Percutaneous coronary stent implantation in children and young infants following surgical repair of congenital heart disease
Highlight box
Key findings
• Coronary stent implantation (CSI) after pediatric cardiac surgery is feasible with 54.5% survival to hospital discharge.
What is known, and what is new?
• There are only a few reports describing CSI for the management of coronary artery obstruction (CAO) after surgical correction of congenital heart disease (CHD), which were small case series and case reports.
• The overall survival rates of CSI following pediatric cardiac surgery are low due to the severity of the underlying disease, technical difficulties, and limited therapeutic options.
What is the implication, and what should change now?
• CSI can be performed with high procedural success, and it may play an essential role in the management of post-surgical coronary artery stenosis.
Introduction
Coronary artery obstruction (CAO) after surgical correction of congenital heart disease (CHD) is rare but significantly impacts morbidity and mortality (1,2). CAO was reported as a postoperative complication following different surgical CHD repairs, including the arterial switch operation (ASO) and from dissection of the ascending aorta extending into the coronary artery (3).
After ASO, the incidence of CAO is reported to be 3–11% due to coronary artery translocation, representing the most difficult aspect (4). Anatomical deformation, stretching, external compression, and intimal proliferation are potential mechanisms for coronary occlusion (5). Rates of postoperative CAO resulting from surgical repair of other CHD are inadequately established, with the majority of published data consisting of individual case reports or limited case series (1).
Percutaneous coronary interventions (PCI) are uncommon interventional procedures in children, and publications detailing short- and long-term outcomes are lacking. PCI in the pediatric population is demanding and requires extensive theoretical and practical expertise. The small vessel size contributes to the procedural difficulty and the high risk of coronary interventions at this young age (4,6). According to the guidelines of the American Heart Association, cardiac catheterization, and coronary angiography can be beneficial in cases of a postoperative course that unexpectedly becomes complicated, including an unexplained requirement for mechanical circulatory support (Class IIa recommendation, Level of Evidence: C) (7). Selective coronary angiography is considered the diagnostic gold standard in such cases of postoperative CAO (8).
There are only a few reports on managing postoperative CAO with coronary stent implantation (CSI), which were small case series and case reports (1,4,5,9,10). Accordingly, the available data describing patient characteristics, presentation modalities, diagnostic criteria, and outcomes of CSI in children is limited (1). We aim to analyze the feasibility, indications, procedural technique, risk factors, and short-term outcomes of CSI performed to treat CAO after pediatric cardiac surgery. We present this article in accordance with the STROBE reporting checklist (available at https://cdt.amegroups.com/article/view/10.21037/cdt-23-54/rc).
Methods
Patients
We retrospectively reviewed all patients who underwent surgical repair of CHD requiring postoperative CSI in two cardiac centers (King Abdulaziz University Hospital and King Faisal Specialist Hospital and Research Center) between November 2012 and November 2022. The study was conducted in accordance with the Declaration of Helsinki (as revised in 2013). The study was approved by the institutional review board and ethics committee of King Abdulaziz University Hospital (No. 539-20), King Faisal Specialist Hospital and Research Center (No. 2021-17), and individual consent for this retrospective analysis was waived.
Data collection
Demographic, preoperative, operative, and postoperative data were gathered from the patients’ medical records from December 2022 to February 2023. Relevant perioperative information was identified, including the requirement of extracorporeal membrane oxygenation (ECMO) support, indication for CSI, procedural details, cardiac enzymes, electrocardiogram (ECG), and echocardiographic findings. The primary outcome was survival to hospital discharge, and the secondary outcomes included procedural success, duration of mechanical ventilation, intensive care unit (ICU) stay, hospital stay, need for coronary reintervention or reoperation, and late mortality.
Early mortality was defined as the occurrence of death either prior to discharge from the hospital or within a period of 30 days following CSI. Late mortality was defined as mortality occurring beyond 30 days from the interventional procedure (4). CSI was considered successful when a significant improvement in lumen gain in the treated vessel and coronary perfusion was achieved and confirmed by angiography at the end of the procedure to visualize the coronary vessels (4). A sentinel event was identified to acknowledge a significant clinical change and deviation from the anticipated postoperative course, a concept utilized in previous analogous research studies (1).
CSI procedure
All procedures were performed under general anesthesia. Routine vascular access and full heparinization were obtained primarily. Contrary to adult coronary angiography, non-selective angiography via aortic root injection was the standard practice in our study to get the origin of the usually not normally located coronaries. A 4-French sheath (Cordis®, Miami Lakes, FL, USA) was used if body weight <10 kg, and a 5-French sheath was used if body weight ≥10 kg. Vertebral Terumo catheter (Terumo®, Tokyo, Japan) or right Judkins catheter (Cordis®) was used for angiography. Different angiographic projections were obtained accordingly to get the best one for intervention. Then a 0.014-inch coronary guidewire (Balance Middle Weight, BMW, Abbott, Chicago, IL, USA) was used to cross the lesion. Occasionally, a 0.035-inch J-tipped Terumo wire (Terumo®) was used to entertain the coronary artery and get the catheter closer to the coronary ostium, then replaced with the regular 0.014-inch guidewire.
Unlike adult and older pediatric coronary interventions, we had never used guiding catheters, coronary vasodilators, or engaged the catheter into the coronary ostium, as coronary vasospasm can occur, especially in small patients with very small coronary arteries. Because of the absence of a guiding catheter and our intention to minimize the amount of contrast to minimize kidney injury, we used anatomical landmarks to localize the lesion and the proper stent place inside.
Based on the size of the coronary artery, site, and stenosis severity, direct stenting or pre-dilation with a monorail or over-the-wire coronary balloon ranging from 1.5 to 2.5 mm was performed (Figures 1,2). Drug-eluting stents (DES) (Promus Element, Boston Scientific, Natick, MA, USA) were used for all patients except two; one (patient 9) had bare metal Micro-Driver coronary stent (Medtronic, Minneapolis, MN, USA), and the other had a bio-absorbable stent (patient 3). CSI was performed on an emergency basis, and choosing the stents depended on the availability of the type and size of the required stent. We had never used stents smaller than 2.25 mm in diameter; on the other hand, we used a 3.5 mm diameter in the 9-year-old patient (patient 10). After coronary stenting, a final angiography determined the appropriate stent position and procedural success (Figures 1,2). Patients on ECMO were initially managed after the procedure with heparin monotherapy. Following ECMO decannulation, they have transitioned to a dual antiplatelet therapy (DAPT) regimen, with a combination of aspirin (3–5 mg/kg body weight) and clopidogrel (0.2 mg/kg body weight), as soon as clinically feasible. Patient 9 did not require ECMO support and received DAPT after CSI. The survivors regularly followed up in the pediatric cardiology outpatient clinic for clinical and imaging evaluation, including ECG and echocardiography.
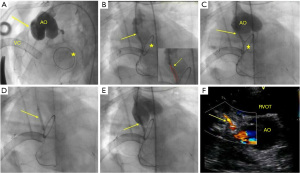
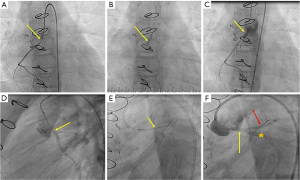
Statistical analysis
The numeric data were presented as a median and interquartile range, and the nominal/categorical variables were expressed as numbers or numbers and percentages.
Results
Patient characteristics
During the study period, 3,567 pediatric patients with CHD underwent cardiac surgery at both centers, including 11 patients who underwent post-surgical CSI (0.003%).
Six patients were females (54.5%). At the time of CSI, the median age was nine months (range, 10 days to 9 years), and the median weight was 6 kg (range, 2.7–47). Five out of 11 patients were ≤6 months of age (10 days, 2 months, 2.5 months, 5 months, and 6 months).
The most common underlying cardiac morphologies were congenital aortic valve stenosis (AS, n=5), transposition of great arteries (TGA) (n=2), single ventricle lesions (n=2), Shone’s complex (n=1), and truncus arteriosus type Ⅲ (n=1). The most common procedures associated with CAO were the Bentall procedure (11) (n=4), ASO (n=2), Damus–Kaye–Stansel (DKS) procedure (12) with Blalock-Taussig (BT) shunt (n=2), Konno procedure (n=2), and Ross-Konno procedure (13) (n=1). All the procedures included coronary arteries reimplantation except DKS and Konno procedures. Nine patients (81.8%) underwent biventricular repair, and two (18.2%) experienced univentricular stage 1 palliation. The median cardiopulmonary bypass time (CPB) and cross-clamp time for the surgical procedures were 187 and 128 min, respectively. Table 1 summarizes the characteristics and the operative details of the patients.
Table 1
Serial | Age at stenting | Sex | Weight (kg) | Height (cm) | Surface area (m2) | Primary diagnosis | Cardiac surgery prior to stenting | Previous interventions | CPB time (min) | Cross clamp time (min) |
---|---|---|---|---|---|---|---|---|---|---|
1 | 2 months | Female | 2.7 | 47 | 0.18 | DORV, TGA, COA, small RV | DKS, BT shunt | – | 360 | 140 |
2 | 9 months | Female | 9 | 74 | 0.37 | Truncus arteriosus type III | Konno, RV-PA conduit, AVR | Truncal valve repair, unifocalization, BT shunt | 322 | 249 |
3 | 8 years | Female | 47 | 138 | 1.3 | AS | Bentall procedure | BAV | 187 | 128 |
4 | 10 days | Male | 3.3 | 48 | 0.2 | TGA | ASO | – | 210 | 84 |
5 | 2.5 months | Male | 4.5 | 55 | 0.25 | TGA | ASO | – | 167 | 96 |
6 | 5 months | Female | 5 | 58 | 0.28 | AS | Bentall procedure | BAV (followed by severe AI) | 253 | 150 |
7 | 3 years | Male | 11 | 90 | 0.53 | AS, Supra AS, IAA, VSD | Bentall procedure | IAA repair; Balloon dilatation of supra AS; aortic arch augmentation, SAM resection | 180 | 130 |
8 | 6 months | Male | 5.1 | 62 | 0.29 | ccTGA, TA, moderate MR | DKS, BT shunt | – | 113 | 79 |
9 | 13 months | Female | 6 | 71 | 0.34 | Shone’s complex (AS, MS) | Konno, MVR | BAV, mitral valve repair | 147 | 107 |
10 | 9 years | Female | 37 | 142 | 1.21 | AS, SAM | Ross Konno | SAM resection | 215 | 150 |
11 | 7 years | Male | 13.3 | 102 | 0.62 | As, SAM, LVOTO | Bentall procedure | SAM resection | 143 | 115 |
CPB, cardiopulmonary bypass; DORV, double outlet right ventricle; TGA, transposition of the great arteries; COA, coarctation; RV, right ventricle; DKS, Damus-Kaye-Stansel; BT shunt, Blalock-Taussig shunt; PA, pulmonary artery; AVR, aortic valve replacement; AS, aortic stenosis; BAV, balloon aortic valvuloplasty; ASO, arterial switch operation; AI, aortic insufficiency; IAA, interrupted aortic arch; VSD, ventricular septal defect; SAM, subaortic membrane; ccTGA, congenitally corrected TGA; TA, tricuspid atresia; MR, mitral regurgitation; MS, mitral stenosis; MVR, mitral valve replacement; LVOTO, left ventricular outflow tract obstruction.
The indications for coronary interventions included six cases of failure to separate from CPB, four cases of unexplained significant ventricular dysfunction, and one case presenting with chest pain 105 days after the Ross Konno procedure (patient 10). Patient 5 was discharged from the hospital 49 days after ASO; then, he was admitted two weeks later (63 days after surgery) with severe left ventricular (LV) dysfunction that required ECMO support.
CSI occurred within a median of 24 hours after a sentinel event (1–168 hours). All patients underwent CSI within 24 hours of the sentinel event except two patients (Table 2); one (patient 7) had CSI 48 hours after failure to disconnect from CPB, and the other one (patient 2) was connected to ECMO in the operation room due to poor right ventricular function which did not improve after seven days on ECMO support then she underwent right coronary artery (RCA) stenting. She was successfully decannulated after 24 hours of CSI.
Table 2
Serial | Indication for CSI | Coronary event to CSI (hour) | Peak troponin before CSI (µg/L) | Troponin 24 h after CSI (µg/L) | Troponin 48 h after CSI (µg/L) | EF before CSI (%) | EF 24–48 h after CSI (%) | EF last seen (%) | Time to last EF (days) |
---|---|---|---|---|---|---|---|---|---|
1 | Failure to separate from CPB | 24 | 49 | 30.6 | 19.8 | 14 | 15 | 15 | 9 |
2 | Failure to separate from CPB | 168 | – | – | – | 40 | 48 | 60 | 42 |
3 | Unexplained ventricular dysfunction | 24 | 9.5 | 5.8 | 3.8 | 15 | 40 | 40 | 117 |
4 | Failure to separate from CPB | 24 | 12.9 | 40.1 | 41.6 | 10 | 15 | 22 | 5 |
5 | Unexplained ventricular dysfunction | 24 | 4.6 | 3.7 | 1.8 | 16 | 25 | 45 | 890 |
6 | Unexplained ventricular dysfunction | 3 | 9.4 | 7.6 | 2.9 | 12 | 20 | 38 | 4 |
7 | Failure to separate from CPB | 48 | 21 | 12.3 | 8.3 | 15 | 24 | 28 | 36 |
8 | Failure to separate from CPB | 24 | 25 | 50 | 34.7 | 10 | 10 | 10 | 5 |
9 | Failure to separate from CPB | 1 | 115.7 | 40 | 90.6 | 25 | 45 | 55 | 1,695 |
10 | Chest pain | 20 | 0.52 | 0.45 | 0.39 | 45 | 55 | 73 | 225 |
11 | Unexplained ventricular dysfunction | 19 | 11 | 4.5 | 2.7 | 30 | 43 | 65 | 20 |
CSI, coronary stent implantation; EF, ejection fraction; CPB, cardiopulmonary bypass.
The median peak troponin level before CSI for the entire cohort was 11 µg/L (0.52–115.7 µg/L). The median troponin level 24 and 48 hours after CSI was 9.95 and 6.05 µg/L, respectively. The median ejection fraction (EF) before CSI was 15%. The median EF 24–48 hours and the last follow-up echocardiography post-CSI were 25% and 40%, respectively (Table 2). All patients had nonspecific ST-T wave abnormalities except patient 3, who developed ventricular fibrillation.
The most common coronary artery involved was the left main coronary artery (LMCA, 5 patients), RCA (4 patients), both LMCA and RCA in one patient, and the circumflex artery was obstructed in another patient. The commonest lesions included the Ostia of the LMCA, followed by the proximal portion of the LMCA and the Ostia of the RCA. The median diameter of the implanted stents was 2.5 mm (range, 2.25 to 3.5), and the average length was 12 mm (range, 8 to 18). The median procedural duration was 105 minutes, and the fluoroscopy time was 28 minutes. The patients received a median contrast volume of 4 mL/kg during the procedures (Table 3).
Table 3
Serial | The stented coronary artery | Approach | Stent type | Stent diameter (mm) | Stent length (mm) | Procedure duration (minutes) | Fluoroscopy time (minutes) | Contrast (mL/kg) |
---|---|---|---|---|---|---|---|---|
1 | LMCA | Hybrid | DES | 2.5 | 8 | 360 | 40 | 2.5 |
2 | RCA | Femoral | DES | 2.25 | 12 | 105 | 29 | 10 |
3 | LMCA | Femoral | Bio-absorbable | 3 | 10 | 65 | 27 | 1.8 |
4 | LMCA | Femoral | DES | 2.25 | 8 | 100 | 28 | 4 |
5 | LMCA | Hybrid | DES | 2.5 | 8 | 120 | 25 | 3 |
6 | LMCA and RCA | Hybrid | DES | 2.25 | 18 | 180 | 40 | 6.5 |
7 | Circumflex | Femoral | DES | 2.5 | 12 | 280 | 60 | 12 |
8 | LMCA | Femoral | DES | 2.5 | 8 | 360 | 58 | 9 |
9 | RCA | Femoral | BMS | 2.5 | 15 | 90 | 24 | 4.4 |
10* | RCA | Femoral | DES | 3.5 | 12 | 80 | 27 | 1.5 |
11 | RCA | Femoral | DES | 3 | 22 | 80 | 21 | 4 |
*, 3 months after RCA stenting, she underwent LMCA, and LAD artery stenting using a DES stent (3 mm × 22 mm). The procedural duration was 80 min, and the fluoroscopy time was 21 min. She received a contrast volume of 4 mL/kg. She was discharged home the next day after the procedure. LMCA, left main coronary artery; DES, drug-eluting stent; RCA, right coronary artery; BMS, bare metal stent; LAD, left anterior descending artery.
Outcomes
Procedural success was achieved in all patients with excellent revascularization documented by post-procedural angiograms. There were no procedural complications. Patient 1 had residual coronary stenosis requiring balloon dilation 72 hours after CSI. Patient 10 presented initially with chest pain and elevated ST segment; she underwent computed tomography (CT), coronary angiography, and cardiac magnetic resonance imaging (MRI) that showed diffuse severe concentric aortic homograft mural wall thickening (up to 10 mm), moderate LMCA stenosis (50%), severe long segment (8 mm), LAD artery stenosis (80%), and severe RCA stenosis (80%). She underwent RCA stenting and received steroid therapy for aortitis. Three months later, she underwent an MRI cardiac stress adenosine study that revealed a significant interval reduction in aortic homograft mural wall thickening (down to 5 mm) and inducible myocardial perfusion defects on adenosine vasodilator stress perfusion in the anterior, septal, and inferior segments. After that, LMCA and LAD artery stenting was performed using DES stent (3 mm × 22 mm), and she was discharged home the next day of the procedure.
All patients underwent cardiac catheterization on ECMO except one patient (Patient 10), who presented with chest pain later after cardiac surgery. Excluding patient 10, the median duration of ECMO support was 4 days (1 to 12), 6 out of 10 patients who required ECMO support were successfully decannulated, and 4 out of the 6 patients who underwent successful decannulation survived until hospital discharge. Patient 7 was successfully decannulated and died after 40 days in the ICU due to septicemia and multiple organ dysfunction. After CSI, the median duration of mechanical ventilation was 12 days (5–38 days), and the median duration of ICU stay was 17 days (5 to 40). For the entire cohort, six patients survived until hospital discharge (54.5%) (Table 4).
Table 4
Serial | Duration of ECMO after CSI (days) | Successful decannulation | Duration of MV after CSI (days) | ICU stay (days) | Total hospital stay (days) | Survival to hospital discharge | Status | Time to status (days) |
---|---|---|---|---|---|---|---|---|
1 | 12 | No | 12 | 13 | 28 | No | Death | 13 |
2 | 1 | Yes | 14 | 25 | 30 | Yes | Death | 426 |
3 | 2 | Yes | 11 | 17 | 32 | Yes | Death | 233 |
4 | 4 | No | 5 | 6 | 7 | No | Death | 5 |
5 | 5 | Yes | 14 | 30 | 38 | Yes | Death | 903 |
6 | 4 | No | 5 | 6 | 6 | No | Death | 4 |
7 | 5 | Yes | 38 | 40 | 43 | No | Death | 37 |
8 | 5 | No | 5 | 5 | 6 | No | Death | 5 |
9 | 5 | Yes | 18 | 31 | 33 | Yes | Alive | 1,695 |
10 | 0 | – | 0 | 0 | 7 | Yes | Alive | 295 |
11 | 3 | Yes | 23 | 30 | 37 | Yes | Alive | 38 |
ECMO, extracorporeal membrane oxygenation; CSI, coronary stent implantation; MV, mechanical ventilation; ICU, intensive care unit.
Follow-up
The six patients who survived hospital discharge were followed up for a median duration of 360.5 days (38 to 1,695). Late mortality occurred in 3 out of 6 patients after hospital discharge resulting in overall survival of 27.3% (Table 4). Patient 2 experienced a chest infection and respiratory failure and expired 426 days post-CSI. Patient 3 had heart failure manifestations due to poor LV systolic function (LVEF was 40%) and developed cardiac arrest 233 days post-CSI. Patient 5 had biventricular dysfunction (LVEF was 45%) and pulmonary artery branch stenosis following ASO that required right and left pulmonary artery stenting; it was complicated by reperfusion lung injury and severe pulmonary hemorrhage leading to death 903 after CSI. The implanted coronary stents for the survivors did not require re-intervention during the follow-up period following hospital discharge.
Variables associated with survival to hospital discharge
Five of the six patients (83.3%) who survived hospital discharge were females. The oldest patients in this cohort were 7, 8, and 9 years who survived hospital discharge after CSI. We identified seven infants <2 years of age (10 days, 2 months, 2.5 months, 5 months, 6 months, 9 months, and 13 months); 3 out of the 7 infants (42.9%) survived until hospital discharge after CSI, the youngest patient was 2.5 months of age. The two patients who underwent univentricular stage 1 palliation did not survive post-CSI (Tables 1,4).
Both patients who had late coronary events 63 and 105 days after cardiac surgery survived hospital discharge. The younger patient (2.5 months) required ECMO support, but the older patient (9 years) did not even need mechanical ventilation. There was no in-hospital mortality among the two patients who required stenting of only the RCA. On the other hand, we reported mortality in 4 out of 7 patients (57.1%) who had LMCA stenting. A bio-absorbable stent and a bare-metal stent (BMS) were used in two patients who survived hospital discharge (patient 3 and patient 9, respectively). The four patients who required more than 120 minutes to complete the procedure had early mortality (Tables 3,4). The procedure was prolonged in some cases because of the time needed for a complete hemodynamic study to identify the cause of clinical deterioration, multidisciplinary discussion with pediatric cardiology and cardiac surgery teams for choosing the appropriate intervention, and sometimes the difficulty in cannulating the coronary artery with a wire.
Discussion
The therapeutic options for CAO after ASO were described in the literature, including percutaneous transluminal coronary angioplasty (PTCA) and stenting. In addition, myocardial revascularization through coronary artery bypass graft surgery (CABG) is possible even in children (5,14). Previous studies have shown early postoperative cardiac catheterization’s safety and potential benefit for CHD patients with a complicated postoperative course (1,7,8,15).
To date, no general recommendations exist concerning the treatment of CAO in children, and the optimal interventional strategy remains controversial. A previous report demonstrated adequate growth of coronary arteries years after PTCA. In the absence of reduced LV function, PTCA was preferred for fear of the risk of sudden cardiac death (14). Conversely, CSI with excellent procedural success has been described (9). Additionally, Bratincsak and colleagues showed that PTCA alone was associated with unsatisfactory clinical results, and CSI was more effective and resulted in the recovery of ventricular function (9). However, no data is available concerning the requirement of stent re-dilatation to keep up with coronary arteries’ somatic growth (4).
CABG is the most commonly reported therapeutic option for myocardial revascularization in the pediatric population with Kawasaki disease (16). In critical conditions, CSI may improve myocardial perfusion and save patients when surgical intervention is not feasible due to the patient’s condition. CABG presents higher risks for patients with poor ventricular function in comparison to PCI. In these instants, a temporary solution of CSI may serve better than surgery. It can be used as a bridge to elective CABG or reparative surgery in patients presenting with CAO and depressed myocardial function (9). CSI may play an essential role in neonates undergoing extensive surgical repairs, particularly in cases where additional coronary manipulation may be challenging (10).
Since the first successful coronary stenting in 1998 for a 4-year-old child following CHD surgery (17), several case reports were published, and a few small case series (1,4,5,9,10). Reviewing the literature, a few reports included infants who underwent CSI after cardiac surgery (10,18-21).
In the current literature, the most common procedures associated with post-surgical CAO were the ASO and, less commonly, Stage 1 (Norwood) procedure, aortic valve repair/replacement, and other procedures generally deemed to pose a lower risk of injury to the coronary arteries (1). Goldsmith and colleagues recommended a high index of suspicion for acute CAO when the patient has an unexpectedly complicated postoperative course, irrespective of the primary diagnosis (1). Interestingly, the most common procedure associated with CAO in this cohort was the surgical management of complex aortic stenosis using Bentall, Konno, or Ross-Konno procedures.
Consistent with a previous report, the most prevalent ECG findings in this study were nonspecific ST-segment abnormalities. This ECG finding is relatively prevalent in the postoperative period; thus, maintaining a high index of suspicion is crucial in this population, especially in the absence of ECG abnormalities suggestive of myocardial ischemia (1).
In the present study, CAO was detected early postoperative in 9 out of 11 patients who required ECMO support due to failure to disconnect from CPB or unexplained ventricular dysfunction. The actual occurrence of all coronary lesions subsequent to CHD surgery may have been undervalued, given that certain patients who exhibit clinical CAO may not have been detected during the initial postoperative phase and, consequently, may have gone unrecorded. Two patients of this series were diagnosed with CAO late after surgery; one was a 2.5-month-old discharged from the hospital after ASO and presented 63 days after surgery with poor LV function. Bonnet and colleagues reported that late coronary events might appear even in asymptomatic patients after ASO with a smooth postoperative course (22). Additionally, it is possible that patients may not experience chest pain due to the denervation of the heart that occurs during ASO (5). The capacity of neonates or infants to develop collaterals or cellular proliferation after an acute CAO is unknown, but it has been proposed that it may be potentially greater than in adults (23).
A recent study suggested that a shorter time from a coronary-related sentinel event to a diagnosis of CAO, followed by a coronary intervention, was linked to better clinical outcomes (1). In line with this recommendation, most of our patients (9 out of 11) underwent CSI within 24 hours of the identified sentinel event.
There is limited data and a lack of guidelines regarding using BMS or DES in the pediatric population. However, both types of stents were utilized in the published pediatric cases (5,9). In this cohort, DES was implanted in 9 out of 11 patients. Our success rate was 100% with complete recanalization of the affected coronary segments documented by angiograms after completing the procedure in all ten children. CSI may induce acute coronary injury that activates a cascade stimulating platelet aggregation (24). DAPT with acetylsalicylic acid (aspirin) and clopidogrel was used in the reported pediatric small case series and proved to be essential to prevent in-stent restenosis (5,9,10). Similarly, we have chosen to treat our patients with DAPT, and the patients did not experience problems using this antiplatelet therapy regimen.
In a previous series describing acute CAO after pediatric cardiac surgery, 80% of patients required ECMO support, 27% were listed for a heart transplant, and 41% had early mortality (1). A recent study reported a high in-hospital mortality rate following coronary interventions for children with CHD despite successful PCI in most patients, which could be due to severe irreversible ischemic myocardial damage before PCI (4). Consistent with these reports, 91% of our patients required ECMO support. In-hospital mortality occurred in 45.5%, with three late mortalities resulting in an overall survival probability of 27.3% for CSI after pediatric cardiac surgery.
Goldsmith and colleagues found that hypoplastic left heart syndrome (HLHS) was a risk factor for mortality or listing for a heart transplant in those with post-surgical acute CAO (1). In this cohort, univentricular stage 1 palliation, failure to disconnect from CPB, LMCA stenting, and a long procedural duration were associated with a relatively higher risk of early mortality. On the other hand, a better chance of survival was associated with older children, late coronary events, and RCA stenting. Importantly, we found that the peak troponin levels were lower, and the LVEF was higher among survivors compared to in-hospital deaths before and 24–48 hours after CSI.
In patients with LV dysfunction, it has been demonstrated that they benefit from PCI and LV function normalized within 3 to 6 weeks (14), or it may take up to 6 months to recover completely after the intervention (9,10,20). Our study showed marked improvement in LV function with EF normalization in 4 out of 6 patients who survived hospital discharge. The LVEF of the other two patients reached 40% and 45%; both had heart failure manifestations and died 233 and 903 days after CSI, respectively.
Bratincsák et al. stated that a more than 2.5 mm stent diameter is likely associated with a more prolonged short-to-mid-term survival free from reintervention (9). The long-term outcome of stenting small coronary vessels in children is unknown (17). There is concern that, as the child grows, the coronary artery will “outgrow” the stent. There is a growing consensus that CABG in the future is a foregone conclusion. Others believe collateral circulation may develop to maintain sufficient blood supply to the affected coronary territory (20).
In this study, the diameter of the implanted stents in 5 out of the six survivors was ≥2.5 mm. Those patients had a follow-up for a duration ranging from 38 to 1,695 days without a requirement for reintervention. However, whether the clinical improvement and the freedom of intervention were due to stent patency or collateral development can only be answered by performing coronary angiography or CT angiography for these patients. In fact, we did not have clinical indications to subject those patients to additional procedures.
This cohort adds to the literature more data about the short-term outcomes of stenting small coronary vessels in children. Further studies, preferably with a larger number of patients and in a multi-center setting, are required to evaluate the long-term outcomes of CSI in children with CAO. The inherent small sample size of this study limits further analyses of risk factors and longitudinal outcomes. Additionally, no universal recommendations exist concerning coronary interventional techniques and strategies leading to an individual approach, which challenges the establishment of guidelines.
Conclusions
CSI is a feasible and acceptable treatment consideration in infants and children with coronary artery stenosis after cardiac surgery. Despite the lower overall survival rates due to the severity of the underlying disease, technical difficulties, and limited therapeutic options, CSI can be performed with excellent procedural success, and it may play an essential role in the management of post-surgical coronary artery stenosis.
Acknowledgments
Funding: None.
Footnote
Reporting Checklist: The authors have completed the STROBE reporting checklist. Available at https://cdt.amegroups.com/article/view/10.21037/cdt-23-54/rc
Data Sharing Statement: Available at https://cdt.amegroups.com/article/view/10.21037/cdt-23-54/dss
Peer Review File: Available at https://cdt.amegroups.com/article/view/10.21037/cdt-23-54/prf
Conflicts of Interest: All authors have completed the ICMJE uniform disclosure form (available at https://cdt.amegroups.com/article/view/10.21037/cdt-23-54/coif). The authors have no conflicts of interest to declare.
Ethical Statement: The authors are accountable for all aspects of the work in ensuring that questions related to the accuracy or integrity of any part of the work are appropriately investigated and resolved. The study was conducted in accordance with the Declaration of Helsinki (as revised in 2013). The study was approved by the ethical committee of King Abdulaziz University Hospital (No. 539-20), King Faisal Specialist Hospital and Research Center (No. 2021-17), and
Open Access Statement: This is an Open Access article distributed in accordance with the Creative Commons Attribution-NonCommercial-NoDerivs 4.0 International License (CC BY-NC-ND 4.0), which permits the non-commercial replication and distribution of the article with the strict proviso that no changes or edits are made and the original work is properly cited (including links to both the formal publication through the relevant DOI and the license). See: https://creativecommons.org/licenses/by-nc-nd/4.0/.
References
- Goldsmith MP, Allan CK, Callahan R, et al. Acute coronary artery obstruction following surgical repair of congenital heart disease. J Thorac Cardiovasc Surg 2020;159:1957-1965.e1. [Crossref] [PubMed]
- Legendre A, Losay J, Touchot-Koné A, et al. Coronary events after arterial switch operation for transposition of the great arteries. Circulation 2003;108:II186-90. [Crossref] [PubMed]
- Mirkovic T, Knezevic I, Brecelj A, et al. Extrinsic left main compression: review of the literature. J Interv Cardiol 2009;22:550-5. [Crossref] [PubMed]
- Schleiger A, Kramer P, Dreysse S, et al. Coronary Interventions in Pediatric Congenital Heart Disease. Pediatr Cardiol 2022;43:769-75. [Crossref] [PubMed]
- El-Segaier M, Lundin A, Hochbergs P, et al. Late coronary complications after arterial switch operation and their treatment. Catheter Cardiovasc Interv 2010;76:1027-32. [Crossref] [PubMed]
- Bergersen L, Marshall A, Gauvreau K, et al. Adverse event rates in congenital cardiac catheterization - a multi-center experience. Catheter Cardiovasc Interv 2010;75:389-400. [PubMed]
- Feltes TF, Bacha E, Beekman RH 3rd, et al. Indications for cardiac catheterization and intervention in pediatric cardiac disease: a scientific statement from the American Heart Association. Circulation 2011;123:2607-52. [Crossref] [PubMed]
- Bonhoeffer P, Bonnet D, Piéchaud JF, et al. Coronary artery obstruction after the arterial switch operation for transposition of the great arteries in newborns. J Am Coll Cardiol 1997;29:202-6. [Crossref] [PubMed]
- Bratincsák A, Salkini A, El-Said HG, et al. Percutaneous stent implantation into coronary arteries in infants. Catheter Cardiovasc Interv 2012;79:303-11. [Crossref] [PubMed]
- Chaszczewski KJ, Nicholson GT, Shahanavaz S, et al. Stent Angioplasty for Post-Operative Coronary Artery Stenosis in Infants. World J Pediatr Congenit Heart Surg 2022;13:203-7. [Crossref] [PubMed]
- Bentall H, De Bono A. A technique for complete replacement of the ascending aorta. Thorax 1968;23:338-9. [Crossref] [PubMed]
- Yang CK, Jang WS, Choi ES, et al. The clinical outcomes of damus-kaye-stansel procedure according to surgical technique. Korean J Thorac Cardiovasc Surg 2014;47:344-9. [Crossref] [PubMed]
- Winlaw DS, Greenberg JW, O’Donnell AP. The Ross and Ross-Konno Operation in Neonates and Infants. Oper Tech Thorac Cardiovasc Surg 2022;27:423-46. [Crossref]
- Kampmann C, Kuroczynski W, Trübel H, et al. Late results after PTCA for coronary stenosis after the arterial switch procedure for transposition of the great arteries. Ann Thorac Surg 2005;80:1641-6. [Crossref] [PubMed]
- Abdelmohsen G, Al-Ata J, Alkhushi N, et al. Cardiac Catheterization During Extracorporeal Membrane Oxygenation After Congenital Cardiac Surgery: A Multi-Center Retrospective Study. Pediatr Cardiol 2022;43:92-103. [Crossref] [PubMed]
- Ino T, Iwahara M, Boku H, et al. Aortocoronary bypass surgery for Kawasaki disease. Pediatr Cardiol 1987;8:195-7. [Crossref] [PubMed]
- Moore JW, Buchbinder M. Successful coronary stenting in a 4-year-old child. Cathet Cardiovasc Diagn 1998;44:202-5. [Crossref] [PubMed]
- Sinelnikov YS, Kornilov IA, Redkin DA, et al. Left main coronary artery stenting in a neonate after arterial switch operation for transposition of the great arteries. World J Pediatr Congenit Heart Surg 2014;5:105-9. [Crossref] [PubMed]
- El-Said HG, Ebrahim M, Moore JW. Left main coronary artery stenting in a 3.6 kg infant after arterial switch operation for transposition of the great arteries. Ann Pediatr Cardiol 2015;8:143-6. [Crossref] [PubMed]
- Chrysant GS, Balzer D, Taniuchi M. Left main stem coronary artery stenting in a 3-month-old child after anomalous left coronary artery from pulmonary artery repair. Pediatr Cardiol 2005;26:734-6. [Crossref] [PubMed]
- Callahan R, Lock JE, Shah PB, et al. Transcatheter Intervention of Coronary Obstructions in Infants, Children, and Young Adults. Pediatr Cardiol 2018;39:1299-307. [Crossref] [PubMed]
- Bonnet D, Bonhoeffer P, Piéchaud JF, et al. Long-term fate of the coronary arteries after the arterial switch operation in newborns with transposition of the great arteries. Heart 1996;76:274-9. [Crossref] [PubMed]
- Tanel RE, Wernovsky G, Landzberg MJ, et al. Coronary artery abnormalities detected at cardiac catheterization following the arterial switch operation for transposition of the great arteries. Am J Cardiol 1995;76:153-7. [Crossref] [PubMed]
- King SB 3rd. Optimizing antiplatelet therapy in coronary interventions. Clin Cardiol 2000;23:VI-8-13. [Crossref] [PubMed]