Chronologic evolution and prognostic implications of impaired coronary flow after chronic total occlusion angioplasty
Highlight box
Key findings
• Coronary angiography derived-index of microcirculatory resistance was elevated in patients with slow flow (SF) phenomenon, indicating impaired microvascular function after chronic total occlusion (CTO) revascularization. Old myocardial infarction, right coronary artery intervention and bilateral collateral circulation were independent predictors of SF.
What is known and what is new?
• Vessels distal to a total occlusion have the potential to recover after successful recanalization. However, coronary slow flow would still increase the risk of restenosis and compromise cardiac systolic function recovery.
What is the implication, and what should change now?
• Interventional cardiologists ought to pay more attention to the restoration of microvascular function besides recanalization to improve the outcomes of CTO percutaneous coronary intervention (PCI). Further studies are needed to confirm the long-term prognostic impact of SF after PCI for CTO in a larger population.
Introduction
Background
At present, chronic total occlusion (CTO) revascularization is the most challenging task in percutaneous coronary intervention (PCI). With improvements in equipment, innovative techniques and operating experience, the success rate of PCI for CTO has substantially increased (1,2). However, the incidence of target lesion failure remains high even with the deployment of drug-eluting stents (3,4). Controversial results on the outcomes of PCI for CTO have been recently reported (5-7). The prognostic importance of CTO recanalization is still a subject of debate. Methods of ensuring the benefits of PCI for CTO are needed.
Rationale and knowledge gap
The slow flow (SF) phenomenon has been frequently reported after PCI, thus leading to myocardial injury and poor prognosis (8). In previous publications, impaired coronary flow after PCI for CTO was correlated with an increased risk of adverse cardiovascular events (9,10). Increasing coronary flow through procedural perfection or medical therapy seems promising for ameliorating a poor long-term prognosis. Notably, the mechanisms underlying SF in vessels distal to total occlusion probably differ from non-total lesions, while few data exist. Some studies have demonstrated that lumen diameter, blood flow and microvascular function recover over a series of months after successful CTO recanalization (11,12). This implies that coronary flow is likely to be in a dynamic condition after PCI for CTO. However, large-scale studies investigating the chronological evolution and prognostic significance of SF do not exist.
Objective
In this study, we aimed to investigate the incidence, influence factors and evolution of SF after CTO revascularization, and to reveal the prognostic implications of SF in the long term. We present this article in accordance with the STROBE reporting checklist (available at https://cdt.amegroups.com/article/view/10.21037/cdt-23-161/rc).
Methods
Population and data collection
Between 2015 and 2020, 514 patients who underwent a successful PCI for CTO and consecutive angiography at Zhongshan Hospital, Fudan University, were consecutively included. Follow-up angiography after a period of 9 to 12 months was routinely recommended after successful recanalization. Among the included patients, 14 patients whose angiograms were unsuitable for caIMR (coronary angiography-derived index of microcirculatory resistance) measurement were excluded, and 500 patients were ultimately included in the final cohort (Figure 1). Demographic, angiographic and procedural data were prospectively collected through an electronic medical record system and analyzed retrospectively. This study was approved by the Ethics Committee of Zhongshan Hospital, Fudan University (No. B2016-018) and conducted in accordance with the guidelines of the Declaration of Helsinki (as revised in 2013). All participants provided written informed consent at the time of hospitalization.
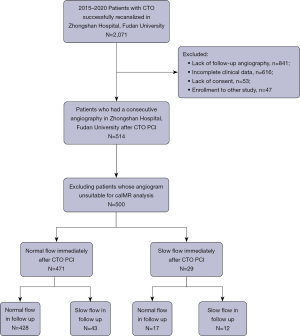
Angiography and intervention
PCI for CTO was performed by a single or double access site from the radial and/or femoral artery according to current algorithms (13,14). Tactics, strategy, use of device and concurrent treatment of non-total lesions were left to the discretion of the operators. In this study, the hybrid strategy was defined as a combination of antegrade and retrograde approaches, which was not identified until the retrograde wire successfully crossed collaterals. Angiograms at the first hospitalization and follow-up were reviewed by at least two qualified cardiologists at the angiographic core laboratory. Images recorded at different frame frequencies were standardized to 30 frames per second. The Thrombolysis in Myocardial Infarction (TIMI) frame count was calculated from the first frame when contrast fully entered the ostium of the artery to the frame when contrast opacified the distal target branch. The distal target branches were defined as follows: the distal bifurcation of the left anterior descending artery (LAD); the distal bifurcation of the longest branch in the left circumflex artery (LCX); and the first branch of the posterolateral artery in the right coronary artery (RCA). Films reviewed for TIMI flow assessment and TIMI frame count were taken after the vasodilator was given. Frame counts in the LAD were divided by 1.7 to correct for its longer length (15).
Study definitions
CTO was defined as 100% occlusion of a coronary artery with TIMI 0 flow for a duration of ≥3 months based on clinical, angiographic, or electrocardiographic criteria (16). Old myocardial infarction (OMI) indicated prior myocardial infraction at least one month before the index procedure, which not specifically occurred in the CTO territory. The perfusion of the occluded artery segment was assessed using the Rentrop classification (17). The J-CTO (Multicenter CTO Registry in Japan) score was calculated by assigning 1 point to each of the following factors: blunt proximal cap, presence of calcification, bend >45°, occlusion length ≥20 mm, and previously failed attempt (18). Side-branch occlusion was identified as TIMI flow grade ≤1 in branches ≥1.5 mm. Periprocedural myocardial infarction (PMI) was defined according to the fourth universal definition of myocardial infarction (UDMI) (19). Binary restenosis was defined as stenosis >50% in the target lesion. Technical success, defined as residual stenosis <30% within the target lesion, residual restenosis < 50% in the target vessel and TIMI flow grade ≥2, was achieved in all cases immediately after CTO PCI, and patients were further classified according to CTFC. Angiograms immediately after PCI for CTO and at the follow up were assessed. A corrected TIMI frame count (CTFC) >27 was defined as SF (15). According to the status of blood flow (normal or slow) on the two consecutive angiographies, patients were further classified into four groups: consistent normal flow, normal-to-slow flow, slow-to-normal flow and consistent SF.
caIMR measurements
Technical advances enable us to derive an index of microcirculatory resistance from angiography with high accuracy compared with conventional methods (20,21). caIMR was calculated retrospectively in an independent core laboratory using commercial software (FlashAngio, Rainmed Ltd., Suzhou, China) (22). The coronary mean flow velocity in diastole is assumed to be proportional to that in hyperemia. Therefore, caIMR was estimated in the following steps. First, a 3-dimensional mesh model was built from the ostium to the distal segment of the target coronary artery to determine the path length and lumen size using at least two angiographic views separated by at least 30°. Second, resting flow velocity was determined using the TIMI frame count method, and the mean aortic pressure recorded during the operation was input to the FlashAngio console. Pdhyp(mean arterial pressure at the distal segment during hyperemia) was estimated by computational pressure-flow dynamic simulation. Vdiastole (flow velocity during hyperemia) was computed in FlashAngio software based on resting flow velocity and proprietary parameters. Finally, caIMR was calculated using the following equation:
L represents the vessel length between the inlet and the distal segment of the target vessel. K was the constant used to adjust the difference in hyperemic flow velocity under different states of illness (20).
Statistical analysis
Statistical analysis was performed with R software (version 4.1.3) and SPSS version 25.0 (IBM, Armonk, New York, USA). Patients with missing data were excluded as shown in Figure 1. Discrete variables are presented as counts (percentage) and were compared by the chi-square test or Fisher’s exact test. Normally distributed continuous variables were reported as the means ± standard deviations (SD) and compared with Student’s t-test; non-normally distributed continuous variables were summarized as the median with interquartile range (IQR) and compared with the Mann-Whitney U test. Paired tests were conducted using paired t-tests and Wilcoxon signed-rank tests, as appropriate. Comparisons among three groups were performed by analysis of variance (ANOVA) or the Kruskal-Wallis H test. The odds ratio (OR) was calculated by univariate and multivariate logistic regression. Correlations between continuous values and endpoints were evaluated using spline curve analysis. Two hypotheses were tested in this study, that is, there is no statistical difference in the long-term outcome between patient with and without SF after CTO PCI (H0), and there is a significant difference between patient with and without SF in relation to the occurrence of PMI, target lesion failure or systolic dysfunction (H1). A two-tailed, P value <0.05 was considered statistically significant.
Results
Baseline characteristics
Of the 500 patients included in the final cohort, 85.4% were male with a median age of 63.0 (IQR, 54.0–69.0) years (Table 1). TIMI flow grade ≥2, residual stenosis within the target lesion <30% and residual stenosis in the target vessel <50% were achieved in all patients after CTO recanalization. Based on CTFC, 29 (5.8%) patients had SF immediately after PCI for CTO. Compared with the normal group, patients with SF were more likely to have a history of OMI and failed occlusion revascularization. The prevalence of other traditional risk factors, such as hypertension, diabetes, smoking and dyslipidemia, was comparable between the two groups. No significant difference was found concerning the presentation and high-sensitivity cardiac troponin T (hs-cTnT) at the first admission. However, hs-cTnT values after PCI were substantially elevated in the SF group [0.133 (0.074–0.331) vs. 0.067 (0.030–0.178) ng/mL, P=0.011].
Table 1
Risk factors | Total (n=500) | Normal flow (n=471) | Slow flow (n=29) | P value |
---|---|---|---|---|
Age (years) | 63.0 [54.0, 69.0] | 63.0 [54.0, 69.0] | 59.0 [50.0, 65.0] | 0.062 |
Male | 427 (85.4) | 399 (84.7) | 28 (96.6) | 0.102 |
Hypertension | 330 (66.0) | 309 (65.6) | 21 (72.4) | 0.453 |
Diabetes mellitus | 170 (34.0) | 162 (34.4) | 8 (27.6) | 0.453 |
Smoking history | 258 (51.6) | 242 (51.4) | 16 (55.2) | 0.692 |
OMI | 136 (27.2) | 123 (26.1) | 13 (44.8) | 0.028* |
Prior PCI | 152 (30.4) | 140 (29.7) | 12 (41.4) | 0.185 |
Prior CABG | 19 (3.8) | 17 (3.6) | 2 (6.9) | 0.369 |
Prior failed attempt | 115 (23.0) | 104 (22.1) | 11 (37.9) | 0.049* |
Stroke | 33 (6.6) | 31 (6.6) | 2 (6.9) | 0.947 |
BMI (kg/m2) | 25.4 [23.4, 27.1] | 23.3 [25.4, 27.1] | 25.5 [23.6, 28.7] | 0.514 |
Follow-up period (months) | 13 [11, 17] | 13 [11, 16] | 13 [12, 17] | 0.357 |
Presentation at the first admission | ||||
Stable angina | 392 (78.4) | 370 (78.6) | 22 (75.9) | 0.732 |
Unstable angina | 50 (10.0) | 48 (10.2) | 2 (6.9) | 0.566 |
NSTEMI | 47 (9.4) | 43 (9.1) | 4 (13.8) | 0.404 |
STEMI | 11 (2.2) | 10 (2.1) | 1 (3.4) | 0.485 |
Presentation in follow up | ||||
Stable angina | 482 (96.4) | 453 (96.2) | 27 (93.1) | 0.325 |
Unstable angina | 13 (2.6) | 13 (2.8) | 1 (3.4) | 0.572 |
NSTEMI | 5 (1.0) | 5 (1.1) | 1 (3.4) | 0.303 |
STEMI | 0 (0.0) | 0 (0.0) | 0 (0.0) | – |
Laboratory and auxiliary examinations | ||||
Creatinine (μmol/L) | 81 [71, 91] | 81 [71, 91] | 82 [74, 90] | 0.646 |
hs-CRP (mg/L) | 1.50 [0.60, 4.20] | 1.50 [0.60, 4.18] | 1.60 [0.50, 6.48] | 0.905 |
White blood cell count (×109/L) | 6.46 [5.48, 7.60] | 6.43 [5.47, 7.57] | 6.68 [5.41, 8.17] | 0.389 |
Platelet (×109/L) | 202 [165, 234] | 202 [164, 234] | 198 [166, 226] | 0.876 |
Hemoglobin (g/L) | 140 [130, 149] | 139 [129, 148] | 143 [139, 154] | 0.010* |
Total cholesterol (mmol/L) | 3.64 [3.04, 4.45] | 3.65 [3.02, 4.45] | 3.56 [3.26, 4.29] | 0.962 |
Low density lipoprotein (mmol/L) | 1.87 [1.33, 2.56] | 1.88 [1.31, 2.56] | 1.80 [1.54, 2.46] | 0.747 |
Triglyceride (mmol/L) | 1.59 [1.12, 2.30] | 1.57 [1.10, 2.30] | 1.66 [1.23, 2.25] | 0.758 |
High density lipoprotein (mmol/L) | 0.96 [0.81, 1.12] | 0.96 [0.80, 1.12] | 0.96 [0.84, 1.09] | 0.664 |
Lp (a) (mg/L) | 179 [82, 439] | 177 [79, 438] | 192 [94, 671] | 0.366 |
HbA1c (%) | 6.0 [5.6, 6.8] | 6.0 [5.6, 6.8] | 5.9 [5.5, 7.1] | 0.694 |
Pre-PCI hs-cTnT (ng/mL) | 0.011 [0.008, 0.024] | 0.011 [0.008, 0.024] | 0.014 [0.009, 0.033] | 0.570 |
Post-PCI hs-cTnT (ng/mL) | 0.072 [0.031, 0.183] | 0.067 [0.030, 0.178] | 0.133 [0.074, 0.331] | 0.011* |
Left ventricular ejection fraction (%) | ||||
Baseline | 59.9±9.2 | 60.0±9.2 | 58.4±10.4 | 0.336 |
Follow-up | 60.5±8.2 | 60.6±8.2 | 59.0±8.9 | 0.282 |
Anti-platelet therapy at discharge | ||||
Aspirin | 455 (91.0) | 429 (91.1) | 26 (89.7) | 0.794 |
Clopidogrel | 379 (75.8) | 358 (76.0) | 21 (72.4) | 0.661 |
Ticagrelor | 121 (24.2) | 113 (24.0) | 8 (27.6) | 0.661 |
Cilostazol | 45 (9.0) | 42 (8.9) | 3 (10.3) | 0.737 |
Data are shown as median [interquartile range], n (%) or mean ± standard deviations. *, P<0.05. OMI, old myocardial infarction; PCI, percutaneous coronary intervention; CABG, coronary artery bypass grafting; BMI, body mass index; NSTEMI, non-ST-segment elevation myocardial infarction; STEMI, ST-segment elevation myocardial infarction; hs-CRP, high-sensitivity C-reactive protein; Lp (a), lipoprotein (a); HbA1c, hemoglobin A1c; hs-cTnT, high-sensitivity cardiac troponin T.
Angiographic and procedural features
Although the Rentrop classification assessing the perfusion provided by collaterals was not significantly different between the two groups, bilateral collateral circulation was more prevalent in the normal flow group (46% vs. 24%, P=0.018) (Figure 2). The procedure was generally accomplished using an antegrade approach (78.4%) and the hybrid strategy (18.6%), accompanied by a wire escalation technique (81.7%). We did not find a significant difference in the strategy or tactics used in PCI for CTO. RCA was more commonly recanalized in the SF group (72.4% vs. 40.1%, P=0.003), while the percentage of PCI for LAD was lower (13.8% vs. 43.7%, P=0.002) (Table 2). The incidence of collateral perforation was higher (10.3% vs. 1.1%, P=0.008) in the SF group, but the differences in other complications (dissection/hematoma and side-branch loss) were not significant. After CTO PCI, patients with SF had prominently increased microvascular resistance [32.2 (22.9–41.5) vs. 17.3 (13.2–22.5), P<0.001].
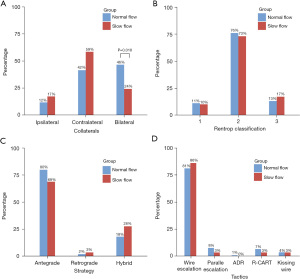
Table 2
Risk factors | Total (n=500) | Normal flow (n=471) | Slow flow (n=29) | P value |
---|---|---|---|---|
Syntax score | 22.5 [16.5, 27.0] | 22.5 [17.0, 27.0] | 20.0 [11.5, 25.5] | 0.094 |
Target vessel | ||||
Left anterior descending artery | 210 (42.0) | 206 (43.7) | 4 (13.8) | 0.002* |
Left circumflex artery | 80 (16.0) | 76 (16.1) | 4 (13.8) | 0.738 |
Right coronary artery | 210 (42.0) | 189 (40.1) | 21 (72.4) | 0.003* |
J-CTO score | 2 [2, 3] | 2 [2, 3] | 2 [2, 3] | 0.779 |
Dual angiography | 249 (49.8) | 233 (49.5) | 16 (55.2) | 0.551 |
Use of rotablator | 7 (1.3) | 6 (1.3) | 1 (3.4) | 0.344 |
Intra-stent occlusion | 42 (8.4) | 38 (8.1) | 4 (13.8) | 0.281 |
Stent length (mm) | 62.5 [40.3, 86.0] | 64.0 [44.0, 86.0] | 56.0 [26.0, 73.5] | 0.053 |
Average stent diameter (mm) | 2.83 [2.64, 3.04] | 2.84 [2.63, 3.03] | 2.83 [2.60, 3.38] | 0.284 |
Minimal stent diameter (mm) | 2.50 [2.50, 2.75] | 2.50 [2.50, 2.75] | 2.75 [2.50, 3.00] | 0.418 |
Side-branch loss | 6 (1.2) | 5 (1.1) | 1 (3.4) | 0.303 |
Dissection/hematoma | 9 (1.8) | 8 (1.7) | 1 (3.4) | 0.419 |
Collateral perforation | 8 (1.6) | 5 (1.1) | 3 (10.3) | 0.008* |
Contrast volume (mL) | 250 [200, 350] | 250 [200, 350] | 220 [180, 325] | 0.368 |
Immediately after CTO PCI | ||||
caIMR | 17.5 [13.3, 23.0] | 17.3 [13.2, 22.5] | 32.2 [22.9, 41.5] | <0.001* |
CTFC | 14 [10, 18] | 13 [9, 16] | 33 [28, 38] | <0.001* |
Follow-up | ||||
Binary target lesion restenosis | 93 (18.6) | 83 (17.6) | 10 (34.5) | 0.024* |
Absolute diameter stenosis** (%) | 17.74±34.35 | 16.95±33.69 | 30.69±42.25 | 0.047* |
CTFC | 16 [12, 20] | 16 [12, 20] | 24 [15, 39] | <0.001* |
Data are shown as median [IQR 25, 75] or n (%) or mean ± standard deviations. *, P<0.05; **, the most severe residual stenosis in the target vessel after the procedure. CTO, chronic total occlusion; PCI, percutaneous coronary intervention; caIMR, coronary angiography derived-index of microcirculatory resistance; CTFC, corrected Thrombolysis in Myocardial Infarction frame count; IQR, interquartile range.
Predictors of SF
Univariate and multivariate logistic models were constructed to identify predictors of SF. As shown in Figure 3, OMI and recanalization in RCA were independent risk factors for SF, while bilateral collaterals were protective against SF following CTO PCI. To investigate the reason RCA was associated with increased risk of SF after the procedure, patients were regrouped according to the target vessel. Demographics and procedural characteristics are summarized in Tables S1,S2. It was demonstrated that in patients accepting RCA recanalization, the incidence of prior coronary artery bypass grafting (CABG) and prior failed attempts was higher (both P<0.05). In addition, procedures using wire escalation were less commonly performed (LAD: 82.9% vs. LCX: 91.3% vs. RCA: 76.7%, P=0.014), while more procedures were accomplished using reverse controlled antegrade and retrograde subintimal tracking (R-CART) (LAD: 5.7% vs. LCX: 1.3% vs. RCA: 9.0%, P=0.046). Stent length was substantially longer in RCA {LAD: 61 [42–71] mm vs. LCX: 45 [33–60] mm vs. RCA: 84 [64–108] mm, P<0.001}. Besides, dissection/hematoma occurred more frequently following PCI (LAD: 0.5% vs. LCX: 1.3% vs. RCA: 3.3%, P=0.030). Of note, the average stent diameter and minimal stent diameter were slightly larger (both P<0.001).
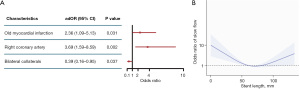
Stent length was not a significant factor influencing SF in binary logistic regression. Otherwise, spline curve analysis revealed that the relationship between stent length and the OR was not linear (Figure 3B). Deployment of a stent that was too long or too short would increase the risk of SF. Among the 14 patients who underwent percutaneous coronary angioplasty (PTCA) without stenting, 4 (28.6%) patients had SF. All 4 patients underwent RCA intervention with an average diameter less than 2.50 mm and an average length greater than 38.0 mm in the target lesions, and 3 of them had total reocclusion during follow up (data not shown).
Follow-up of coronary flow
The median interval of follow-up was 13 (IQR, 11–17) months. Fifty-five (11.0%) patients had SF at the follow-up. According to the blood flow on the two consecutive angiographies, patients were further classified into 4 groups: consistent normal flow (n=428, 85.6%), normal-to-slow flow (n=43, 8.6%), slow-to-normal flow (n=17, 3.4%) and consistent SF (n=12, 2.4%). Clinical, angiographic and procedural statistics are summarized in Tables S3,S4. In accordance with previous results, RCA was the dominant target vessel in patients with consistent SF and normal-to-slow flow (P<0.01).
Prognosis and SF
According to the fourth UDMI, 246 (49.2%) patients had PMI after PCI for CTO. After adjustment, SF was independently associated with PMI [adjusted odds ratio (adOR): 4.12; 95% confidence interval (CI): 1.68–10.07; P=0.002] (Table S5). Absolute diameter stenosis was significantly more severe in patients with impaired coronary flow (Table 2, Table S4). Overall, the incidence of binary restenosis in the target lesion was 18.6%. Notably, the rates of binary restenosis in patients with baseline SF, normal-to-slow flow and consistent SF were 34.5%, 69.8%, and 66.7% in comparison with 17.6%, 12.6% and 11.8% in patients with baseline normal flow, consistent normal flow and slow-to-normal flow, respectively. The association between flow status and occurrence of restenosis was displayed in Figure 4. As shown in Table 3, SF (adOR: 2.50; 95% CI: 1.10–5.72; P=0.030), female sex (adOR: 2.19; 95% CI: 1.21–3.96; P=0.010), prior history of percutaneous or surgical revascularization (adOR: 2.08; 95% CI: 1.29–3.35; P=0.003) and low-density lipoprotein (LDL) levels at follow-up (adOR: 1.34; 95% CI: 1.02–1.75; P=0.034) were independent risk factors for restenosis. Of note, it was revealed that a >15% reduction in LDL from baseline values would decrease the risk of target lesion restenosis (OR: 0.59; 95% CI: 0.37–0.94; P=0.026).
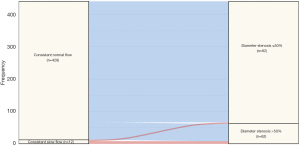
Table 3
Risk factors | Unadjusted | Adjusted | |||
---|---|---|---|---|---|
Odds ratio (95% CI) | P value | Odds ratio (95% CI) | P value | ||
Female | 1.96 (1.11–3.45) | 0.020 | 2.19 (1.21–3.96) | 0.010 | |
Age | 1.02 (1.00–1.04) | 0.119 | – | – | |
Hypertension | 0.89 (0.56–1.42) | 0.622 | – | – | |
Diabetes mellitus | 0.95 (0.59–1.52) | 0.817 | – | – | |
Smoking history | 0.79 (0.50–1.24) | 0.303 | – | – | |
OMI | 1.03 (0.62–1.70) | 0.912 | – | – | |
Prior revascularization* | 2.01 (1.27–3.18) | 0.003 | 2.08 (1.29–3.35) | 0.003 | |
Stent length (per 10 mm increase) | 1.04 (0.97–1.12) | 0.256 | – | – | |
Attained target lesion residual diameter | 1.01 (0.96–1.03) | 0.664 | – | – | |
Syntax score | 0.98 (0.96–1.01) | 0.208 | – | – | |
Re-vascularizing strategy | 1.23 (0.71–2.15) | 0.460 | – | – | |
Dissection/hematoma | 3.56 (0.94–13.54) | 0.062 | – | – | |
Slow flow after the procedure | 2.43 (1.09–5.40) | 0.030 | 2.50 (1.10–5.72) | 0.030 | |
caIMR after the procedure | 1.01 (0.98–1.03) | 0.513 | – | – | |
LDL at follow-up | 1.39 (1.07–1.81) | 0.013 | 1.34 (1.02–1.75) | 0.034 |
*, including percutaneous coronary intervention and surgical intervention. caIMR, coronary angiography derived-index of microcirculatory resistance; CI, confidence interval; OMI, old myocardial infarction; LDL, low density lipoprotein.
It seems that echocardiographic parameters, such as the left ventricular ejection fraction (LVEF), did not substantially improve after CTO PCI and showed no difference between patients with or without SF. Herein, we conducted pairwise analysis including only those with a baseline LVEF ≤60%. In patients with normal flow immediately after PCI for CTO, there was a significant improvement in LVEF (55.7%±9.3% vs. 51.6%±8.5%, P<0.001) in comparison with patients with SF (55.4%±9.6% vs. 52.1%±9.4%, P=0.147), mainly due to a decreased left ventricular end systolic diameter (LVESD) (36.3±7.2 vs. 39.3±6.8, P=0.006). Patients who had slow-to-normal flow did not benefit from CTO recanalization, and in patients with consistent SF, there was even a trend of decreased LVEF. In contrary, LVEF could still be improved in patients with normal flow after CTO PCI even if they developed SF at follow-up.
Discussion
Key findings
In the present study, we demonstrated that SF was a common phenomenon after PCI for CTO associated with OMI, RCA intervention and bilateral collateral circulation. Although coronary flow could recover over time, impaired flow immediately after the procedure was associated with increased risk of PMI, target lesion failure and cardiac systolic dysfunction.
Explanations of findings and comparison with previous researches
Over the past years, the clinical benefits of PCI for CTO have received increasing attention (5,6). Symptom relief seems to be the primary indication of PCI for CTO (23). However, Schumacher et al. reported that the magnitude of improvement in myocardial perfusion played a pivotal role in the long-term outcomes of PCI for CTO (7). These differences inspire researchers to investigate methods of conducting the procedure in a beneficial way in respect of the excessive operative time and financial cost during CTO revascularization (24). SF frequently occurs after PCI and is correlated with poor outcomes (25). Ischemia-reperfusion injury, vessel wall damage and microembolization caused by plaque debris during angioplasty could contribute to impaired coronary blood flow (26). Overall, the antegrade, retrograde, or hybrid strategy nor specific wire crossing techniques significantly influenced coronary flow after the procedure in this study. This could be limited by the fact that only patients who had a consecutive follow-up angiography after PCI for CTO in our center were recruited rather than all patients who underwent PCI for CTO. However, this implied that not the specific techniques or guide wire entering the subintimal space, but controlling the length of false lumen and the preservation of the distal vessels were more crucial for the restoration of normal flow. Stent length and SF were correlated; that is, stents that were too short or too long would increase the risk of SF. Longer stenting and high prevalence of complications would increase the risk of side-branch loss, vessel wall damage and plaque debris embolization, which could impair blood flow. The main reasons for implanting less stents were small vessels with long lesions and long segment in the false lumen. Impaired coronary flow without stenting was associated with a profoundly high rate of reocclusion (3 out of 4). While excessive stenting could impair small vessel function, partially due to side-branch loss and plaque debris embolization, and increase the risk of mismatch between the stents and the detracted vessel lumen.
In addition, there are unique features of the vessel distal to a total occlusion influencing coronary flow. Endothelial and smooth muscle cell dysfunction were observed in the coronary segment distal to a CTO, leading to compromised antegrade blood flow and was thus negatively correlated with the grade of collateral circulation (27). We found that caIMR was profoundly elevated in the SF group after PCI for CTO. However, this could be a consequence of both the complex intervention and intrinsic microvascular dysfunction. The ameliorated microvascular dysfunction by well-developed collaterals could account for our findings that bilateral collateral circulation was protective against SF after CTO PCI. From another point of view, these data implied that recanalization of a complex CTO unsuitable for stenting in the presence of low-grade collaterals could bear a high risk of SF and poor outcomes.
We collected the data about how SF was treated during the procedure. In our center, nitroglycerin intra-coronary injection was the most common treatment, followed by verapamil and nitroprusside intra-coronary injection. However, many patients received more than one drug treatment, making the assessment of which drug was more effective confounded. The efficacy of these drugs was not the focus of this study, and we aimed to analyze the long-term prognostic impact of SF.
Positive vascular remodeling and vascular function improvement could occur after successful PCI for CTO (11,12). In addition, collateral circulation would also regress over time (28). These results indicated that the recanalized vessel had great potential to recover on its own, which may reduce the demand for coronary flow immediately after CTO PCI. In this study, we demonstrated that SF was an independent risk factor for target lesion restenosis. Residual stenosis after PCI for CTO was slightly more severe in the SF group, but it was not significantly associated with the occurrence of restenosis. Further assessment found that more than half of the patients with baseline SF would become normal in follow-up, and those with consistent SF were truly disposed to target lesion restenosis. The exact etiology between SF and target lesion failure was not fully understood, however, impaired shear stress, activation of inflammation reaction within the vessel could be part of the explanation.
Implications and actions needed
Coronary flow had the potential to recover after recanalization of CTO, however, this was far from enough to allow interventional cardiologists to tolerate the existence of impaired coronary flow. Evaluation of the echocardiography implied that improvement in systolic function (LVEF and LVESD) was compromised in the SF group. These results implied not merely the target lesion failure, but the function of microvascular circulation played a crucial role in the rehabilitation of the myocardium. Restoring normal vascular physiology could be more essential than solely achieving an open artery. Moreover, improvements in LVEF and LVESD were discovered among patients with a baseline LVEF ≤60%. This was an arbitrary cutoff value that indicated that perhaps patients with more severe systolic dysfunction, that is, more ischemic burden caused by CTO, would benefit from recanalization (29). The precise threshold needs to be validated in future, larger-scale studies.
Strengths and limitations
This study had several strengths. Compared with previous publications, our study included patients who had both the index and follow-up angiograph in our center, which guaranteed the quality of angiograms and precise assessment of coronary flow and the extent of restenosis. Besides, core laboratory were used in this study to ensure the objective review of angiograms. Third, the functional status of coronary microcirculation was evaluated by TIMI flow grade and caIMR, which made the results comprehensive and plausible.
There are some limitations of this study. First, this was a single-center retrospective study with a moderate sample size. As mentioned above, only patients who underwent consecutive surveillance angiography in our center were included, and the results need to be evaluated in a larger complex population. Second, in the present study, caIMR measurement could only be used in vessels with stenosis <50%. We did not report the results of caIMR during follow up because data from patients with restenosis did not exist. Third, there were patients with a normal baseline flow who deteriorated into SF at follow-up. These patients had an increased incidence of restenosis, some of whom developed a severely narrowed lumen with substantially impaired coronary flow. However, in this group, we could not verify whether SF or restenosis occurred first or dominated in the pathogenesis due to the limitations of this study. In addition, patients with SF also had profound diameter restenosis. Whether SF (i.e., microvascular dysfunction) or stenosis played a causal role in impeding systolic function recovery requires further investigation. Moreover, pressure wire-based IMR is the gold standard to assess the function of microcirculation. caIMR we used in this study has been validated by prior publications, which prove to be accurate and reliable as a surrogate for conventional IMR. Still, it hasn’t been broadly validated like the original method. Our findings would inspire prospective studies using pressure wire-based IMR in the future. Finally, we mainly used angiography and echocardiography to assess the prognostic benefits of PCI for CTO. Integration of more examinations, such as positron emission tomography perfusion imaging and cardiac magnetic resonance imaging, could provide more information, which also brings considerable expenses.
Conclusions
SF is a common phenomenon after PCI for CTO that holds profound prognostic significance. Adequate preoperative evaluation, optimized final coronary flow and restored vascular physiology would contribute to the improved prognosis of CTO recanalization.
Acknowledgments
Funding: This study was supported by the National Program on Key Basic Research Project of China (No. 2019YFC0840601), National Natural Science Foundation of China (Nos. 82170338, 81970295, 81870267, 81521001 and 81670318), Shanghai Clinical Research Center for Interventional Medicine (No. 19MC1910300), Grant of Shanghai Shenkang on Key Clinical Research Project (Nos. SHDC2020CR2015A and SHDC12019104), Grant of Shanghai Science and Technology Committee (No. 20JC1410800), Key Medical and Health Projects of Xiamen City (No. 3502Z20204004), and Grant of Zhongshan Hospital Affiliated to Fudan University (Nos. 2018ZSLC01 and 2021ZSGG07).
Footnote
Reporting Checklist: The authors have completed the STROBE reporting checklist. Available at https://cdt.amegroups.com/article/view/10.21037/cdt-23-161/rc
Data Sharing Statement: Available at https://cdt.amegroups.com/article/view/10.21037/cdt-23-161/dss
Peer Review File: Available at https://cdt.amegroups.com/article/view/10.21037/cdt-23-161/prf
Conflicts of Interest: All authors have completed the ICMJE uniform disclosure form (available at https://cdt.amegroups.com/article/view/10.21037/cdt-23-161/coif). The authors have no conflicts of interest to declare.
Ethical Statement: The authors are accountable for all aspects of the work in ensuring that questions related to the accuracy or integrity of any part of the work are appropriately investigated and resolved.
Open Access Statement: This is an Open Access article distributed in accordance with the Creative Commons Attribution-NonCommercial-NoDerivs 4.0 International License (CC BY-NC-ND 4.0), which permits the non-commercial replication and distribution of the article with the strict proviso that no changes or edits are made and the original work is properly cited (including links to both the formal publication through the relevant DOI and the license). See: https://creativecommons.org/licenses/by-nc-nd/4.0/.
References
- Brilakis ES, Mashayekhi K, Tsuchikane E, et al. Guiding Principles for Chronic Total Occlusion Percutaneous Coronary Intervention. Circulation 2019;140:420-33. [Crossref] [PubMed]
- Tajti P, Karmpaliotis D, Alaswad K, et al. The Hybrid Approach to Chronic Total Occlusion Percutaneous Coronary Intervention: Update From the PROGRESS CTO Registry. JACC Cardiovasc Interv 2018;11:1325-35. [Crossref] [PubMed]
- Azzalini L, Dautov R, Ojeda S, et al. Procedural and Long-Term Outcomes of Percutaneous Coronary Intervention for In-Stent Chronic Total Occlusion. JACC Cardiovasc Interv 2017;10:892-902. [Crossref] [PubMed]
- Kwon O, Lee PH, Lee SW, et al. Retrograde approach for the percutaneous recanalisation of coronary chronic total occlusions: contribution to clinical practice and long-term outcomes. EuroIntervention 2019;15:e354-e361. [Crossref] [PubMed]
- Lee SW, Lee PH, Ahn JM, et al. Randomized Trial Evaluating Percutaneous Coronary Intervention for the Treatment of Chronic Total Occlusion. Circulation 2019;139:1674-83. [Crossref] [PubMed]
- Werner GS, Martin-Yuste V, Hildick-Smith D, et al. A randomized multicentre trial to compare revascularization with optimal medical therapy for the treatment of chronic total coronary occlusions. Eur Heart J 2018;39:2484-93. [Crossref] [PubMed]
- Schumacher SP, Stuijfzand WJ, de Winter RW, et al. Ischemic Burden Reduction and Long-Term Clinical Outcomes After Chronic Total Occlusion Percutaneous Coronary Intervention. JACC Cardiovasc Interv 2021;14:1407-18. [Crossref] [PubMed]
- Kawai Y, Hisamatsu K, Matsubara H, et al. Intravenous administration of nicorandil immediately before percutaneous coronary intervention can prevent slow coronary flow phenomenon. Eur Heart J 2009;30:765-72. [Crossref] [PubMed]
- Guan C, Yang W, Song L, et al. Association of Acute Procedural Results With Long-Term Outcomes After CTO PCI. JACC Cardiovasc Interv 2021;14:278-88. [Crossref] [PubMed]
- Wang Y, Zhao HW, Wang CF, et al. Incidence, Predictors, and Prognosis of Coronary Slow-Flow and No-Reflow Phenomenon in Patients with Chronic Total Occlusion Who Underwent Percutaneous Coronary Intervention. Ther Clin Risk Manag 2020;16:95-101. [Crossref] [PubMed]
- Keulards DCJ, Karamasis GV, Alsanjari O, et al. Recovery of Absolute Coronary Blood Flow and Microvascular Resistance After Chronic Total Occlusion Percutaneous Coronary Intervention: An Exploratory Study. J Am Heart Assoc 2020;9:e015669. [Crossref] [PubMed]
- Okuya Y, Saito Y, Takahashi T, et al. Novel predictors of late lumen enlargement in distal reference segments after successful recanalization of coronary chronic total occlusion. Catheter Cardiovasc Interv 2019;94:546-52. [Crossref] [PubMed]
- Galassi AR, Werner GS, Boukhris M, et al. Percutaneous recanalisation of chronic total occlusions: 2019 consensus document from the EuroCTO Club. EuroIntervention 2019;15:198-208. [Crossref] [PubMed]
- Harding SA, Wu EB, Lo S, et al. A New Algorithm for Crossing Chronic Total Occlusions From the Asia Pacific Chronic Total Occlusion Club. JACC Cardiovasc Interv 2017;10:2135-43. [Crossref] [PubMed]
- Gibson CM, Cannon CP, Daley WL, et al. TIMI frame count: a quantitative method of assessing coronary artery flow. Circulation 1996;93:879-88. [Crossref] [PubMed]
- Ybarra LF, Rinfret S, Brilakis ES, et al. Definitions and Clinical Trial Design Principles for Coronary Artery Chronic Total Occlusion Therapies: CTO-ARC Consensus Recommendations. Circulation 2021;143:479-500. [Crossref] [PubMed]
- Rentrop KP, Cohen M, Blanke H, et al. Changes in collateral channel filling immediately after controlled coronary artery occlusion by an angioplasty balloon in human subjects. J Am Coll Cardiol 1985;5:587-92. [Crossref] [PubMed]
- Morino Y, Abe M, Morimoto T, et al. Predicting successful guidewire crossing through chronic total occlusion of native coronary lesions within 30 minutes: the J-CTO (Multicenter CTO Registry in Japan) score as a difficulty grading and time assessment tool. JACC Cardiovasc Interv 2011;4:213-21. [Crossref] [PubMed]
- Thygesen K, Alpert JS, Jaffe AS, et al. Fourth Universal Definition of Myocardial Infarction (2018). J Am Coll Cardiol 2018;72:2231-64. [Crossref] [PubMed]
- Li J, Gong Y, Wang W, et al. Accuracy of computational pressure-fluid dynamics applied to coronary angiography to derive fractional flow reserve: FLASH FFR. Cardiovasc Res 2020;116:1349-56. [Crossref] [PubMed]
- Choi KH, Dai N, Li Y, et al. Functional Coronary Angiography-Derived Index of Microcirculatory Resistance in Patients With ST-Segment Elevation Myocardial Infarction. JACC Cardiovasc Interv 2021;14:1670-84. [Crossref] [PubMed]
- Ai H, Feng Y, Gong Y, et al. Coronary Angiography-Derived Index of Microvascular Resistance. Front Physiol 2020;11:605356. [Crossref] [PubMed]
- Azzalini L, Karmpaliotis D, Santiago R, et al. Contemporary Issues in Chronic Total Occlusion Percutaneous Coronary Intervention. JACC Cardiovasc Interv 2022;15:1-21. [Crossref] [PubMed]
- Salisbury AC, Karmpaliotis D, Grantham JA, et al. In-Hospital Costs and Costs of Complications of Chronic Total Occlusion Angioplasty: Insights From the OPEN-CTO Registry. JACC Cardiovasc Interv 2019;12:323-31. [Crossref] [PubMed]
- Ndrepepa G, Tiroch K, Fusaro M, et al. 5-year prognostic value of no-reflow phenomenon after percutaneous coronary intervention in patients with acute myocardial infarction. J Am Coll Cardiol 2010;55:2383-9. [Crossref] [PubMed]
- Piana RN, Paik GY, Moscucci M, et al. Incidence and treatment of 'no-reflow' after percutaneous coronary intervention. Circulation 1994;89:2514-8. [Crossref] [PubMed]
- Brugaletta S, Martin-Yuste V, Padró T, et al. Endothelial and smooth muscle cells dysfunction distal to recanalized chronic total coronary occlusions and the relationship with the collateral connection grade. JACC Cardiovasc Interv 2012;5:170-8. [Crossref] [PubMed]
- Lee JH, Kim CY, Kim N, et al. Coronary Collaterals Function and Clinical Outcome Between Patients With Acute and Chronic Total Occlusion. JACC Cardiovasc Interv 2017;10:585-93. [Crossref] [PubMed]
- Mashayekhi K, Nührenberg TG, Toma A, et al. A Randomized Trial to Assess Regional Left Ventricular Function After Stent Implantation in Chronic Total Occlusion: The REVASC Trial. JACC Cardiovasc Interv 2018;11:1982-91. [Crossref] [PubMed]