Three-dimensional optical coherence tomography for guidance of percutaneous coronary intervention for coronary bifurcation disease: a review of current clinical applications
Introduction
Revascularization of coronary bifurcation lesions (CBLs) with percutaneous coronary intervention (PCI) entails technical challenges and carries a higher risk of periprocedural and long-term adverse events. Recent technological improvements have made the acquisition of three-dimensional optical coherence tomography (3D-OCT) datasets feasible. This article aims to provide a review of current clinical applications of 3D-OCT, which provide additive information on plaque distribution of bifurcation lesions, the optimal stent cell at the side branch (SB) ostium for recrossing the guidewire, stent expansion, apposition and edge dissection following stent implantation, and the neointimal formation for bifurcation stent during follow-up. Although 3D-OCT imaging presents in-depth insights into stent optimization for CBLs, clinical evidence for its improved prognosis remains to be established. Nonetheless, we are sanguine about its wide application in the future with the development and refinement of technology.
OCT and coronary revascularization
There are 2 primary applications of intravascular imaging: intravascular ultrasound (IVUS) and OCT. OCT is a near-infrared, interferometric, ‘histology-like’ imaging modality for evaluating the microstructure of coronary arteries with high spatial resolution of approximately 10–15 µm available for in vivo use to date (1). Moreover, OCT with higher signal-to-noise ratio and the ability to distinguish plaque components can achieve detailed visualization of vascular structures, which is superior to those of other imaging modalities, such as angiography and IVUS (2-4). Nevertheless, the physical curvature of the coronary arteries is not captured by OCT alone and requires an additional modality to complement the OCT (such as multiple angiographic planes or coronary CT angiography). Systematic review and network meta-analysis revealed that the use of OCT for guiding PCI improves long-term clinical outcomes compared with angiography-guided PCI (5,6). In fact, the latest guidelines from European Society of Cardiology (ESC)/EACTS have updated the indications for OCT for stent optimization to class IIa level recommendations, which is consistent with the recommendations for IVUS (7). In recent years, OCT is increasingly used to investigate the pathological mechanisms of coronary artery disease and/or to optimize the results of PCI (8-11), especially for bifurcation PCI (12). Overall, OCT can display the true condition of CBLs without overlapping or shortening, which frequently occurs on angiography, providing useful guidance for the treatment of CBL (13).
Challenges of PCIs for bifurcation lesions
CBL refers to severe stenosis of the main vessel (MV) and SB either separately or simultaneously, including left anterior descending, diagonal branch, left circumflex, obtuse marginal branch, distal bifurcation of the right coronary artery, and bifurcation of the left main (LM). It involves a large variety of complex anatomic subsets due to several factors including plaque burden and location, the angle between the MV and the SB and the diameter of the branch (14). As estimated by the European Bifurcation Club, CBLs account for 15% to 20% of all PCI (15). PCI for CBLs entails technical challenges with high risk of periprocedural risk and even long-term adverse cardiovascular events, driven by repeat revascularization and stent thrombosis (16), even in the era of the latest generation of drug-eluting stents and in the setting of complex bifurcation PCI.
Bifurcation stenting techniques are still being continuously refined, improved and developed. Stepwise layered provisional stenting (PS) is the most used strategy to treat CBLs, starting with implantation of a MV stent across the SB ostium followed by the proximal optimization technique and implantation of a second stent in the SB only when required (17). However, in clinical practice, it is difficult to define a “suboptimal” result for the SB ostium and there is no unified standard. Even in the absence of a suboptimal SB result, the necessity of clearing stent struts from the SB ostium continues remains controversial. If the single stent strategy cannot provide sufficient support for the ostium of the SB, the two-stent technique should be considered in patients with complex CBL stratified by the DEFINITION criteria (18,19). Nevertheless, the current high rates of restenosis and re-intervention in CBLs treating by 2-stent strategies may be due to factors such as the high metal mass at the stent overlap, irregular overlapping of struts at the carina, twisting of the SB stent, polymer rupture at the origin of SB, and uneven distribution of stent struts, as well as changes in natural flow dynamics (20).
When performing PCI for CBLs, the main challenges are the highly variable bifurcated anatomy and the extent of myocardium in jeopardy. Angiographic assessment of the three main sectors of the coronary bifurcation has limitations, including the degree of stenosis (e.g., area stenosis) and other predictors that jeopardize the bifurcation segment in question (e.g., plaque burden, carina tip angle, or distance from branching point to carina tip) (21), which affects the choice of PCI strategy for CBLs, thereby affecting the short-term and long-term outcomes of PCI. Undoubtedly, intracoronary imaging could overcome some important limitations of angiography. Findings from large observational cohort studies, randomized trials, and meta-analyses have shown that OCT-guided PCI might reduce major adverse cardiovascular events (5,6).
Advantage of 3D-OCT in PCIs
With the development of new OCT technologies, precise 3D reconstruction of coronary arteries can more accurately evaluate the morphology and function of plaques (13). Online automatically generated 3D reconstruction is available. However, current techniques cannot fully capture the complex morphology-modulated mechanical responses that affect plaque stability, leading to catastrophic failure and muting the benefit of device and drug interventions. Biomechanical simulation could assist in this prediction, but this requires extracting morphological features from OCT imaging to construct accurate 3D simulations of patients’ arteries (22,23). 3D-OCT can be used to gain a more informed understanding of the geometry and morphology of CBL (24). Following stent implantation, 3D-OCT can also guide the re-crossing of guide wires through stent struts jailing the SB ostium and more clearly display the jailing strut configuration, as well as the ideal position of the guidewire recrossing point and stent struct link connection, to confirm the optimal wiring and SB expansion and reduce acute incomplete stent apposition (1).
However, the curved centerline of the artery couldn't be obtained by the OCT. The actual images are reconstructed from OCT relative to the catheter tip and imaging is subject to rotational artifacts, which may have an impact on the vessel shape estimated from OCT. The combined application of biplane angiography and OCT Imaging can achieve quantitative analysis of 3D artery volume reconstructions (25).
Accurate assessment of CBL anatomy by 3D-OCT
A thorough understanding of the anatomical structure of CBLs is crucial for better procedural planning and clinical outcomes. The definition of coronary bifurcation angle is the angle between centerlines of the distal MV and the SB; the angle between crossing lines of the proximal MV line before the branch point and the SB axis at the point of divergence was defined as carina angle (Figure 1). At present, the bifurcation angle and carina angle are all considered as the critical factors influencing flow disturbance, plaque formation, PCI procedure, SB occlusion, and clinical outcomes (26). Both plaque shift (dilation or stent implantation can cause the lesion at the proximal or distal of the bifurcation to shift towards the SB) and carina shift (stent-induced increase in lumen diameter of the MV shifted the carina into the SB ostial) have been considered as a potential mechanism in the pinching of the SB during bifurcation stenting (Figure 2), since atheroma is rarely seen at the carina alone because of its high wall shear stress area. Therefore, accuracy and precision of bifurcation and carina angle measurements could help us better analyze the anatomical characteristics of CBLs and optimize stent implantation strategies.
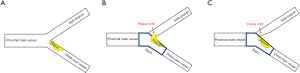
Images of coronary computed tomography angiography, quantitative coronary angiography, IVUS or OCT can be used for 3D reconstruction of CBL (26). However, coronary computed tomography angiography is limited by cardiac and lung motion and calcium blooming artifacts; 3D quantitative coronary angiography cannot provide the accurate bifurcation angle due to the inherent shortcomings of using two 2D angiography images which result in overlapping of branches and vessel foreshortening; IVUS lacks the imaging resolution to fully appreciate the complex architecture of the bifurcation (27). Notably, the bifurcation reconstructed by 3D-OCT can inform the operator about the precise anatomical structure (26,27). Using 3D-OCT to determine the type of CBLs, whether vertical or parallel, can serve as another supplement to visual assessment of bifurcation appearance (28). The perpendicular type was defined as a bifurcation in which the SB opening was visible as an elliptical shape and was not concealed by the carina when viewed perpendicular to the vessel wall on the cut-away view of the 3D-OCT image. In contrast, parallel type bifurcation was defined as a bifurcation in which the proximal course of the SB was concealed behind the carina. Nishimura et al. analyzed 60 CBLs treated by MV stenting with kissing balloon inflation under OCT guidance and found that the optimal cut-off distal bifurcation angle for predicting vertical bifurcations was 51.0° (29). Nonetheless, clinical implications of 3D-OCT bifurcation type are needed to be further investigated. In brief, evaluating the anatomical structure of CBLs through 3D-OCT is accurate, feasible, highly reproducible, and can be completed in a short time.
3D-OCT guided optimal guidewire rewiring into SB during bifurcation stenting
The optimal stenting procedure in bifurcations is still under debate (18,30), despite various techniques, devices and diagnostic methods currently available. In clinical practice, SB orifices would usually be narrowed or occluded due to plaque embolization, carina or plaque shift, spasm and dissection at the ostium, and interference by the stent struts after the implantation of stents in the MV. Especially, 3D-OCT can not only evaluate the anatomical characteristics of CBLs, but also clearly display the stent struct link location overhanging the SB ostium and guide rewiring of the distal cell of the jailed SB ostium prior to the final kissing balloon inflation to minimize the risk of struts pushed inside the MV creating a so-called de novo “metal carina” (31,32). Overhanging metallic struts in the SB ostium could lead to neointima bridges between the SB ostium and the metal structure, and when performing revascularization in the distal SB in future, it may hinder the entry of stents or balloons into SB (33). The computational flow simulation of the shear rate showed that after proximal re-crossing the remaining unopposed metal carina (strut jailing the SB) could cause an abnormal high shear rate level above 2,000 s−1, whereas after distal crossing and kissing balloon less disturbance of shear rate exists (34). Furthermore, the metal carina leads to flow disturbances, which is an important reason for the induction of platelet activation and stent thrombosis at the bifurcation stent site.
After the implantation of stent in bifurcation, configurations for the stent-link location overhanging the SB ostium displayed by 3D OCT can be divided into the link-free type and the link-connecting type. Results from a retrospective study (35) and a prospective multicenter registry (28) both showed that the incidence of incomplete stent apposition in cases with the link-free type was significantly lower than in those with the link-connecting type after the final kissing balloon inflation with distal cell guidewire rewiring. In a multicenter center registry study by Nagoshi et al. (36), 150 CBLs treated with single stenting following kissing balloon inflation, and results demonstrated that compared with 2D-OCT guidance, the incidence of incomplete stent apposition under 3D-OCT guidance was significantly reduced. The above study results indicate that it is feasible to select the guidewire recrossing point and consider the stent link position prior to the final kissing balloon inflation and that the stent link location in the SB ostium is out of control after stent implantation (35,36). Nowadays, the reconstruction of 3D-OCT images during the surgical process can be quickly completed online, and stent optimization can be completed simultaneously based on the results.
After MV stent implantation, when the guidewire passes through the stent cell to SB, the success rate of guidewire successfully passing through the distal stent cell to SB is 55–66% under angiography guidance, but under 3D-OCT guidance, the success rate can be increased to 87–100% (28,32,37). 3D-OCT imaging can also contribute to detect the guidewire partially or entirely recrosses outside the stent through the SB (38). Based on 3D-OCT images, although the guidewire passes through the optimal distal cell to SB, if the stent link is located at the SB ostia, severe stent deformation may occur during kissing balloon inflation, which may affect patients’ clinical outcomes. Surprisingly, the balloon-push technique (39), by which an inflated balloon is forced into the SB from the proximal MV is a feasible way to remove jailed struts without causing severe stent deformation. Nagoshi et al. analyzed the imaging data of 51 cases of bifurcation stent and found that it was feasible to remove jailed struts with the balloon-push technique, which would not cause severe stent deformation, and factors related to failure to remove jailed struts included a smaller balloon diameter/SB diameter ratio and trifurcation lesions (40). Thus, 3D-OCT guided guidewire rewiring into SB during bifurcation stenting significantly improves the success rate for optimal guidewire crossing, resulting SB ostia to have a large opening area.
In the multicenter-prospective 3D-OCT Bifurcation registry, 75 patients with LM (n=35) and non-LM (n=40) CBLs treated with single stent strategy were enrolled, and the relationship between the optimal rewiring position at the SB ostium and the SB ostial area was investigated (41). They found that the SB ostial areas with the optimal rewiring position showed no significant change at the post-procedure and at the 9-month follow-up regardless of whether LM or non-LM, however, the areas of SB ostia with the sub-optimal rewiring were significantly decreased at the 9-month follow-up.
Evaluation of post-PCI results by 3D-OCT
By utilizing the superior resolution of OCT, it is now possible to assess a broad spectrum of post-PCI stent- and vessel-related anomalies in three dimensions with a high resolution, including malapposed stent struts, underexpansion with calcification, stent edge dissection, residual focal lesion, jailed SB, geographic miss, residual stent edge disease (42). Additionally, by combining 3D-OCT with automatic strut detection and longitudinal view, the stent platform can be accurately identified (43). Especially exciting, the latest OCTOBER trial showed that OCT-guided PCI has a lower 2-year incidence of major adverse cardiac events at 2 years compared with angiography-guided PCI in patients with complex CBLs (12).
After using a single stent strategy for the treatment of CBLs, angiography usually reveals narrowing of the SB ostium (44,45), which may increase the subsequent risk of adverse clinical events. However, numerous studies reported that pressure wire detected no impairment of blood flow supply in jailed SB stenosis evaluated by angiography (46-48). After stenting in the MV, the pullback from the SB is not always feasible and implies additional contrast medium administration. Quantitative measurements of the SB ostial area based on 3D reconstructed OCT pullback from the MV may therefore have clinical application, facilitating the assessment as to whether the SB is hemodynamically obstructed. In the 3D-OCT analysis, the morphology of the SB ostium appeared elliptical instead of circular after MV stent crossover implantation, which leads to the appearance of stenosis in the SB ostium on angiography, overestimating the degree of stenosis in the SB ostium. Meanwhile, 3D-OCT measurements did not find any statistically significant changes in the minimum lumen area of the SB ostium during the subsequent 1–2 years follow-up (49). Therefore, 3D-OCT analysis may be helpful to guide decisions about additional intervention after CBL stent implantation compared with quantitative coronary angiography measurements.
The jailed strut at the SB orifice may be a cause of delayed neointimal coverage after single stenting to bifurcation, which would have an effect on the SB ostium area and might result in SB flow disturbance during long-term follow-up. A retrospective study showed that tissue coverage thickness and the ratio of uncovered to total struts was correlated, with high intra-observer and inter-observer agreement (41). It could also be a useful tool for assessing the safety of stent. Additionally, Kume et al. (50) analyzed 29 cases of 3D OCT-guided single-stent implantation for the treatment of CBLs and followed at 18 months after PCI, they found that although neointimal obstruction at the SB ostium was significantly greater in the link group than that in the no-link group, SB flow was not affected during the follow-up period. Just as inevitably, jailing of a stent strut link at the SB orifice after MV stent implantation should be avoided as much as possible. Similarly, Nakamura et al. (51) classified the configuration of overhanging struts at the SB orifice into three groups according to the 3D aspect of the jailing configuration: “No-jail type” (N-type), in which there were no overhanging struts at SB orifice; “Simple jail type” (S-type), in which there were overhanging struts, but no longitudinal stent link between crowns at the carina; and “Complex jail type” (C-type), in which there were overhanging struts with a longitudinal link connecting to the carina (Figure 3). Comparison of the change in the side-branch flow area from baseline to 6- to 12-month follow-up based on cut-plain 3D-OCT analysis revealed that the side-branch flow area in the N-type and S-type groups did not change remarkably, whereas that in the C-type group was significantly decreased and some of the compartments were filled with tissue. Serial changes in side-branch flow area were similar between different stents and might be influenced by jailed type complexity rather than by the type of drug eluting stent, suggesting that the complexity of the stent jail is associated with the progression of SB ostial stenosis. Thus, stents with fewer stent strut links at SB orifice might be better for CBL PCI employing a provisional single stent strategy. It is necessary to conduct further large-scale studies with long-term follow-up to determine the exact relationship between the jailed strut pattern at the SB orifice and SB flow disturbance as well as clinical outcomes due to delayed neointimal coverage.
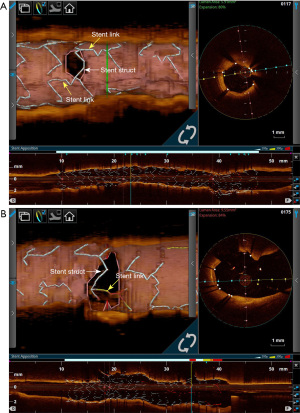
Conclusions
In general, the potential clinical applications of 3D-OCT include accurate assessment of CBL anatomy, optimal guidewire rewiring into SB during bifurcation stenting and evaluation of post-PCI results. Although the information from 3D-OCT imaging offers us with in-depth insights into stent optimization for CBL, its clinical evidence still needs to be established. In the future, 3D-OCT should be further improved in frame rates, pullback speeds and electrocardiogram-gated image acquisition, automated strut identification algorithms and quantitative measurements to provide more sensitive, specific, reproducible, and high-resolution measurements and improve early diagnosis and monitor diseases and expand its clinical application scenarios. Furthermore, a hybrid IVUS-OCT system can overcome the limitations associated with each technology and integrate the advantages of both modalities (52). Based on this fusion imaging system, 3D reconstruction may provide more information on blood vessels and lesions, thereby providing more accurate guidance for PCI for CBLs.
Acknowledgments
Funding: This work was supported by
Footnote
Peer Review File: Available at https://cdt.amegroups.com/article/view/10.21037/cdt-24-163/prf
Conflicts of Interest: All authors have completed the ICMJE uniform disclosure form (available at https://cdt.amegroups.com/article/view/10.21037/cdt-24-163/coif). The authors have no conflicts of interest to declare.
Ethical Statement: The authors are accountable for all aspects of the work in ensuring that questions related to the accuracy or integrity of any part of the work are appropriately investigated and resolved.
Open Access Statement: This is an Open Access article distributed in accordance with the Creative Commons Attribution-NonCommercial-NoDerivs 4.0 International License (CC BY-NC-ND 4.0), which permits the non-commercial replication and distribution of the article with the strict proviso that no changes or edits are made and the original work is properly cited (including links to both the formal publication through the relevant DOI and the license). See: https://creativecommons.org/licenses/by-nc-nd/4.0/.
References
- Araki M, Park SJ, Dauerman HL, et al. Optical coherence tomography in coronary atherosclerosis assessment and intervention. Nat Rev Cardiol 2022;19:684-703. [Crossref] [PubMed]
- Hartman EMJ, De Nisco G, Kok AM, et al. Wall shear stress-related plaque growth of lipid-rich plaques in human coronary arteries: an near-infrared spectroscopy and optical coherence tomography study. Cardiovasc Res 2023;119:1021-9. [Crossref] [PubMed]
- Ali ZA, Maehara A, Généreux P, et al. Optical coherence tomography compared with intravascular ultrasound and with angiography to guide coronary stent implantation (ILUMIEN III: OPTIMIZE PCI): a randomised controlled trial. Lancet 2016;388:2618-28. [Crossref] [PubMed]
- Yonetsu T, Jang IK. Cardiac Optical Coherence Tomography: History, Current Status, and Perspective. JACC Asia 2023;4:89-107. [Crossref] [PubMed]
- Giacoppo D, Laudani C, Occhipinti G, et al. Coronary Angiography, Intravascular Ultrasound, and Optical Coherence Tomography for Guiding of Percutaneous Coronary Intervention: A Systematic Review and Network Meta-Analysis. Circulation 2024;149:1065-86. [Crossref] [PubMed]
- Stone GW, Christiansen EH, Ali ZA, et al. Intravascular imaging-guided coronary drug-eluting stent implantation: an updated network meta-analysis. Lancet 2024;403:824-37. [Crossref] [PubMed]
- Byrne RA, Rossello X, Coughlan JJ, et al. 2023 ESC Guidelines for the management of acute coronary syndromes. Eur Heart J 2023;44:3720-826. [Crossref] [PubMed]
- Räber L, Ueki Y, Otsuka T, et al. Effect of Alirocumab Added to High-Intensity Statin Therapy on Coronary Atherosclerosis in Patients With Acute Myocardial Infarction: The PACMAN-AMI Randomized Clinical Trial. JAMA 2022;327:1771-81. [Crossref] [PubMed]
- Jia H, Dai J, He L, et al. EROSION III: A Multicenter RCT of OCT-Guided Reperfusion in STEMI With Early Infarct Artery Patency. JACC Cardiovasc Interv 2022;15:846-56. [Crossref] [PubMed]
- Kedhi E, Berta B, Roleder T, et al. Thin-cap fibroatheroma predicts clinical events in diabetic patients with normal fractional flow reserve: the COMBINE OCT-FFR trial. Eur Heart J 2021;42:4671-9. [Crossref] [PubMed]
- Ali ZA, Landmesser U, Maehara A, et al. Optical Coherence Tomography-Guided versus Angiography-Guided PCI. N Engl J Med 2023;389:1466-76. [Crossref] [PubMed]
- Holm NR, Andreasen LN, Neghabat O, et al. OCT or Angiography Guidance for PCI in Complex Bifurcation Lesions. N Engl J Med 2023;389:1477-87. [Crossref] [PubMed]
- Cai JZ, Man CY, Zhu YX. Clinical applications and research advances of optical coherence tomography in guiding percutaneous coronary intervention for bifurcation lesions. Zhonghua Xin Xue Guan Bing Za Zhi 2017;45:67-69. [PubMed]
- Han Y, Li Y. Techniques and Evidence for Percutaneous Coronary Intervention for Coronary Bifurcation Lesions: An Ongoing Journey. Cardiology Discovery 2022;2:131-3. [Crossref]
- Zhang JJ, Ye F, Xu K, et al. Multicentre, randomized comparison of two-stent and provisional stenting techniques in patients with complex coronary bifurcation lesions: the DEFINITION II trial. Eur Heart J 2020;41:2523-36. [Crossref] [PubMed]
- Moroni F, Shue-Min Yeh J, Attallah A, et al. Crush techniques for percutaneous coronary intervention of bifurcation lesions. EuroIntervention 2022;18:71-82. [Crossref] [PubMed]
- Albiero R, Burzotta F, Lassen JF, et al. Treatment of coronary bifurcation lesions, part I: implanting the first stent in the provisional pathway. The 16th expert consensus document of the European Bifurcation Club. EuroIntervention 2022;18:e362-76. [Crossref] [PubMed]
- Ge Z, Gao XF, Zhan JJ, et al. Coronary Bifurcation Lesions. Interv Cardiol Clin 2022;11:405-17. [Crossref] [PubMed]
- Kan J, Zhang JJ, Sheiban I, et al. 3-Year Outcomes After 2-Stent With Provisional Stenting for Complex Bifurcation Lesions Defined by DEFINITION Criteria. JACC Cardiovasc Interv 2022;15:1310-20. [Crossref] [PubMed]
- Rathore S, Tehrani S, Prvulovic D, et al. Drug coated balloons and their role in bifurcation coronary angioplasty: appraisal of the current evidence and future directions. Expert Rev Med Devices 2020;17:1021-33. [Crossref] [PubMed]
- Akase H, Okamura T, Nagoshi R, et al. Risk Assessment of Side Branch Compromise After Coronary Bifurcation Stenting- A Substudy of the 3D-OCT Bifurcation Registry. Circ J 2024;88:959-69. [Crossref] [PubMed]
- Straughan R, Kadry K, Parikh SA, et al. Fully automated construction of three-dimensional finite element simulations from Optical Coherence Tomography. Comput Biol Med 2023;165:107341. [Crossref] [PubMed]
- Carpenter HJ, Ghayesh MH, Zander AC, et al. Automated Coronary Optical Coherence Tomography Feature Extraction with Application to Three-Dimensional Reconstruction. Tomography 2022;8:1307-49. [Crossref] [PubMed]
- Ali ZA, Karimi Galougahi K, Thomas SV, et al. Optical Coherence Tomography-Guided Percutaneous Coronary Intervention: Practical Application. Interv Cardiol Clin 2023;12:215-24. [Crossref] [PubMed]
- Latus S, Neidhardt M, Lutz M, et al. Quantitative Analysis of 3D Artery Volume Reconstructions Using Biplane Angiography and Intravascular OCT Imaging. Annu Int Conf IEEE Eng Med Biol Soc 2019;2019:6004-7. [Crossref] [PubMed]
- Wu W, Samant S, de Zwart G, et al. 3D reconstruction of coronary artery bifurcations from coronary angiography and optical coherence tomography: feasibility, validation, and reproducibility. Sci Rep 2020;10:18049. [Crossref] [PubMed]
- Farooq V, Serruys PW, Heo JH, et al. New insights into the coronary artery bifurcation hypothesis-generating concepts utilizing 3-dimensional optical frequency domain imaging. JACC Cardiovasc Interv 2011;4:921-31. [Crossref] [PubMed]
- Okamura T, Nagoshi R, Fujimura T, et al. Impact of guidewire recrossing point into stent jailed side branch for optimal kissing balloon dilatation: core lab 3D optical coherence tomography analysis. EuroIntervention 2018;13:e1785-93. [Crossref] [PubMed]
- Nishimura T, Okamura T, Fujimura T, et al. Feasibility, reproducibility and characteristics of coronary bifurcation type assessment by three-dimensional optical coherence tomography. PLoS One 2022;17:e0263246. [Crossref] [PubMed]
- Cornelissen A, Guo L, Sakamoto A, et al. Histopathologic and physiologic effect of bifurcation stenting: current status and future prospects. Expert Rev Med Devices 2020;17:189-200. [Crossref] [PubMed]
- Onuma Y, Okamura T, Muramatsu T, et al. New implication of three-dimensional optical coherence tomography in optimising bifurcation PCI. EuroIntervention 2015;11 Suppl V:V71-4.
- Nagoshi R, Okamura T, Murasato Y, et al. Data on two- and three-dimensional optical coherence tomography guidance for the treatment for the bifurcation lesion. Data Brief 2017;16:865-8. [Crossref] [PubMed]
- Onuma Y, Grundeken MJ, Nakatani S, et al. Serial 5-Year Evaluation of Side Branches Jailed by Bioresorbable Vascular Scaffolds Using 3-Dimensional Optical Coherence Tomography: Insights From the ABSORB Cohort B Trial (A Clinical Evaluation of the Bioabsorbable Everolimus Eluting Coronary Stent System in the Treatment of Patients With De Novo Native Coronary Artery Lesions). Circ Cardiovasc Interv 2017;10:e004393. [Crossref] [PubMed]
- Foin N, Torii R, Alegria E, et al. Location of side branch access critically affects results in bifurcation stenting: Insights from bench modeling and computational flow simulation. Int J Cardiol 2013;168:3623-8. [Crossref] [PubMed]
- Okamura T, Onuma Y, Yamada J, et al. 3D optical coherence tomography: new insights into the process of optimal rewiring of side branches during bifurcational stenting. EuroIntervention 2014;10:907-15. [Crossref] [PubMed]
- Nagoshi R, Okamura T, Murasato Y, et al. Feasibility and usefulness of three-dimensional optical coherence tomography guidance for optimal side branch treatment in coronary bifurcation stenting. Int J Cardiol 2018;250:270-4. [Crossref] [PubMed]
- Onuma Y, Kogame N, Sotomi Y, et al. A Randomized Trial Evaluating Online 3-Dimensional Optical Frequency Domain Imaging-Guided Percutaneous Coronary Intervention in Bifurcation Lesions. Circ Cardiovasc Interv 2020;13:e009183. [Crossref] [PubMed]
- Murasato Y. How to use three-dimensional optical coherence tomography effectively in coronary bifurcation stenting. Front Cardiovasc Med 2022;9:1023834. [Crossref] [PubMed]
- Nagoshi R, Okamura T, Shite J. A Novel Push-Fold Method for Removing Side Branch-Jailed Stent Struts Under 3D Optical Coherence Tomography Guidance. JACC Cardiovasc Interv 2016;9:e107-9. [Crossref] [PubMed]
- Nagoshi R, Kijima Y, Kozuki A, et al. Success rate and influencing factors of a balloon-push technique: A new technique to remove side branch-jailed struts under three-dimensional optical coherence tomography guidance. Catheter Cardiovasc Interv 2023;101:528-35. [Crossref] [PubMed]
- Fujimura T, Okamura T, Nagoshi R, et al. Serial changes of the side-branch ostial area after single crossover stenting with kissing-balloon inflation. Int J Cardiovasc Imaging 2023;39:1593-603. [Crossref] [PubMed]
- van Zandvoort LJC, Ali Z, Kern M, et al. Improving PCI Outcomes Using Postprocedural Physiology and Intravascular Imaging. JACC Cardiovasc Interv 2021;14:2415-30. [Crossref] [PubMed]
- Cortes C, Chu M, Schincariol M, et al. Identification of the type of stent with three-dimensional optical coherence tomography: the SPQR study. EuroIntervention 2021;17:e140-8. [Crossref] [PubMed]
- Ahn JM, Lee JY, Kang SJ, et al. Functional assessment of jailed side branches in coronary bifurcation lesions using fractional flow reserve. JACC Cardiovasc Interv 2012;5:155-61. [Crossref] [PubMed]
- Vassilev DI, Kassab GS, Collet C, et al. Elliptical stretch as a cause of side branch ostial compromise after main vessel stenting in coronary bifurcations: New insights from numerical analysis. Cardiol J 2020;27:507-17. [PubMed]
- Koh JS, Koo BK, Kim JH, et al. Relationship between fractional flow reserve and angiographic and intravascular ultrasound parameters in ostial lesions: major epicardial vessel versus side branch ostial lesions. JACC Cardiovasc Interv 2012;5:409-15. [Crossref] [PubMed]
- Kang SJ, Kim WJ, Lee JY, et al. Hemodynamic impact of changes in bifurcation geometry after single-stent cross-over technique assessed by intravascular ultrasound and fractional flow reserve. Catheter Cardiovasc Interv 2013;82:1075-82. [Crossref] [PubMed]
- Grodecki K, Cadet S, Staruch AD, et al. Noncalcified plaque burden quantified from coronary computed tomography angiography improves prediction of side branch occlusion after main vessel stenting in bifurcation lesions: results from the CT-PRECISION registry. Clin Res Cardiol 2021;110:114-23. [Crossref] [PubMed]
- Yang PS, Ha J, Kim JS, et al. Eccentric morphology of jailed side-branch ostium after stent crossover in coronary bifurcation lesions: a three-dimensional optical coherence tomographic analysis. J Cardiol 2015;65:305-10. [Crossref] [PubMed]
- Kume T, Yamada R, Terumasa K, et al. Neointimal coverage of jailed side branches in coronary bifurcation lesions: an optical coherence tomography analysis. Coron Artery Dis 2018;29:114-8. [Crossref] [PubMed]
- Nakamura T, Okamura T, Fujimura T, et al. Serial changes in the three-dimensional aspect of the side-branch ostium jailed by a drug-eluting stent assessed by optical coherence tomography. Int J Cardiovasc Imaging 2017;33:797-806. [Crossref] [PubMed]
- Jia H, Zhao C, Yu H, et al. Clinical performance of a novel hybrid IVUS-OCT system: a multicentre, randomised, non-inferiority trial (PANOVISION). EuroIntervention 2023;19:e318-20. [Crossref] [PubMed]