Computer simulation help predict the frame deformation following a Venus-A transcatheter aortic valve implantation in patients with pure aortic regurgitation: a retrospective study
Highlight box
Key findings
• Patient-specific computer modelling of transcatheter aortic valve implantation (TAVI) may help predict frame deformation in Chinese patients with pure aortic regurgitation (AR). The main anchoring at the aortic annular level was similar in the migration group and the non-migration group, while the forces at the sinotubular junction (STJ) help stabilize the device within the aortic root.
What is known and what is new?
• TAVI is recommended as the major treatment option for patients with aortic stenosis (AS), but may be a risk in the treatment for pure AR. The patient-specific computational modeling with FEops HEARTguide (FEops, Ghent, Belgium) based on computed tomography (CT) images has been applied to predict the risk of TAVI in AS patients.
• The patient-specific computational modeling with FEops HEARTguide based on CT images helps predict the frame deformation in Chinese patients with pure AR in this study. The forces at the STJ help stabilize the device within the aortic root.
What is the implication, and what should change now?
• The patient-specific computational modeling with FEops HEARTguide may need to be applied in large samples to validate its efficacy in predicting the risk for pure AR.
• The force at the STJ might be used as a discriminator to identify patients at risk of device migration prior to intervention.
Introduction
Background
Transcatheter aortic valve implantation (TAVI) is recommended as the major treatment option for patients with aortic stenosis (AS) (1). As demonstrated by the PARTNER trial, TAVI is a valid alternative with a lower surgical risk in patients with severe AS who are not eligible for valve replacement or who are at high operative risk (2,3).
TAVI typically relies on a heavily calcified native aortic valve for radial stent fixation and prevention of valve migration, and critical fluoroscopic landmarking to guide safe implantation (2). Pure aortic regurgitation (AR) is considered a contraindication for TAVI because the limited calcium volume on the aortic valve might compromise device anchoring (4). Small case studies have reported an increased risk of device migration in pure AR TAVI (4,5). A meta-analysis that included 11 eligible studies concluded that new-generation devices have higher device success, a lower complication rate, and a lower all-cause mortality rate than early-generation devices (5). Given the limited experience, however, the device anchoring mechanism in AR patients has not been established (6).
Sizing the aortic annulus correctly and identifying the location and depth of transannular stent fixation also represent challenges in treating AR patients with TAVI (7). Pre-procedural planning consists of an intraprocedural transesophageal echocardiogram, computed tomography (CT) fusion-guided imaging, and other methods to aid in selecting the appropriate size and positioning of the device (8,9). However, current imaging modalities do not always allow for accurate measurements of the anatomy and may not provide the exact contours of the aorta required for TAVI (10). Therefore, it is essential to standardize the technique for appropriate device sizing and positioning to improve clinical outcomes.
Rationale and knowledge gap
CT images are commonly used in the clinical practice. Recently, patient-specific computational modeling with FEops HEARTguide (FEops, Ghent, Belgium) based on pre-procedural CT was introduced as a potential tool for TAVI (11,12). This cloud-based technology uses acquired pre-procedural CT images to accurately predict the interaction between the implanted TAVI device and the surrounding anatomy for patients with bicuspid and TAVs (13-15). These three-dimensional computer models provide detailed insight that cannot be obtained through peri- and post-procedural imaging. Furthermore, the predictive value of the computer simulation for the risk of a paravalvular leak (PVL) and new conduction abnormalities following TAVI in patients with AS has been documented in small-scale studies (11,16). However, the computer simulation to predict the risk of prosthesis malposition in patients with pure AR is rarely reported.
Using the FEops HEARTguide with different device sizes and implant positions can be simulated in a patient-specific anatomic model to identify the optimal size/position combination that reduces the risk for new conduction disturbances and PVL. However, because TAVI in patients with AR is limited, there are no studies that have determined the potential of the FEops HEARTguide for pre-operative planning of the Venus-A TAVI system in patients with pure AR. Due to the current technology limitation, FEops can only be used in venus for finite element simulation.
Objective
In this study, we validated the predictive value of the FEops HEARTguide for frame deformation following a Venus-A TAVI implant in patients with pure AR. Furthermore, we used the validated computational model to evaluate the anchoring mechanism of the Venus-A device within the same cohort. Indeed, the results of this study may promote the clinical application of TAVI in patients with pure AR. We present this article in accordance with the STROBE reporting checklist (available at https://cdt.amegroups.com/article/view/10.21037/cdt-24-60/rc).
Methods
Patient characteristics
This was a single-center, retrospective study. The study was approved by the Ethics Committee of Sir Run Run Shaw Hospital, School of Medicine, Zhejiang University (No. 2023-E-0003) and complied with the Declaration of Helsinki (as revised in 2013). Written informed consent was waived by the ethics committee for this retrospective study but oral consent was obtained from the patients. The study included 30 patients with pure AR who had successfully undergone TAVI using a Venus A-valve from April 2019 to January 2023 in the Sir Run Run Shaw Hospital, School of Medicine, Zhejiang University. These patients were divided into the migration group (n=10) and the non-migration group (n=20). The flow chart of the participants included is shown in Figure 1. The inclusion criteria were as follows: (I) severe AR (defined as echocardiographic evidence of aortic regurgitant bundles >65%) of the left ventricular outflow tract width, or flow neck width of 0.6 cm, or reversal of proximal full diastolic flow in the abdominal aorta, or a regurgitant flow rate of ≥60 mL per beat, or a regurgitant flow fraction of ≥50%, or an effective regurgitant orifice area of ≥5.0%, or a contrast classification of 3 and 4, with left ventricular dilatation, according to the Expert Consensus on Clinical Pathway for Transcatheter Aortic Valve Replacement in China (2021 edition) (17); (II) signed informed consent before treatment; and (III) agree to complete all clinical follow-up evaluations. The exclusion criteria were as follows: (I) no CT images after TAVI; and (II) image quality did not meet the needs of computer simulation to reconstruct an accurate 3D model of the aortic root. Dedicated software (3 Mensio; Pie Medical Imaging BV, Maastricht, The Netherlands) was used for quantitative analysis of the aortic root in all patients (18). The three-class grading scheme of PVL, as described in Valve Academic Research Consortium 3, was used in this study (19).
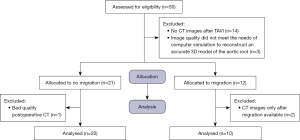
TAVI procedure
Each patient gave written informed consent before treatment. The right femoral artery was exposed and punctured after general anesthesia was administered and a 6-F femoral sheath was placed. The right radial artery was punctured, and a 5-F radial artery sheath was placed. A 5-F pigtail catheter was placed in the aortic root via a retrograde route and a contrast examination was performed. A loach wire guided the AL2 catheter to the aortic root. A 260-cm straight loach guidewire was replaced to guide the AL2 catheter through the aortic valve and a 20-F aortic sheath was placed. A Lunderquist guidewire was placed into the left ventricle via an AL2 catheter, then the AL2 catheter was withdrawn. Contrast imaging of the aortic root was obtained to visualize the annulus size and regurgitation. The appropriate Venus-A valve model (Venus Medtech, Hangzhou Qiming Co. Ltd., Hangzhou, China) was selected based on the combined intraoperative transesophageal ultrasound results and preoperative CT evaluation. The valve delivery system followed the Lunderquist guidewire to the level of the annulus. The release position was adjusted under contrast imaging. Contrast imaging of the valve after release confirmed the valve position and determined if a complication had occurred. The blood pressure and other cardiac indicators were monitored, the valve position, and leaflet opening and closing were monitored by transesophageal ultrasound in the immediate postoperative period. The system was withdrawn after confirmation of normalcy. The hemodynamic indices were closely monitored, the patient was transferred to the monitoring room for observation after stabilization, and the patient was transferred back to the general ward for treatment after sustained stabilization. The surgical procedure can be referenced according to the Expert Consensus on Clinical Pathway for Transcatheter Aortic Valve Replacement in China (2021 edition) (17). The surgical procedures were performed by the same transcatheter aortic valve replacement (TAVR) surgery team with experience of more than 6 years, who performed over 110 TAVR surgeries annually.
Computer simulations
The FEops HEARTguide technology was used to simulate the virtual implantation of a Venus-A valve model in a patient-specific geometry by the same TAVR surgery team with experience of more than 6 years, who performed over 110 TAVR surgeries annually. FEops HEART guide technology was used in the postoperative period with preoperative CT images. Briefly, the 3D geometrical model of all aortic roots, including the calcified native leaflets, was reconstructed from pre-procedural CT images using image segmentation software (Mimics v21.0; Materialise, Leuven, Belgium), as shown in Figure 2. Different linear elastic material models were assigned to different tissue regions. An elastic modulus (2 and 0.6 MPa) was assigned to the native aortic wall and leaflets, respectively, while a stiffer elastic material with perfect plasticity (E =4 MPa; yield stress =0.6 MPa) was used for the calcifications (11,12).
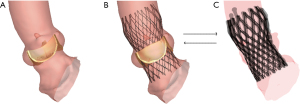
In the finite-element analysis simulation, the crimped Venus-A valve model was positioned co-axially within the aortic root and deployed by retracting the sheath. The device size was in line with the implant in each simulation.
The successful TAVI technique was defined in Valve Academic Research Consortium-3 (VARC-3), as follows: (I) technical success (successful transfer from the operating room); (II) alive; (III) successful establishment of access, device delivery, and device retrieval; (IV) correct positioning of individual prosthetic heart valves in the correct anatomic location; and (V) freedom from surgical or interventional procedures or interventions related to the device, and major vascular-, access-, or cardiac structure-related complications. The temporal onset of device migration was defined as post-procedural.
Device migration did not occur in 20 of the 30 patients and was used to validate the predicted frame deformation. The simulated device position was aligned with the clinical procedure for each patient. The device implantation was iteratively simulated until the final device position matched the actual depth of implantation, as extracted from post-procedural CT images by overlaying the predicted and postoperative frames (Figure 2).
Device migration occurred in 10 of the 30 patients. It was not possible to simulate the device position prior to migration in the 10 patients. Therefore, the device was simulated at target implantation depth 4–5 mm below the aortic annular plane at the non-coronary cusp with physician acceptance.
Frame deformation comparison
The predicted frame deformation was quantitatively compared to the postoperative device deformation at multiple levels (ventricular end, nadir level, central coaptation, and commissural level). The minimum and maximum frame diameter, perimeter, and area were extracted at each level.
Anchoring force analysis
The outward forces acting on the frame at the last step of the computer simulation were extracted for each patient and the total outward force acting around the aortic annular (AA) and sinotubular junction (STJ) planes were recorded; 4.7 N at 5 mm above and below of STJ can be used as a factor to differentiate the risk of displacement. Specifically, 3 different bandwidths (3, 5, and 10 mm) defining the area of interest around the AA and STJ levels were analyzed (Figure 3).
Statistical analysis
Continuous variables are expressed as the mean ± standard deviation (SD) or median (lower and upper quartiles) and categorical variables are reported as numbers (percentage). The correlation between the predicted and observed continuous variables was analyzed using the coefficient of determination (R2). A comparison within the anchoring force analysis was performed using the Mann-Whitney U test for skewed variable distribution. Bland-Altman analysis was performed to analyze the consistency and correlation between the morphologic parameters of the observed (postoperative CT) and simulated (model) frame. Statistical significance was defined as a two-tailed P<0.05. The statistical analysis was performed with SciPy Stats, a Python module for probability functions and statistical distributions.
Results
Baseline and procedural details
Thirty patients with pure AR were enrolled in the study. The mean age was 70.43±8.37 years and 22 (73.3%) were male. Approximately one-half of the patients were classified as class III–IV according to the New York Heart Association class and 93.3% of the patients had tricuspid aortic valves (TAVs). The mean AA diameter was 25.78±2.60 mm and the mean STJ diameter was 33.37±5.17 mm. The mean ejection fraction was 53.16%±14.44%. An oversized prosthesis without pre- or post-dilatation was implanted in all patients. The majority of devices used were Venus-A 29 mm and Venus-A 32 mm. The mean depth of implantation was 10.95±7.54 mm. Additional details of the baseline clinical and procedural characteristics of the patients with pure AR are presented in Table 1.
Table 1
Indicators | Overall | No migration | Migration | P value |
---|---|---|---|---|
Number | 30 | 20 (66.7) | 10 (33.3) | – |
Age, years | 70.43±8.37 | 69.15±9.29 | 73.00±5.70 | 0.24 |
Male | 22 (73.3) | 12 (60.0) | 10 (100.0) | 0.02 |
NYHA class | 0.59 | |||
Class I | 0 | 0 | 0 | |
Class II | 14 (46.7) | 9 (45.0) | 5 (50.0) | |
Class III | 14 (46.7) | 9 (45.0) | 5 (50.0) | |
Class IV | 2 (6.7) | 2 (10.0) | 0 | |
Aortic valve morphology | 0.30 | |||
TAV | 28 (93.3) | 18 (90.0) | 10 (100.0) | |
Sievers type 0 | 0 | 0 | 0 | |
Sievers type 1 | 2 (6.7) | 2 (10.0) | 0 | |
Aortic root dimensions | ||||
Aortic annulus diameter, mm | 25.78±2.60 | 25.62±3.01 | 26.12±1.55 | 0.62 |
Sinotubular junction diameter, mm | 33.37±5.17 | 32.05±5.09 | 36.32±4.25 | 0.04 |
2D echocardiography | ||||
Ejection fraction, % | 53.16±14.44 | 55.46±13.96 | 48.56±15.03 | 0.22 |
Left ventricular end diastolic diameter, cm | 63.20±12.31 | 61.60±12.37 | 66.40±12.17 | 0.32 |
Left ventricular end systolic diameter, cm | 46.83±14.14 | 45.25±13.88 | 49.99±14.88 | 0.40 |
Left ventricular end diastolic wall thickness, cm | 10.11±2.29 | 10.05±1.94 | 10.22±3.00 | 0.85 |
Left ventricular end systolic wall thickness, cm | 11.16±2.29 | 10.91±2.19 | 11.67±2.53 | 0.40 |
Oversizing | 30 (100.0) | 20 (100.0) | 10 (100.0) | – |
Oversizing degree, % | 19.30±5.63 | 18.98±6.49 | 19.94±3.54 | 0.67 |
Pre-dilatation | 0 | 0 | 0 | – |
Post-dilatation | 0 | 0 | 0 | – |
Device size | 0.64 | |||
Venus-A 23 mm | 1 (3.3) | 1 (5.0) | 0 | |
Venus-A 26 mm | 2 (6.7) | 2 (10.0) | 0 | |
Venus-A 29 mm | 11 (36.7) | 7 (35.0) | 4 (40.0) | |
Venus-A 32 mm | 16 (53.3) | 10 (50.0) | 6 (60.0) | |
Depth of actual implantation, mm | 10.95±7.54 | 7.73±4.89 | 17.40±7.95 | <0.001 |
The data are presented as mean ± standard deviation or number (%). NYHA, New York Heart Association; TAV, transcatheter aortic valve.
Device migration results
Among the 30 patients, 10 had device migration. The device migrated in the aortic root and left ventricular outflow tract directions in 2 and 8 patients, respectively.
Compared to patients with no migration, patients with device migration were more likely to be male (100.0% vs. 60.0%; P=0.02) with a larger mean STJ diameter (36.32±4.25 vs. 32.05±5.09 mm; P=0.04) and deeper mean implantation death (17.40±7.95 vs. 7.73±4.89 mm; P<0.001), as shown in Table 1. No significant differences were detected for other baseline characteristics between the two groups. No differences were noted in conduction disturbances between the groups.
All patients who had device migration also had moderate PVL after TAVI and received a second TAVI device, the size of which was same as the first TAVI device. Post-TAVI PVL after the second device implantation was reduced to mild, scant, or nil. For the patients who experienced device migration and underwent a second device implantation, there were no subsequent occurrences of migration based on cardiography and cardiac ultrasound during follow up.
Analysis of predicted frame deformation
The mean difference and coefficient of determination between the post-operative and simulated frames are summarized in Table 2 and Figure 4. The dimensions of the simulated and observed frames had good correlations at Dmax (mean difference post-op model =0.06±1.31 mm, R2=0.88), Dmin (mean difference post-op model =0.20±1.23 mm, R2=0.91), perimeter (mean difference post-op model =0.42±3.53 mm, R2=0.92), and area (mean difference post-op model =−0.22±49.63 mm2, R2=0.92) (Table 2 and Figure 4).
Table 2
Measurement | Mean difference (post-op model) | R2 |
---|---|---|
Dmax (mm) | 0.06±1.31 | 0.88 |
Dmin (mm) | 0.20±1.23 | 0.91 |
Perimeter (mm) | 0.42±3.53 | 0.92 |
Area (mm2) | −0.22±49.63 | 0.92 |
Data are presented as mean ± standard deviation.
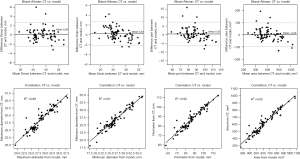
Comparison of predicted and clinical PVLs
The PVL predicted with the FEops HEARTguide was compared to the clinical evaluation before discharge for the 20 patients with no migration. All patients had nil or scant PVL post-TAVI. Similarly, simulations predicted low PVL (4.0±8.4 mL/s). Only 1 patient had a higher predicted PVL (35.5 mL/s).
Anchoring force analysis
The total outward force acting on the simulated frame in the region around the AA and STJ planes was extracted for all patients and compared between the migration and no migration groups. The extension of each region was defined by the bandwidth. Three different bandwidths (3, 5, and 10 mm) leading to different area extensions were investigated (Figure 3).
The predicted outward force acting on the frame at the AA level was comparable between the migration and no migration groups in any selected bandwidth. Instead, the predicted outward force acting on the frame at the STJ level was always significantly higher in the no migration group: bandwidth 3 mm (P=0.002), bandwidth 5 mm (P=0.005), bandwidth 10 mm (P=0.002). The results are summarized in Table 3.
Table 3
Bandwidths | Migration group | No migration group | P value |
---|---|---|---|
Outward forces at AA level (N) | |||
Bandwidth, 3 mm | 18.1 (14.5–19.0) | 15.9 (11.8–20.1) | 0.40 |
Bandwidth, 5 mm | 25.4 (20.5–28.0) | 20.4 (15.3–26.1) | 0.20 |
Bandwidth, 10 mm | 32.6 (24.7–34.1) | 27.8 (20.5–30.2) | 0.40 |
Outward forces at the STJ level (N) | |||
Bandwidth, 3 mm | 0.0 (0.0–0.0) | 0.9 (0.2–3.6) | 0.002 |
Bandwidth, 5 mm | 0.02 (0.0–0.8) | 1.7 (0.7–7.3) | 0.005 |
Bandwidth, 10 mm | 3.2 (1.9–4.4) | 11.7 (5.7–20.0) | 0.002 |
The data are presented as median (interquartile range). AA, aortic annular; STJ, sinotubular junction.
Discussion
Key findings
We evaluated the accuracy of the FEops HEARTguide, a patient-specific computational modeling in Chinese patients with pure AR treated with the Venus-A Valve in this observational, retrospective study. We compared the predicted device frame deformation with post-procedural CT findings, the dimensions of the simulated and observed frames had good correlations at Dmax, Dmin, perimeter, and area. Moreover, we evaluated the anchoring force of the simulated device in patients who did and did not have device migration. The anchoring forces acting on the frame at the AA level were comparable in patients who did and did not have device migration, while the force at the STJ level was significantly higher in patients who did not have device migration.
Explanations of findings
TAVI for isolated AR comprises <1.0% of all TAVI procedures that are performed (20). Even though several cases involving TAVI for isolated AR in a large and non-calcified annulus have been previously reported, treatment of AR is a challenge (21,22). Observational studies have shown that TAVI outcomes for AR are worse than TAVI outcomes for AS, including postoperative PVL, device migration, and new conduction abnormalities (4-6,23). It has been reported that the mismatch between the AA and the prosthetic aortic valve size is a factor determining postoperative PVL (24,25). The development and use of an accurate predictive simulator for TAVI may have important clinical relevance. The FEops HEARTguide, as a patient-specific computer simulation of TAVI, has been previously described and validated (15,16).
The frame deformation predicted by the FEops HEARTguide was shown to correlate well with the observed frame deformation in the current study; the small dimension differences were negligible. In addition, previous studies indicated that the FEops HEARTguide is an accurate predictor for risk of severe PVL in patients with AS (11,16). The PVL model prediction in patients with AR agreed with the clinical observations, enforcing the preoperative power of the tool in assisting cardiologists during preoperative planning. In fact, the FEops HEARTguide could help cardiologists to better understand the risks of the procedure and optimize device sizing and positioning for each individual prior to intervention.
In the absence of a calcified anchoring zone, the transcatheter aortic valve anchors within the aortic root due to a complex and dynamic interaction between the valve and the host (26). Therefore, the interaction between the prosthesis and anatomy occurs at multiple levels (left ventricular outflow tract, AA, aortic leaflets, STJ, and the initial segment of the ascending aorta). One strategy that has been used to promote prosthetic aortic valve anchoring in the absence of a calcified anchoring zone is to oversize the prosthetic aortic valve to promote contact force between the valve and the host (20). However, an oversized prosthetic aortic valve could cause severe complications, such as embolism, dissection, and conduction abnormalities (9,27). It is crucial to determine the key anchor position to increase the interaction force between the valve frame and the anatomy to reduce the occurrence of device migration while avoiding the complications caused by oversized devices.
The STJ anatomic parameters, including the height and diameter, are risk factors for postoperative malposition of the Venus valve (28,29). The tall aortic sinuses, the annulus perimeter, and the left ventricular outflow tract perimeter are predictors of Venus malposition (28). The larger and higher the STJ, and the greater the STJ-to-valve crown diameter ratio, can also help predict severe prosthesis malposition (29). The FEops HEART guide can reproduce the interaction between the device and the host by evaluating the interaction forces between the valve frame and the anatomy at different levels in patients with and without migration. In the current study the interaction force at the AA and STJ levels of two groups was predicted by the FEops HEARTguide. The results showed that the forces acting on the frame at the AA were comparable in patients who did and did not have device migration, while the forces acting on the frame at the STJ were significantly higher in patients who did not have device migration. This finding indicates that anchoring at the STJ helps to stabilize the device position, which avoids migration.
This study performed a computer simulation using the preoperative CT and compared the simulation data to the postoperative CT measurements, which subsequently validated consistency with the actual values. The results of this study were consistent with previous studies (11,16), which affirms the development and use of an accurate predictive simulator for TAVI may have meaningful clinical relevance. Overall, the advantage of using the preoperative computational modelling supports improved preprocedural planning. This finding may not only reduce the procedural time and radiation exposure but also the operative risk and postoperative complications.
Limitations
The present study had some limitations to acknowledge. First, this study was a retrospective, single-center study and the sample size was small. This study had the final clinical data but the cases studied were simulated using standard positions that might lead to biased results. A larger cohort study and randomized controlled trials are needed to further validate the findings. Ideally, using a larger cohort would define a cut-off value to discriminate patients at high risk of device migration. Second, this study only compared the interaction force at the AA and STJ levels between the no migration and migration groups. However, the interaction between the TAVI device and the surrounding anatomic structure is not clear and might occur at multiple levels. This analysis could be extended to evaluate the anchoring mechanism at multiple levels within the aortic root. Finally, the device position prior to migration could not be derived in the migration group and the device was implanted at the target position. To what extent the device position impacts the outward forces acting on the frame warrants a corollary study.
Conclusions
Patient-specific computer modelling of TAVI may help predict frame deformation in Chinese patients with pure AR. The main anchoring at the AA level was similar in the migration group and the non-migration group, while the forces at the STJ help stabilize the device within the aortic root. The force at the STJ might be used as a discriminator to identify patients at risk of device migration prior to intervention. Because this was a single-center, retrospective study with a small sample size, a larger cohort study and randomized controlled trials are needed to further validate the findings.
Acknowledgments
Funding: None.
Footnote
Reporting Checklist: The authors have completed the STROBE reporting checklist. Available at https://cdt.amegroups.com/article/view/10.21037/cdt-24-60/rc
Data Sharing Statement: Available at https://cdt.amegroups.com/article/view/10.21037/cdt-24-60/dss
Peer Review File: Available at https://cdt.amegroups.com/article/view/10.21037/cdt-24-60/prf
Conflicts of Interest: All authors have completed the ICMJE uniform disclosure form (available at https://cdt.amegroups.com/article/view/10.21037/cdt-24-60/coif). The authors have no conflicts of interest to declare.
Ethical Statement: The authors are accountable for all aspects of the work in ensuring that questions related to the accuracy or integrity of any part of the work are appropriately investigated and resolved. The study was approved by the Ethics Committee of Sir Run Run Shaw Hospital, School of Medicine, Zhejiang University (No. 2023-E-0003) and complied with the Declaration of Helsinki (as revised in 2013). Written informed consent was waived by the ethics committee for this retrospective study but oral consent was obtained from the patients.
Open Access Statement: This is an Open Access article distributed in accordance with the Creative Commons Attribution-NonCommercial-NoDerivs 4.0 International License (CC BY-NC-ND 4.0), which permits the non-commercial replication and distribution of the article with the strict proviso that no changes or edits are made and the original work is properly cited (including links to both the formal publication through the relevant DOI and the license). See: https://creativecommons.org/licenses/by-nc-nd/4.0/.
References
- Khan SA, Baron SJ. Point: patients with native aortic regurgitation can be treated with transcatheter aortic valve implantation. Heart 2021;107:1938-41. [Crossref] [PubMed]
- Smith CR, Leon MB, Mack MJ, et al. Transcatheter versus surgical aortic-valve replacement in high-risk patients. N Engl J Med 2011;364:2187-98. [Crossref] [PubMed]
- Bjursten H, Rasmussen M, Nozohoor S, et al. Infective endocarditis after transcatheter aortic valve implantation: a nationwide study. Eur Heart J 2019;40:3263-9. [Crossref] [PubMed]
- Schneeberger Y, Seiffert M, Schaefer A, et al. TAVI for Pure Non-calcified Aortic Regurgitation Using a Self-Expandable Transcatheter Heart Valve. Front Cardiovasc Med 2022;8:743579. [Crossref] [PubMed]
- Takagi H, Hari Y, Kawai N, et al. Meta-Analysis and Meta-Regression of Transcatheter Aortic Valve Implantation for Pure Native Aortic Regurgitation. Heart Lung Circ 2020;29:729-41. [Crossref] [PubMed]
- Ravalli F, Kossar AP, Takayama H, et al. Aortic Valve Regurgitation: Pathophysiology and Implications for Surgical Intervention in the Era of TAVR. Struct Heart 2020;4:87-98. [Crossref] [PubMed]
- Nakamura Y, Teefy P, Kiaii B, et al. Transcatheter aortic valve implantation in a patient with severe aortic insufficiency and minimal aortic annular calcification. Can J Cardiol 2013;29:1138.e9-1138.e11. [Crossref] [PubMed]
- Arias EA, Bhan A, Lim ZY, et al. TAVI for Pure Native Aortic Regurgitation: Are We There Yet? Interv Cardiol 2019;14:26-30. [Crossref] [PubMed]
- Roy DA, Schaefer U, Guetta V, et al. Transcatheter aortic valve implantation for pure severe native aortic valve regurgitation. J Am Coll Cardiol 2013;61:1577-84. [Crossref] [PubMed]
- Wang H, Hanna JM, Ganapathi A, et al. Comparison of aortic annulus size by transesophageal echocardiography and computed tomography angiography with direct surgical measurement. Am J Cardiol 2015;115:1568-73. [Crossref] [PubMed]
- de Jaegere P, De Santis G, Rodriguez-Olivares R, et al. Patient-Specific Computer Modeling to Predict Aortic Regurgitation After Transcatheter Aortic Valve Replacement. JACC Cardiovasc Interv 2016;9:508-12. [Crossref] [PubMed]
- Rocatello G, El Faquir N, De Santis G, et al. Patient-Specific Computer Simulation to Elucidate the Role of Contact Pressure in the Development of New Conduction Abnormalities After Catheter-Based Implantation of a Self-Expanding Aortic Valve. Circ Cardiovasc Interv 2018;11:e005344. [Crossref] [PubMed]
- Schultz C, Rodriguez-Olivares R, Bosmans J, et al. Patient-specific image-based computer simulation for theprediction of valve morphology and calcium displacement after TAVI with the Medtronic CoreValve and the Edwards SAPIEN valve. EuroIntervention 2016;11:1044-52. [Crossref] [PubMed]
- Dowling C, Bavo AM, El Faquir N, et al. Patient-Specific Computer Simulation of Transcatheter Aortic Valve Replacement in Bicuspid Aortic Valve Morphology. Circ Cardiovasc Imaging 2019;12:e009178. [Crossref] [PubMed]
- Halim J, Brouwer J, Lycke M, et al. Transcatheter aortic valve replacement: impact of pre-procedural FEops HEARTguide assessment on device size selection in borderline annulus size cases. Neth Heart J 2021;29:654-61. [Crossref] [PubMed]
- Liu X, Fan J, Mortier P, et al. Sealing Behavior in Transcatheter Bicuspid and Tricuspid Aortic Valves Replacement Through Patient-Specific Computational Modeling. Front Cardiovasc Med 2021;8:732784. [Crossref] [PubMed]
- Structural Heart Disease Specialized Committee of Cardiovascular Physicians Branch, Chinese Physicians Association (CPA). Expert consensus on clinical pathway for transcatheter aortic valve replacement in China (2021 edition). Chin J Interv Cardio 2022;30:7-16.
- El Faquir N, Rocatello G, Rahhab Z, et al. Differences in clinical valve size selection and valve size selection for patient-specific computer simulation in transcatheter aortic valve replacement (TAVR): a retrospective multicenter analysis. Int J Cardiovasc Imaging 2020;36:123-9. [Crossref] [PubMed]
- VARC-3 WRITING COMMITTEE. Valve Academic Research Consortium 3: Updated Endpoint Definitions for Aortic Valve Clinical Research. J Am Coll Cardiol 2021;77:2717-46. [Crossref] [PubMed]
- Huded CP, Allen KB, Chhatriwalla AK. Counterpoint: challenges and limitations of transcatheter aortic valve implantation for aortic regurgitation. Heart 2021;107:1942-5. [Crossref] [PubMed]
- Orzalkiewicz M, Bruno AG, Taglieri N, et al. Transcatheter Aortic Valve Replacement for Pure Aortic Regurgitation in a Large and Noncalcified Annulus. JACC Cardiovasc Interv 2021;14:e271-3. [Crossref] [PubMed]
- Graziani F, Mencarelli E, Burzotta F, et al. Early Hemodynamic and Structural Impact of Transcatheter Aortic Valve Replacement in Pure Aortic Regurgitation. JACC Cardiovasc Interv 2020;13:2582-4. [Crossref] [PubMed]
- Liu L, Chen S, Shi J, et al. Transcatheter Aortic Valve Replacement in Aortic Regurgitation. Ann Thorac Surg 2020;110:1959-65. [Crossref] [PubMed]
- Athappan G, Patvardhan E, Tuzcu EM, et al. Incidence, predictors, and outcomes of aortic regurgitation after transcatheter aortic valve replacement: meta-analysis and systematic review of literature. J Am Coll Cardiol 2013;61:1585-95. [Crossref] [PubMed]
- Hagar A, Li Y, Wei X, et al. Incidence, Predictors, and Outcome of Paravalvular Leak after Transcatheter Aortic Valve Implantation. J Interv Cardiol 2020;2020:8249497. [Crossref] [PubMed]
- Chodór P, Wilczek K, Przybylski R, et al. Percutaneous access to coronary arteries in patients after transcatheter aortic valve implantation procedures - is it a real problem? Postepy Kardiol Interwencyjnej 2019;15:274-82. [Crossref] [PubMed]
- Franzone A, Piccolo R, Siontis GCM, et al. Transcatheter Aortic Valve Replacement for the Treatment of Pure Native Aortic Valve Regurgitation: A Systematic Review. JACC Cardiovasc Interv 2016;9:2308-17. [Crossref] [PubMed]
- Li J, Sun Y, Zheng S, et al. Anatomical Predictors of Valve Malposition During Self-Expandable Transcatheter Aortic Valve Replacement. Front Cardiovasc Med 2021;8:600356. [Crossref] [PubMed]
- Wang Y, Yu S, Qian D, et al. Anatomic predictor of severe prosthesis malposition following transcatheter aortic valve replacement with self- expandable Venus-A Valve among pure aortic regurgitation: A multicenter retrospective study. Front Cardiovasc Med 2022;9:1002071. [Crossref] [PubMed]