Blood pool contrast agents for venous magnetic resonance imaging
Introduction
Despite technical imaging advances in the evaluation of the venous system, many challenges remain in the clinical application of these imaging techniques. Historically, one of the earliest imaging modalities used in venous imaging was ascending contrast venography, which is rarely used today due its invasiveness and risk of complications. Instead, ultrasound (US) has now become the modality of choice in clinical practice for the evaluation of deep vein thrombosis (DVT), with advantages in cost effectiveness, noninvasiveness, and portability in critically ill patients (1). However, key limitations of US exist, and include decreased sensitivity due to body habitus or edema, individual operator dependence (2), and difficulty in evaluation of calf veins and recurrent or chronic DVT (3). Ultrasound is also technically unable to evaluate the abdominopelvic deep venous system or the pulmonary arterial system (4), limiting its use in the workup of patients with venous thromboembolism at other sites.
Computed tomography is another available imaging tool for venous imaging, with advantages including widespread accessibility, noninvasiveness, rapid acquisition, accuracy, high spatial and temporal resolution and the option of reconstruction and post-processing on multiple planes (5) (Figure 1). A key disadvantage of CT is the need for ionizing radiation, especially in young or pregnant patients. The greatest challenge of CT venography, however, is ensuring sufficient and homogenous opacification of the all lower extremity veins (6), as absence of adequate opacification leads to a non-diagnostic study.
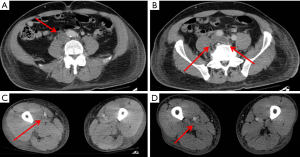
These challenges can be overcome by the use of magnetic resonance imaging (MRI). MR venography (MRV) can be performed with or without gadolinium based contrast. Non-contrast techniques include time-of-flight (TOF), gradient recalled echo (GRE), spoiled gradient recalled (SPGR) and steady state free precession (SSFP) sequences. Although an exhaustive overview is beyond the scope of this discussion, each technique suffers some limitations regarding length of acquisition times, background suppression, inflow effects, motion, and image artifacts. Gadolinium-enhanced magnetic resonance angiography (MRA) of the abdomen was first introduced in 1995 (7), and has the advantages of faster acquisition times, independence from inflow effects, and decreased image artifacts. In a study with 30 patients evaluated for lower extremity or abdominopelvic deep vein thrombosis with MRV, Huang et al. found that contrast enhanced MRV had a higher sensitivity and specificity compared to non-contrast MRV with gradient-recalled-echo sequence (91.0% and 99.8% for contrast-enhanced vs. 80.8% and 95.8% for GRE MRV); this study also demonstrate the additional benefit of decreased time for image acquisition and interpretation for contrast-enhanced MRV (8).
Historically, gadolinium based MRI contrast agents were considered to have a broad safety profile until 2006, when the relationship between gadolinium based contrast agents and nephrogenic systemic fibrosis (NSF) was described in patients with renal disease (9). NSF is a debilitating disease characterized by widespread fibrosis in the skin and connective tissues that is progressive and irreversible. Although the exact pathophysiology remains unclear, it is postulated that delayed excretion of the chelated gadolinium agent increases the risk for dissociation and subsequent proliferation of fibroblasts (10). However, with more selective contrast use and the advent of new chelated compounds, there have been no new documented cases of NSF since 2009.
More recently, serious attention has been paid to the observation made by McDonald et al. concerning the dose dependent association of intravenous gadolinium administration and brain deposition in patients with normal renal function (11). Deposition of gadolinium was found to be most concentrated in the dentate nucleus and globi pallidi, even in the presence of an intact blood brain barrier. This deposition occurs irrespective of renal or hepatobiliary dysfunction. The clinical consequences of gadolinium deposition are not known at this time but is currently an issue of great interest.
Gadolinium contrast agents (GCA) can be divided into extracellular, hepatobiliary and blood-pool agents according to their pharmacokinetic profile. Extracellular agents are most commonly used and share similar pharmacokinetic profile to that of iodinated contrast agents after intravenous injection (12-14). Elimination is exclusively by passive glomerular filtration in the kidneys. In patients without renal insufficiency, 98% of the administered GCA is excreted within 24 hours (12). Following the arterial first-pass phase, these agents are distributed to the extracellular compartment and rapidly diffuse out of the blood pool into the interstitium which results in a decrease in vascular signal and increased background enhancement (poor contrast-to-noise ratio) and a limited acquisition window. This short intravascular retention limits the application of dynamic imaging to evaluate flow patterns and requires repeated contrast administration to evaluate multiple stations or the effect of provocative maneuvers (15). Adequate bolus timing and patient cooperation are thus essential to obtain adequate MR angiographic images with these agents (15). While non contrast maging techniques are limited in detecting slow flow in smaller veins, contrast enhanced venous imaging with extracellular gadolinium-based agents is technically more challenging than arterial imaging due to unpredictable venous opacification as contrast bolus traverses from the blood pool to interstial space (15). Gadolinium-based blood-pool contrast agents (BPCA) are characterized by prolonged intravascular circulation. Intuitively, MR angiographic applications of these agents include both first-pass and steady-state MRA. Due to the long plasma half-life of blood-pool agents, they are often referred to as intravascular contrast agents. The blood-pool effect of these agents is due to the large size of the macromolecules, which limits diffusion across the endothelial membrane (16), ensuring low or absent leakage into the extracellular compartment. BPCA also allows steady-state imaging from 5 min up to 60 min post injection if needed (17). The Gd-based macromolecules are high relaxivity agents because their large size leads to slow rotational dynamics. The binding of albumin by BPCA further increases the relaxivity compared to conventional agents. These properties allow for a lower dose of BPCA needed for quality image acquisition (Table 1).
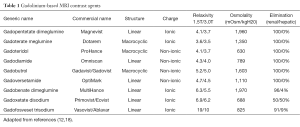
Full table
Contrast agents
Gadofosveset trisodium (Ablavar®, Lantheus Medical Imaging, North Billerica, MA) is the most prominent agent in the low-molecular group of Gadolinium-based blood-pool agents. Gadofosveset is a high-relaxivity agent due to slow molecular rotational speed when bound to albumin. In human plasma, 4–20% of gadofosveset follows the same distribution as extracellular Gd-CA (12). Elimination of gadofosveset is predominantly by renal excretion and to a lesser extent by hepatobiliary excretion (9%) (12). Gadofosveset has been the first blood pool MRI contrast agent to become commercially available and received approval by the U.S. Food and Drug Administration in 2008 for imaging of aortoiliac occlusive disease in adults (≥18 years) with known or suspected peripheral vascular disease. It was also known as Vasovist® (Bayer Schering Pharma, Berlin, Germany), which received its first marketing authorization in the European Union in 2005 (15). The large molecular size of the chelate-albumin complex results in decrease the molecular rotation rate and in significantly shorter T1 relaxation times (19.0 L/mmol/sec at 37 °C, 1.5 T); approximately five times that of extracellular agents at 1.5 T. This results in greater efficacy per unit gadolinium dose so that lower injection volumes and fewer injections are sufficient for diagnostic purposes (7) (Figures 2,3). The standard dose for MRA is 0.03 mmol/kg (0.12 mL/kg body weight) (2,7,9), compared to a dose of 0.1 mmol/kg for all other conventional agents (17).
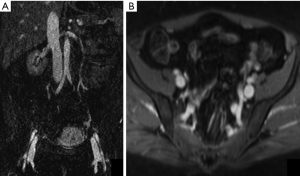
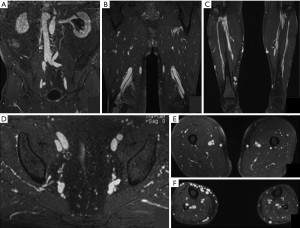
Multiple studies have demonstrated its safety. In phase III trials of Ablavar performing MR angiography of the aortoiliac region in adults patients with known or suspected to have peripheral vascular disease, the most common adverse events were feeling hot (4.4%), nausea (3.6%), headache (3.3%) and burning sensation (2.9%); the incidence of severe and serious adverse events was low (19,20). Of note, QTc prolongation has been reported following gadofosveset administration, and therefore patients should be assessed for a history of underlying conditions that may predispose to arrhythmias from QTc prolongation. The most feared adverse effect of gadolinium-based contrast agents, nephrogenic systemic fibrosis (NSF), has not been reported with gadofosveset (15).
An important application of MRV and therefore BPCA is evaluating the venous system in children, who are sensitive to ionizing radiation. Rigsby et al. identified an adverse event rate of 0.46% following gadofosveset trisodium administration in children and young adults (21), with most reactions being mild without any adverse outcomes. Interestingly, a large number of their scans were performed under anesthesia and anesthetized patients were 5.7 times more likely to experience an adverse event than unanesthetized patients, which could be an alternative explanation for the adverse events observed.
Another agent that has been studied in the past decade is Ferumoxytol, a superparamagnetic particle of iron developed as an intravenous iron supplement for patients with anemia of renal failure (4). It is composed of an iron oxide core surrounded by a polyglucose sorbitol carboxymethyl ether envelope, with a particle size of 30 nm and a molecular weight of 750 kD (4). It has strong T1- and T2*-shortening properties, making it an attractive agent for MRI. In addition, due to its carbohydrate coating, the agent is not filtered by the renal glomeruli, causing it to behave as a blood pool agent. Because of its size and carbohydrate coating, Ferumoxytol has a prolonged intravascular residence time (approximately 14 hours) (1) and a very long intravascular half-life, making it very attractive as an intravascular contrast agent. This extended plateau of increased vascular signal can be used for much longer imaging acquisitions than is possible with extracellular gadolinium-based agents, allowing for improved performance of navigated MRI sequences, venous imaging, and the option for repeat imaging without the need for additional contrast (22) (Figure 4). Ferumoxytol has an excellent safety profile and has been administered extensively to patients with class 1–5 chronic kidney disease (CKD). In fact, it was developed for use in this specific patient population (4) and should be considered as an alternative in vascular imaging when gadolinium-based agents are contraindicated. Its use as a contrast agent in MRI is off-label, as it is only approved by the FDA for the treatment of iron-deficiency anemia. The highest dose for imaging is 4 mg (71.6 micromols) iron per kilogram of body weight (1).
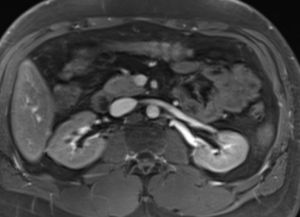
Applications
Deep venous thrombosis
One of the main indications of venous MR imaging is deep vein thrombosis, with several studies demonstrating the feasibility and accuracy of MRV with BPCA (Figures 5,6).
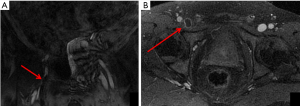

Hansch et al. investigated the use of gadofosveset trisodium-enhanced MR in 43 patients with suspected DVT who underwent compression US. They found that MR detected 10 additional thrombi in the pelvic region and inferior vena cava compared to DUS, 3 additional thrombi in the upper leg, 4 additional thrombi in the popliteal vein, and 17 additional thrombi in the lower leg. Furthermore, pulmonary emboli (PE) were detected in 16 of the 43 patients with DVT that were identically correlated with CT in location, size and shape. There were no cases with non-diagnostic image quality. Therefore, gadofosveset trisodium enhanced MR had increased sensitivity for the detection of DVT compared to US with the advantage of high accuracy detection for concurrent pulmonary embolism (23).
Pfeil et al. also showed the feasibility and quality of imaging of the unaffected venous system of the lower limbs using the VIBE sequence with fat saturation and blood pool contrast agent in a study with 25 individuals without clinical signs for DVT and normal Doppler US of the lower limbs (2). Again, no nondiagnostic images were acquired and excellent inter-rater reliability was noted (κ value for measurement interrater reliability ranged as significant between 0.75 for the external iliac vein and 0.85 for the common iliac vein).
Enden et al. compared two different imaging techniques (Balanced Turbo Field Echo and Contrast-Enhanced T1 Fast Field Echo) using a blood pool agent in 15 healthy volunteers and 6 patients with verified acute proximal DVT on routine ultrasound (3). Both MR techniques produced a complete visualization of the deep venous system in the vast majority of images covering the calf, femoral, and pelvic veins. In general, all three image quality parameters analyzed were best on calf examinations and, despite the poor grading of image quality obtained in pelvic images, both MR techniques maintained complete visualization of the pelvic veins.
In a study of MR venography of iliocaval veins with use of MS-325 (initial name for Ablavar) conducted by Sharafuddin et al., it was demonstrated that MS-325 was reliable in providing consistent diagnostic visualization of the entire inferior vena cava (IVC). There was improved venous conspicuity and subjective contrast enhancement compared to gadodiamide (24). Diagnostically limiting artifacts were present in seven of 29 infrarenal IVC segments on unsubtracted Gd-MRA studies compared to none of the 12 segments on unsubtracted MS-325-MRA studies.
Ferumoxytol has also been studied as a contrast agent in the investigation of deep vein thrombosis. Bashir et al. compared Ferumoxytol with Gadofosveset as contrast agents and demonstrated comparable subjective and objective enhancement of the abdominopelvic and lower extremity venous systems as well as comparable visualization of venous thrombosis (4). Ferumoxytol may therefore be an attractive option for patients with renal function impairment since iron-based agents are not associated with risk of nephrogenic systemic fibrosis, and can be considered in vascular imaging when gadolinium-based agents are contraindicated. In addition, Li et al. evaluated extremity deep venous thrombosis with Ferumoxytol-enhanced MR and found that image quality scores for Ferumoxytol-enhanced MR were uniformly superior to those of non-contrast 2D time-of-flight images (average image quality score for Ferumoxytol was 3.6 vs. 1.4 for precontrast images) (1). Also, MR showed agreement with duplex US in depicting 90% of the thrombi and an increased detection of additional contralateral thrombi.
May-Thurner/Cockett syndrome
May-Thurner syndrome results from the compression of the left common iliac vein (LCIV) between the right common iliac artery (RCIA) and fifth lumbar vertebra, an anatomic relationship that was defined by May and Thurner in 1956. May- Thurner anatomy is thought to be the cause of approximately 2–3% of all lower extremity deep venous thrombosis (25,26), particularly in young to middle aged women. Symptomatic patients may present with left leg edema, pain, varicosities, venous claudication, and ulceration (27). However, it is important to note that the majority of patients with venous compression of the LCIV by the RCIA are asymptomatic. Kibbe et al. demonstrated that up to 24% of patients had greater than 50% compression and 66% had greater than 25% compression (28), and thus May-Thurner variant anatomy alone is not sufficient for pathology.
MRV is an attractive modality for the diagnosis and characterization of May-Thurner Syndrome (MTS) for several reasons. Iliac vein compression and thrombi can be directly visualized, with quantification of flow across the stenosis and accompanying reversal of flow in the internal iliac vein and collateral vessels (29). In addition, the lack of ionizing radiation is a distinct advantage over CT venography. In a case study by Wolpert et al., MRV was able to confirm a diagnosis of MTS in all 9 patients while ruling out the presence of DVT and pelvic masses (27). When utilizing MRV for the evaluation of MTS, blood pool contrast agents are valuable because they both minimize the contrast dose and decrease the chance for mistimed acquisitions.
There is no consensus about specific radiologic signs that are diagnostic of MTS. However, the most useful finding is of compression of the LCIV by the RCIA (Figure 7). Tortuous venous collaterals crossing the pelvis to drain into the contralateral veins and thrombus formation are also suggestive features (30). A visualization of greater than 50% stenosis in the luminal diameter of the vein is considered an adequate indicator of LCIV compression related to MTS (31).
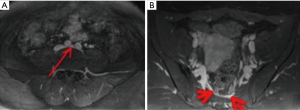
It is important to note that scanning more than once may be necessary to confirm the diagnosis since the degree of venous compression can be variable and asymptomatic. McDermott et al. found that the compressed LCIV on a single MRV study was not stable over time, with the mean compression of the LCIV at 62% in the index study and 39% in the comparison study in the same patients, with the mean change in the degree of compression between the two studies being 23% (32). The authors postulate that the compression in a single study may be insufficient to diagnose MTS.
Nutcracker syndrome (NS)
NS describes the compression of the left renal vein (LRV) between the aorta and superior mesenteric artery (SMA) (“anterior nutcracker”) or, if the LRV has a retroaortic or circumaortic course, between the aorta and an underlying vertebral body (“posterior nutcracker”), predisposing to venous hypertension and the formation of intra and extrarenal hypertensive venous collaterals, gonadal vein reflux, and periureteric varices. The most accepted hypotheses proposed to explain the compression are posterior renal ptosis, abnormal high course of the LRV or an abnormal origin of the SMA from the aorta (30,33).
It is relatively more common in females and usually presents in the 3rd or 4th decade of life. The severity of NS is variable, ranging from asymptomatic to severe pelvic congestion. The most common presenting symptom is micro- or macroscopic hematuria from the rupture of fragile varices into the collecting system (5,30), mild to severe orthostatic proteinuria, left flank pain secondary to the passage of ureteral blood clots and symptoms of pelvic venous congestion (chronic pelvic pain, dyspareunia, dysuria, and dysmenorrhea) (5).
The gold standard for diagnosis of NS is retrograde venography with a renocaval pressure gradient measurement of more than 3 mm Hg (30). However, noninvasive evaluation through CTV and MRV is far more common as they allow for evaluation of the anatomic structures and its hemodynamic consequences. Due to its pharmacokinetic profile and long intravascular stay, blood pool contrast agents have several advantages over extracellular agents in this scenario but none study so far have compared diagnostic accuracy between both class of agents. Many radiologic relationships have been studied in evaluating for possible NS. The beak sign, an abrupt narrowing of the LRV between the aorta and SMA with proximal dilatation of the LRV, can be visualized on CT and MR and has a sensitivity of 91.7% and specificity of 88.9% in the diagnosis of Nutcracker Syndrome (30). Another measure, the ratio of the LRV diameters at the hilar and aortomesenteric regions of more than 4.9, has a sensitivity of 66.7% and specificity of 100% for this condition (30). The aortomesenteric angle (between the SMA and aorta) of less than 41° is considered 100% sensitive and 55.6% specific for nutcracker syndrome (34) since the normal aortomesenteric angle measures approximately 90° (33,35).
It is important to note however that these anatomic findings alone are not sufficient without matching clinical symptoms. For example, approximately 44% of asymptomatic patients were found to have mild narrowing of the LRV without the beak sign (35), and therefore careful correlation of symptoms is necessary before a diagnosis is made.
Pelvic congestion syndrome
This syndrome is a common cause of chronic pelvic pain and it is believed to be caused by pelvic varices. Pelvic varicosities are enlarged tortuous tubular structures in the trajectory of the ovarian veins, around the uterus and the adnexa, as well as in the pelvic floor. The retrograde blood flow and the dysfunctional venous valves within the ovarian veins increase the pressure in the pelvic venous system, which cannot be compensated and generates dilation. The syndrome may also result from obstructing anatomic anomalies such as retroaortic left ovarian vein and Nutcracker syndrome or be secondary (36,37). Risk factors include pelvic surgery, retroverted uterus, hormonal influence (affects mostly premenopausal women) and multiple pregnancies (there may be a significant increase in intravascular volume at each gestation and the venous distention can lead to valve incompetency over time). Most patients present with a noncyclical pain lasting more than 6 months, which can be worsened by movement, posture and activities that increase pelvic pressure (36,37). Interestingly, not all patients presenting with enlarged pelvic veins are symptomatic, and symptomatic patients may only have minimal or even no dilation at all. On imaging, possible causes of compression include tumors, cysts, or arterial aneurysms, and therefore renal and the iliac veins should be assessed for signs of compression.
Several criteria have been proposed for pelvic varicosities. On CT and MRI, the cut-off value for ovarian vein diameter is 8 mm. Early filling of the ovarian veins, internal iliac veins and/or left parauterine veins can be used as a sign of venous reflux (38). In nondiagnostic cases, conventional venography with direct visualization of the dilated ovarian veins can be performed, with the added advantage of simultaneous treatment if necessary.
During conventional angiographic and CT examinations, the Valsalva maneuver and table tilting have been advocated by some to induce sufficient reflux into the ovarian veins to allow visible ovarian vein engorgement. MR contrast-enhanced techniques are superior to both non-enhanced MR and CT. In a study with 10 patients diagnosed with retrograde flow through the ovarian veins, Kim et al. demonstrated that retrograde flow through incompetent ovarian vein valves can be easily visualized in the absence of dilation using time resolved-MRA during arterial phase, with an average time of 15 seconds after the initial aortic enhancement (39). Additional sequences can be obtained during a Valsalva maneuver, increasing the sensitivity for detecting varicose veins, especially in cases with a very slow reflux. No study has compared so far the visualization of ovarian vein reflux with blood pool and extracellular contrast agents; however the steady state imaging with gadofosveset can allow a clear depiction of the ovarian veins at high resolution (17).
Thoracic venous outlet syndrome (TOS)
TOS is a constellation of symptoms caused by the compression of the subclavian vessels and brachial plexus during their passage from the thoracic cavity to the axilla (40). The most common type is neurogenic and an arterial and venous TOS account for less than 10% of cases (41). There is no sex predilection, except for the neurogenic type, which presents a female/male ratio of 3.5/1. Some established causes for compression are the presence of a cervical rib, long C7 transverse process, exostosis, congenital fibromuscular anomalies, repetitive movements and posttraumatic fibrosis of the scalene muscle (40) The symptoms include pain, claudication and coldness (for arterial compression) and upper extremity edema and thrombosis (venous compression). The diagnosis can be suspected with history and physical examination, although imaging is required to identify vascular abnormalities.
Contrast-enhanced MRA with provocative arm positioning has emerged as the primary tool to evaluate patients with TOS. For contrast-enhanced MRA with conventional extracellular contrast agents, a dual injection protocol is required, first during abduction and then at rest for acquiring post contrast images with good arterial and venous opacification in both positions, since arm abduction can exacerbate vascular compression (40,41). The degree of venous compression during hyperabduction is interpreted in post-contrast images and any lesion with more than 50% of subclavian vein compression should be considered significant. However, with a blood pool contrast agent, only a single-injection is necessary to achieve diagnostic image quality with high vessel contrast similar to a double-injection due to the prolonged intravascular stay. The experience of imaging of the thoracic outlet vessels with extracellular contrast agents is still poor but the feasibility for this scenario was confirmed by a study performed by Lim et al. with 31 patients assessed for vascular TOS using either a blood pool or an extracellular contrast agent; diagnostic quality imaging was achieved in all patients and BPS clearly demonstrated arterial and venous pathology, as well as functional changes in vessel caliber during arm position changes (41).
Other possible applications of BPCA in venous imaging include pulmonary embolism, upper extremity venous system, venous malformations and porto-splenic-mesenteric veins despite few studies demonstrating these uses. One study with 20 volunteers comparing gadofosveset trisodium and gadobenate dimeglumine during thoracic MR angiography at 3T by Frydrychowicz et al. did however find similar signal to noise ratio and contrast to noise ratio between both agents, suggesting that gadofosveset can depict thoracic veins and arteries with high accuracy, comparable to extracellular contrast agents (42). Also, the combination of dynamic and steady-state imaging with gadofosveset allows for evaluation of anatomical and flow characteristics of vascular malformations, allowing the differentiation between high-flow and low-flow vascular malformation. First-pass images delineate the arterial anatomy and steady-state images facilitate assessment of the venous anatomy and enhancement pattern of the lesion (15).
Conclusions
Contrast-enhanced MRV has emerged as an important tool for venous imaging over the last decade due to its advantages in anatomic evaluation without ionizing radiation. However, limitations in acquisition timing and administration of gadolinium-based agents in renal impairment are well known. Blood pool contrast agents are a very promising alternative in these situations and can yield quality images when compared to conventional extracellular agents.
Acknowledgements
Irai S. Oliveira would like to acknowledge the support from Hospital Sírio-Libanês, São Paulo, Brazil.
Footnote
Conflicts of Interest: The authors have no conflicts of interest to declare.
References
- Li W, Salanitri J, Tutton S, et al. Lower extremity deep venous thrombosis: evaluation with ferumoxytol-enhanced MR imaging and dual-contrast mechanism--preliminary experience. Radiology 2007;242:873-81. [Crossref] [PubMed]
- Pfeil A, Betge S, Poehlmann G, et al. Magnetic resonance VIBE venography using the blood pool contrast agent gadofosveset trisodium--an interrater reliability study. Eur J Radiol 2012;81:547-52. [Crossref] [PubMed]
- Enden T, Storås TH, Negård A, et al. Visualization of deep veins and detection of deep vein thrombosis (DVT) with balanced turbo field echo (b-TFE) and contrast-enhanced T1 fast field echo (CE-FFE) using a blood pool agent (BPA). J Magn Reson Imaging 2010;31:416-24. [Crossref] [PubMed]
- Bashir MR, Mody R, Neville A, et al. Retrospective assessment of the utility of an iron-based agent for contrast-enhanced magnetic resonance venography in patients with endstage renal diseases. J Magn Reson Imaging 2014;40:113-8. [Crossref] [PubMed]
- Lamba R, Tanner DT, Sekhon S, et al. Multidetector CT of vascular compression syndromes in the abdomen and pelvis. Radiographics 2014;34:93-115. [Crossref] [PubMed]
- Arnoldussen CW, de Graaf R, Wittens CH, et al. Value of magnetic resonance venography and computed tomographic venography in lower extremity chronic venous disease. Phlebology 2013;28 Suppl 1:169-75. [Crossref] [PubMed]
- Lewis M, Yanny S, Malcolm PN. Advantages of blood pool contrast agents in MR angiography: a pictorial review. J Med Imaging Radiat Oncol 2012;56:187-91. [Crossref] [PubMed]
- Huang SY, Kim CY, Miller MJ, et al. Abdominopelvic and lower extremity deep venous thrombosis: evaluation with contrast-enhanced MR venography with a blood-pool agent. AJR Am J Roentgenol 2013;201:208-14. [Crossref] [PubMed]
- Grobner T. Gadolinium--a specific trigger for the development of nephrogenic fibrosing dermopathy and nephrogenic systemic fibrosis? Nephrol Dial Transplant 2006;21:1104-8. [Crossref] [PubMed]
- Christensen KN, Lee CU, Hanley MM, et al. Quantification of gadolinium in fresh skin and serum samples from patients with nephrogenic systemic fibrosis. J Am Acad Dermatol 2011;64:91-6. [Crossref] [PubMed]
- McDonald RJ, McDonald JS, Kallmes DF, et al. Intracranial Gadolinium Deposition after Contrast-enhanced MR Imaging. Radiology 2015;275:772-82. [Crossref] [PubMed]
- Nielsen YW, Thomsen HS. Contrast-enhanced peripheral MRA: technique and contrast agents. Acta Radiol 2012;53:769-77. [Crossref] [PubMed]
- Thomsen HS. Guidelines for contrast media from the European Society of Urogenital Radiology. AJR Am J Roentgenol 2003;181:1463-71. [Crossref] [PubMed]
- Thomsen HS, Morcos SK. Contrast media and the kidney: European Society of Urogenital Radiology (ESUR) guidelines. Br J Radiol 2003;76:513-8. [Crossref] [PubMed]
- Farmakis SG, Khanna G. Extracardiac applications of MR blood pool contrast agent in children. Pediatr Radiol 2014;44:1598-609. [Crossref] [PubMed]
- Vessie EL, Liu DM, Forster B, et al. A practical guide to magnetic resonance vascular imaging: techniques and applications. Ann Vasc Surg 2014;28:1052-61. [Crossref] [PubMed]
- Knuttinen MG, Karow J, Mar W, et al. Blood Pool Contrast-enhanced Magnetic Resonance Angiography with Correlation to Digital Subtraction Angiography: A Pictorial Review. J Clin Imaging Sci 2014;4:63. [PubMed]
- ACR Committee on Drugs and Contrast Media. Appendix A – Contrast Media Specifications - ACR Manual on Contrast Media version 10.2. 2016.
- Goyen M, Edelman M, Perreault P, et al. MR angiography of aortoiliac occlusive disease: a phase III study of the safety and effectiveness of the blood-pool contrast agent MS-325. Radiology 2005;236:825-33. [Crossref] [PubMed]
- Rapp JH, Wolff SD, Quinn SF, et al. Aortoiliac occlusive disease in patients with known or suspected peripheral vascular disease: safety and efficacy of gadofosveset-enhanced MR angiography--multicenter comparative phase III study. Radiology 2005;236:71-8. [Crossref] [PubMed]
- Rigsby CK, Popescu AR, Nelson P, et al. Safety of Blood Pool Contrast Agent Administration in Children and Young Adults. AJR Am J Roentgenol 2015;205:1114-20. [Crossref] [PubMed]
- Hope MD, Hope TA, Zhu C, et al. Vascular Imaging With Ferumoxytol as a Contrast Agent. AJR Am J Roentgenol 2015;205:W366-73. [Crossref] [PubMed]
- Hansch A, Betge S, Poehlmann G, et al. Combined magnetic resonance imaging of deep venous thrombosis and pulmonary arteries after a single injection of a blood pool contrast agent. Eur Radiol 2011;21:318-25. [Crossref] [PubMed]
- Sharafuddin MJ, Stolpen AH, Dang YM, et al. Comparison of MS-325- and gadodiamide-enhanced MR venography of iliocaval veins. J Vasc Interv Radiol 2002;13:1021-7. [Crossref] [PubMed]
- Brinegar KN, Sheth RA, Khademhosseini A, et al. Iliac vein compression syndrome: Clinical, imaging and pathologic findings. World J Radiol 2015;7:375-81. [Crossref] [PubMed]
- O'Sullivan GJ, Semba CP, Bittner CA, et al. Endovascular management of iliac vein compression (May-Thurner) syndrome. J Vasc Interv Radiol 2000;11:823-36. [Crossref] [PubMed]
- Wolpert LM, Rahmani O, Stein B, et al. Magnetic resonance venography in the diagnosis and management of May-Thurner syndrome. Vasc Endovascular Surg 2002;36:51-7. [Crossref] [PubMed]
- Kibbe MR, Ujiki M, Goodwin AL, et al. Iliac vein compression in an asymptomatic patient population. J Vasc Surg 2004;39:937-43. [Crossref] [PubMed]
- Lugo-Fagundo C, Nance JW, Johnson PT, et al. May-Thurner syndrome: MDCT findings and clinical correlates. Abdom Radiol (NY) 2016;41:2026-30. [Crossref] [PubMed]
- Fong JK, Poh AC, Tan AG, et al. Imaging findings and clinical features of abdominal vascular compression syndromes. AJR Am J Roentgenol 2014;203:29-36. [Crossref] [PubMed]
- Liu Z, Gao N, Shen L, et al. Endovascular treatment for symptomatic iliac vein compression syndrome: a prospective consecutive series of 48 patients. Ann Vasc Surg 2014;28:695-704. [Crossref] [PubMed]
- McDermott S, Oliveira G, Ergül E, et al. May-Thurner syndrome: can it be diagnosed by a single MR venography study? Diagn Interv Radiol 2013;19:44-8. [PubMed]
- Fu WJ, Hong BF, Gao JP, et al. Nutcracker phenomenon: a new diagnostic method of multislice computed tomography angiography. Int J Urol 2006;13:870-3. [Crossref] [PubMed]
- Kim KW, Cho JY, Kim SH, et al. Diagnostic value of computed tomographic findings of nutcracker syndrome: correlation with renal venography and renocaval pressure gradients. Eur J Radiol 2011;80:648-54. [Crossref] [PubMed]
- Ahmed K, Sampath R, Khan MS. Current trends in the diagnosis and management of renal nutcracker syndrome: a review. Eur J Vasc Endovasc Surg 2006;31:410-6. [Crossref] [PubMed]
- Ignacio EA, Dua R, Sarin S, et al. Pelvic congestion syndrome: diagnosis and treatment. Semin Intervent Radiol 2008;25:361-8. [Crossref] [PubMed]
- Kuligowska E, Deeds L 3rd, Lu K 3rd. Pelvic pain: overlooked and underdiagnosed gynecologic conditions. Radiographics 2005;25:3-20. [Crossref] [PubMed]
- Arnoldussen CW, de Wolf MA, Wittens CH. Diagnostic imaging of pelvic congestive syndrome. Phlebology 2015;30:67-72. [Crossref] [PubMed]
- Kim CY, Miller MJ Jr, Merkle EM. Time-resolved MR angiography as a useful sequence for assessment of ovarian vein reflux. AJR Am J Roentgenol 2009;193:W458-63. [Crossref] [PubMed]
- Aghayev A, Rybicki FJ. State-of-the-art Magnetic Resonance Imaging in Vascular Thoracic Outlet Syndrome. Magn Reson Imaging Clin N Am 2015;23:309-20. [Crossref] [PubMed]
- Lim RP, Bruno M, Rosenkrantz AB, et al. Comparison of blood pool and extracellular gadolinium chelate for functional MR evaluation of vascular thoracic outlet syndrome. Eur J Radiol 2014;83:1209-15. [Crossref] [PubMed]
- Frydrychowicz A, Russe MF, Bock J, et al. Comparison of gadofosveset trisodium and gadobenate dimeglumine during time-resolved thoracic MR angiography at 3T. Acad Radiol 2010;17:1394-400. [Crossref] [PubMed]