Imaging of venous compression syndromes
Introduction
Venous compression syndromes are caused by extrinsic mass effect on a vein by adjacent structures such as arteries, bones, muscles, or ligaments (1). In a narrow and confined anatomic space, the otherwise morphologically normal vein becomes entrapped between rigid or semirigid surfaces, usually related to an underlying anatomic abnormality (2,3). Repetitive microtrauma causing endothelial injury at the site of external venous compression is also implicated in the pathogenesis of these disorders (1,3). Venous compression syndromes are rare; in fact, vascular entrapment (either venous or arterial) affects less than 1% of the general population (3). Nevertheless, the complications can be substantial and debilitating, including venous stasis, insufficiency, or thrombosis (3). Moreover, these entities disproportionally affect young and otherwise healthy patients who are most likely to benefit long-term from prompt and effective treatment (1-3).
Diagnosing venous compression can be challenging, as many patients are asymptomatic and when symptoms such as pain, swelling, or hematuria do occur, they are often vague and nonspecific, leading to delayed diagnosis or misdiagnosis (1,4). Often, clinical manifestations are not apparent until pressure in the affected vein increases or changes flow direction, leading to venous collateral and varicosity formation and possibly acute deep vein thrombosis (1). At the same time, caution must be stressed to not overdiagnose or treat patients who are incidentally discovered to have anatomical factors that predispose to venous compression but are otherwise asymptomatic (4).
Continued advances in imaging, taken in clinical context, have helped facilitate more prompt and accurate diagnosis of venous compression syndromes. Noninvasive options such as ultrasound (US) with Doppler, contrast-enhanced computed tomography (CT), CT venography (CTV), magnetic resonance imaging (MRI), and magnetic resonance venography (MRV) allow precise definition of anatomical abnormalities and prediction of hemodynamic significance, providing a roadmap prior to therapy (1). In turn, invasive modalities such as catheter venography may be obviated, limited, or reserved for situations in which intervention is planned. Herein, we review the broad spectrum of venous compression syndromes, which include the May-Thurner syndrome (MTS), variant iliac vein compression syndrome (IVCS), venous thoracic outlet syndrome (VTOS)/Paget-Schroetter syndrome, nutcracker syndrome (NCS), and popliteal venous compression (PVC) (1,3,4). The central role of imaging is emphasized, while clinical features and treatment options are highlighted.
Imaging evaluation
Ultrasound (US)
US is typically the first modality obtained in the assessment of a possible venous compression syndrome. Although operator-dependent (4), it is highly portable and essentially risk-free, providing an excellent initial and sometimes definitive imaging overview. In general, US is very accurate for identifying deep venous thrombosis (DVT) within the veins affected by compression (1). The overall accuracy of US for the detection of DVT approaches 100% for the thighs and 95% for the upper extremities (5). Other advantages include the ability to interrogate flow direction using Doppler techniques and to discern hemodynamic significance of venous compression using dynamic maneuvers (1). Nevertheless, anatomical and patient factors may ultimately obscure venous visualization and diagnosis of a venous compression syndrome [e.g., difficult assessment of iliac veins and inferior vena cava (IVC) within the abdomen, or the subclavian vein below the clavicle] (5,6).
CT
Multidetector computed tomography (MDCT) with CTV provides a rapid, accurate, and reliable assessment of suspected venous compression, overcoming many of the limitations of US. Further advantages include exquisite spatial, contrast, and temporal resolution. Moreover, isotropic CT datasets allow robust post-processing capabilities. Multiplanar reformation (MPR) facilitates image creation in any desired two-dimensional (2D) plane that may best depict the patient’s anatomy, while three-dimensional (3D) volume rendering (VR) provides an overall perspective. In addition, maximum intensity projection (MIP) techniques are ideally suited to highlighting vascular structures. Disadvantages of CT include risks associated with ionizing radiation exposure, which are of greater significance in the population most likely to be imaged for venous compression (young patients, premenopausal women) (4). Moreover, achieving optimal venous contrast opacification can prove challenging in patients with variable venous physiology. In addition, the robust bolus geometry enjoyed at arterial vascular CT imaging is lost, as venous imaging requires crossing of both the pulmonary and systemic capillary beds, yielding less bright venous opacification and more difficult timing (1).
MRI
In recent years, MRI with MRV has emerged as a leading imaging modality in the assessment of venous compression syndromes. Although exam times typically exceed those of CT, the potential risks of ionizing radiation exposure are mitigated, and optimal venous contrast timing is in general more readily achieved. Noncontrast exams are also possible in patients with renal insufficiency, who are at increased risk of nephrogenic systemic fibrosis (NSF) with gadolinium administration. Like CT, MRI datasets allow for robust 2D and 3D postprocessing techniques. Surpassing CT capabilities, MR flow-sensitive sequences also allow assessment of venous flow directionality, helping to predict the hemodynamic significance of compressive lesions. Many advances in MRV are credited to the growing (albeit off-label) use of blood-pool contrast agents, namely gadofosveset trisodium (Ablavar™, Lantheus Medical Imaging, North Billerica, MA, USA), which allow excellent venous and arterial visualization during the steady-state phase (1). An alternative to gadofosveset is the ultra-small superparamagnetic iron oxide nanoparticle ferumoxytol (Feraheme™, AMAG Pharmaceuticals, Waltham, MA, USA), which has shown promise as a cardiovascular MR contrast agent in investigational studies and can be used even in patients with end-stage renal disease. However, historically high reported rates of contrast reactions limit its potential use; safety and efficacy research is ongoing (7-10).
Conventional venography
Conventional invasive catheter venography remains the gold standard for diagnosis and may demonstrate thrombus, pressure gradients, or positional flow alterations within the compressed vein (1). In addition, endovascular treatments may be performed at the time of venography. With continued refinements in noninvasive imaging modalities, the use of invasive venography can be limited to cases that present diagnostic challenges or in which intervention is planned. Moreover, some anatomical features such as bony landmarks are better demonstrated by cross-sectional imaging methods, which assist in pre-surgical planning. Ultimately, the decision to undertake invasive venography should be made only after reviewing all available clinical data and alternative, less invasive imaging options have been exhausted.
Spectrum of disorders
MTS
First described by May and Thurner in 1957, MTS, also referred to as Cockett’s syndrome, IVCS, and iliocaval compression syndrome, is characterized by compression of the left common iliac vein (LCIV) between the right common iliac artery (RCIA) anteriorly and the 5th lumbar vertebral body posteriorly (Figure 1) (1-3,11,12). The RCIA both mechanically compresses the LCIV against the vertebral body and repetitively contacts it due to arterial pulsation (3). While even ≥50% compression is observed in up to 1/4 of normal individuals, chronic compression and pulsation may cause endothelial damage and intimal fibrosis, leading to the development of intraluminal venous webs, channels, and spurs with resultant pressure gradients and distal slow flow (13). These factors predispose the affected lower extremity to venous hypertension, stenosis, obstruction, and DVT (13,14).
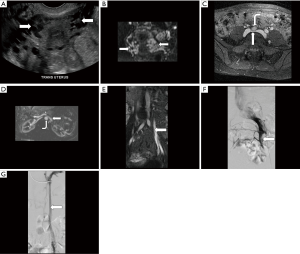
The true incidence and prevalence of MTS remain uncertain (14), although the syndrome is ultimately diagnosed in 2–5% of patients being evaluated for a chronic venous disorder and may contribute to 18–49% of cases of left-sided DVT (2,3). The existence of MTS may also help account for the 3–8 times greater likelihood of DVT in the left compared to the right leg (12). Individuals in the 2nd through 4th decades of life are most commonly affected, with a female:male ratio of 5:1 (3,13). The clinical presentation is variable and has been divided into three stages: asymptomatic LCIV compression (stage 1), intraluminal spur formation (stage 2), and left iliac DVT (stage 3) (13,15). Symptoms may include lower extremity swelling and pain, most commonly due to acute DVT but sometimes secondary to chronic venous congestion in the absence of clot (1-4). Other chronic findings may include exertional pain, varicose veins, telangiectasias, skin hyperpigmentation, venous eczema, and ulceration (1-4,13,15). Occasionally, patients may present with pulmonary embolism, stroke, or even myocardial infarction secondary to paradoxical emboli (1,13). Ultimately, the syndrome may not be clinically apparent unless other factors predisposing to DVT such as trauma, surgery, malignancy, immobility, pregnancy, and oral contraceptive use are also present, precipitating an acute event (13,15). A dreaded complication is phlegmasia cerulea dolens characterized by massive iliofemoral DVT, which may lead to acute limb ischemia and a risk of venous gangrene (4,12).
Duplex US of the lower extremities with Doppler is typically the first radiologic test obtained in the evaluation of MTS (13,15). To optimize the exam, a 4–7 megahertz (MHz) linear array transducer is suggested to image the common femoral vein, while a 2–3 MHz transducer is preferred for the iliocaval veins (14,16). The Doppler angle of insonation should be <60 degrees, and B-mode can be used to measure the minimal vein diameter and compare it to normal segments (14,16). While US is highly accurate for detection of acute iliofemoral DVT, assessment of the iliac veins is not possible in 20–50% of exams due to their location deep within the pelvis and overlying bowel gas, exacerbated by large body habitus and variation in sonographer expertise (3,14,15). Even if the iliac veins are not visualized, several sonographic findings should raise suspicion for a more proximal compression or obstruction. These include: reduced or absent respiratory variation and response to augmentation and Valsalva maneuvers; an abnormal Doppler spectral tracing at the stenotic area; and a “noisy”, mosaic Doppler appearance in the poststenotic vessel indicating turbulent flow (1,14). In addition, a peak vein velocity (PVV) ratio, calculated as poststenotic divided by prestenotic velocity, of more than 2.0 is considered significant (14). Although invasive, the additional use of intravascular ultrasound (IVUS) may facilitate more precise diagnosis and endovascular treatment planning (17).
Both CT and MRV are useful tools in the further workup of suspected MTS. CT is in general less expensive, less time-consuming, more ubiquitous, and better able to assess stent patency post intervention, whereas MR has no ionizing radiation, mitigates mixing artifact, can be performed without contrast, and allows quantification of flow magnitude and direction (1,13). When CT is performed, a scan delay of at least 90–100 seconds after the start of intravenous contrast injection is recommended for optimal opacification of the iliac veins. Narrow collimation and thin reconstruction slices allow optimal 2D and 3D reformats, while new techniques such as iterative reconstruction and dual-energy acquisition allow improved or sustained image quality despite radiation and contrast dose reduction (13). CT allows direct visualization of iliac vein compression, which is often best seen in the axial plane (4,13). The full extent of iliofemoral venous thrombosis is demonstrated with greater accuracy than is possible using US (3,4,13). Other findings may include collateral vessels, lower extremity swelling, venous congestion, and perivenous inflammation (13). CT is also useful for excluding alternative causes of venous compression such as pelvic masses (4,18). Findings supportive of MTS may be visible on CT exams tailored for other indications; thus scrutiny of the iliac and lower extremity veins and IVC is important on all scans covering these anatomic regions. Even acute DVT may be evident on noncontrast scans as an intraluminal hyperattenuating structure (13).
MRV for MTS typically includes time-of-flight sequence and multiphase 3D pre- and post-contrast T1-weighted gradient echo images, which are begun approximately 2 minutes after the start of contrast injection. Blood pool contrast agents such as gadofosveset help to minimize contrast dose and better ensure appropriate venous timing (13). The major diagnostic benefit of MRV compared to CTV is the ability to visualize retrograde flow in the ipsilateral internal iliac vein and in collateral veins (ascending lumbar, presacral, transpelvic, abdominal wall) (1). It has been shown that iliac vein compression may change dramatically on MRV exams repeated within a short time period due to such factors as volume depletion and differences in patient positioning. Thus, the finding of apparent iliac vein compression on a single exam without other ancillary features is not sufficient to diagnose MTS (15,19). A recent report showed a higher prevalence of LCIV compression in patients with cryptogenic stroke undergoing MRV compared to normal controls (20).
Conventional venography is considered the gold standard imaging test for MTS but now usually reserved for cases in which intervention is planned or noninvasive modalities are equivocal (15). The hemodynamic significance of MTS may be assessed via pressure gradient measurement across the area of iliofemoral stenosis; a gradient >2 mmHg at rest or >3 mmHg during exercise is considered significant (1,13,15). Flow reversal in the ipsilateral internal iliac vein, cross-pelvic collaterals, and enlargement of the ascending lumbar vein may also be observed and are related to chronic venous hypertension (1).
Patients with MTS anatomy but no DVT may be managed conservatively with compression stockings. When acute DVT is present, catheter-directed thrombolysis and anticoagulation is also indicated (1). However, invasive therapy is also needed to correct the underlying mechanical venous compression and prevent recurrent DVT. In the past, surgical techniques such as thrombectomy followed by vessel relocation or venous bypass were favored (2). However, catheter-directed endovascular venous thrombolysis using pharmacological (e.g., tissue plasminogen activator) and mechanical thrombectomy followed by self-expanding stent placement is now preferred (1,13,14,21). The procedure may even be performed in a single session (22). Long-term primary and secondary stent patency rates are generally excellent, estimated in the range of 80% and 90%, respectively, at 1 year post therapy without significant change in subsequent years (1,13,23). However, anticoagulation and compression stockings are still recommended after stent placement to prevent stent occlusion and recurrent DVT (1).
Variant IVCS
A variety of less common forms of iliac vein compression have been described, which some term MTS variants (1). These include: compression of the LCIV by a tortuous left common iliac artery (LCIA) or left internal iliac artery (LIIA); compression of the right common iliac vein (RCIV) by the RCIA, LCIA, or right internal iliac artery (RIIA); compression of the left external iliac vein (LEIV) by the left external iliac artery (LEIA); compression of the right external iliac vein (REIV) by the right external iliac artery (REIA); and compression of the IVC by the RCIA, more frequent with a high aortic bifurcation (Figure 2) (24-28). Rarely, an aneurysm of the RCIA may cause compression of the LCIV (1). In addition, non-vascular structures may cause extrinsic compression of the IVC or iliac veins. Reported culprits of iliocaval compression include: lymphadenopathy, tumor, a distended bladder, endometriosis, and penile prostheses (Figure 3) (13,29-36).
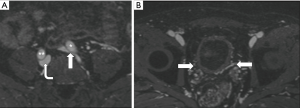
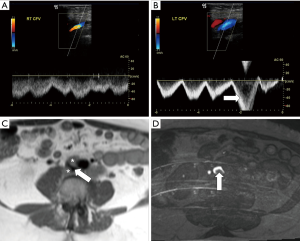
The clinical features, radiologic workup, and imaging findings in this heterogeneous group of disorders are analogous to those described in MTS, although less well systematically studied. The goal of imaging should be to elucidate the underlying anatomy and its hemodynamic significance in preparation for possible intervention. Management strategies in general mimic those of MTS, accounting for anatomical differences. However, in the case of non-vascular compression, the underlying cause of extrinsic iliocaval mass effect (e.g., neoplasm) must be addressed. Similarly, compressive aneurysms may require targeted intervention.
VTOS
The thoracic outlet (cervicothoracobrachial junction) consists of three confined anatomic compartments: the interscalene triangle, the costoclavicular space, and the retropectoralis minor space (sometimes referred to as the subcoracoid space) (37). Thoracic outlet syndrome (TOS) is characterized by dynamic compression of structures coursing between the thoracic outlet and axilla via the cervico-axillary tunnel (38). Three types have been described: neurogenic, arterial, and venous, usually caused by compression of the brachial plexus, subclavian artery, and subclavian vein, respectively. Venous TOS that results in primary axillosubclavian vein thrombosis is also known as Paget-Schroetter syndrome (formerly effort thrombosis); however, Paget-Schroetter is sometimes used interchangeably with venous TOS in general (Figure 4) (1,39). In addition, McCleery syndrome denotes symptomatic intermittent obstruction in venous TOS without thrombosis (38). Unlike neurogenic and arterial TOS, in which compression occurs within the interscalene triangle formed by the anterior and middle scalene muscles and first rib, venous TOS arises in the costoclavicular space bordered by the costoclavicular ligament/subclavius muscle, clavicle, and first rib (1,37,39). Many underlying etiologies may facilitate venous compression in the costoclavicular space, such as hypertrophy of the costoclavicular ligament, scalene muscles, or scalene tendons secondary to repetitive compression cycles (1,39). Other precipitating factors include the presence of a cervical rib, medical clavicular osteophytes, callus from prior fracture, tumor, and even automatic implantable cardiac defibrillation (AICD) insertion (1,39-41). As in other compression syndromes, stasis in the compressed vein leads to venous hypertension, while continued microtrauma causes endothelial damage and eventual thrombosis; with chronic thrombosis, the vein becomes fibrotic, and collaterals form in the neck and upper chest (1).
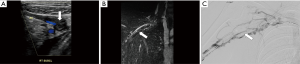
Venous TOS is rare, with an estimated annual incidence of 1–11/100,000 (1,39). However, it is the second-most common form of TOS, comprising 4% of cases; the neurogenic and arterial forms constitute the other 95% and 1%, respectively (42). While TOS is overall more common in women with a female:male ratio of 4:1, the venous form is more frequent in men by a 2:1 margin (39,42,43). Affected patients are typically young, with a peak age of onset in the 30s (1,39). The right arm is more commonly affected, thought to be related to a higher per population percentage of right-hand dominance (39). Characteristically, venous TOS affects athletic individuals who pursue physically intensive activities that involve repetitive upper arm motion including weightlifting, baseball, swimming, javelin-throwing, rowing, rock-climbing, judo, and cheerleading (39,44-47). Similarly, the syndrome has been reported in a variety of professions involving heavy upper extremity use ranging from soldiers to television cameramen to kebab chefs (1,48-50). Underlying thrombophilia also increases the risk of venous TOS precipitating DVT (39). Patients with venous TOS may be asymptomatic but typically present with unilateral arm pain and swelling; hand cyanosis and enlarged chest wall and shoulder collateral veins may also be apparent (1). Nevertheless, the syndrome is commonly mis- or underdiagnosed by general practitioners (39). A variety of physical exam provocative maneuvers causing effective narrowing of the thoracic outlet have been described and are intended to increase diagnostic accuracy (37,43). However, they are now known to be unreliable (39). Thus, imaging plays a central role in diagnosis.
Widely available, generally safe, and relatively inexpensive, radiographs of the chest and cervical spine are considered first-line in the imaging evaluation of all forms of TOS to evaluate for potential bony abnormalities. A variety of osseous pathology may be elucidated, including cervical ribs, anomalies of the first rib or clavicle, an elongated C7 transverse process, degenerative disease, and bone destruction related to a primary or secondary neoplasm (3,37,43). Nevertheless, radiographs are ultimately only useful for screening purposes, as findings such as compressive soft tissue tumors (e.g., lung cancer) may be readily missed (43).
Duplex US is the initial test choice in suspected venous TOS with upper extremity DVT (Paget-Schroetter presentation). It is highly accurate for detecting acute thrombus in the subclavian, axillary, and more distal deep veins of the arm, with reported sensitivity and specificity of 78–100% and 82–100%, respectively (1,39). In addition, US is a rapid and relatively low-cost exam that can be performed portably with no ionizing radiation (43). Nonetheless, US does have limitations in DVT identification, such as the inability to adequately visualize the proximal subclavian vein at the costoclavicular junction and perform compression maneuvers in this location (1,39). The value of US in venous TOS without thrombus is equivocal (39). Certainly, a unique technical advantage of US compared to other modalities is its dynamic capabilities. The axillosubclavian veins can be imaged with the patient upright, sitting, or supine, while various provocative maneuvers are performed such as arm abduction. Axillosubclavian venous flow acceleration raises suspicion for narrowing at the thoracic outlet and can be correlated with those body/arm positions that reproduce symptoms; absent flow suggests occlusion (37,39,43). Nonetheless, the clinical significance of these imaging findings remains uncertain (43). Moreover, deeper pathology such as tumor is readily missed. Therefore, US alone is not considered an adequate imaging strategy (37,43).
Both CT and MR with angiographic and venographic techniques allow more precise anatomical definition (1). Scans are typically performed first with the arms adducted (“neutral” position) and then elevated (provocative maneuver) to exacerbate thoracic outlet narrowing. A typical CT protocol consists of injecting 90 mL iodinated contrast at 4 mL/second via the contralateral arm with either bolus tracking over the ascending aorta or an empiric scan delay of 50–60 seconds for venous opacification. A sample MR protocol may consist of high-resolution T1- and T2-weighted sequences followed by 3D MRA and MRV (37,43). Both modalities accurately depict underlying anatomical abnormalities as well as the presence and extent of DVT, the location of venous compression, and any collateral vein formation (1). Of note, reduction in venous (unlike arterial) diameter or cross-sectional area after arm elevation is common among normal asymptomatic controls and thus not a reliable sign in elucidating venous TOS. Some suggest that ≥50% subclavian venous diameter decrease with abduction is supportive of the diagnosis of venous TOS. Advantages of CT include rapid acquisition and precise bone detail, whereas MR offers better soft tissue contrast (e.g., muscle and nerve delineation) with no ionizing radiation and the potential for noncontrast (albeit artifact-prone) vessel imaging. Limitations common to both CT and MR include supine-only positioning and inability to elevate the upper arm >130 degrees (due to small scanner width); these factors may result in underdiagnosis (37,43).
Contrast venography, once considered the imaging gold standard for venous TOS, is now reserved for situations in which intervention is planned or other modalities are equivocal (1,37,39,43). It is effective in demonstrating venous thrombosis and stenosis (>50% considered significant) as well neck and chest wall collaterals, which are typical of chronic occlusion (1,39). Venography, unlike CT and MR, allows more flexibility to position the arm in abduction and external rotation, which may help elucidate the underlying compression and its hemodynamic significance (1). However, venography fails to show the external compressive structures and nerve bundles, which are best demonstrated on cross-sectional imaging (43).
The current standard treatment for venous TOS manifested by acute DVT (Paget-Schroetter variant) is mechanical or pharmacologic catheter-directed thrombolysis, followed by decompressive surgery such as clavicle, rib, or muscle resection or tendon release (1). These invasive methods are combined with oral anticoagulation for at least 3–6 months and in the short-term limb elevation, warm compresses, and bed rest (1,42). A transaxillary operative approach is often preferred (38). Nevertheless, there are no absolute guidelines for the management of venous TOS, and the timing of surgery is a point of contention (43,44). Refraining from surgery altogether has been reported, although data on long-term DVT recurrence are not available (51).
NCS
NCS is characterized by symptomatic compression of the left renal vein (LRV) between the abdominal aorta posteriorly and superior mesenteric artery (SMA) anteriorly (1-4,52-57). If asymptomatic, it is called nutcracker phenomenon (NCP) (52,56,57). NCS is also known as mesoaortic LRV compression, LRV entrapment syndrome, and “anterior” NCS (1,54,56,57). A “posterior” NCS has also been described (approximately 20% of all NCS) cases, in which a circumaortic (17%) or retroaortic (3%) LRV is compressed between the aorta and vertebral body (54,56-58). Increased LRV and capillary pressure related to IVC venous outflow obstruction results in the formation of left-predominant venous collaterals such as dilation of the gonadal vein, which is prone to reflux (Figures 1,5) (1,3,4). Other potential collaterals may include dilated parapelvic, capsular, periureteral, adrenal, and ascending lumbar veins (53). Venous rupture into a renal calyceal fornix is also possible (1,59). Factors that may contribute to the characteristic location of compression in NCS include: the 3 times longer length of the left compared to right renal vein, an abnormally high LRV course, a narrow angle of origin or branching pattern of the SMA, periaortic fibrosis tissue, posterior renal ptosis (3,54,58,60). Nonetheless, as in MTS/IVCS, many variations in nutcracker anatomy and renal vein compression have been described. These include compression of the LRV between the aorta and duodenum, which is in turn compressed by the SMA (SMA or Wilkie syndrome), and compression of the LRV between the SMA and right renal artery (RRA) (57,59,61). While not “true” NCS, extrinsic compression of the LRV may also occur from a wide variety of structures, including pancreatic and retroperitoneal tumors, para-aortic lymphadenopathy, the testicular artery, and an abnormally lordotic lumbar spine (4,57,59). RRV compression has also been described in association with a gravid uterus (57).
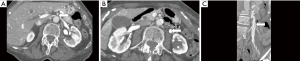
The prevalence of NCS remains unknown, in part due to the lack of universally accepted diagnostic criteria (3,4,53-55,57). It is more common in thin women in the 2nd through 4th decades of life, with a possible second peak in middle age (1,3,54,57). Lack of mesenteric and retroperitoneal fat is thought to contribute to a reduction in aortomesenteric angle (AMA), predisposing to LRV compression (53). The most common and characteristic clinical presentation is unilateral and otherwise unexplained left hematuria (as confirmed by cystoscopy), caused by variceal rupture into the renal collecting system (53,57). Other presenting symptoms may include left flank and abdominal pain, chronic fatigue, and orthostatic proteinuria (1). Associated significant reflux into the gonadal vein (ovarian vein in women and testicular vein in men) causes a variety of secondary clinical findings in both genders. Men may develop left testicular pain, a left-sided varicocele, and infertility. Women may experience symptoms of pelvic congestion (pelvic pain, dysuria, dysmenorrhea, dyspareunia, and postcoital ache) due to engorgement of the parametrial and ovarian venous plexuses and pelvic, genital, and thigh varices (1,3,4). Ultimately, because the symptoms in NCS are so heterogeneous, the diagnosis is frequently not considered based on clinical grounds (4).
US is often used to initially evaluate for NCS, with a reported sensitivity and specificity of 78% and 100%, respectively (1,3). The site of LRV compression can be identified as well as any collateral vessels (dilated gonadal, adrenal, and lumbar veins, pelvic varices, varicocele) (1). A mirror image of the SMA in the aorta due to near-parallel proximal course has reported specificity of 99% for NCP though a sensitivity of only 50% (57). Doppler allows visualization of retrograde LRV flow and flow patterns in collaterals that correlate with renocaval pressure gradients (53,54). In addition, a variety of measurements are considered supportive of NCS. These include: ratio of anteroposterior (AP) diameter of the LRV at the renal hilum to the diameter at the point of compression >5, peak velocity (PV) at the point of LRV compression >100 cm/second, and ratio of PV at the renal hilum to PV at the compression point >3 when supine and >5 when standing (for at >15 minutes) (1,3,4,53,54,59,62,63). However, the inherent operator dependence of US may make these cutoffs less reliable in clinical practice (1).
Both CTV and MRV effectively demonstrate the exact location of LRV compression between the aorta and SMA and have replaced intravenous urography (IVU) (3,4). The LRV proximal to the point of stenosis may be dilated, thus creating a “beak sign” (1,54). Morphology and course of the LRV (preaortic, retroaortic, or circumaortic) can also be readily assessed (1). Unlike US, routine CTV and MRV techniques do not offer flow visualization or measurement (53). However, the full extent of collaterals, which suggest hemodynamic significance, is well seen, often based on coronal reconstructions (3,4). As on US, an LRV AP diameter ratio >5 is considered significant (54). Other supportive measurements, often best assessed on sagittal images, include an AMA <16–41 degrees (normal approximately 90 degrees), an aortomesenteric distance (AMD) ≤3 mm (normal 10–14 mm), and a “beak” angle >32 degrees (2,4,54). Finally, both CT and MR help to exclude alternative cause of renal vein compression such as an underlying mass (57).
Retrograde left renal venography remains the gold-standard for diagnosing NCS (3). However, it is an invasive and uncomfortable procedure and thus should be reserved for cases where there is strong suspicion or intervention is anticipated (53,59). A retrocaval pullback pressure gradient of >3 mmHg between the LRV and IVC provides the strongest evidence of NCS (1).
Most patients are managed conservatively. Thin patients may experience symptom relief with weight gain. Treatment nonresponders who experience more severe symptoms such as significant hematuria, orthostatic proteinuria, or pain are candidates for more invasive options (1). A variety of surgical approaches have been described, such as collateral ligation, venous transposition or bypass, SMA transposition, and even renal autotransplantation into the contralateral iliac fossa (1,53,59,64). Minimally invasive endovascular stenting of the LRV appear promising but can be prone to significant complications such as in-stent thrombosis with subsequent pulmonary embolism (1).
PVC
PVC refers to symptomatic extrinsic narrowing of the popliteal vein by adjacent musculoskeletal structures in the popliteal fossa. It is a subtype of popliteal entrapment syndrome (PES), which refers more generally to popliteal artery, popliteal vein, or tibial nerve compression (65). Concurrent arterial and venous involvement is not unusual, as the vessels course together (1). Usually, the compression is related to an anomalous course or hypertrophy of the medial head of gastrocnemius muscle (1,2,66). However, the popliteal vein may be compressed by other structures such as a popliteal (Baker) cyst, aneurysm, popliteus muscle, or fibrous band (1,67,68). The compression is typically dynamic, precipitated by active plantar flexion and full knee extension (Figure 6) (1).
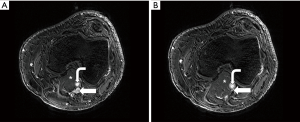
Anatomic compression of the popliteal vein is a common finding, reported to arise in 27% of normal individuals (69). However, the incidence of PVC causing symptoms remains unclear. In one small series of patients with popliteal artery entrapment (PAE), 13% had simultaneous venous compression (1,69). PAE, for which more data is available, has a prevalence of <1% but is the culprit for rest pain in 60% of young patients with limb ischemia; it predominantly affects men (male:female ratio of 9:1) and is bilateral in 22–67% of cases (1,2). Hemodynamically significant PVC may be manifested by a spectrum of limb ischemia from tingling toes to rest claudication, particularly with concurrent PAE in athletic individuals (66). Below-the-knee venous thrombosis and varices may also develop (1). Chronically, limb pain, swelling, edema, and congestive dermatitis may develop related to chronic venous insufficiency (1,66).
US with Doppler is a useful first-line imaging test. As previously described, it is highly accurate for identifying or excluding lower extremity DVT. In addition, the compression may be evident either at rest or only with a provocative maneuver (i.e., plantar flexion). Lesser saphenous vein reflux may also be evident (1). However, anatomic detail may be limited.
CT and MR with venographic techniques allow more precise anatomical definition, with MR offering superior visualization of fibrous bands, abnormal muscle insertions, and other soft tissue structures (1). MR blood pool contrast agents such as gadofosveset also allow vessel imaging up to 45 minutes after injection; thus scans may conceivably be performed both before and after provocative positioning (1,66). Although experience is limited, the off-label contrast use of ferumoxytol, which has a circulating half-life of 14–15 hours, may also be a unique option for enabling multiple acquisitions with various stress maneuvers (8-10).
Positional ascending venography is the gold-standard imaging test. However, as in other compression syndromes, invasive venography is reserved for cases in which noninvasive methods are inadequate. At the time of venography, pressure gradients in response to various provocative maneuvers can be measured. Changes of venous hypertension can also be established (1).
Conservative treatment with compressive stockings may sufficient. Anticoagulation is indicated if concurrent DVT is present. Ultimately, aberrant anatomy can only be corrected surgically, if symptoms warrant. PAE and PVC occurring together can be corrected in one operation; the timing should be based on the most pressing problem (1).
Conclusions
Imaging has assumed a central role in the diagnostic workup of venous compression syndromes, whose nonspecific symptoms in characteristically healthy, young patients might otherwise be overlooked, leading to serious consequences such as venous thrombosis or venous hypertension. US with Doppler is in general the preferred first test, but CT and MR with angiographic/venographic technique are typically superior in demonstrating the underlying anatomy and excluding alternative causes. Recent advances in MR such as blood pool contrast agents not only allow more visual detail but also improved prediction of hemodynamic significance, helping to define which patients will benefit most from therapy or can confidently forgo invasive venography. Definitive management may be surgical or endovascular, depending on the clinical situation. However, one must always remember to refrain from aggressive intervention for incidentally discovered venous compression in the absence of correlative hemodynamically significant symptoms. Continued refinements in noninvasive imaging may help further unravel this enigmatic group of disorders and promote evermore tailored treatment at the time it is most needed.
Acknowledgements
None.
Footnote
Conflicts of Interest: The authors have no conflicts of interest to declare.
References
- Butros SR, Liu R, Oliveira GR, et al. Venous compression syndromes: clinical features, imaging findings and management. Br J Radiol 2013;86:20130284. [Crossref] [PubMed]
- Noorani A, Walsh SR, Cooper DG, et al. Entrapment syndromes. Eur J Vasc Endovasc Surg 2009;37:213-20. [Crossref] [PubMed]
- Eliahou R, Sosna J, Bloom AI. Between a rock and a hard place: clinical and imaging features of vascular compression syndromes. Radiographics 2012;32:E33-E49. [Crossref] [PubMed]
- Lamba R, Tanner DT, Sekhon S, et al. Multidetector CT of vascular compression syndromes in the abdomen and pelvis. Radiographics 2014;34:93-115. [Crossref] [PubMed]
- Katz DS, Fruauff K, Kranz AO, et al. Imaging of deep venous thrombosis: A multimodality overview. Appl Radiol 2014. Available online: http://appliedradiology.com/articles/imaging-of-deep-venous-thrombosis-a-multimodality-overview
- Gulsun Akpinar M, Goodman LR. Imaging of pulmonary thromboembolism. Clin Chest Med 2008;29:107-16. [Crossref] [PubMed]
- Finn JP, Nguyen KL, Han F, et al. Cardiovascular MRI with ferumoxytol. Clin Radiol 2016;71:796-806. [Crossref] [PubMed]
- Walker JP, Nosova E, Sjgovan M, et al. Ferumoxytol-enhanced magnetic resonance angiography is a feasible method for the clinical evaluation of lower extremity arterial disease. Ann Vasc Surg 2015;29:63-8. [Crossref] [PubMed]
- Ruangwattanapaisarn N, Hsiao A, Vasanawala SS. Ferumoxytol as an off-label contrast agent in body 3T MR angiography: a pilot study in children. Pediatr Radiol 2015;45:831-9. [Crossref] [PubMed]
- Vasanawala SS, Nguyen KL, Hope MD, et al. Safety and technique of ferumoxytol administration for MRI. Magn Reson Med 2016;75:2107-11. [Crossref] [PubMed]
- May R, Thurner J. The cause of the predominantly sinistral occurrence of thrombosis of the pelvic veins. Angiology 1957;8:419-27. [Crossref] [PubMed]
- Cil BE, Akpinar E, Karcaaltincaba M, et al. Case 76: May-Thurner syndrome. Radiology 2004;233:361-5. [Crossref] [PubMed]
- Lugo-Fagundo C, Nance JW, Johnson PT, et al. May-Thurner syndrome: MDCT findings and clinical correlates. Abdom Radiol (NY) 2016;41:2026-30. [Crossref] [PubMed]
- Mousa AY, AbuRahma AF. May-Thurner syndrome: update and review. Ann Vasc Surg 2013;27:984-95. [Crossref] [PubMed]
- Brinegar KN, Sheth RA, Khademhosseini A, et al. Iliac vein compression syndrome: Clinical, imaging and pathologic findings. World J Radiol 2015;7:375-81. [Crossref] [PubMed]
- Oguzkurt L, Tercan F, Pourbagher MA, et al. Computed tomography findings in 10 cases of iliac vein compression (May-Thurner) syndrome. Eur J Radiol 2005;55:421-5. [Crossref] [PubMed]
- Ahmed HK, Hagspiel KD. Intravascular ultrasonographic findings in May-Thurner syndrome (iliac vein compression syndrome). J Ultrasound Med 2001;20:251-6. [PubMed]
- Wu WL, Tzeng WS, Wu RH, et al. Comprehensive MDCT evaluation of patients with suspected May-Thurner syndrome. AJR Am J Roentgenol 2012;199:W638-45. [Crossref] [PubMed]
- McDermott S, Oliveira G, Ergül E, et al. May-Thurner syndrome: can it be diagnosed by a single MR venography study? Diagn Interv Radiol 2013;19:44-8. [PubMed]
- Prabhakar AM, Misono AS, Brinegar KN, et al. Use of Magnetic Resonance Venography in Screening Patients With Cryptogenic Stroke for May-Thurner Syndrome. Curr Probl Diagn Radiol 2016;45:370-2. [Crossref] [PubMed]
- Zhang X, Shi X, Gao P, et al. Endovascular management of May-Thurner syndrome: A case report. Medicine (Baltimore) 2016;95:e2541. [Crossref] [PubMed]
- Zhu QH, Zhou CY, Chen Y, et al. Percutaneous manual aspiration thrombectomy followed by stenting for iliac vein compression syndrome with secondary acute isolated iliofemoral deep vein thrombosis: a prospective study of single-session endovascular protocol. Eur J Vasc Endovasc Surg 2014;47:68-74. [Crossref] [PubMed]
- Jeon UB, Chung JW, Jae HJ, et al. May-Thurner syndrome complicated by acute iliofemoral vein thrombosis: helical CT venography for evaluation of long-term stent patency and changes in the iliac vein. AJR Am J Roentgenol 2010;195:751-7. [Crossref] [PubMed]
- Abboud G, Midulla M, Lions C, et al. "Right-sided" May-Thurner syndrome. Cardiovasc Intervent Radiol 2010;33:1056-9. [Crossref] [PubMed]
- Fretz V, Binkert CA. Compression of the inferior vena cava by the right iliac artery: a rare variant of May-Thurner syndrome. Cardiovasc Intervent Radiol 2010;33:1060-3. [Crossref] [PubMed]
- Steinberg JB, Jacocks MA. May-Thurner syndrome: a previously unreported variant. Ann Vasc Surg 1993;7:577-81. [Crossref] [PubMed]
- Molloy S, Jacob S, Buckenham T, et al. Arterial compression of the right common iliac vein; an unusual anatomical variant. Cardiovasc Surg 2002;10:291-2. [Crossref] [PubMed]
- Dheer S, Joseph AE, Drooz A. Retroperitoneal hematoma caused by a ruptured pelvic varix in a patient with iliac vein compression syndrome. J Vasc Interv Radiol 2003;14:387-90. [Crossref] [PubMed]
- Kibbe MR, Ujiki M, Goodwin AL, et al. Iliac vein compression in an asymptomatic patient population. J Vasc Surg 2004;39:937-43. [Crossref] [PubMed]
- Palma L, Peterson MC, Ingebretsen R. Iliac vein compression syndrome from urinary bladder distension due to prostatism. South Med J 1995;88:959-60. [Crossref] [PubMed]
- Ducharme SE, Herring D, Tripp HF. Unilateral iliac vein occlusion, caused by bladder enlargement, simulating deep venous thrombosis. J Vasc Surg 1999;29:724-6. [Crossref] [PubMed]
- Vaidyanathan S, Hughes P, Soni BM, et al. Occlusion of left common iliac vein by a distended urinary bladder in a male with paraplegia due to spinal cord injury. Spinal Cord 2001;39:394-8. [Crossref] [PubMed]
- Rosengarten AM, Wong J, Gibbons S. Endometriosis causing cyclic compression of the right external iliac vein with cyclic edema of the right leg and thigh. J Obstet Gynaecol Can 2002;24:33-5. [Crossref] [PubMed]
- da Justa DG, Bianco FJ Jr, Ogle A, et al. Deep venous thrombosis due to compression of external iliac vein by the penile prosthesis reservoir. Urology 2003;61:462. [Crossref] [PubMed]
- Janczak D, Ruciński A, Skóra J, et al. Iliac-femoral vein thrombosis as a first symptom of the isolated common and internal iliac artery aneurysm. Wiad Lek 2000;53:458-61. [PubMed]
- Rosenthal D, Matsuura JH, Jerius H, et al. Iliofemoral venous thrombosis caused by compression of an internal iliac artery aneurysm: a minimally invasive treatment. J Endovasc Surg 1998;5:142-5. [Crossref] [PubMed]
- Demondion X, Herbinet P, Van Sint Jan S, et al. Imaging assessment of thoracic outlet syndrome. Radiographics 2006;26:1735-50. [Crossref] [PubMed]
- Klaassen Z, Sorenson E, Tubbs RS, et al. Thoracic outlet syndrome: a neurological and vascular disorder. Clin Anat 2014;27:724-32. [Crossref] [PubMed]
- Moore R, Wei Lum Y. Venous thoracic outlet syndrome. Vasc Med 2015;20:182-9. [Crossref] [PubMed]
- Werner-Gibbings K, Dubenec S. Medial clavicular osteophyte: a novel cause of paget-schroetter syndrome. Case Rep Vasc Med 2015;2015:723182.
- Wadhawan A, Laage Gaupp FM, Sista AK. Automatic implantable cardiac defibrillator implantation may precipitate effort-induced thrombosis in young athletes: a case report and literature review. Clin Imaging 2014;38:510-4. [Crossref] [PubMed]
- Kellar J, Trigger C. Thoracic outlet syndrome with secondary Paget Schroetter Syndrome: a rare case of effort-induced thrombosis of the upper extremity. West J Emerg Med 2014;15:364-5. [Crossref] [PubMed]
- Moriarty JM, Bandyk DF, Broderick DF, et al. ACR Appropriateness Criteria imaging in the diagnosis of thoracic outlet syndrome. J Am Coll Radiol 2015;12:438-43. [Crossref] [PubMed]
- Thiruchelvam N, Mbuvah F, Kistangari G, et al. Upper-limb deep vein thrombosis in Paget-Schroetter syndrome. Cleve Clin J Med 2015;82:658-9. [PubMed]
- Lutter C, Monasterio E, Schöffl V. Rock climbing-related subclavian vein thrombosis. BMJ Case Rep 2015;2015. pii: bcr2015212021.
- Ijaopo R, Oguntolu V. A case of Paget-Schroetter syndrome (PSS) in a young judo tutor: a case report. J Med Case Rep 2016;10:63. [Crossref] [PubMed]
- Chu AS, Harkness J, Witmer CM. Spontaneous subclavian vein thrombosis in a healthy adolescent cheerleader: A case of Paget-Schroetter syndrome. Pediatr Emerg Care 2016. [Epub ahead of print]. [Crossref] [PubMed]
- Bullock C, Johnston AM. Upper extremity deep vein thrombosis in a military patient. J R Army Med Corps 2016;162:299-301. [Crossref] [PubMed]
- Beasley R, Braithwaite I, Evans R. Upper extremity deep vein thrombosis in a TV cameraman. Occup Med (Lond) 2015;65:337-9. [Crossref] [PubMed]
- Aytekin E, Dogan YP, Okur SC, et al. Differential diagnosis of a rare case of upper limb pain: Paget-Schroetter syndrome in a doner kebab chef. J Phys Ther Sci 2015;27:3333-5. [Crossref] [PubMed]
- Edo Fleta G, Torres Blanco Á, Gómez Palonés F, et al. Combined non-surgical treatment for Paget-Schroetter syndrome: a case report. J Med Case Rep 2016;10:171. [Crossref] [PubMed]
- Quevedo HC, Arain SA, Abi Rafeh N. Systematic review of endovascular therapy for nutcracker syndrome and case presentation. Cardiovasc Revasc Med 2014;15:305-7. [Crossref] [PubMed]
- Takeyama PH, Bhatt S, Dogra VS. Nutcracker syndrome. Appl Radiol 2012. Available online: http://appliedradiology.com/articles/nutcracker-syndrome
- Fong JK, Poh AC, Tan AG, et al. Imaging findings and clinical features of abdominal vascular compression syndromes. AJR Am J Roentgenol 2014;203:29-36. [Crossref] [PubMed]
- Grimm LJ, Engstrom BI, Nelson RC, et al. Incidental detection of nutcracker phenomenon on multidetector CT in an asymptomatic population: prevalence and associated findings. J Comput Assist Tomogr 2013;37:415-8. [Crossref] [PubMed]
- Poyraz AK, Firdolas F, Onur MR, et al. Evaluation of left renal vein entrapment using multidetector computed tomography. Acta Radiol 2013;54:144-8. [Crossref] [PubMed]
- Kurklinsky AK, Rooke TW. Nutcracker phenomenon and nutcracker syndrome. Mayo Clin Proc 2010;85:552-9. [Crossref] [PubMed]
- Cuéllar i Calàbria H, Quiroga Gómez S, Sebastià Cerqueda C, et al. Nutcracker or left renal vein compression phenomenon: multidetector computed tomography findings and clinical significance. Eur Radiol 2005;15:1745-51. [Crossref] [PubMed]
- Avgerinos ED, McEnaney R, Chaer RA. Surgical and endovascular interventions for nutcracker syndrome. Semin Vasc Surg 2013;26:170-7. [Crossref] [PubMed]
- Venkatachalam S, Bumpus K, Kapadia SR, et al. The nutcracker syndrome. Ann Vasc Surg 2011;25:1154-64. [Crossref] [PubMed]
- Polguj M, Topol M, Majos A. An unusual case of left venous renal entrapment syndrome: a new type of nutcracker phenomenon? Surg Radiol Anat 2013;35:263-7. [Crossref] [PubMed]
- Kim SH, Cho SW, Kim HD, et al. Nutcracker syndrome: diagnosis with Doppler US. Radiology 1996;198:93-7. [Crossref] [PubMed]
- Shin JI, Park JM, Lee JS, et al. Doppler ultrasonographic indices in diagnosing nutcracker syndrome in children. Pediatr Nephrol 2007;22:409-13. [Crossref] [PubMed]
- White JV, Ryjewski C, Messersmith RN, et al. Left ovarian to left external iliac vein transposition for the treatment of nutcracker syndrome. J Vasc Surg Venous Lymphat Disord 2016;4:114-8. [Crossref] [PubMed]
- Sinha S, Houghton J, Holt PJ, et al. Popliteal entrapment syndrome. J Vasc Surg 2012;55:252-262.e30. [Crossref] [PubMed]
- Beitzke D, Wolf F, Juelg G, et al. Diagnosis of popliteal venous entrapment syndrome by magnetic resonance imaging using blood-pool contrast agents. Cardiovasc Intervent Radiol 2011;34 Suppl 2:S12-S16. [Crossref] [PubMed]
- Sanchez JE, Conkling N, Labropoulos N. Compression syndromes of the popliteal neurovascular bundle due to Baker cyst. J Vasc Surg 2011;54:1821-9. [Crossref] [PubMed]
- Misselbeck T, Dangleben D, Celani V. Isolated popliteal vein entrapment by the popliteus muscle: a case report. Vasc Med 2008;13:37-9. [Crossref] [PubMed]
- Raju S, Neglen P. Popliteal vein entrapment: a benign venographic feature or a pathologic entity? J Vasc Surg 2000;31:631-41. [Crossref] [PubMed]