Minimally invasive treatments for venous compression syndromes
Introduction
Extrinsic compression of venous structures can be caused by a wide variety of anatomical variants throughout the body. While this phenomenon can be seen incidentally in asymptomatic patients, there are several known syndromes that can lead to significant morbidity if left untreated. These syndromes are relatively uncommon (less than 1% of the general population), and are most commonly seen in young and otherwise healthy individuals (1).
The pathophysiology is similar for all venous compression syndromes, with repetitive endothelial injury initiating the coagulation cascade, leading to acute thrombus. This may eventually progress to chronic deep vein thrombosis (DVT), venous occlusion or venous insufficiency (2).
The clinical features of venous compression syndromes vary based on their anatomical location and can include pain, swelling, varicosities and hematuria (2). Suspected venous compression syndrome can be evaluated with several noninvasive imaging modalities including ultrasonography, computed tomography (CT) with venography (CTV), and magnetic resonance imaging (MRI) with venography (MRV) (1). The gold standard in diagnosis is digital subtraction venography (DSV) with multiple projections and obliquities (1). Intravascular ultrasound (IVUS) is an additional imaging technique sometimes performed during angiography to aid in both diagnosis and treatment.
Conservative measures are typically first-line treatment in the majority of cases, but invasive operative management is often necessary to correct the underlying anatomical cause of venous compression (2). Endovascular treatment options offer a minimally invasive approach to select cases, with several reports of long-term efficacy demonstrated in the literature (1).
This article will discuss three of the most commonly encountered venous compression disorders, namely Paget-Schroetter syndrome (PSS), nutcracker syndrome and May-Thurner syndrome. The pathophysiology and clinical features of each syndrome are discussed, as well as the pertinent diagnostic findings seen with a variety of imaging modalities. The optimal management of each syndrome will be described, with particular emphasis on endovascular therapy based on our institutional experience and review of the literature.
PSS
PSS, also known as effort thrombosis, is a rare entity (estimated annual incidence of 1–2 cases per 100,000 in the general population) primarily seen in the dominant arm of young, physically active male patients (1,2). Anatomic abnormalities involving the thoracic outlet are thought to play a major role in the development of effort thrombosis. These include cervical ribs, congenital bands, hypertrophic scalene muscles or tendons, callus formation from prior fractures and abnormal insertion of the costoclavicular ligament (2,3). These conditions invoke a predisposition to subclavian vein compression exacerbated by vigorous and sustained upper extremity movements (2). As a consequence, repetitive microtrauma to the endothelium initiates the coagulation cascade and causes thrombosis (2,3). In addition, extrinsic compression of the vein directly impairs blood flow causing venous hypertension in the affected extremity and the development of collateral vessels in the neck and upper chest (2).
Effort thrombosis is usually symptomatic with swelling and arm discomfort being the most frequent complaints (3). Other symptoms include arm heaviness, erythema, cyanosis and varicosities across the shoulder and upper arm (3). Symptom onset is usually acute or subacute, and patients often report a recent sports-related over-exertion of the affected arm (3). Post-thrombotic syndrome is the major source of morbidity associated with PSS, leading to significant disability caused by pain and swelling of the affected upper extremity (3). A recent systematic review showed that approximately 15% of all patients with upper extremity deep vein thrombosis (UEDVT), regardless of cause, develop post-thrombotic syndrome (4). Post-thrombotic syndrome is more likely to occur with PSS than with other causes of UEDVT such as indwelling central venous catheters (3,5). Pulmonary embolism (PE) is an additional complication that may develop, although the risk is significantly higher with catheter-related UEDVT than with PSS (6,7).
Compression ultrasonography with color Doppler remains the preferred tool for initial evaluation of a patient with clinical features suggestive of UEDVT (Figure 1). Ultrasonography has the advantages of speed, portability, low cost and widespread availability with zero radiation and no contraindications that may be encountered with other modalities (3,8,9). Sonography is operator dependent and has a reported sensitivity of 78–100% and a specificity of 88–100% for detecting acute DVT involving the axillary vein or more distal veins of the arm (10). Evaluation of the subclavian vein is often limited by the operator’s inability to apply adequate compression (2).
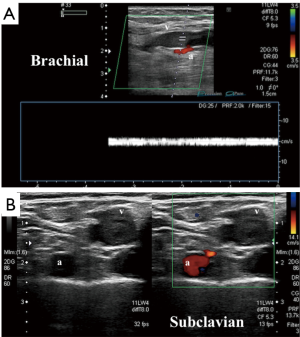
Cross-sectional studies such as CTV and MRV offer detailed anatomical evaluation of venous compression and can accurately estimate the degree of thrombosis (Figure 2). These modalities may also show secondary signs such as collateral vessel formation in cases of chronic occlusion or inflammatory changes secondary to acute thrombosis. Iodinated contrast is an obvious necessity in CTV, so a history of allergic reactions and/or renal failure may limit its utility. Although MRV is the most sensitive non-invasive modality, it is not commonly used due to its high cost and limited availability (3). Cross-sectional evaluation is particularly important for treatment planning and in determining the indication for more invasive diagnostic work-up.
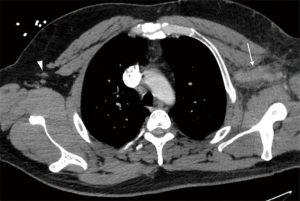
The gold standard for diagnosis of PSS is DSV (2,3). This is usually performed in conjunction with endovascular therapy. Positional venography is a specific technique used for PSS, which involves manipulating the patient’s affected arm in several positions including abduction and adduction to determine the extent of subclavian vein stenosis with and without compression (2). Venography can also help to identify chest wall and neck collaterals, which are usually a sign of subacute to long-standing chronic venous compression (2). Due to the increased availability of CTV and MRV, the role of conventional venography is now commonly reserved as an adjunct to endovascular treatment, and is not necessarily indicated as a confirmatory step prior to invasive surgical management (3).
The goals of treatment for PSS are to relieve the patient’s acute symptoms, prevent recurrent thrombosis, and avoid complications such as PE or post-thrombotic syndrome (2). There is currently no widespread consensus on an appropriate treatment algorithm given the low prevalence of the disease (3). The mainstays of treatment are conservative measures including bed rest, limb elevation, warm compresses and anticoagulation (2). However, the use of conservative therapy without accompanying surgical or endovascular treatment has been shown throughout the literature to result in high rates of residual symptoms, recurrent thrombosis and long-standing disability (3,11-13).
Systemic thrombolysis with tissue plasminogen activator (TPA) is more effective than standard anticoagulation at treating acute thrombosis; however, this treatment carries an associated risk of major bleeding (3). Catheter-directed thrombolysis is safer and more effective than systemic thrombolysis in the treatment of DVT and recommended in all patients with acute to subacute presentation (14). Catheter-directed thrombolysis should ideally be performed in patients with symptoms lasting less than 2 weeks to increase the probability of treatment success (3,14,15). Most patients require prolonged infusion for 24–48 hours. Follow-up venography is typically performed after catheter-directed thrombolysis to assess clot resolution and the need for additional treatment (Figure 3).
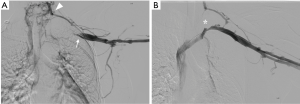
While catheter-directed thrombolysis is effective in treating acute DVT, it does not address the anatomical compression of the subclavian vein. Because these patients remain at risk for recurrent thrombosis, many surgeons advocate for operative decompression of the thoracic inlet regardless of post-thrombolytic outcome (2,11). Other authors have shown successful long-term results following catheter-directed thrombolysis without surgical decompression (16). Lee et al. reported that less than 25% of patients who underwent local thrombolytic therapy needed surgical decompression after a mean follow-up of 13 months (16). These patients were treated with oral anticoagulation for 3–12 months after thrombolysis (mean 5.2 months) (16). Recurrent thrombosis was more likely to occur in patients of younger age (less than 28 years old) and those that received stents during initial thrombolysis (16). Duration of anticoagulation therapy, history of trauma, patient sex, time delay to thrombolysis and degree of initial clot burden were not clinically significant predictors for recurrent thrombosis (16). Most surgeons agree that percutaneous venoplasty should not be attempted prior to surgical decompression. This practice can lead to even greater endothelial damage and potentially complicate further surgical treatment (17-19).
Surgical techniques for thoracic outlet decompression involve resection of the first rib with division of the scalene muscles and the costoclavicular ligament from either a transaxillary or infraclavicular approach (3). The infraclavicular approach is preferred by many due to the improved access to the costoclavicular ligament and subclavius tendon, two structures that are directly involved in compression of the subclavian vein (15). Both surgical approaches are complex operations that have significant risks such as pneumothorax, hemorrhage, or injury to the adjacent arteries and/or nerves (3). This underscores the need for further evaluation of endovascular options as first-line treatment in appropriate candidates.
Interventional radiologists play an important role in the immediate or delayed post-operative setting in certain cases of PSS. Molina et al. described a standardized treatment protocol for management of acute and chronic subclavian vein thrombosis secondary to thoracic outlet compression (15). In acute cases, catheter-directed thrombolysis is initiated immediately upon diagnosis and continued until clot resolution is demonstrated on next-day venography. This is followed by surgical decompression with the application of a saphenous vein patch to the stenotic segment of subclavian vein (15). Ultrasonography is performed the day after surgery to assess for residual stenosis, followed by venography in equivocal cases (15). Balloon venoplasty, with or without deployment of a self-expanding stent, can then be performed if there is evidence of residual stenosis on venography (15). In the case of chronic obstruction, a long saphenous vein graft is used to bypass the unsalvageable segment of fibrosed subclavian vein (15). This may be followed by venoplasty and possible stenting of the implanted graft (15).
Stent implantation should never be performed without first ensuring adequate surgical decompression. Repetitive trauma in the setting of chronic compression invariably leads to irreversible stent occlusion, fracture, and fibrosis, potentially limiting future surgical options (15). Stenting after transaxillary resection of the first rib has also been suggested to cause re-occlusion due to the inadequate decompression provided by this surgical approach (15). Additionally, stenting should not be performed in a partially or completely thrombosed subclavian vein due to the risk of clot propagation into the pulmonary vasculature (20).
Nutcracker syndrome
The nutcracker phenomenon refers to compression of the left renal vein (LRV) between the aorta and superior mesenteric artery (SMA) due to an abnormally acute takeoff of the SMA (21). Nutcracker syndrome describes signs and symptoms related to renal venous hypertension secondary to aortomesenteric compression. A less commonly seen “posterior nutcracker syndrome” has also been described in patients with similar symptoms caused by a retro-aortic renal vein being compressed between the aorta and vertebral column (22). Rarely cases of combined anterior and posterior nutcracker syndrome have been described in patients with a circumaortic LRV (23).
Nutcracker syndrome is most commonly seen in young and middle-aged adults, particularly females with low body mass index (2). Increased pressure within the LRV, whether due to aortomesenteric compression or retro-aortic compression, can manifest itself in a variety of presentations ranging from asymptomatic microhematuria to severe pelvic congestion syndrome (24). The most commonly reported symptom of nutcracker syndrome is hematuria, caused by rupture of thin-walled varices into the renal collecting system (25). Gonadal vein pain syndrome is another common manifestation, characterized as left flank pain radiating to the posteromedial thigh and buttock exacerbated by sitting, standing or walking (24). Left flank pain may also be due to renal colic, as a result of blood clots passing down the left ureter (24).
Chronic renal venous hypertension may cause reflux into the left gonadal vein leading to dilation of the gonadal vein and the formation of paraovarian/parauterine varices in women or scrotal varices in men (26). A left-sided varicocele is commonly seen in men with nutcracker syndrome, who may present with scrotal pain or infertility. Enlarged paraovarian and parauterine varices causing chronic pelvic pain, pelvic pressure, dyspareunia, dysuria, and/or dysmenorrhea is seen in females with pelvic congestion syndrome. Orthostatic proteinuria is another common sign of nutcracker syndrome, with a relatively high incidence among pubertal-age patients (26). Unilateral left-sided abdominal varices are a somewhat specific finding sometimes seen with nutcracker syndrome. This sign may indicate a high degree of clinical significance, and should prompt the clinician to consider more invasive treatment.
Doppler ultrasonography is the preferred modality for initial assessment of suspected nutcracker syndrome, with a sensitivity of 78% and specificity as high as 100% (27). Peak velocity at the renal hilum and the point of aortomesenteric compression are often used in addition to measurements of the inner diameter of the LRV at these points (Figure 4). An anteroposterior (AP) diameter ratio of 5.0 and a peak velocity ratio of 5.0 are often used as general cutoff criteria, although this is not strictly followed if clinical presentation and other imaging characteristics are strongly suggestive (28). Ultrasound is also helpful in evaluating secondary manifestations of renal vein entrapment including enlarged gonadal veins, pelvic varices and varicoceles (29).
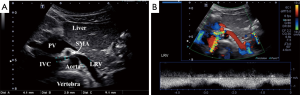
Cross-sectional venography with CTV or MRV is often recommended as a next step in the workup of patients with clinical and sonographic findings suspicious for nutcracker syndrome (23). Cross-sectional imaging is useful in evaluating the anatomical course of the LRV and its related structures, as well as establishing the degree of pelvic varices and extrapelvic collateral vessels (2). Measurement of the aortomesenteric angle on sagittal images is often helpful for diagnosis. This should be approximately 90 degrees in normal patients, while most symptomatic patients affected by nutcracker syndrome have an angle of less than 35 degrees (Figure 5) (24). Often a split-bolus technique may be used with CT in order to ensure optimal opacification of both the aortomesenteric angle and the LRV in the same imaging phase. CT venography is often preferred due to its lower cost and higher availability, although MRV may be offered as a radiation-free alterative for young or pregnant patients (23).
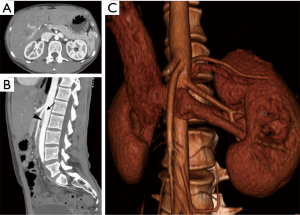
The gold standard in diagnosis of nutcracker syndrome is retrograde venography with reno-caval pressure gradient measurements (2). DSV accurately demonstrates the LRV at the point of compression and may show secondary signs of compression such as perirenal or periureteral collateral vessels, stagnation of contrast in the renal vein, and reflux of contrast into the adrenal or gonadal veins (22,23). A pressure gradient between the LRV and the inferior vena cava (IVC) measuring greater than 3 mmHg has historically been used for confirmatory diagnosis of nutcracker syndrome (2,23,25). However, in the era of advanced noninvasive cross-sectional imaging, conventional venography is usually not necessary for diagnostic confirmation.
Patients with mild hematuria often benefit from a trial of conservative management. This is especially effective in patients younger than 18 years, as complete resolution of hematuria within 2 years has been reported in as many as 75% of patients in this cohort (30). Conservative measures include modest weight gain for thin patients, elastic compression stockings for patients with pelvic or flank pain, and angiotensin inhibitors for patients with orthostatic proteinuria (2,31).
In patients with significant hematuria, severe flank/pelvic pain or renal functional impairment, endovascular or open surgical treatment is often indicated (2). Several surgical options have been described in the literature, including medial nephropexy, gonadocaval bypass, transposition of the SMA and renal autotransplantation (2). Transposition of the LRV is currently the preferred approach due to its relative efficiency and low risk profile (2,32,33). In general, surgery is associated with a high rate of morbidity compared to less invasive methods. Complications include DVT, PE, retroperitoneal hematoma, paralytic ileus and adhesion-related small bowel obstruction (32,34).
Definitive surgical decompression is still the preferred treatment for young and healthy patients; however, those of greater surgical risk may benefit from a less invasive endovascular approach. Endovascular stenting of the renal vein has been reported for nutcracker syndrome with a promising safety and efficacy profile (34). Typically, a self-expanding nitinol or stainless steel stent is used. The distal end of the stent is deployed within the first major branch of the LRV in order to achieve adequate fixation (34,35). This can be performed with or without preceding balloon venoplasty (34). Patients are often treated with oral anticoagulation for at least 6 months after the procedure to prevent venous in-stent thrombosis (34). Complications of LRV stenting include stent occlusion due to fibromuscular hyperplasia, proximal migration of thrombus, and PE (2,23). Mid-term stent patency rates are encouraging but convincing long-term data has yet to be reported (34).
IVUS is an evolving technique used during endovascular treatment of nutcracker syndrome. This approach offers superior visualization of cross-sectional detail and assessment of venous stenosis (36). IVUS can also be used to optimize stent deployment and assess post-stenting treatment success (37).
In men with left-sided varicocele, metallic coil embolization of the left gonadal vein can be performed in conjunction with LRV stenting (34). Embolization of the left gonadal vein and pelvic varices with coils and/or chemical sclerosants is an alternative approach for relieving pelvic congestion syndrome in as little as 24 hours, although data on its long-term efficacy is lacking (2,38).
May-Thurner syndrome
Virchow first described iliofemoral DVT secondary to venous stasis in 1851, noting a 5-fold left-sided predominance in lower-extremity deep venous thromboses (39). It was not until 1956, however, that May and Thurner first illustrated the anatomical basis of iliac compression syndrome, defining the disease as left common iliac vein (CIV) compression by the right common iliac artery (CIA) against the spine and pelvic brim (40). The syndrome is seen in as many as 18–49% of patients with left-sided lower extremity DVT, predominantly affecting young to middle-aged females (2,41).
The pathogenesis of May-Thurner syndrome is unique to other venous compression syndromes due its direct relation to the CIA. In addition to the obvious extrinsic compression leading to stasis and hypercoagulability within the vein, there are intrinsic factors specifically resulting from the chronic pulsatile force of the overlying right CIA (40,42). This leads to endothelial damage and local intimal hyperplasia, which subsequently results in the formation of webs, bands or spurs within the vein (43). These internal synechiae further exacerbate venous stasis and predispose to the development of acute and chronic DVT (2,40).
May-Thurner syndrome most frequently presents as acute left-sided iliofemoral DVT, characterized as sudden-onset severe lower extremity pain and swelling (2). The risks of acute DVT are greater in patients with additional risk factors such as prolonged immobility, recent surgery, obesity and pregnancy (2). It is not uncommon for May-Thurner syndrome to present as an acute PE without any history of lower extremity symptoms (44). Patients may alternatively present with chronic symptoms such as varicosities, hyperpigmentation, venous eczema or venous stasis ulcers (1,43). Phlegmasia cerulean dolens is a rare but lethal complication of severe iliofemoral vein thrombosis characterized as acute limb ischemia in the setting of near-total thrombosis of venous outflow (43). This entity, characterized as a massively swollen, blue, and mottled lower extremity, progresses to venous gangrene in as many as 40–60% of cases and carries a mortality of 20–40% (45).
Doppler ultrasonography is the most common modality used in the initial evaluation of lower extremity DVT; however, its utility in evaluating the iliac veins is limited due to their location deep within the pelvis (2,43). Assessment of the common femoral vein (CFV) can often provide clues to the interpreting radiologist if May-Thurner syndrome is suspected. Even if CFV patency without thrombus is demonstrated, a lack of respiratory variations or absence of response to Valsalva maneuver should prompt clinicians to consider a more proximal compression or obstruction (2).
Cross-sectional venography with CTV or MRV has demonstrated both excellent sensitivity and specificity in the evaluation of lower-extremity DVT (2). These modalities are imperative for evaluating thrombosis within the deep pelvis due to the limitations of ultrasound in this location (Figure 6). MRV has particular advantages over CTV in May-Thurner syndrome, including the ability to demonstrate retrograde flow in the ipsilateral iliac vein and collateral veins, as well as superior differentiation of intraluminal thrombus from contrast mixing when compared to CT (2).
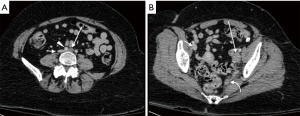
DSV is the gold standard for diagnosis of May-Thurner syndrome and is usually performed in conjunction with endovascular treatment. Typical findings include reversal of flow within the left internal iliac vein, tortuous venous collaterals crossing the pelvis, and an enlarged ascending lumbar vein (Figure 7) (1,2,46). Assessment of the pressure gradient between the left CIV and IVC may also be performed, with a gradient of >2 mmHg indicating hemodynamic significance (2,41). IVUS is sometimes used as an adjunct to conventional venography, allowing real-time visualization of intraluminal abnormalities such as mural spurs, webs or intramural venous channels (2). Similar to its utility discussed in nutcracker syndrome, IVUS can also be used during endovascular stenting to facilitate accurate placement across the stenosed vessel.
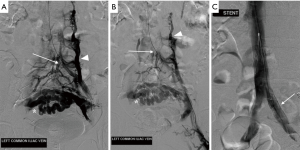
Conservative methods such as compression stockings and systemic anticoagulation are appropriate initial treatments for early signs of May-Thurner syndrome. These are aimed at preventing or decreasing the symptoms of post-thrombotic syndrome; however, conservative therapy alone is not adequate for long-term management due to its inability to address the underlying cause of venous compression (47).
Several surgical decompression techniques have been described, including saphenous vein bypass, placement of the right CIA into a peritoneal sling, reimplantation of the left CIV onto the vena cava, venotomy with excision of intraluminal adhesions, and vein patch venoplasty (48). Surgical treatment has an overall success rate, defined as patency of the left CIV or venous bypass, of 40–88% (49). The current standard of care for treatment of acute iliofemoral DVT is, however, primarily reliant on minimally invasive endovascular therapy. Operative decompression is typically reserved as second-line treatment in cases where endovascular methods fail.
May-Thurner syndrome is perhaps the most well-documented venous compression syndrome in regards to endovascular management. The current standard of care in acute iliofemoral DVT is urgent catheter-directed thrombolysis with mechanical thrombectomy, venoplasty and common iliac venous stenting (45). Many authors advocate emergently placing a retrievable IVC filter via transjugular approach prior to endovascular thrombolysis in order to prevent embolization of the significant clot burden from the CIV (45,50,51). This is followed by popliteal venous access from the prone position in order to gain access to the CIV (45).
Early thrombolytic therapy, whether achieved through pharmacologic or mechanical methods, is thought to play a major role in preserving valve function and preventing post-thrombotic syndrome (49,52). Pharmacologic thrombolysis is performed by direct infusion of TPA into the thrombosed vein 12 to 48 hours (45,48,53). Some authors prefer primary treatment with mechanical thrombectomy or pulsed infusion of TPA prior to stenting, followed by an additional 12 to 48 hours of catheter-directed thrombolysis (45,54).
Following thrombolysis, venoplasty with stent placement is the mainstay of endovascular treatment (45). This is typically performed with a large (10 to 16 mm) self-expanding nitinol or stainless-steel stent across the stenosis and into the IVC (45,48,49,51). All patients in whom stents are placed are typically treated with oral anticoagulation for at least 6 months (2). These patients are typically re-evaluated in clinic with duplex sonography at 3 and/or 6 months intervals following the initial procedure to ensure stent patency (49). In the event of stent occlusion or in-stent stenosis, attempts at revascularization are often performed successfully (51). Several studies have demonstrated long-term primary and secondary patency rates as high as 78% and 95%, respectively, at 2-year follow-up evaluation (49,51).
In the absence of thrombus, patients with May-Thurner syndrome may be treated with venoplasty and stenting alone. This is typically non-emergent and aimed at relieving the anatomical compression to prevent or decrease symptoms. These patients may be managed post-stenting with anti-platelet therapy rather than anticoagulation, although evidence to support this practice is mainly theoretical and based on pre-existing data from arterial interventions (55).
In summary, endovascular stenting with catheter-directed thrombolysis is a well-documented initial treatment for May-Thurner syndrome. Endovascular therapy is currently the standard of care, and surgery is often reserved for cases of stent failure not amenable to additional endovascular treatment.
Acknowledgements
None.
Footnote
Conflicts of Interest: The authors have no conflicts of interest to declare.
References
- Eliahou R, Sosna J, Bloom AI. Between a rock and a hard place: clinical and imaging features of vascular compression syndromes. Radiographics 2012;32:E33-49. [Crossref] [PubMed]
- Butros SR, Liu R, Oliveira GR, et al. Venous compression syndromes: clinical features, imaging findings and management. Br J Radiol 2013;86:20130284. [Crossref] [PubMed]
- Alla VM, Natarajan N, Kaushik M, et al. Paget-schroetter syndrome: review of pathogenesis and treatment of effort thrombosis. West J Emerg Med 2010;11:358-62. [PubMed]
- Elman EE, Kahn SR. The post-thrombotic syndrome after upper extremity deep venous thrombosis in adults: a systematic review. Thromb Res 2006;117:609-14. [Crossref] [PubMed]
- Kommareddy A, Zaroukian MH, Hassouna HI. Upper extremity deep venous thrombosis. Semin Thromb Hemost 2002;28:89-99. [Crossref] [PubMed]
- Monreal M, Lafoz E, Ruiz J, et al. Upper-extremity deep venous thrombosis and pulmonary embolism. A prospective study. Chest 1991;99:280-3. [Crossref] [PubMed]
- Kooij JD, van der Zant FM, van Beek EJ, et al. Pulmonary embolism in deep venous thrombosis of the upper extremity: more often in catheter-related thrombosis. Neth J Med 1997;50:238-42. [Crossref] [PubMed]
- Baarslag HJ, van Beek EJ, Koopman MM, et al. Prospective study of color duplex ultrasonography compared with contrast venography in patients suspected of having deep venous thrombosis of the upper extremities. Ann Intern Med 2002;136:865-72. [Crossref] [PubMed]
- Mustafa BO, Rathbun SW, Whitsett TL, et al. Sensitivity and specificity of ultrasonography in the diagnosis of upper extremity deep vein thrombosis: a systematic review. Arch Intern Med 2002;162:401-4. [Crossref] [PubMed]
- Chin EE, Zimmerman PT, Grant EG. Sonographic evaluation of upper extremity deep venous thrombosis. J Ultrasound Med 2005;24:829-38. [PubMed]
- Urschel HC Jr, Patel AN. Surgery remains the most effective treatment for Paget-Schroetter syndrome: 50 years' experience. Ann Thorac Surg 2008;86:254-60; discussion 260. [Crossref] [PubMed]
- Urschel HC Jr, Razzuk MA. Paget-Schroetter syndrome: what is the best management? Ann Thorac Surg 2000;69:1663-8; discussion 1668-9.
- AbuRahma AF, Robinson PA. Effort subclavian vein thrombosis: evolution of management. J Endovasc Ther 2000;7:302-8. [Crossref] [PubMed]
- Grunwald MR, Hofmann LV. Comparison of urokinase, alteplase, and reteplase for catheter-directed thrombolysis of deep venous thrombosis. J Vasc Interv Radiol 2004;15:347-52. [Crossref] [PubMed]
- Molina JE, Hunter DW, Dietz CA. Protocols for Paget-Schroetter syndrome and late treatment of chronic subclavian vein obstruction. Ann Thorac Surg 2009;87:416-22. [Crossref] [PubMed]
- Lee JT, Karwowski JK, Harris EJ, et al. Long-term thrombotic recurrence after nonoperative management of Paget-Schroetter syndrome. J Vasc Surg 2006;43:1236-43. [Crossref] [PubMed]
- Machleder HI. Evaluation of a new treatment strategy for Paget-Schroetter syndrome: spontaneous thrombosis of the axillary-subclavian vein. J Vasc Surg 1993;17:305-15; discussion 316-7. [Crossref] [PubMed]
- Meier GH, Pollak JS, Rosenblatt M, et al. Initial experience with venous stents in exertional axillary-subclavian vein thrombosis. J Vasc Surg 1996;24:974-81; discussion 981-3. [Crossref] [PubMed]
- Bjarnason H, Hunter DW, Crain MR, et al. Collapse of a Palmaz stent in the subclavian vein. AJR Am J Roentgenol 1993;160:1123-4. [Crossref] [PubMed]
- Khalifa M, Patel N, Moser S. Superior Vena Cava Obstruction Complicated by Upper Extremity Deep Vein Thrombosis: A Novel Endovascular Approach. Vasc Endovascular Surg 2016;50:180-2. [Crossref] [PubMed]
- El-Sadr AR, Mina E. Anatomical and surgical aspects in the operative management of varicocele. Urol Cutaneous Rev 1950;54:257-62. [PubMed]
- Lau JL, Lo R, Chan FL, et al. The posterior "nutcracker": hematuria secondary to retroaortic left renal vein. Urology 1986;28:437-9. [Crossref] [PubMed]
- Ahmed K, Sampath R, Khan MS. Current trends in the diagnosis and management of renal nutcracker syndrome: a review. Eur J Vasc Endovasc Surg 2006;31:410-6. [Crossref] [PubMed]
- Kurklinsky AK, Rooke TW. Nutcracker phenomenon and nutcracker syndrome. Mayo Clin Proc 2010;85:552-9. [Crossref] [PubMed]
- Beinart C, Sniderman KW, Saddekni S, et al. Left renal vein hypertension: a cause of occult hematuria. Radiology 1982;145:647-50. [Crossref] [PubMed]
- He Y, Wu Z, Chen S, et al. Nutcracker syndrome--how well do we know it? Urology 2014;83:12-7. [Crossref] [PubMed]
- Takebayashi S, Ueki T, Ikeda N, et al. Diagnosis of the nutcracker syndrome with color Doppler sonography: correlation with flow patterns on retrograde left renal venography. AJR Am J Roentgenol 1999;172:39-43. [Crossref] [PubMed]
- Kim SH, Cho SW, Kim HD, et al. Nutcracker syndrome: diagnosis with Doppler US. Radiology 1996;198:93-7. [Crossref] [PubMed]
- Park SJ, Shin JI. Renal Doppler ultrasonography in the diagnosis of nutcracker syndrome. Eur J Pediatr 2013;172:135-6. [Crossref] [PubMed]
- Shin JI, Park JM, Lee SM, et al. Factors affecting spontaneous resolution of hematuria in childhood nutcracker syndrome. Pediatr Nephrol 2005;20:609-13. [Crossref] [PubMed]
- Ha TS, Lee EJ. ACE inhibition can improve orthostatic proteinuria associated with nutcracker syndrome. Pediatr Nephrol 2006;21:1765-8. [Crossref] [PubMed]
- Hohenfellner M, D'Elia G, Hampel C, et al. Transposition of the left renal vein for treatment of the nutcracker phenomenon: long-term follow-up. Urology 2002;59:354-7. [Crossref] [PubMed]
- Reed NR, Kalra M, Bower TC, et al. Left renal vein transposition for nutcracker syndrome. J Vasc Surg 2009;49:386-93; discussion 393-4. [Crossref] [PubMed]
- Wang X, Zhang Y, Li C, et al. Results of endovascular treatment for patients with nutcracker syndrome. J Vasc Surg 2012;56:142-8. [Crossref] [PubMed]
- Policha A, Lamparello P, Sadek M, et al. Endovascular Treatment of Nutcracker Syndrome. Ann Vasc Surg 2016;36:295.e1-295.e
- Eskandari M, Pearce WH, Yao JS. Current Vascular Surgery: 40th Anniversary of the Northwestern Vascular Symposium. Shelton: People's Medical Publishing House, 2016.
- Jayaraj A, Gloviczki P, Peeran S, et al. Hybrid intervention for treatment of the nutcracker syndrome. J Vasc Surg Cases 2015;1:268-71. [Crossref]
- Ferreira M, Lanziotti L, Abuhadba G, et al. Chronic pelvic pain: the role of the nutcracker syndrome. J Vasc Bras 2008;7:76-9.
- Virchow R. Uber die Erweiterung kleiner Gefasse. Arch Path Anat 1851;3:427. [Crossref]
- May R, Thurner J. The cause of the predominantly sinistral occurrence of thrombosis of the pelvic veins. Angiology 1957;8:419-27. [Crossref] [PubMed]
- Oğuzkurt L, Ozkan U, Tercan F, et al. Ultrasonographic diagnosis of iliac vein compression (May-Thurner) syndrome. Diagn Interv Radiol 2007;13:152-5. [PubMed]
- Taheri SA, Williams J, Powell S, et al. Iliocaval compression syndrome. Am J Surg 1987;154:169-72. [Crossref] [PubMed]
- Lamba R, Tanner DT, Sekhon S, et al. Multidetector CT of vascular compression syndromes in the abdomen and pelvis. Radiographics 2014;34:93-115. [Crossref] [PubMed]
- Kishi K, Homma S, Kawabata M, et al. Pulmonary emboli caused by iliac compression syndrome without leg symptoms. Respiration 2002;69:269-72. [Crossref] [PubMed]
- Suwanabol PA, Tefera G, Schwarze ML. Syndromes associated with the deep veins: phlegmasia cerulea dolens, May-Thurner syndrome, and nutcracker syndrome. Perspect Vasc Surg Endovasc Ther 2010;22:223-30. [Crossref] [PubMed]
- Cil BE, Akpinar E, Karcaaltincaba M, et al. Case 76: May-Thurner syndrome. Radiology 2004;233:361-5. [Crossref] [PubMed]
- Cockett FB, Thomas ML. The iliac compression syndrome. Br J Surg 1965;52:816-21. [Crossref] [PubMed]
- Lamont JP, Pearl GJ, Patetsios P, et al. Prospective evaluation of endoluminal venous stents in the treatment of the May-Thurner syndrome. Ann Vasc Surg 2002;16:61-4. [Crossref] [PubMed]
- Patel NH, Stookey KR, Ketcham DB, et al. Endovascular management of acute extensive iliofemoral deep venous thrombosis caused by May-Thurner syndrome. J Vasc Interv Radiol 2000;11:1297-302. [Crossref] [PubMed]
- Nazarian GK, Bjarnason H, Dietz CA Jr, et al. Iliofemoral venous stenoses: effectiveness of treatment with metallic endovascular stents. Radiology 1996;200:193-9. [Crossref] [PubMed]
- Titus JM, Moise MA, Bena J, et al. Iliofemoral stenting for venous occlusive disease. J Vasc Surg 2011;53:706-12. [Crossref] [PubMed]
- Meissner MH, Manzo RA, Bergelin RO, et al. Deep venous insufficiency: the relationship between lysis and subsequent reflux. J Vasc Surg 1993;18:596-605; discussion 606-8. [Crossref] [PubMed]
- Dake MD, Semba CP. Thrombolytic therapy in venous occlusive disease. J Vasc Interv Radiol 1995;6:73S-77S. [Crossref] [PubMed]
- Murphy EH, Davis CM, Journeycake JM, et al. Symptomatic ileofemoral DVT after onset of oral contraceptive use in women with previously undiagnosed May-Thurner Syndrome. J Vasc Surg 2009;49:697-703. [Crossref] [PubMed]
- Meissner MH. Indications for platelet aggregation inhibitors after venous stents. Phlebology 2013;28 Suppl 1:91-8. [Crossref] [PubMed]