Circulating miRNA-21 as a diagnostic biomarker for acute coronary syndrome: a systematic review and meta-analysis of diagnostic test accuracy study
Highlight box
Key findings
• The systematic review and meta-analysis of 11 case-control studies involving 2,413 subjects found that circulating miR-21 has a pooled area under the standard receiver operating characteristic curve of 0.779 for discriminating between acute coronary syndrome (ACS) and non-ACS individuals.
What is known and what is new?
• ACS is a significant cause of morbidity and mortality, and early detection is crucial for improving prognosis.
• This study suggests that miR-21 in circulation could serve as a candidate diagnostic biomarker for ACS.
What is the implication, and what should change now?
• The findings implicate miR-21 as a potential ACS diagnostic tool. More research is needed to validate miR-21’s diagnostic value with larger prospective studies and to investigate its post-ACS prognostic role.
Introduction
Acute coronary syndrome (ACS) is a group of ischemic conditions associated with decreased blood flow in the coronary arteries, including, ST segment elevation myocardial infarction (STEMI), non-ST segment elevation myocardial infarction (NSTEMI), and unstable angina (UA), which occurs most commonly as a result of a thrombus forming within the lumen of a coronary artery and lead to acute myocardial infarction (AMI) (1,2). Globally around 110 million men and 80 million women have coronary heart disease (CHD) (3). The overall mortality for those patients was estimated to be 52% (4). Early diagnosis of ACS is critical since timely intervention may improve patients’ prognosis (5).
In the context of clinical and electrocardiography (ECG) findings, the diagnosis of ACS primarily relies on elevated high-sensitivity cardiac troponin I or T levels (hs-cTnI or hs-cTnT). However, their predictive accuracy within the first 2 hours after ACS onset is below 65%, driving the search for more accurate biomarkers for improved diagnosis at the critical early stages of ACS (1,6).
The microRNAs (miRNAs) are endogenous, small, noncoding RNAs that help to regulate gene expression of target mRNA in post-transcription processing (7). Last decade, miRNAs have been shown to be involved in various physiological and pathological processes, including cardiac hypertrophy, fibrosis, and apoptosis (8), stroke (9); and cellular differentiation, proliferation, apoptosis and stress response (8,10). Otherwise, circulating miRNAs are suggested in several studies to be potential biomarkers for the diagnosis of cardiovascular diseases (2,11-13), such as improving the diagnostic accuracy of AMI and predicting cardiovascular events.
Recently, miR-21, has been shown to participate in metabolic and inflammatory processes, and may have diagnostic potential as a biomarker for ACS, including myocardial infarction and UA, among others (13-17), although the studies cited are with relatively small sample sizes and had inconsistent outcomes. While a list of meta-analyses have been conducted on the diagnostic roles of various miRNAs such as miR-133 in CHD and AMI (18-21), no prior literature has systemically reviewed the evidence regarding the roles of miR-21 in AMI or ACS. Therefore, this systematic review and meta-analysis aimed to comprehensively evaluate the diagnostic potential of miR-21 for ACS based on the up-to-date evidence. We present this article in accordance with the PRISMA-DTA reporting checklist (available at https://cdt.amegroups.com/article/view/10.21037/cdt-23-385/rc) (22).
Methods
Search strategy
The protocol was registered on the PROSPERO registry (CRD42020209424). The search included: PubMed, EMBASE, and CENTRAL databases until March 17, 2024. The search used combinations of the keywords “acute coronary syndrome”, “ACS”, “myocardial infarction”, “AMI”, “angina”, “microRNA-21”, and “miR-21” properly combined with Boolean operators and using Medical Subject-Headings (MeSH) terms where appropriate. As an example, the specific search formula applied to PubMed was: (((“acute coronary syndrome” OR ACS) OR (“myocardial infarction” OR AMI)) OR (angina)) AND (microRNA-21 OR miR-21).
The reference lists of eligible articles were also searched manually for additional eligible studies. A comprehensive search strategy, which includes both the keywords and Boolean operators across all queried databases, along with the applied filters and limits, is detailed in the Table S1 for further reference (Table S1).
Selection criteria and data extraction
Inclusion criteria for eligible studies were: (I) case-control or cohort studies assessing the diagnostic value of circulating microR-21 in patients with ACS (including AMI, UA, or patients with ACS not specified as AMI or UA) compared to non-ACS; ACS was diagnosed by clinical symptoms and tests based on American College of Cardiology (ACC)/American Heart Association (AHA) guidelines; (II) miR-21 was quantified from plasma or serum using quantitative reverse transcription polymerase chain reaction (qRT-PCR); (III) sample size and area under the standard receiver operating characteristic curve (area under ROC curve; AUC) was reported with standard error (SE) or contained sufficient information for calculating SE. Non-human studies; reports written in languages other than English or Chinese; and letters, comment, reviews, editorials, case reports, proceedings, conference abstracts, personal communications and protocols were excluded. Eligibility of the studies were determined by two independent reviewers (J.G.H. and X.H.C.) using the above inclusion/exclusion criteria. When there was uncertainty regarding eligibility, a third reviewer (Z.W.D.) was consulted.
The following data were extracted from the included studies: name of first author, year of publication, study country, study design, characteristics of case and control groups, number of patients in each group, participants’ age, gender, specimen type, detection method for miR-21, maximum time from symptom onset to sample acquisition, relative fold increases in miR-21 and AUC values.
Quality assessment
The quality of each article included in this diagnostic meta-analysis was assessed using the Quality Assessment of Diagnostic Accuracy Studies-2 (QUADAS-2) score system, which has been demonstrated to be an effective tool for evaluating the quality of diagnostic accuracy studies (23). The QUADAS-2 tool, including four key domains (patient selection, index test, reference standard, flow and timing). Each is evaluated based on their risk of bias, and the first three are also examined for potential concerns related to applicability. Discrepancies were resolved by discussion to reach a consensus. Specifically, risk of bias and concerns were rated as either a high, low, or unclear risk/concern.
Statistical analysis
The primary outcome for this meta-analysis is the pooled discriminative ability of circulating miR-21 for the presence of ACS, which was assessed by the AUC. A χ2-based test of heterogeneity was performed and the inconsistency index (I2) and Q statistics were determined. If I2 was >50% or >75%, the trials were considered to be heterogeneous or highly heterogeneous, respectively. If I2 was <25%, the studies were considered to be homogeneous. The meta-analysis was conducted based on a random-effects model (DerSimonian-Laird method) because the pooled ROC curve only represents the relationship between sensitivity and specificity values across studies with different thresholds for each method. Pooled effects were calculated and a two-sided P value of <0.05 was regarded as statistical significance. In addition, subgroup analyses were performed according to types of ACS (AMI and UA) and types of control group (healthy and unhealthy) as well. Publication bias analysis was assessed by constructing funnel plots. The absence of publication bias was indicated by the data points forming a symmetric funnel-shaped distribution (24). All analyses were performed using MedCalc version 19.4 (MedCalc Software Ltd., Belgium).
Results
Literature search and study selection
A flowchart of the search and study selection process is shown in Figure 1. The electronic search identified a total of 455 citations. Amongst, 204 duplicate records were excluded, leaving 251 records. After screening titles and abstracts, 86 studies were retrieved for further full-text assessment for eligibility. Amongst, 11 studies (2,14,15,17,25-31) were included for the qualitative review and quantitative synthesis. The most common reasons for exclusion were: study had different objectives, studies investigated mRNAs other than miR-21, as well as no quantitative outcomes of interest were reported.
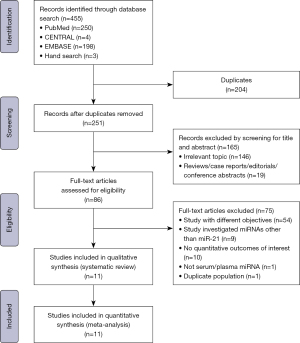
Study characteristics
Characteristics of the studies included are summarized in Table 1. The 11 included studies were published between 2012 and 2023. Six studies were conducted in China, one in Iran, one in China/Sweden, one in India, one in Germany and one in the Netherlands. Study sample sizes ranged from 27 to 1,042 subjects. Overall, the included studies reported on a total of 2,413 subjects, with 1,236 ACS cases and 1,177 controls. Participants’ mean age ranged from 51.0 to 69.0 years and the proportion of males ranged from 38.5% to 79.6% across the studies.
Table 1
First author [year] | Country | Case/control | No. of patients | Mean age (years) | Male (%) | Specimen | Detection method | Maximum time from symptom onset to sample acquisition | Relative fold increase |
---|---|---|---|---|---|---|---|---|---|
Xu [2023] (31) | China | AMI | 40 | 55.7 | 75.0 | Plasma | SYBR | 6 h | NA |
UA | 22 | 56.9 | 54.5 | ||||||
Healthy (clinically unsuspected of CAD) | 22 | ||||||||
Biener [2021] (26) | Germany | AMI | 137 | 69.0 | 79.6 | Plasma | TransTaq | NA | NA |
With symptoms suggestive of ACS including chest pain, dyspnea, or atypical chest pain | 905 | 64.0 | 64.0 | ||||||
Kumar [2020] (27) | India | AMI and UA | 32 | 58.7 | 62.8 | Plasma | TransTaq | NA | 2.46 |
Healthy (clinically unsuspected of CAD) | 50 | 53.1 | 64.8 | ||||||
Ling [2020] (28) | China | AMI | 34 | 57.8 | 70.6 | Serum | SYBR | Within 2 h after AMI | NA |
UA | 31 | 60.1 | 61.3 | ||||||
Healthy (clinically unsuspected of CAD) | 22 | 58.5 | 50.0 | ||||||
Gao [2019] (14) | China | AMI | 184 | 59.0 | 67.4 | Serum | TransTaq | NA | NA |
Healthy (clinically unsuspected of CAD) | 150 | 60.4 | 68.0 | ||||||
Darabi [2017] (25) | Iran | AMI and UA | 53 | 63.1 | 62.3 | Serum | ROX | NA | 2.39 |
Stable CAD | 52 | 62.3 | 61.5 | ||||||
Liu [2017] (15) | China | UA | 98 | 68.1 | 51.0 | Plasma | TaqMan | 12 h | 2.02 |
Non-cardiac chest pain | 95 | 51.0 | 50.5 | ||||||
Zhang [2016] (17) | China | AMI | 17 | 62.8 | 70.6 | Plasma | TaqMan | NA | 4.7 |
Non-cardiac chest pain | 10 | 56.2 | 50.0 | ||||||
Wang [2014] (30) | China | AMI | 17 | 52.0 | 70.6 | Plasma | SYBR | 24 h | NA |
Healthy volunteer | 28 | 58.0 | 42.9 | ||||||
Ren [2013] (29) | China, Sweden | UA | 45 | 61.0 | 53.8 | Plasma | TaqMan | NA | NA |
Non-cardiac chest pain | 37 | 56.0 | 38.5 | ||||||
Oerlemans [2012] (2) | Netherland | AMI and UA | 106 | 68.7 | 66.0 | Serum | TaqMan | NA | NA |
Stable angina, rhythm disorders, heart failure, pericarditis, other cardiac diagnosis and non-cardiac chest pain | 226 | 60.2 | 53.1 |
AMI, acute myocardial infarction; UA, unstable angina; CAD, coronary artery disease; NA, not applicable.
Meta-analysis
The forest plot in Figure 2 shows the results of the meta-analysis. Eleven studies (2,14,15,17,25-31) provided AUCs, from which a pooled AUC of 0.779 [SE =0.033; 95% confidence interval (CI): 0.715–0.843] was derived. High heterogeneity was noted among the studies (Q statistic =190.64, I2=94.23%, P<0.001) (Figure 2).
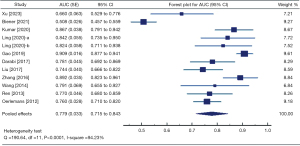
Six studies (14,17,26,28,30,31) reported AUCs in discriminating AMI from control group, and three studies (15,28,29) reported AUCs for UA from control. Subgroup analyses were performed in order to determine whether circulating miR-21 has different discriminative performance by which to distinguish AMI and UA from controls. A pooled AUC of 0.767 (SE =0.061; 95% CI: 0.648–0.887) was derived for discriminating AMI from non-ACS control, with high heterogeneity detected (Q statistic =182.76, I2=97.26%) (Figure 3A). Pooled AUC for discriminating UA from non-ACS control was 0.770 (SE 0.027; 95% CI:0.718–0.822), with no heterogeneity detected (Q statistic =1.297, I2=0.0%) (Figure 3B).
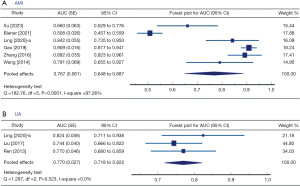
In subgroup analyses based on different types of control group, the pooled AUC for discriminating ACS versus healthy controls, derived from five studies (14,27,28,30,31), was 0.779 (SE =0.033; 95% CI: 0.715–0.843), with high heterogeneity detected (Q statistic =18.54, I2=73.03%) (Figure 4A). The pooled AUC for discriminating ACS versus unhealthy controls, derived from six studies (2,15,17,25,26,29), was 0.740 (SE =0.049; 95% CI: 0.645–0.836), also with high heterogeneity detected (Q statistic =95.10, I2=94.74%) (Figure 4B).
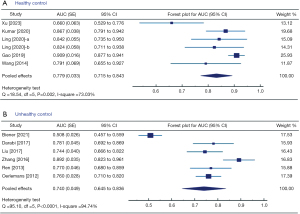
Publication bias
The results of funnel plot revealed there was no evidence of publication bias (P=0.49 by the Egger’s test) (Figure 5).
Quality of the included studies
The result of QUADAS-2 quality assessment of the included studies is shown in Table S2. The overall quality of the included studies was considered moderate. All of the studies were of a case-control design, and thus had a high risk of bias in patient selection. Not all the studies mention a definite threshold of miR-21 for predicting ACS. The studies included also had raised high applicability concerns in the patient selection domain (Table S2).
Discussion
The present systematic review and meta-analysis combined the diagnostic performance of miR-21 for the detection of ACS based on 2,413 subjects and 11 studies from the most up-to-date literature. No publication bias was noted. The pooled AUC of 0.779 demonstrates a ’satisfactory’ discriminatory ability (31), indicating that miR-21 may be useful for distinguishing between ACS and non-ACS individuals. Subgroup analyses were performed according to the subtypes of ACS and to the characteristics of the control groups. Although the results revealed that miR-21 also exhibited a satisfactory discriminating ability for AMI from non-ACS controls; however, high heterogeneity still existed. Nevertheless, the pooled AUC for UA demonstrated a satisfactory diagnostic accuracy (AUC =0.770) with no heterogeneity, indicating that such results can be considered reliable. In addition, analyses of miR-21’s ability to distinguish ACS from both healthy and unhealthy controls also demonstrated AUCs over 0.7 but with notable heterogeneity among the studies in the subgroups detected.
As of the current date, the investigation into the role of miR-21 in ACS has been relatively limited, leading to a restricted pool of eligible studies for our analysis. Despite this limitation, the subgroup analyses focused on UA individually revealed no heterogeneity, suggesting that the initial heterogeneity might have been partly influenced by studies that included a combination of non-unified cases (mix of AMI and UA). Heterogeneity could also have come from the choice of controls, which could be a significant methodological issue in research on the diagnostic potential of miRNAs, as pointed out in other studies (32). Among the included studies, five studies were controlled using healthy participants (14,27,28,30,31), three using participants with non-cardiac chest pain (15,17,29), one using patients with pre-existing stable CAD (25), one with symptoms suggestive of ACS (26), and one with mixed controls (2). We also attempted to tackle heterogeneity by analyzing the value of miR-21 in distinguishing ACS from healthy and unhealthy controls separately. Despite these efforts, significant heterogeneity was still evident in the subgroup analyses. One of the included studies, Wang et al. [2014] (30), reported a higher diagnostic value for AMI vs. non-ischemic controls (AUC =0.981) than for AMI vs. ischemic controls (AUC =0.889), which indicates that miR-21 might be more effective in differentiating ACS from healthy individuals than from those with pre-existing CHD. Furthermore, the heterogeneity observed in the subgroup analyses may result from a combination of clinical, demographic, methodological, and procedural factors. For example, the heterogeneity found in the subgroup of studies on AMI might be sourced from the inability to analyze NSTEMI and STEMI separately. Grouping these conditions in reports may obscure the differences between them. As mentioned previously, varied characteristics of the control groups might reflect underlying differences in risk factors or comorbidities, thus leading to heterogeneity. Even in the studies using ‘healthy controls’, inconsistencies in participant demographics such as age and race, diverse miRNA detection and processing methods, and potential difference in time window of miRNA sample collection might all introduce variability and could influence the analytic outcomes. Nevertheless, the findings underscore the necessity for further research to fully understand miR-21’s diagnostic potential and its role in ACS. Future meta-analyses are warranted to address the issue of heterogeneity by distinguishing NSTEMI and STEMI, or further adjusting for relevant confounders if possible.
In the clinical setting, the diagnostic methods for ACS include evaluating circulating biomarkers, which are suggested in the guidelines of the ACC and AHA (1,4) to be essential for confirming the diagnosis of ACS because of the limitations of clinical symptoms and ECG alone in diagnosing patients with NSTEMI or UA. Currently, in the context of clinical and ECG findings, the diagnosis of ACS is based on elevation of hs-cTnI or hs-cTnT (33,34), which the guidelines (1) and independent authors (16) also recommend. Although hs-cTn tests are recommended for ACS prediction, the sensitivity and specificity of accurate prediction are below 65% within a 2-hour window after the onset of ACS (6). This makes researchers continue their search for novel biomarkers with better accuracy in prediction, such as miRNAs, to gain additional diagnostic advantages, especially at the instance of ACS occurrence. Despite discovering the diagnostic value of miR-21 for ACS in the current meta-analysis, we acknowledge that certain gaps still exist before its routine application in diagnostic investigations. First, non-cardiac diseases and phenotypes (e.g., end-stage kidney disease, age, and sex) and intake of medicines (e.g., statins and angiotensin-converting enzyme inhibitor/angiotensin receptor blocker) may influence circulating miRNA levels (11,13). The other key issue associated with applying miR-21 as a biomarker for ACS is its cardiac specificity (35). As reported previously, miR-21 has exhibited certain diagnostic capabilities in several types of cancer (36) and has been shown to be significantly involved in diabetes (35) and pulmonary hypertension. One of the studies included in this meta-analysis, Oerlemans et al. (2), reported that when miRNA-21 was combined with hs-cTn, it offered a greater diagnostic accuracy than did miRNA-21 or troponin alone, supporting proper integration of this novel and the traditional biomarkers may further benefit the diagnosis. The authors of that study concluded that miR-21 could be used at least complementarily with conventional biomarkers to improve early diagnosis.
Features of miRNAs and evidence supporting their use in diagnosing cardiovascular disease are found in fairly recent studies. Several miRNAs have been evaluated in previous meta-analyses, including miR-499, miR-1, miR-133a, miR-208b for AMI (2,19-21,37). The miRNAs appear to function in a regulated process to repress protein synthesis, although the precise molecular mechanisms are not fully understood; however, they are known to directly regulate the activity of up to 60% of protein-coding genes, which may help to explain their presence in miRNA-mediated events such as ACS (10). The current findings add to previous knowledge and are the first to examine the role of miR-21 in ACS. Other studies have reported that miR-21 is expressed in cardiomyocytes, fibroblasts, and endothelial cells where apoptosis is adequately regulated, as well as cardiac fibrosis, proliferation, and cellular migration (32,35,38-40). Dong et al. [2014] (38) suggested that elevated miR-21 expression may precipitate cardiac hypertrophy and fibrosis, but that silencing the miR-21 gene inhibited fibrosis and improved cardiac function. A systematic review and meta-analysis by Chen et al. [2017] (41) identified miRNAs as diagnostic biomarkers for AMI in Asian populations. The authors of that study suggested that 2–3 miRNAs may need to be combined to provide more accurate diagnostic ability. This notion was supported by several studies. Oerlemans et al. (2) found that three miRNAs produced a significantly higher AUC (0.94) than hs-troponin T (0.89), displaying the great potential of miRNAs in early diagnosis of AMI. Shalaby et al. (42) also reported that combining miRNA-499 and miRNA-210 significantly improved the AUC to 0.96 for ACS onset <3 hours. Besides these combinations, miR-361-5 and miR-145 also provide AUC at 0.870 (43) and 0.852 (44), respectively. Monitoring potential biomarkers might accelerate the diagnosis of ACS patients in the emergency unit. Clearly, more evidence is needed to confirm the results of studies conducted to date.
Strengths and limitations
This is the first meta-analysis to evaluate the diagnostic value of miR-21 for discriminating ACS patients from non-ACS individuals. The inclusion criteria were established rigorously and heterogeneity across studies was carefully managed using a random-effects model and subgroup analyses. In particular, AUC was used to explicitly demonstrate the diagnostic value, which is more informative than only showing associations of dysregulation of certain miRNA as done in many previous studies. Nevertheless, the present review had several limitations. First, it included only a small number of studies with relatively small sample sizes, especially in subgroup analyses, in which the effect size might have been overestimated. NSTEMI and STEMI were not reported individually in any of the included studies, hence they could not be analyzed separately in this meta-analysis. The control groups were not homogenous, and even the studies that included healthy controls might have included subjects with different risk factors or comorbidities that could influence the results. We also were unable to control the discrepancies of age, race/ethnicity, detection and processing techniques of miRNAs or other potential factors that could possibly bias the meta-analysis. The precise time points of miRNA sample collection were not stated clearly in all included studies, thus the impact that time has could not be investigated. We did not assess the prognostic role of miR-21 after ACS. Some authors suggested that miR-21 could be used as a predictor of prognosis and survival after AMI (13), which was not assessed in this review due to limited data.
Conclusions
This meta-analysis of the most updated literature indicates that circulating miR-21 has satisfactory discriminative performance in differentiating between ACS and non-ACS. Circulating miR-21 may be considered a potential candidate as a novel diagnostic biomarker for ACS, although some limitations including the presence of study heterogeneity pose challenges to the interpretation and applicability of the findings. Future research involving larger, prospective studies is needed. As more studies become available, an updated meta-analysis will be crucial to corroborate the findings of this review and further validate the use of miRNAs in the clinical diagnosis of ACS.
Acknowledgments
We would like to thank asiamedcom.com for the help in English proof-reading for our paper.
Funding: This work was funded by
Footnote
Reporting Checklist: The authors have completed the PRISMA-DTA reporting checklist. Available at https://cdt.amegroups.com/article/view/10.21037/cdt-23-385/rc
Peer Review File: Available at https://cdt.amegroups.com/article/view/10.21037/cdt-23-385/prf
Conflicts of Interest: All authors have completed the ICMJE uniform disclosure form (available at https://cdt.amegroups.com/article/view/10.21037/cdt-23-385/coif). The authors have no conflicts of interest to declare.
Ethical Statement: The authors are accountable for all aspects of the work in ensuring that questions related to the accuracy or integrity of any part of the work are appropriately investigated and resolved.
Open Access Statement: This is an Open Access article distributed in accordance with the Creative Commons Attribution-NonCommercial-NoDerivs 4.0 International License (CC BY-NC-ND 4.0), which permits the non-commercial replication and distribution of the article with the strict proviso that no changes or edits are made and the original work is properly cited (including links to both the formal publication through the relevant DOI and the license). See: https://creativecommons.org/licenses/by-nc-nd/4.0/.
References
- Prejean SP, Din M, Reyes E, et al. Guidelines in review: Comparison of the 2014 AHA/ACC guideline for the management of patients with non-ST-elevation acute coronary syndromes and the 2015 ESC guidelines for the management of acute coronary syndromes in patients presenting without persistent ST-segment elevation. J Nucl Cardiol 2018;25:769-76. [Crossref] [PubMed]
- Oerlemans MI, Mosterd A, Dekker MS, et al. Early assessment of acute coronary syndromes in the emergency department: the potential diagnostic value of circulating microRNAs. EMBO Mol Med 2012;4:1176-85. [Crossref] [PubMed]
- Timmis A, Kazakiewicz D, Townsend N, et al. Global epidemiology of acute coronary syndromes. Nat Rev Cardiol 2023;20:778-88. [Crossref] [PubMed]
- Ellis CJ, Gamble GD, Williams MJA, et al. All-Cause Mortality Following an Acute Coronary Syndrome: 12-Year Follow-Up of the Comprehensive 2002 New Zealand Acute Coronary Syndrome Audit. Heart Lung Circ 2019;28:245-56. [Crossref] [PubMed]
- Katsioupa M, Kourampi I, Oikonomou E, et al. Novel Biomarkers and Their Role in the Diagnosis and Prognosis of Acute Coronary Syndrome. Life (Basel) 2023;13:1992. [Crossref] [PubMed]
- Lazar DR, Lazar FL, Homorodean C, et al. High-Sensitivity Troponin: A Review on Characteristics, Assessment, and Clinical Implications. Dis Markers 2022;2022:9713326. [Crossref] [PubMed]
- O'Brien J, Hayder H, Zayed Y, et al. Overview of MicroRNA Biogenesis, Mechanisms of Actions, and Circulation. Front Endocrinol (Lausanne) 2018;9:402. [Crossref] [PubMed]
- Wang J, Liew OW, Richards AM, et al. Overview of MicroRNAs in Cardiac Hypertrophy, Fibrosis, and Apoptosis. Int J Mol Sci 2016;17:749. [Crossref] [PubMed]
- Mirzaei H, Momeni F, Saadatpour L, et al. MicroRNA: Relevance to stroke diagnosis, prognosis, and therapy. J Cell Physiol 2018;233:856-65. [Crossref] [PubMed]
- Naeli P, Winter T, Hackett AP, et al. The intricate balance between microRNA-induced mRNA decay and translational repression. FEBS J 2023;290:2508-24. [Crossref] [PubMed]
- Chen Z, Li C, Lin K, et al. MicroRNAs in acute myocardial infarction: Evident value as novel biomarkers? Anatol J Cardiol 2018;19:140-7. [Crossref] [PubMed]
- Deddens JC, Colijn JM, Oerlemans MI, et al. Circulating microRNAs as novel biomarkers for the early diagnosis of acute coronary syndrome. J Cardiovasc Transl Res 2013;6:884-98. [Crossref] [PubMed]
- Roy S, Khanna S, Hussain SR, et al. MicroRNA expression in response to murine myocardial infarction: miR-21 regulates fibroblast metalloprotease-2 via phosphatase and tensin homologue. Cardiovasc Res 2009;82:21-9. [Crossref] [PubMed]
- Gao C, Zhao D, Wang J, et al. Clinical significance and correlation of microRNA-21 expression and the neutrophil-lymphocyte ratio in patients with acute myocardial infarction. Clinics (Sao Paulo) 2019;74:e1237. [Crossref] [PubMed]
- Liu J, Li SF, Lee CY, et al. Circulating microRNAs as potential biomarkers for unstable angina. Int J Clin Exp Pathol 2017;10:9073-83. [PubMed]
- Thygesen K, Alpert JS, Jaffe AS, et al. Third universal definition of myocardial infarction. Eur Heart J 2012;33:2551-67. [Crossref] [PubMed]
- Zhang Y, Liu YJ, Liu T, et al. Plasma microRNA-21 is a potential diagnostic biomarker of acute myocardial infarction. Eur Rev Med Pharmacol Sci 2016;20:323-9. [PubMed]
- Zhang L, Ding H, Zhang Y, et al. Circulating MicroRNAs: Biogenesis and Clinical Significance in Acute Myocardial Infarction. Front Physiol 2020;11:1088. [Crossref] [PubMed]
- Wang SS, Wu LJ, Li JJ, et al. A meta-analysis of dysregulated miRNAs in coronary heart disease. Life Sci 2018;215:170-81. [Crossref] [PubMed]
- Zhao J, Yu H, Yan P, et al. Circulating MicroRNA-499 as a Diagnostic Biomarker for Acute Myocardial Infarction: A Meta-analysis. Dis Markers 2019;2019:6121696. [Crossref] [PubMed]
- Zhu L, Liu F, Xie H, et al. Diagnostic performance of microRNA-133a in acute myocardial infarction: A meta-analysis. Cardiol J 2018;25:260-7. [PubMed]
- McInnes MDF, Moher D, Thombs BD, et al. Preferred Reporting Items for a Systematic Review and Meta-analysis of Diagnostic Test Accuracy Studies: The PRISMA-DTA Statement. JAMA 2018;319:388-96. [Crossref] [PubMed]
- Whiting PF, Rutjes AW, Westwood ME, et al. QUADAS-2: a revised tool for the quality assessment of diagnostic accuracy studies. Ann Intern Med 2011;155:529-36. [Crossref] [PubMed]
- Sutton AJ, Duval SJ, Tweedie RL, et al. Empirical assessment of effect of publication bias on meta-analyses. BMJ 2000;320:1574-7. [Crossref] [PubMed]
- Darabi F, Aghaei M, Movahedian A, et al. Association of serum microRNA-21 levels with Visfatin, inflammation, and acute coronary syndromes. Heart Vessels 2017;32:549-57. [Crossref] [PubMed]
- Biener M, Giannitsis E, Thum T, et al. Diagnostic value of circulating microRNAs compared to high-sensitivity troponin T for the detection of non-ST-segment elevation myocardial infarction. Eur Heart J Acute Cardiovasc Care 2021;10:653-60. [Crossref] [PubMed]
- Kumar D, Narang R, Sreenivas V, et al. Circulatory miR-133b and miR-21 as Novel Biomarkers in Early Prediction and Diagnosis of Coronary Artery Disease. Genes (Basel) 2020;11:164. [Crossref] [PubMed]
- Ling H, Guo Z, Shi Y, et al. Serum Exosomal MicroRNA-21, MicroRNA-126, and PTEN Are Novel Biomarkers for Diagnosis of Acute Coronary Syndrome. Front Physiol 2020;11:654. [Crossref] [PubMed]
- Ren J, Zhang J, Xu N, et al. Signature of circulating microRNAs as potential biomarkers in vulnerable coronary artery disease. PLoS One 2013;8:e80738. [Crossref] [PubMed]
- Wang F, Long G, Zhao C, et al. Atherosclerosis-related circulating miRNAs as novel and sensitive predictors for acute myocardial infarction. PLoS One 2014;9:e105734. [Crossref] [PubMed]
- Xu L, Tian L, Yan Z, et al. Diagnostic and prognostic value of miR-486-5p, miR-451a, miR-21-5p and monocyte to high-density lipoprotein cholesterol ratio in patients with acute myocardial infarction. Heart Vessels 2023;38:318-31. [Crossref] [PubMed]
- Ke-Gang J, Zhi-Wei L, Xin Z, et al. Evaluating Diagnostic and Prognostic Value of Plasma miRNA133a in Acute Chest Pain Patients Undergoing Coronary Angiography. Medicine (Baltimore) 2016;95:e3412. [Crossref] [PubMed]
- Suzuki K, Komukai K, Nakata K, et al. The Usefulness and Limitations of Point-of-care Cardiac Troponin Measurement in the Emergency Department. Intern Med 2018;57:1673-80. [Crossref] [PubMed]
- Xu RY, Zhu XF, Yang Y, et al. High-sensitive cardiac troponin T. J Geriatr Cardiol 2013;10:102-9. [PubMed]
- Jiang Q, Lyu XM, Yuan Y, et al. Plasma miR-21 expression: an indicator for the severity of Type 2 diabetes with diabetic retinopathy. Biosci Rep 2017;37:BSR20160589. [Crossref] [PubMed]
- Rupaimoole R, Slack FJ. MicroRNA therapeutics: towards a new era for the management of cancer and other diseases. Nat Rev Drug Discov 2017;16:203-22. [Crossref] [PubMed]
- Zhai C, Li R, Hou K, et al. Value of Blood-Based microRNAs in the Diagnosis of Acute Myocardial Infarction: A Systematic Review and Meta-Analysis. Front Physiol 2020;11:691. [Crossref] [PubMed]
- Dong S, Ma W, Hao B, et al. microRNA-21 promotes cardiac fibrosis and development of heart failure with preserved left ventricular ejection fraction by up-regulating Bcl-2. Int J Clin Exp Pathol 2014;7:565-74. [PubMed]
- Fan X, Wang E, Wang X, et al. MicroRNA-21 is a unique signature associated with coronary plaque instability in humans by regulating matrix metalloproteinase-9 via reversion-inducing cysteine-rich protein with Kazal motifs. Exp Mol Pathol 2014;96:242-9. [Crossref] [PubMed]
- Liu X, Dong Y, Chen S, et al. Circulating MicroRNA-146a and MicroRNA-21 Predict Left Ventricular Remodeling after ST-Elevation Myocardial Infarction. Cardiology 2015;132:233-41. [Crossref] [PubMed]
- Chen YN, Chang CW, Lin CW, et al. Numerical investigation of fracture impaction in proximal humeral fracture fixation with locking plate and intramedullary nail. Int Orthop 2017;41:1471-80. [Crossref] [PubMed]
- Shalaby SM, El-Shal AS, Shoukry A, et al. Serum miRNA-499 and miRNA-210: A potential role in early diagnosis of acute coronary syndrome. IUBMB Life 2016;68:673-82. [Crossref] [PubMed]
- Wu S, Sun H, Sun B. MicroRNA-145 is involved in endothelial cell dysfunction and acts as a promising biomarker of acute coronary syndrome. Eur J Med Res 2020;25:2. [Crossref] [PubMed]
- Zhang W, Chang G, Cao L, et al. Dysregulation of serum miR-361-5p serves as a biomarker to predict disease onset and short-term prognosis in acute coronary syndrome patients. BMC Cardiovasc Disord 2021;21:74. [Crossref] [PubMed]