Prediction of left ventricular ejection fraction improvement in patients with ischemic cardiomyopathy after coronary artery bypass grafting based on cardiac magnetic resonance
Highlight box
Key findings
• A predictive model was developed to identify patients with improved left ventricular ejection fraction (LVEF) after coronary artery bypass grafting (CABG).
What is known and what is new?
• The number of transmural scar segments and left ventricular end-systolic volume index (LVESVI) were independent factors in predicting the LVEF improvement after CABG.
• The prediction model based on the number of transmural scar segments and LVESVI has good diagnostic efficacy.
What is the implication, and what should change now?
• Our findings help to identify patients with improved LVEF and thus guide the selection of clinical treatment strategies.
• Potential treatments following the non-improvement of LVEF mainly include: guideline-directed medical therapy, cardiac resynchronization therapy, implantable cardioverter defibrillator, heart transplant, or mechanical heart.
Introduction
Background
Ischemic cardiomyopathy (ICM) is a common cardiovascular disease with high morbidity and mortality (1). Coronary artery bypass grafting (CABG) is the main treatment method for ICM (2). However, these patients still have a high incidence of adverse cardiovascular events (3,4). In previous studies, left ventricular ejection fraction (LVEF) for 17.6–63.0% of ICM patients had not improved after revascularization, and the non-improvement was closely associated with poor prognostic outcomes (5-7). Therefore, it is important to identify patients with improved LVEF after CABG.
Rationale and knowledge gap
Evaluation of myocardial viability can predict LVEF improvement after revascularization (8). Revascularization of viable and dysfunctional myocardium may improve its systolic functions, while extensive infarcted myocardial regions are unlikely to benefit from revascularization (9,10). Although stress echocardiography, single-photon emission computed tomography (SPECT), and positron emission tomography (PET) can be used to assess myocardial ischemia and viability, cardiac magnetic resonance-late gadolinium enhancement (CMR-LGE) is the gold standard for detecting myocardial scar tissues caused by previous myocardial infarctions (11-13).
Objective
The objective of this study was to evaluate the relationship between myocardial scar and LVEF non-improvement after CABG by CMR-LGE and to develop a predictive model. We present this article in accordance with the STROBE reporting checklist (available at https://cdt.amegroups.com/article/view/10.21037/cdt-23-220/rc).
Methods
Patient enrollment
This was a single center, retrospective, observational cohort study. Patients who had been diagnosed with ICM and undergone CMR-LGE examination at Beijing Anzhen Hospital, Capital Medical University from January 2017 to June 2022 were continuously enrolled in this study. The inclusion criteria were: (I) transthoracic resting echocardiography revealed LVEF ≤40%. In cases of multiple preoperative echocardiographic results, the last preoperative result prevailed; (II) patients who had been diagnosed with coronary heart disease by percutaneous coronary angiography and required CABG surgery; (III) patients who had been subjected to CMR-LGE examination before operation; (IV) echocardiographic evaluation of LVEF at 6 months after surgery; (V) coronary computed tomography angiography (CTA) revealed patency of the graft during postoperative follow-up; and (VI) patients with complete clinical data. The exclusion criteria were: (I) patients with a history of acute myocardial infarction in the past 3 months; (II) simultaneous combinations of other cardiac surgeries (e.g., aortic valve, mitral valve, tricuspid valve, congenital heart disease, macrovascular disease, resection of ventricular aneurysm); (III) patients with preoperative arrhythmias, such as atrial fibrillation; (IV) patients with preoperative malignant tumors; (V) emergency surgery due to cardiogenic shock before surgery; (VI) patients with poor CMR-LGE image quality that could not be analyzed.
Study protocol
Clinical baseline characteristics for patients, including demographic information, echocardiography, and CMR-LGE data were collected retrospectively. Follow-up information from patients were collected through phone, WeChat or outpatient services. Based on outcomes of thoracic echocardiography 6 months after surgery, patients were assigned into the LVEF improvement and LVEF non-improvement groups. LVEF improvement is defined as a 5% increase in LVEF absolute value compared to preoperative values (ΔLVEF ≥5%), on the contrary, LVEF is not improved (14,15). Baseline characteristics for the two groups were determined and compared, after which the risk factors for the post-CABG LVEF non-improvement were analyzed. The study was conducted in accordance with the Declaration of Helsinki (as revised in 2013). The study was approved by Beijing Anzhen Hospital, Capital Medical University Ethics Committee (No. 2021104X). The requirement for informed consent was waived because this was a retrospective observational study that did not require specific information about patients. Data collection and analysis personnel are not clear about the grouping.
Collection of CMR images
At 1 week before CABG, patients underwent CMR-LGE examination, and images were collected in supine positions. Images were obtained using the German Siemens Verio 3.0T superconducting magnetic resonance system, with a 32-channel phased array coil dedicated to the heart. The maximum scan gradient was 45 mT/m, while the maximum climb rate was 200 mT/(m·s). All sequences were electrocardiographically gated. Image acquisition included: (I) functional/morphological CMR cine and (II) infarction quantitative CMR-LGE.
Cinematic scans for cardiac functions/morphology were performed using breath-holding balanced steady state free precession (SSFP) sequences to obtain the following cine CMR images: standard long axis views (4, 3, and 2 chambers) and continuous short axis coverage of the entire left ventricle from the level of the mitral annulus to the apex, to assess overall and local ventricular functions. Imaging parameters were: repetition time (TR) 3.50 ms; echo time (TE) 1.51 ms; the field of view (FOV) was 340 mm × 289 mm. The long axis was 5 mm, the short axis was 8 mm, while the average temporal resolution was 40 ms (16).
After intravenous injection of 0.1 mmol/kg Gadopentetate dimeglumine contrast agent for about 10 min, delayed enhancement imaging of the heart was performed using phase sensitive inversion recovery (PSIR) magnetic moment pre-preparation rapid small angle excitation (Turbo-FLASH) sequence. The parameters were: TR/TE 4.1 ms/1.56 ms; imaging FOV 350 mm2, matrix 2.1 mm × 1.4 mm × 5.0 mm, turning angle 35°, acceleration factor 2. The left ventricular short axis imaging layer was 8 mm thick with an interval of 0 mm; thickness of the imaging layer of the left ventricular two and four chambers was 5 mm, with an interval of 0 mm. The scanning layer was consistent with cine scanning layer. First, the optimal T1 time during delayed scan imaging was determined for all patients to suppress normal myocardial signals for scanning (17).
Post-processing and analysis of the CMR image
The commercial CVI 42 (Circle Cardiovascular Imaging Inc., Calgary, Canada) cardiovascular post-processing software was used to analyze left ventricular myocardial activities. The analyses were conducted by experienced radiologists with professional titles of associate chief physicians or above (more than 5 years of CMR experience) who were not aware of patients’ clinical data and grouping information.
The LVEF and ventricular volumes were analyzed based on continuous short axis cine images. During diastole and systole, endocardial and epicardial edges of the left ventricular wall were depicted on CMR cine images. The conventional indicators for measuring left ventricular structure and functions are: LVEF, left ventricular end-diastolic volume (LVEDV), left ventricular end-systolic volume (LVESV), left ventricular stroke volume (LVSV), and left ventricular cardiac output (LVCO). Then, LVEDV and LVESV are divided by BSA to determine the left ventricular end-diastolic volume index (LVEDVI) and left ventricular end-systolic volume index (LVESVI). Due to the absence of routine examination of postoperative CMR in this study, the postoperative LVEF value measured by CMR could not be obtained, thus, the LVEF measured by echocardiography was used.
Based on the 17-segment segmentation method of the heart proposed by the American Heart Association and the American Society of Cardiology (AHA/ACA) (18), along its long axis, the left ventricle can be divided into basal, central, and apical segments. The basal and central segments can each be divided into 6 segments at 60° per segment on the short axis level, while the apical segments can be divided into 4 segments at 90° per segment, which together form 17 segments with the apex without a ventricular cavity. Given that the apex is too thin to evaluate, 16 segments were analyzed after subtracting the apical segment from 17 segments.
In the PSIR sequence, the endocardial and epicardial membranes (except papillary muscles) were delineated at the short-axis level, and the insertion points of the ventricular septum were identified at the short-axis level, and the areas of interest of normal myocardium were delineated. Normal myocardium was defined as no LGE and far from the LGE region, while scar myocardium was defined as the LGE region. The LGE region was defined as the myocardial gray threshold being 5 standard deviations above the mean value of normal myocardium.
The left ventricular was analyzed by segment while the myocardial segment was divided into five levels according to LGE penetration degree (19): LGE =0, LGE =1–25%, LGE =26–50%, LGE =51–75%, and LGE =76–100%. The greater the degree of LGE penetration through the wall, the less likely it is to improve myocardial segment functions after revascularization, therefore, segments with LGE =76–100% were defined as transmural scar segments. To quantify the scar size of the entire left ventricular myocardium, a software was used to calculate the end diastolic epicardial volume, the endocardial volume was subtracted, and multiplied by 1.05 g/cm3 to calculate the LV mass and LGE mass. Then, the LGE mass was divided by LV mass to calculate the myocardial scar size of the entire left ventricular myocardium (LGE mass/LV mass × 100%).
Surgical operation
All patients received CABG and general anesthesia through tracheal intubation. Then, they were placed in the supine position and subjected to median sternal incisions. The decision to perform CABG without extracorporeal or extracorporeal circulation was informed by the general condition of each patient, cardiac function, hemodynamic status after anesthesia, and the experience of the surgeon in charge. The internal thoracic artery was obtained by ossification or pedicled techniques, while the great saphenous vein and radial artery were obtained using open techniques. The anterior descending branch, circumflex branch, and right coronary artery were sequentially revascularized. All patients achieved anatomical complete revascularization (20), implying that coronary angiography suggests at least one posture, and revascularization was performed on blood vessels with diameters of >1.5 mm and stenosis of ≥50%. This is because complete revascularization can significantly reduce the frequency of angina pectoris and the incidence of adverse events in patients with coronary heart disease, and improve the quality of life (21,22). The instantaneous blood flow measurement technique was used to assess the quality of grafts anastomosis. A graft flow rate with a pulsatile index >5 and/or an average graft flow rate <10 mL/min was defined as non-functional graft anastomosis (23). For non-functional grafts, there is the need to re-anastomose them to achieve satisfactory quality of graft anastomosis. After surgery, all patients received guided anti heart failure medication (24,25).
Patient follow-up
After surgery, patients were regularly followed up at 3 and 6 months, and then every 6 months thereafter. If a patient exhibited heart failure or coronary heart disease symptoms during follow-up, a clinical follow-up was conducted at that time. The follow-up period ends in December 2022. The follow-up mainly assessed the improvement of LVEF, the New York heart association (NYHA) cardiac function grading and incidence of major adverse cardiovascular and cerebrovascular events (MACCE). LVEF improvement was defined as ΔLVEF ≥5%, as revealed by chest echocardiography 6 months after CABG (14,15). In this study, MACCE included: all cause death, myocardial infarction, cerebral infarction, and re-admission due to heart failure. Coronary CTA was used to assess the patency of the grafts. As defined by the FitzGibbon classification system (26), patency of the grafts was evaluated, with FitzGibbon-A as patency and FitzGibbon-B/O as occlusion. All patient data were obtained from an online database and collected using a standardized data collection table. Data collection was completed by trained staff who were unaware of the purpose of the study.
Statistical analysis
The Student’s t-test was used to analyze normally distributed measurement data, expressed as mean ± standard deviation. The Mann-Whitney U test was used to analyze the non-normally distributed measurement data, expressed as medians (M) and interquartile ranges [M (P25, P75)]. The Chi-square test or Fisher’s exact test were used for counting data, expressed as frequencies (rates). The rank sum test was used for rank data, expressed as frequencies (rates). Univariate logistic regression analysis was performed to assess the relationships between various variables and unimproved LVEF. Variables with P<0.05 in univariate logistics regression analysis and those clinically considered to be closely related to the end point event were included in multivariate logistic regression analysis. The stepwise regression forward or backward method was further used to screen the variables and to finally determine the independent risk factors that predicted the unimproved LVEF. Least absolute shrinkage and selection operator (LASSO) regression was also applied to the selection of predictors to test the importance of the selected predictors in the stepwise regression analysis. Based on the selected variables, a logistic regression model is established and expressed in the nomogram.
Bootstrap method (repeated sampling 200 times) was used to verify the model internally. ROC curve and calibration curve were used to verify the model’s differentiation and calibration. Decision curve analysis (DCA) was used to determine the net benefits of the model under different threshold probabilities, and DCA curves were drawn. The MACCE-free survival curve of the two groups was calculated using the Kaplan-Meier method. Comparisons of survival curves of the two groups was performed using the log-rank test. All statistical tests were conducted using a two-tailed method, with P<0.05 as the threshold for significance. Data analyses were performed using SPSS 23.0 (IBM), Stata 16 and R softwares.
Results
Baseline information
We continuously enrolled 240 patients diagnosed with ICM and subjected to CMR-LGE examination at Beijing Anzhen Hospital, Capital Medical University from January 2017 to June 2022. Out of the 240 patients, 42 received medications, 50 underwent mitral valve surgery, 5 underwent tricuspid valve surgery, and 23 underwent resection of ventricular aneurysm. A total of 120 patients underwent CABG alone. Among them, 5 died during the perioperative period, 1 died within 6 months after surgery, 3 patients were lost within 6 months after surgery, 3 patients had grafts occlusion within 6 months after surgery, and scar myocardium could not be accurately assessed in 2 patients. Therefore, 106 patients who met the inclusion criteria were included in this study (Figure 1).
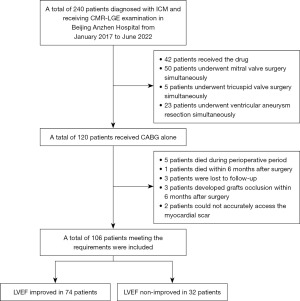
Based on echocardiographic results 6 months after surgery, the 106 patients were assigned into the LVEF improvement group [74 patients (69.8%)] and the LVEF non-improvement group [32 patients (30.2%)]. For the enrolled cases, the average age was 61.3±8.6 years (range, 39–80 years), and 83.0% (88/106) of them were male. Differences in age, gender, body mass index (BMI), body surface area (BSA), past medical history, or NYHA grading (P>0.05 in all cases) between the groups were insignificant (Table 1).
Table 1
Variables | LVEF improvement group (n=74) | LVEF non-improvement group (n=32) | P value |
---|---|---|---|
Age (years) | 61.3±9.3 | 61.3±6.8 | 0.987 |
Male | 59 (79.7) | 29 (90.6) | 0.170 |
BMI (kg/m2) | 25.2±3.3 | 25.2±3.2 | 0.941 |
BSA (m2) | 1.79±0.18 | 1.80±0.22 | 0.914 |
Past medical history | |||
Hypertension | 40 (54.1) | 20 (62.5) | 0.421 |
Diabetes | 31 (41.9) | 12 (37.5) | 0.672 |
Hyperlipidemia | 43 (58.1) | 16 (50.0) | 0.440 |
Smoking | 44 (59.5) | 15 (46.9) | 0.231 |
Drinking | 22 (29.7) | 12 (37.5) | 0.431 |
Renal insufficiency | 4 (5.4) | 1 (3.1) | 0.992 |
Cerebral infarction | 8 (10.8) | 7 (21.9) | 0.231 |
COPD | 2 (2.7) | 1 (3.1) | >0.99 |
PCI | 7 (9.5) | 10 (31.3) | 0.005 |
NYHA grading | 0.461 | ||
I | 4 (5.4) | 1 (3.1) | |
II | 31 (41.9) | 12 (37.5) | |
III | 26 (35.1) | 12 (37.5) | |
IV | 13 (17.6) | 7 (21.9) | |
Laboratory tests | |||
BNP (pg/mL) | 278.5 (168.0, 480.0) | 357.5 (141.3, 681.3) | 0.322 |
Creatinine (μmol/L) | 81.5 (68.4, 94.4) | 83.0 (74.8, 94.7) | 0.296 |
The data are presented as mean ± standard deviation, n (%) or median (range). LVEF, left ventricular ejection fraction; BMI, body mass index; BSA, body surface area; COPD, chronic obstructive pulmonary disease; PCI, percutaneous coronary intervention; NYHA, New York Heart Association; BNP, B-type natriuretic peptide.
Preoperative imaging data
Preoperative imaging data showed that left ventricular end-diastolic diameter (LVEDD), LVEDVI and LVESVI of the LVEF non-improvement group were significantly higher than those of the LVEF improvement group (P<0.05). According to the CMR-LGE results, the number of segments with LGE ≤50%, the number of segments with LGE >50% and the number of segments with transmural scar in the LVEF non-improvement group were significantly higher than those in the LVEF improvement group (P<0.05). At the same time, myocardial scar size in the LVEF non-improvement group was also significantly higher than that in the LVEF improvement group (33.0%±5.5% vs. 39.1%±4.7%, P<0.001) (Table 2). Figure 2 shows the CMR-LGE image of patients with LVEF non-improvement in 6 months after CABG while Figure 3 shows the CMR-LGE image of patients with LVEF improvement in 6 months after CABG.
Table 2
Variables | LVEF improvement group (n=74) | LVEF non-improvement group (n=32) | P value |
---|---|---|---|
Echocardiogram | |||
LVEF (%) | 35.5±4.8 | 35.1±3.7 | 0.619 |
LVEDD (mm) | 57.7±6.1 | 60.8±6.8 | 0.025 |
LVESD (mm) | 45.1±7.0 | 48.0±8.3 | 0.065 |
Interventricular septum thickness (mm) | 9.8±1.9 | 9.2±1.7 | 0.098 |
Left ventricle posterior wall thickness (mm) | 8.3±1.7 | 8.5±1.2 | 0.560 |
Left atrial diameter (mm) | 40.5±5.0 | 42.6±4.6 | 0.052 |
Coronary angiography | 0.356 | ||
One vessel lesion | 2 (2.7) | 2 (6.2) | |
Two vessel lesion | 11 (14.9) | 6 (18.8) | |
Triple vessel lesion | 61 (82.4) | 24 (75.0) | |
Left main lesion | 19 (25.7) | 7 (21.9) | 0.676 |
SYNTAX score | 41.5±7.2 | 42.9±6.8 | 0.361 |
CMR cine | |||
LVEDVI (mL/m²) | 115.7±25.1 | 137.7±30.3 | <0.001 |
LVESVI (mL/m²) | 84.1±22.9 | 104.0±23.6 | <0.001 |
LVSV (mL) | 56.2±20.6 | 60.2±21.3 | 0.362 |
LVCO (L/min) | 3.9±1.3 | 4.1±1.3 | 0.607 |
CMR-LGE | |||
Number of segments with LGE ≤50% | 11.3±1.8 | 9.3±1.6 | <0.001 |
Number of segments with LGE >50% | 4.7±1.8 | 6.7±1.6 | <0.001 |
Number of segments with LGE =51–75% | 3.0±0.8 | 3.1±0.7 | 0.748 |
Number of transmural scar segments | 1.7±1.4 | 3.6±1.3 | <0.001 |
Myocardial scar size (%) | 33.0±5.5 | 39.1±4.7 | <0.001 |
The data are presented as mean ± standard deviation or n (%). LVEF, left ventricular ejection fraction; LVEDD, left ventricular end-diastolic diameter; LVESD, left ventricular end-systolic diameter; SYNTAX, Synergy between Percutaneous Coronary Intervention with Taxus and Cardiac Surgery; CMR, cardiac magnetic resonance; LVEDVI, left ventricular end-diastolic volume index; LVESVI, left ventricular end-systolic volume index; LVSV, left ventricular stroke volume; LVCO, left ventricular cardiac output; CMR-LGE, CMR-late gadolinium enhancement.
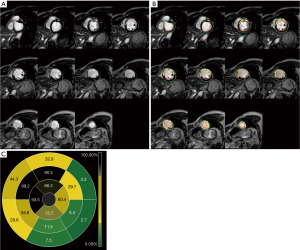
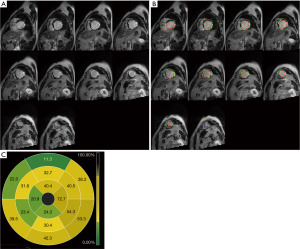
Surgical related information
Comparing the surgical data between the two group, there were no significant difference in the use of cardiopulmonary bypass and the utilization rate of left internal mammary artery between the two groups (79.7% vs. 78.1%, P=0.852; 83.8% vs. 71.9%, P=0.158), and there were no significant difference in the number of bypass grafts and surgical time between the two groups (3.4±0.7 vs. 3.5±0.7, P=0.344; 4.2±0.8 vs. 4.4±0.8 h, P=0.437). Moreover, there were no significant differences between the two groups in blood transfusion product dosage, intensive care unit (ICU) stay time, ventilator use time and postoperative hospital stay (all P>0.05, Table 3).
Table 3
Variables | LVEF improvement group (n=74) | LVEF non-improvement group (n=32) | P value |
---|---|---|---|
Off-pump coronary | 59 (79.7) | 25 (78.1) | 0.852 |
Left internal mammary artery | 62 (83.8) | 23 (71.9) | 0.158 |
Number of grafts (pcs) | 3.4±0.7 | 3.5±0.7 | 0.344 |
Operation duration (h) | 4.2±0.8 | 4.4±0.8 | 0.437 |
Suspended red blood cells (u) | 0 (0, 2) | 0 (0, 1.5) | 0.778 |
Plasma (mL) | 0 (0, 0) | 0 (0, 0) | 0.609 |
Platelet (u) | 0 (0, 0) | 0 (0, 0) | 0.604 |
ICU stay time (h) | 47.3 (27.0, 90.0) | 47.5 (24.9, 106.0) | 0.964 |
Ventilator use time (h) | 27.0 (21.0, 50.9) | 26.0 (20.0, 57.5) | 0.922 |
Postoperative hospitalization time (d) | 8.0 (7.0, 11.0) | 9.0 (7.0, 12.0) | 0.242 |
The data are presented as mean ± standard deviation, n (%) or median (range). LVEF, left ventricular ejection fraction; ICU, intensive care unit.
Predictive variable screening
Variables with univariate analysis P<0.05, including PCI history, LVEDD, LVEDVI, LVESVI, number of segments with LGE ≤50%, number of segments with LGE >50%, number of transmural scar segments, myocardial scar size, as well as variables clinically considered to be closely related to end events, including LVEF and LVESD were included in multivariate regression. It was found that the number of transmural scar segments and LVESVI were independent correlated factors affecting LVEF non-improvement in 6 months after CABG [odds ratio (OR) =2.398, 95% CI: 1.607–3.579, P<0.001; OR =1.036, 95% CI: 1.009–1.063, P=0.008] (Table 4). In addition, two predictive variables were also generated by LASSO regression, which were the same as those screened by stepwise regression method (Figure 4).
Table 4
Variables | Univariate analysis | Multivariate analysis | |||
---|---|---|---|---|---|
OR (95% CI) | P value | OR (95% CI) | P value | ||
PCI history | 4.351 (1.479–12.802) | 0.008 | – | – | |
LVEF | 0.977 (0.893–1.070) | 0.616 | – | – | |
LVEDD | 1.080 (1.008–1.157) | 0.028 | – | – | |
LVESD | 1.055 (0.996–1.118) | 0.067 | – | – | |
LVEDVI | 1.030 (1.012–1.047) | 0.001 | – | – | |
LVESVI | 1.037 (1.016–1.059) | <0.001 | 1.036 (1.009–1.063) | 0.008 | |
Number of segments with LGE ≤50% | 0.518 (0.383–0.700) | <0.001 | – | – | |
Number of segments with LGE >50% | 1.932 (1.430–2.611) | <0.001 | – | – | |
Number of transmural scar segments | 2.499 (1.700–3.674) | <0.001 | 2.398 (1.607–3.579) | <0.001 | |
Myocardial scar size (%) | 1.267 (1.139–1.410) | <0.001 | – | – |
OR, odds ratio; CI, confidence interval; PCI, percutaneous coronary intervention; LVEF, left ventricular ejection fraction; LVEDD, left ventricular end-diastolic diameter; LVESD, left ventricular end-systolic diameter; LVEDVI, left ventricular end-diastolic volume index; LVESVI, left ventricular end-systolic volume index; LGE, late gadolinium enhancement.
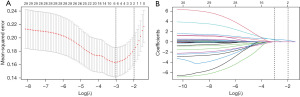
Model development and validation
The two selected predictive variables were used to develop a model for predicting the LVEF non-improvement in ICM patients after CABG, which was shown in the form of nomogram (Figure 5). The results of 200 resampling using bootstrap method are shown that the area under curve (AUC) of the nomogram was 0.866 (95% CI: 0.792–0.940), with sensitivity of 59.4%, specificity of 91.9%, positive predictive value of 76.0%, and negative predictive value of 84.0% (Figure 6A), and the calibration curve showed that the probability predicted by the nomogram was well matched with the clinical outcome (Figure 6B). The DCA showed that the model had potential clinical application value (Figure 6C). By comparing the distinction between LVESVI, the number of transmural scar segments and the nomogram, it was found that the diagnostic efficiency of nomogram was superior to the number of transmural scar segments and LVESVI, while the diagnostic efficiency of the number of transmural scar segments was superior to LVESVI (Figure 6D).
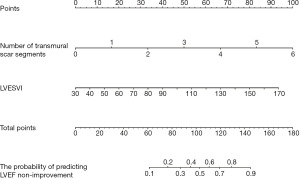
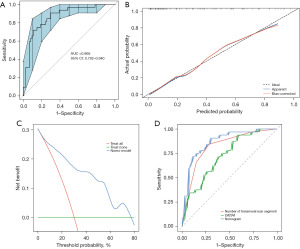
Follow-up
Echocardiography showed that 6 months after CABG, LVEF, LVEDD, LVESD, and left interventricular septum thickness in the LVEF improvement group were significantly improved, compared to the LVEF non-improvement group (P<0.05 in all cases). Meanwhile, 6 months after surgery, the NYHA level of the LVEF improvement group was lower, while heart failure symptoms were significantly alleviated (P=0.016). After an average follow-up time of 1.5 years (range, 0.5–4.1 years), four patients in the LVEF improvement group and eight patients in the LVEF non-improvement group developed MACCE, including one patient who died of myocardial infarction and one patient who died due to non-cardiac disease, one patient developed cerebral infarction, and one patient was re-admitted due to heart disease in the LVEF improvement group. The LVEF non-improvement group had six deaths due to heart failure and two readmissions due to heart disease. Kaplan-Meier survival analysis showed that incidences of MACCE in the LVEF non-improvement group were significantly lower than those of the LVEF improvement group (P=0.009) (Table 5, Figure 7).
Table 5
Variables | LVEF improvement group (n=74) | LVEF non-improvement group (n=32) | P value |
---|---|---|---|
Echocardiogram | |||
LVEF (%) | 46.0±6.6 | 37.7±3.2 | <0.001 |
LVEDD (mm) | 55.5±4.9 | 57.7±6.1 | 0.048 |
LVESD (mm) | 40.4±4.2 | 44.3±5.0 | <0.001 |
Interventricular septum thickness (mm) | 10.1±1.5 | 9.5±1.8 | 0.09 |
Left ventricle posterior wall thickness (mm) | 9.0±1.4 | 8.0±1.5 | 0.002 |
NYHA level | 0.016 | ||
I | 15 (20.2) | 4 (12.5) | |
II | 46 (62.2) | 15 (46.9) | |
III | 10 (13.5) | 7 (21.9) | |
IV | 3 (4.1) | 6 (18.7) | |
MACCE | 4 (5.4) | 8 (25.0) | 0.009 |
The data are presented as mean ± standard deviation or n (%). NYHA, New York Heart Association; MACCE, major adverse cardiovascular and cerebrovascular events; LVEF, left ventricular ejection fraction; LVEDD, left ventricular end diastolic diameter; LVESD, left ventricular end-systolic diameter.
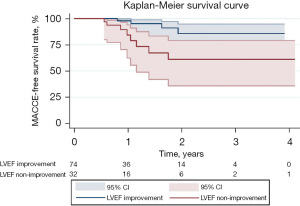
Discussion
Key findings
We found that 69.8% of ICM patients had improved their LVEF 6 months after CABG, while 30.2% of patients did not have improved LVEF. Second, the number of transmural scar segments and LVESVI were independent factors in predicting the LVEF improvement after CABG, and the nomogram based on these two factors showed good sensitivity, specificity, and clinical applicability. Third, LVEF non-improvement was associated with poor short-term and medium-term prognostic outcomes, while prognostic outcomes of the LVEF improvement group were superior to those of the LVEF non-improvement group. Our findings inform on identification of patients who can benefit from CABG.
Strengths and limitations
The prediction model based on the number of transmural scar segments and LVESVI has good diagnostic efficacy. Our findings help to identify patients with improved LVEF and thus guide the selection of clinical treatment strategies. This study has some limitations. Firstly, the model was developed using retrospective single-center data, and the sample size was small. In the next step, we will further expand the sample size and conduct multi-center, prospective verification. Secondly, scanning thickness in CMR affects the accurate measurement and calculation of myocardial scars to a certain extent, and affects the accuracy of predicting LVEF non-improvement. Thirdly, the exclusion of patients with cardiac resynchronization therapy (CRT) or implantable cardioverter defibrillator (ICD) and patients with claustrophobia may result in patient selection bias. Finally, patients were not re-examined for CMR before discharge and during follow-up after surgery. In the future, we will strengthen the CMR follow-up of these patients.
Comparison with similar researches
Patients with ICM exhibited varying degrees of post-CABG LVEF improvement. Pegg et al. (27) found that 63.6% (21/33) of patients had LVEF improvement 6 months after CABG; Yang et al. (14) reported that 61.5% (32/52) of patients had LVEF improvement 6 months after surgery; Hwang et al. (15) demonstrated that 73% (51/70) of patients had LVEF improvement one year after surgery. Nakae et al. (7) reported that the average postoperative period was 64.5±45.5 months, with about 60% of patients undergoing LVEF improvement. In this study, 69.8% of 106 ICM patients had LVEF improvement 6 months after CABG, while 30.2% did not have LVEF improvement.
Explanations of findings
Scarred myocardium is more accurate than viable myocardium in predicting LVEF improvement (11,28). Therefore, assessing the degree of myocardial scar can predict LVEF improvement. This could be attributed to various reasons: first, the normal functions of viable myocardium does not contribute to functional improvement after CABG; second, as a non-renewable cell, once a myocardial scar is formed, even if blood supply is restored, the function of the scar myocardium cannot be restored; third, the myocardial scar may affect peripheral myocardial movement, and tethering of myocardial scar tissues may offset the systolic improvement brought about by viable myocardium, thereby preventing the overall improvement of myocardial functions. In summary, myocardial scar degree may have a greater impact on LVEF improvement. Therefore, this study analyzed the transmural scar segment, rather than normal or viable segments.
Improvement of contractility of myocardial segment was negatively correlated with scar formation degree. Selvanayagam et al. (29) and Hwang et al. (30) showed that improvement of myocardial segment contractility is negatively correlated with scar formation degree, that is, the greater the degree of myocardial scar, the less likely the improvement of myocardial motor functions after revascularization. In this study, differences in the number of segments with preoperative LGE =51–75% between the two groups were insignificant, while the number of transmural scar segments (LGE =76–100%) in the LVEF non-improvement group was significantly larger than that of the LVEF improvement group. This was attributed to several factors. First, the possibility of improving myocardial segment functions of the non-transmural scar after revascularization is high, while functional improvement of the transmural scar segment is still difficult even if revascularization is restored; second, some segments have less or no LGE, and these segments may not show functional improvement after revascularization; third, occurrence of intraoperative myocardial infarction may be the cause of non-improvement of segmental functions; fourth, the adjacent viable myocardium of scar myocardium may be affected by poor motor functions of the scar myocardium, therefore, it does not show improvements in segmental functions.
Ventricular remodeling is a key process in the development of ICM. During ventricular remodeling, the shape of the left ventricle gradually changes from conical to elliptical or spherical, which, if uncontrolled, is associated with worsening cardiac function and poor prognosis. Conversely, reversing ventricular remodeling to more closely resemble normal heart shape is associated with improved cardiac function and better prognosis (31,32). Although CABG can reverse ventricular remodeling by improving myocardial blood supply, the degree of myocardial scarring is negatively correlated with the degree of ventricular remodeling reversal (33), especially for the myocardium with transmural scar cannot reverse ventricular remodeling even if the myocardial blood supply is improved. Although this study found that LVESVI and the number of transmural scar segments were independent factors affecting the LVEF non-improvement after CABG, the number of transmural scar segments with was superior to LVESVI in diagnostic efficacy.
Implications and actions needed
The prediction model based on the number of transmural scar segments and LVESVI has good diagnostic efficacy. Our findings help to identify patients with improved LVEF and thus guide the selection of clinical treatment strategies. Potential treatments following the non-improvement of LVEF mainly include: guideline-directed medical therapy (GDMT), CRT, ICD, heart transplant, or mechanical heart (2).
Conclusions
The prediction model based on the number of transmural scar segments and LVESVI has good diagnostic efficacy. Our findings help to identify patients with improved LVEF and thus guide the selection of clinical treatment strategies.
Acknowledgments
The authors would like to thank all the reviewers who participated in the review and MJEditor (www.mjeditor.com) for its linguistic assistance during the preparation of this manuscript.
Funding: This study was supported by the Beijing Nova Program (No. Z201100006820088, No. 20220484174), Beijing Natural Science Foundation (No. L222098, No. 7232041).
Footnote
Reporting Checklist: The authors have completed the STROBE reporting checklist. Available at https://cdt.amegroups.com/article/view/10.21037/cdt-23-220/rc
Data Sharing Statement: Available at https://cdt.amegroups.com/article/view/10.21037/cdt-23-220/dss
Peer Review File: Available at https://cdt.amegroups.com/article/view/10.21037/cdt-23-220/prf
Conflicts of Interest: All authors have completed the ICMJE uniform disclosure form (available at https://cdt.amegroups.com/article/view/10.21037/cdt-23-220/coif). K.Z., W.F., T.L., J.Z., Y.S., and R.D. report that the study was supported by the Beijing Nova Program (No. Z201100006820088, No. 20220484174), and Beijing Natural Science Foundation (No. L222098, No. 7232041). J.B. is an employee of the Circle Cardiovascular Imaging company. The other authors have no conflicts of interest to declare.
Ethical Statement: The authors are accountable for all aspects of the work in ensuring that questions related to the accuracy or integrity of any part of the work are appropriately investigated and resolved. The study was conducted in accordance with the Declaration of Helsinki (as revised in 2013). The study was approved by Beijing Anzhen Hospital, Capital Medical University Ethics Committee (No. 2021104X). The requirement for informed consent was waived because this was a retrospective observational study that did not require specific information about patients.
Open Access Statement: This is an Open Access article distributed in accordance with the Creative Commons Attribution-NonCommercial-NoDerivs 4.0 International License (CC BY-NC-ND 4.0), which permits the non-commercial replication and distribution of the article with the strict proviso that no changes or edits are made and the original work is properly cited (including links to both the formal publication through the relevant DOI and the license). See: https://creativecommons.org/licenses/by-nc-nd/4.0/.
References
- Savarese G, Becher PM, Lund LH, et al. Global burden of heart failure: a comprehensive and updated review of epidemiology. Cardiovasc Res 2023;118:3272-87. [Crossref] [PubMed]
- Bakaeen FG, Gaudino M, Whitman G, et al. 2021: The American Association for Thoracic Surgery Expert Consensus Document: Coronary artery bypass grafting in patients with ischemic cardiomyopathy and heart failure. J Thorac Cardiovasc Surg 2021;162:829-850.e1. [Crossref] [PubMed]
- Velazquez EJ, Lee KL, Jones RH, et al. Coronary-Artery Bypass Surgery in Patients with Ischemic Cardiomyopathy. N Engl J Med 2016;374:1511-20. [Crossref] [PubMed]
- Velazquez EJ, Lee KL, Deja MA, et al. Coronary-artery bypass surgery in patients with left ventricular dysfunction. N Engl J Med 2011;364:1607-16. [Crossref] [PubMed]
- Mandegar MH, Yousefnia MA, Roshanali F, et al. Interaction between two predictors of functional outcome after revascularization in ischemic cardiomyopathy: left ventricular volume and amount of viable myocardium. J Thorac Cardiovasc Surg 2008;136:930-6. [Crossref] [PubMed]
- Rizzello V, Poldermans D, Biagini E, et al. Prognosis of patients with ischaemic cardiomyopathy after coronary revascularisation: relation to viability and improvement in left ventricular ejection fraction. Heart 2009;95:1273-7. [Crossref] [PubMed]
- Nakae M, Kainuma S, Toda K, et al. Incidence, determinants and clinical impact of left ventricular function recovery after surgical treatments for ischaemic cardiomyopathy. Eur J Cardiothorac Surg 2021;60:689-96. [Crossref] [PubMed]
- Ryan M, Morgan H, Chiribiri A, et al. Myocardial viability testing: all STICHed up, or about to be REVIVED? Eur Heart J 2022;43:118-26. [Crossref] [PubMed]
- McDiarmid AK, Loh H, Nikitin N, et al. Predictive power of late gadolinium enhancement for myocardial recovery in chronic ischaemic heart failure: a HEART sub-study. ESC Heart Fail 2014;1:146-53. [Crossref] [PubMed]
- Sawada SG, Dasgupta S, Nguyen J, et al. Effect of revascularization on long-term survival in patients with ischemic left ventricular dysfunction and a wide range of viability. Am J Cardiol 2010;106:187-92. [Crossref] [PubMed]
- Dhore-Patil AS, Aneja A. Role of Cardiovascular Magnetic Resonance in Ischemic Cardiomyopathy. Heart Fail Clin 2021;17:41-56. [Crossref] [PubMed]
- Dang Y, Hou Y. The prognostic value of late gadolinium enhancement in heart diseases: an umbrella review of meta-analyses of observational studies. Eur Radiol 2021;31:4528-37. [Crossref] [PubMed]
- McDonagh TA, Metra M, Adamo M, et al. 2021 ESC Guidelines for the diagnosis and treatment of acute and chronic heart failure. Eur Heart J 2021;42:3599-726. [Crossref] [PubMed]
- Yang T, Lu MJ, Sun HS, et al. Myocardial scar identified by magnetic resonance imaging can predict left ventricular functional improvement after coronary artery bypass grafting. PLoS One 2013;8:e81991. [Crossref] [PubMed]
- Hwang HY, Yeom SY, Choi JW, et al. Cardiac Magnetic Resonance Predictor of Ventricular Function after Surgical Coronary Revascularization. J Korean Med Sci 2017;32:2009-15. [Crossref] [PubMed]
- Zhang C, Zhao L, Zhu E, et al. Predictors of moderate to severe ischemic mitral regurgitation after myocardial infarction: a cardiac magnetic resonance study. Eur Radiol 2021;31:5650-8. [Crossref] [PubMed]
- Zhang LJ, Tian JF, Yang XY, et al. Clinical value of left ventricular strain analysis by cardiovascular magnetic resonance in patients with coronary chronic total occlusion. Zhonghua Xin Xue Guan Bing Za Zhi 2021;49:601-9. [PubMed]
- Cerqueira MD, Weissman NJ, Dilsizian V, et al. Standardized myocardial segmentation and nomenclature for tomographic imaging of the heart. A statement for healthcare professionals from the Cardiac Imaging Committee of the Council on Clinical Cardiology of the American Heart Association. Int J Cardiovasc Imaging 2002;18:539-42. [PubMed]
- Di Bella G, Pizzino F, Aquaro GD, et al. CMR predictors of secondary moderate to severe mitral regurgitation and its additive prognostic role in previous myocardial infarction. J Cardiol 2022;79:90-7. [Crossref] [PubMed]
- Neumann FJ, Sousa-Uva M, Ahlsson A, et al. 2018 ESC/EACTS Guidelines on myocardial revascularization. Eur Heart J 2019;40:87-165. [Crossref] [PubMed]
- Mavromatis K, Jones PG, Ali ZA, et al. Complete Revascularization and Angina-Related Health Status in the ISCHEMIA Trial. J Am Coll Cardiol 2023;82:295-313. [Crossref] [PubMed]
- Bianco V, Kilic A, Aranda-Michel E, et al. Complete revascularization during coronary artery bypass grafting is associated with reduced major adverse events. J Thorac Cardiovasc Surg 2023;166:104-113.e5. [Crossref] [PubMed]
- Zhu E, Zhang C, Wang S, et al. The association between myocardial scar and the response of moderate ischemic mitral regurgitation to isolated coronary artery bypass grafting. Ann Transl Med 2021;9:1328. [Crossref] [PubMed]
- Heidenreich PA, Bozkurt B, Aguilar D, et al. 2022 AHA/ACC/HFSA Guideline for the Management of Heart Failure: A Report of the American College of Cardiology/American Heart Association Joint Committee on Clinical Practice Guidelines. J Am Coll Cardiol 2022;79:e263-421. [Crossref] [PubMed]
- Otto CM, Nishimura RA, Bonow RO, et al. 2020 ACC/AHA Guideline for the Management of Patients With Valvular Heart Disease: Executive Summary: A Report of the American College of Cardiology/American Heart Association Joint Committee on Clinical Practice Guidelines. Circulation 2021;143:e35-71. [Crossref] [PubMed]
- Fitzgibbon GM, Kafka HP, Leach AJ, et al. Coronary bypass graft fate and patient outcome: angiographic follow-up of 5,065 grafts related to survival and reoperation in 1,388 patients during 25 years. J Am Coll Cardiol 1996;28:616-26. [Crossref] [PubMed]
- Pegg TJ, Selvanayagam JB, Jennifer J, et al. Prediction of global left ventricular functional recovery in patients with heart failure undergoing surgical revascularisation, based on late gadolinium enhancement cardiovascular magnetic resonance. J Cardiovasc Magn Reson 2010;12:56. [Crossref] [PubMed]
- Doukas D, Porcaro K, Marot J, et al. Clinical characteristics and outcomes of patients with severe left ventricular dysfunction undergoing cardiac MRI viability assessment prior to revascularization. Int J Cardiovasc Imaging 2021;37:675-84. [Crossref] [PubMed]
- Selvanayagam JB, Kardos A, Francis JM, et al. Value of delayed-enhancement cardiovascular magnetic resonance imaging in predicting myocardial viability after surgical revascularization. Circulation 2004;110:1535-41. [Crossref] [PubMed]
- Hwang HY, Yeom SY, Park EA, et al. Serial cardiac magnetic resonance imaging after surgical coronary revascularization for left ventricular dysfunction. J Thorac Cardiovasc Surg 2020;159:1798-805. [Crossref] [PubMed]
- Aimo A, Gaggin HK, Barison A, et al. Imaging, Biomarker, and Clinical Predictors of Cardiac Remodeling in Heart Failure With Reduced Ejection Fraction. JACC Heart Fail 2019;7:782-94. [Crossref] [PubMed]
- Gibb AA, Hill BG. Metabolic Coordination of Physiological and Pathological Cardiac Remodeling. Circ Res 2018;123:107-28. [Crossref] [PubMed]
- Barison A, Aimo A, Ortalda A, et al. Late gadolinium enhancement as a predictor of functional recovery, need for defibrillator implantation and prognosis in non-ischemic dilated cardiomyopathy. Int J Cardiol 2018;250:195-200. [Crossref] [PubMed]