Crossref] [PubMed]
- van Velzen SGM, Lessmann N, Velthuis BK, et al. Deep Learning for Automatic Calcium Scoring in CT: Validation Using Multiple Cardiac CT and Chest CT Protocols. Radiology 2020;295:66-79. [Crossref] [PubMed]