Predictive models of in-hospital deterioration of Society of Cardiovascular Angiography and Intervention shock stage in patients with acute myocardial infarction initially presenting with stable hemodynamic condition
Highlight box
Key findings
• In patients with acute myocardial infarction (AMI) initially presenting with Society of Cardiovascular Angiography and Intervention (SCAI) shock stage A or B, 17.3% of them exhibited the progression of their SCAI shock stage after hospitalization. This worsening SCAI shock stage was associated with higher in-hospital mortality. Our developed risk score [systolic blood pressure (BP), left ventricular ejection fraction (LVEF), and initial Thrombolysis in Myocardial Infarction (TIMI) flow grade] predicted worsening SCAI shock stage.
What is known and what is new?
• Patients with AMI who are initially hemodynamically stable could develop cardiogenic shock (CS) during hospitalization. This worsening course of AMI increases in-hospital mortality.
• The current study elucidated that 17.3% of AMI patients with initially hemodynamically stable condition presented the progression of their SCAI shock stage after hospitalization. Three risk scores (systolic BP, LVEF, and initial TIMI flow grade) enabled to predict worsening SCAI shock stage.
What is the implication, and what should change now?
• Our findings suggest the importance to promptly identify patients with AMI at high risk for worsening CS. In hemodynamically stable patients with AMI presenting high deterioration of CS risk score, appropriate adoption of anti-CS management prior to CS development should be considered.
Introduction
Cardiogenic shock (CS) is a dynamic and hemodynamically heterogenous condition in patients with acute myocardial infarction (AMI) (1-4). A recent observational study of patients with AMI and CS reported that only 9% of them presented with CS upon arrival (5). Of note, many patients were initially hemodynamically stable, and developed CS during hospitalization. Given that this worsening course of AMI increases in-hospital mortality (5-7), prompt recognition of patients with AMI at a higher risk of CS might enable appropriate management to stabilize their hemodynamic status and thereby improve their outcomes. However, to date, there is no applicable model to predict this deteriorated clinical course in patients presenting hemodynamically stable AMI.
The Society of Cardiovascular Angiography and Intervention (SCAI) has recently proposed a new CS classification scheme with five stages (A–E) based on clinical characteristics (8,9). Serial evaluation of SCAI CS stage might help identify deterioration of CS (D-CS) in patients with AMI (10-12). Therefore, we characterized patients with AMI who had worsening SCAI CS stage and developed a risk score for predicting their clinical course. We present this article in accordance with the STROBE reporting checklist (available at https://cdt.amegroups.com/article/view/10.21037/cdt-24-226/rc).
Methods
The current study retrospectively analyzed 430 consecutive patients with de novo AMI that underwent percutaneous coronary intervention (PCI) at our institution (2019.07.01–2021.09.30) as a derivation cohort. AMI was diagnosed according to the published definition (13). The following patients were excluded: patients presenting with SCAI shock stage C, D, or E on arrival (n=55), patients requiring the prophylactic use of intra-aortic balloon pump (IABP) due to a complex PCI procedure (n=14), and patients with major PCI complications (n=14). The remaining 347 patients with AMI presenting with SCAI shock stage A or B on arrival were included in the derivation cohort (Figure 1).
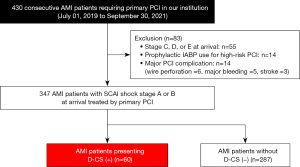
The validation cohort included 188 consecutive patients with de novo AMI who underwent PCI at our institution (2021.10.01–2022.09.30). Of these, 25 patients were excluded due to SCAI shock stage C to E on arrival (n=14), prophylactic use of IABP for a complex PCI procedure (n=5), or major PCI complication (n=6). Consequently, 163 patients with AMI with SCAI shock stage A or B were included in the validation cohort. The research protocol was approved by the ethics committee of National Cerebral & Cardiovascular Center (No. M24-055-9). Informed consent was not required due to the observational analysis of hospitalized patients. The study was conducted in accordance with the Declaration of Helsinki (as revised in 2013).
Two cardiologists evaluated each patient’s condition in the emergency room (ER). The initial blood pressure (BP) levels in the ER were measured. Left ventricular ejection fraction (LVEF) was measured in the ER based on two-dimensional echocardiography images. All patients were then immediately transferred to the catheterization laboratory for emergency coronary angiography. Throughout acquisition of the initial angiographic images of the culprit vessel, its Thrombolysis in Myocardial Infarction (TIMI) flow grade was evaluated by the PCI operator. Procedural decisions were made according to the discretion of each PCI operator. After PCI was completed, patients were transferred to the cardiac intensive care unit (CICU). Dual antiplatelet with any additional anticoagulation therapy, if needed, was commenced according to Japanese Circulation Society guidelines (14).
SCAI CS stage was evaluated in the ER according to the SCAI shock stage classification (8,9). When patients were hospitalized in the CICU after PCI, CS stage was immediately reclassified. SCAI CS stages were defined based on bedside findings, biochemical markers, and hemodynamic data as proposed in the SCAI shock stage classification expert consensus update (9). D-CS was defined as progression from SCAI shock stage A or B in the ER to SCAI shock stage C to E after transfer to the CICU. Evaluation of CS stage was conducted by two independent physicians (T.M. and Y.K.) who were blinded to the participants’ in-hospital outcomes. When there was discordance, consensus was reached with a third independent physician (M.F.). The primary outcome was in-hospital all-cause mortality. In-hospital all-cause mortality was defined as the mortality rate for a population across any causes during hospitalization. Outcomes were ascertained through medical record review by two physicians (T.M. and Y.K.). All of physicians (T.M., Y.K., and M.F.) have been served as an interventional cardiologist for more than 10 years.
Statistical analysis
Categorical variables were summarized as numbers and percentages and compared with Fisher’s exact test or the Chi-square test as appropriate. Continuous variables were summarized as mean ± standard deviation and compared using the t-test if data were normally distributed. When continuous variables were not normally distributed, they were summarized as median [interquartile range (IQR)] and compared with the Mann-Whitney U test. The Kaplan-Meier method was used to estimate 30-day survival curves. The log-rank test was used to estimate differences between patients with or without D-CS. Unadjusted hazard ratios (HRs) for in-hospital mortality were calculated with univariate Cox proportional hazards models. The univariate Cox proportional hazards model analysis included any variables which have been considered to associate with the risk of CS. Adjusted HRs were calculated with multivariate Cox proportional hazards models that included variables with P<0.10 in univariate models.
Univariate logistic regression was used to identify predictors of D-CS. The univariate logistic regression analysis included any variables which have been considered to associate with the risk of CS. Then, multivariate logistic regression that included variables with P<0.10 in univariate analyses and the SCAI shock stage in the ER was performed. The D-CS risk score was developed with three independent variables: systolic BP, LVEF, and initial TIMI flow grade. Logistic regression was conducted to calculate odds ratios (ORs) for systolic BP of 100–139 and <100 mmHg, with systolic BP ≥140 mmHg as the reference. Similarly, LVEF ≥50% was set as the reference and OR for LVEF of 40–49% and <40% were calculated. The OR for initial TIMI flow grade 0 or 1 was calculated using initial TIMI flow grade 2 or 3 as the reference. Risk scores were assigned as follows: 1 point for variables with OR <5 and 2 points for variables with OR ≥5. Points were summed and used to calculate D-CS risk score, which can range from 0 to 5 points. The prevalence of D-CS and in-hospital mortality were compared in participants stratified by D-CS risk score with the Cochran-Armitage test. Receiver operating characteristic analysis was performed to assess the discriminative power of the D-CS risk score for predicting D-CS risk and in-hospital mortality. The Youden index was used to determine the appropriate cut-off value of the D-CS risk score for estimating D-CS risk. Two-tailed P values <0.05 were considered statistically significant. All analyses were performed using R version 4.0.2 (R Foundation for Statistical Computing, Vienna, Austria).
Results
In the derivation cohort, 88.2% (306/347) of patients had SCAI shock stage A in the ER, and the remaining 11.8% (41/347) presented with SCAI shock stage B. Following PCI, 16.4% (57/347) and 0.9% (3/347) exhibited SCAI shock stage C and E, respectively. As a result, D-CS was observed in 17.3% (60/347) of the derivation cohort (Figure 2). The remaining 287 patients who developed CS stage A or B in the CICU did not have any subsequent deterioration of SCAI CS stage.
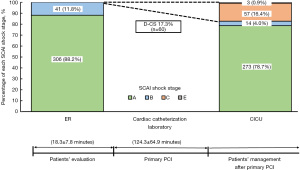
Table 1 summarizes the clinical characteristics of patients with D-CS in the derivation cohort. Patients with D-CS were more likely to be male (90.0% vs. 75.6%, P=0.01), and to present with ST-segment elevation myocardial infarction (86.7% vs. 66.9%, P=0.002) and Killip class ≥ II (41.7% vs. 9.4%, P<0.001). Moreover, they had severe myocardial damage, reflected by higher levels of peak creatinine kinase [2,642 (IQR, 1,331, 5,315) vs. 990 (IQR, 269, 2,323) IU/L, P<0.001], creatine kinase-MB [232 (IQR, 111, 441) vs. 84 (IQR, 21, 221) IU/L, P<0.001] and lower LVEF [45% (IQR, 35%, 50%) vs. 55% (IQR, 45%, 60%), P<0.001]. There was one case who presented out-of-hospital cardiac arrest with its return of spontaneous circulation before arrival (1.7% vs. 0.0%, P=0.17). While patients with D-CS had lower systolic BP than without D-CS CS (130.2±32.7 vs. 148.1±26.2 mmHg, P<0.001), diastolic BP (87.0±22.1 vs. 89.5±18.7 mmHg, P=0.36) and heart rate (80.3±19.7 vs. 77.4±16.8 beats/min, P=0.25) did not differ between the two groups. Regarding biochemical measures, significant differences were observed in measures of renal function [estimated glomerular filtration rate: 56.6 (IQR, 38.8, 70.0) vs. 62.1 (IQR, 50.5, 73.1) mL/min/m2, P=0.02] and liver functions [alanine aminotransferase: 26.0 (IQR, 18.0, 48.0) vs. 22.0 (IQR, 15.0, 35.0) IU/mL, P=0.01], as well as metabolic parameters [glucose: 163 (IQR, 131, 198) vs. 131 (IQR, 113, 164) mg/dL, P<0.001; pH: 7.35 (IQR, 7.32, 7.42) vs. 7.41 (IQR, 7.38, 7.55), P=0.005, HCO3−: 22.9 (IQR, 19.6, 24.1) vs. 24.3 (IQR, 22.4, 26.5) mEq/L, P=0.001] (Table 1).
Table 1
Variables | D-CS (+) (n=60) | D-CS (−) (n=287) | P value |
---|---|---|---|
Age (years) | 66.0±13.7 | 69.7±13.5 | 0.05 |
Male | 54 (90.0) | 217 (75.6) | 0.01 |
Hypertension | 38 (63.3) | 197 (68.6) | 0.44 |
Dyslipidemia | 42 (70.0) | 211 (73.5) | 0.62 |
Diabetes mellitus | 17 (28.3) | 76 (26.5) | 0.87 |
CKD | 18 (30.0) | 59 (20.6) | 0.11 |
History of MI | 7 (11.7) | 27 (9.4) | 0.63 |
History of CABG | 5 (8.3) | 8 (2.8) | 0.05 |
History of stroke | 8 (13.3) | 31 (10.8) | 0.50 |
Severity of AMI | |||
STEMI | 52 (86.7) | 192 (66.9) | 0.002 |
NSTEMI | 8 (13.3) | 95 (33.1) | 0.002 |
OHCA | 1 (1.7) | 0 (0.0) | 0.17 |
LVEF (%) | 45 [35, 50] | 55 [45, 60] | <0.001 |
Killip class ≥ II | 25 (41.7) | 27 (9.4) | <0.001 |
Peak CK (IU/L) | 2,642 [1,331, 5,315] | 990 [269, 2,323] | <0.001 |
Peak CK-MB (IU/L) | 232 [111, 441] | 84 [21, 221] | <0.001 |
Troponin T levels in the ER (ng/mL) | 0.070 [0.019, 0.392] | 0.108 [0.030, 0.523] | 0.15 |
Hemodynamic measures in the ER | |||
Systolic BP (mmHg) | 130.2±32.7 | 148.1±26.2 | <0.001 |
Diastolic BP (mmHg) | 87.0±22.1 | 89.5±18.7 | 0.36 |
Heart rate (beats/min) | 80.3±19.7 | 77.4±16.8 | 0.25 |
Biochemical measures reflecting end-organ hypoperfusion | |||
Hemoglobin (g/dL) | 14.6 [12.3, 15.7] | 14.1 [12.7, 15.2] | 0.30 |
Creatinine (mg/dL) | 1.03 [0.83, 1.46] | 0.90 [0.75, 1.07] | <0.001 |
eGFR (mL/min/m2) | 56.6 [38.8, 70.0] | 62.1 [50.5, 73.1] | 0.02 |
BUN (mg/dL) | 17.0 [13.0, 26.0] | 16.0 [13.0, 20.0] | 0.12 |
ALT (IU/mL) | 26.0 [18.0, 48.0] | 22.0 [15.0, 35.0] | 0.01 |
Glucose (mg/dL) | 163 [131, 198] | 131 [113, 164] | <0.001 |
BNP (pg/mL) | 38.7 [14.7, 213.3] | 56.8 [20.8, 185.9] | 0.34 |
Arterial lactate (mmol/L) | 1.8 [1.3, 1.9] | 1.2 [1.0, 1.5] | 0.12 |
pH | 7.35 [7.32, 7.42] | 7.41 [7.38, 7.55] | 0.005 |
HCO3− (mEq/L) | 22.9 [19.6, 24.1] | 24.3 [22.4, 26.5] | 0.001 |
Data are presented as mean ± standard deviation, n (%), or median [interquartile range]. D-CS, deterioration of cardiogenic shock; CKD, chronic kidney disease; MI, myocardial infarction; CABG, coronary artery bypass graft; AMI, acute myocardial infarction; STEMI, ST-segment elevation myocardial infarction; NSTEMI, non-ST-segment elevation myocardial infarction; OHCA, out-of-hospital cardiac arrest; LVEF, left ventricular ejection fraction; CK, creatinine kinase; ER, emergency room; BP, blood pressure; eGFR, estimated glomerular filtration rate; BUN, blood urea nitrogen; ALT, alanine aminotransferase; BNP, brain natriuretic peptide; HCO3−, sodium bicarbonate.
Table 2 presents PCI features of therapeutic managements in the derivation cohort. Patients with D-CS had higher frequencies of left main trunk stenosis (10.0% vs. 1.7%, P<0.001), chronic total occlusion (18.3% vs. 8.7%, P=0.03), and initial TIMI flow grade 0 or 1 (70.0% vs. 47.7%, P=0.002). Patients with D-CS were less likely to undergo stent implantation (83.3% vs. 93.4%, P=0.01). Four patients did not achieve final TIMI flow grade 3 after PCI. This suboptimal final TIMI flow grade was driven by right coronary artery ectasia with large amounts of thrombus (n=2) and no-reflow phenomenon after stent implantation attributable to distal embolization of lipidic plaque materials (n=2). There was a trend toward a lower frequency of final TIMI flow grade 3 in patients with D-CS (93.3% vs. 98.3%, P=0.05). After these procedures, patients with D-CS had a significantly shorter onset-to-reperfusion time [200 (IQR, 138, 284) vs. 255 (IQR, 115, 506) minutes, P=0.01] (Table 2). Patients with D-CS were more likely to receive catecholamines, mechanical circulatory support (MCS), mechanical ventilation, and continuous renal-replacement therapy (Table 2). Acute kidney injury more frequently occurred in patients with D-CS (8.3% vs. 0.3%, P<0.001) (Table 2).
Table 2
Variables | D-CS (+) (n=60) | D-CS (−) (n=287) | P value |
---|---|---|---|
PCI procedural characteristics | |||
LMT | 6 (10.0) | 5 (1.7) | <0.001 |
LAD | 29 (48.3) | 126 (43.9) | 0.53 |
RCA | 17 (28.3) | 106 (36.9) | 0.24 |
LCx | 2 (3.3) | 28 (9.8) | 0.10 |
ACC/AHA lesion classification | |||
A | 0 (0.0) | 5 (1.7) | 0.30 |
B1 | 2 (3.3) | 21 (7.3) | 0.26 |
B2 | 16 (26.7) | 140 (48.8) | 0.002 |
C | 42 (70.0) | 121 (42.2) | <0.001 |
Chronic total occlusion | 11 (18.3) | 25 (8.7) | 0.03 |
Initial TIMI flow grade 0/1 | 42 (70.0) | 137 (47.7) | 0.002 |
Multivessel disease | 42 (70.0) | 180 (62.7) | 0.30 |
Stent implantation | 50 (83.3) | 268 (93.4) | 0.01 |
Stent diameter (mm) | 3.1±0.5 | 3.0±0.5 | 0.22 |
Stent length (mm) | 27.1±8.5 | 25.2±9.1 | 0.14 |
Final TIMI flow grade 3 | 56 (93.3) | 282 (98.3) | 0.05 |
Onset-to-reperfusion time (min) | 200 [138, 284] | 255 [155, 506] | 0.01 |
Medication use at admission | |||
Antiplatelet | 13 (21.7) | 64 (22.3) | >0.99 |
ACE-I/ARB | 18 (30.0) | 94 (32.8) | 0.76 |
β-blocker | 11 (18.3) | 48 (16.7) | 0.70 |
Statin | 15 (25.0) | 82 (28.6) | 0.75 |
Other therapies | |||
In the ER | |||
Norepinephrine | 2 (3.3) | 0 (0.0) | 0.03 |
Dobutamine | 2 (3.3) | 0 (0.0) | 0.03 |
Dopamine | 1 (1.7) | 0 (0.0) | 0.17 |
Mechanical ventilation | 6 (10.0) | 0 (0.0) | <0.001 |
Cardiac catheterization laboratory | |||
Norepinephrine | 5 (8.3) | 0 (0.0) | <0.001 |
Dobutamine | 3 (5.0) | 0 (0.0) | 0.005 |
Dopamine | 0 (0.0) | 0 (0.0) | >0.99 |
Mechanical ventilation | 3 (5.0) | 1 (0.3) | 0.01 |
IABP | 22 (36.7) | 0 (0.0) | <0.001 |
Impella | 2 (3.3) | 0 (0.0) | 0.03 |
ECMO | 6 (10.0) | 0 (0.0) | <0.001 |
In the CICU | |||
Norepinephrine | 4 (6.6) | 0 (0.0) | <0.001 |
Dobutamine | 10 (16.7) | 0 (0.0) | <0.001 |
Dopamine | 1 (1.7) | 0 (0.0) | 0.17 |
Mechanical ventilation | 2 (3.3) | 0 (0.0) | 0.03 |
CRRT | 7 (11.7) | 2 (0.7) | <0.001 |
Acute kidney injury† | 5 (8.3) | 1 (0.3) | <0.001 |
Data are presented as mean ± standard deviation, n (%), or median [interquartile range]. †, acute kidney injury was defined as an increase of creatinine level ≥0.3 mg/dL. D-CS, deterioration of cardiogenic shock; PCI, percutaneous coronary intervention; LMT, left main trunk; LAD, left anterior descending artery; RCA, right coronary artery; LCx, left circumflex artery; ACC, American College of Cardiology; AHA, American Heart Association; TIMI, Thrombolysis in Myocardial Infarction; ACE-I, angiotensin converting enzyme inhibitor; ARB, angiotensin receptor blocker; ER, emergency room; IABP, intra-aortic balloon pump; ECMO, extracorporeal membrane oxygenation; CRRT, continuous renal-replacement therapy.
During hospitalization (13.9±9.4 days), D-CS was associated with a greater likelihood of experiencing all-cause death [HR, 17.27; 95% confidence interval (CI): 2.07–144.40; P=0.009] (Figure S1, Table S1). A multivariate Cox proportional hazards model demonstrated that D-CS predicted in-hospital mortality (adjusted HR, 12.95; 95% CI; 1.46–114.97; P=0.02) (Table S1). Further multivariate logistic regression analysis revealed that LVEF (adjusted OR, 0.95; 95% CI, 0.92–0.99; P=0.01), systolic BP upon arrival (adjusted OR, 0.98; 95% CI, 0.96–0.99; P=0.009), and initial TIMI flow grade (adjusted OR, 2.61; 95% CI, 1.19–5.72; P=0.01) independently predicted D-CS (Table 3).
Table 3
Variables | Univariate analysis | Multivariate analysis | |||
---|---|---|---|---|---|
OR (95% CI) | P value | OR (95% CI) | P value | ||
Age | 0.98 (0.96–1.00) | 0.05 | 0.97 (0.94–1.00) | 0.05 | |
Male | 2.90 (1.20–7.04) | 0.01 | 2.51 (0.90–6.99) | 0.07 | |
eGFR | 0.99 (0.97–1.00) | 0.03 | 0.98 (0.96–1.00) | 0.15 | |
History of MI | 1.27 (0.53–3.07) | 0.59 | – | – | |
History of CABG | 3.17 (1.00–10.10) | 0.05 | 1.87 (0.22–15.99) | 0.56 | |
History of stroke | 1.30 (0.57–3.00) | 0.53 | – | – | |
Glucose | 1.01 (1.00–1.01) | <0.001 | 1.00 (1.00–1.01) | 0.05 | |
ALT | 1.01 (1.00–1.01) | 0.01 | 1.00 (0.99–1.01) | 0.17 | |
LMT | 6.26 (1.84–21.26) | <0.001 | 5.24 (0.44–61.31) | 0.18 | |
LAD | 1.19 (0.68–2.08) | 0.53 | 1.07 (0.19–5.95) | 0.93 | |
LCx | 0.31 (0.07–1.37) | 0.12 | 0.63 (0.07–5.57) | 0.68 | |
RCA | 0.70 (0.38–1.27) | 0.24 | 0.42 (0.07–2.40) | 0.33 | |
STEMI | 3.22 (1.47–7.04) | 0.003 | 2.75 (0.92–8.18) | 0.06 | |
Chronic total occlusion | 2.35 (1.09–5.09) | 0.03 | 1.39 (0.40–4.80) | 0.59 | |
SCAI shock stage B in the ER | 2.56 (1.24–5.30) | 0.01 | 1.07 (0.40–2.83) | 0.88 | |
LVEF | 0.94 (0.91–0.96) | <0.001 | 0.95 (0.92–0.99) | 0.01 | |
Systolic BP | 0.98 (0.97–0.99) | <0.001 | 0.98 (0.96–0.99) | 0.009 | |
Initial TIMI flow grade 0/1 | 2.55 (1.40–4.65) | 0.002 | 2.61 (1.19–5.72) | 0.01 |
D-CS, deterioration of cardiogenic shock; OR, odds ratio; CI, confidence interval; eGFR, estimated glomerular filtration rate; MI, myocardial infarction; CABG, coronary artery bypass graft; ALT, alanine aminotransferase; LMT, left main trunk; LAD, left anterior descending artery; LCx, left circumflex artery; RCA, right coronary artery; STEMI, ST-segment elevation myocardial infarction; SCAI, Society for Cardiovascular Angiography and Intervention; ER, emergency room; LVEF, left ventricular ejection fraction; BP, blood pressure; TIMI, Thrombolysis in Myocardial Infarction.
The D-CS risk score was developed based on ORs from the logistic regression model used to determine weighting (range, 0–5 points). Two points each were assigned for LVEF <40% and systolic BP <100 mmHg. One point was assigned for LVEF of 40–49%, systolic BP of 100–139 mmHg, and initial TIMI grade flow 0 or 1 (Table 4). Figures 3,4 show that higher D-CS risk score is associated with proportionally elevated risks of D-CS (P value for trend <0.001) and higher in-hospital mortality (P value for trend <0.001). The area under the curve (AUC) for D-CS risk score in predicting D-CS and in-hospital mortality was 0.744 (95% CI: 0.677–0.812) and 0.884 (95% CI: 0.766–1.000), respectively (Figures 3,4).
Table 4
Risk factors | OR (95% CI) | P value | Points |
---|---|---|---|
Systolic BP (mmHg) | |||
≥140 | 1 (reference) | – | 0 |
100–139 | 1.62 (0.83–3.16) | 0.16 | 1 |
<100 | 7.10 (2.48–20.30) | <0.001 | 2 |
LVEF (%) | |||
≥50 | 1 (reference) | – | 0 |
40–49 | 1.94 (0.93–4.05) | 0.07 | 1 |
<40 | 8.10 (3.51–18.70) | <0.001 | 2 |
Initial TIMI flow grade | |||
2/3 | 1 (reference) | – | 0 |
0/1 | 2.94 (1.47–5.91) | 0.002 | 1 |
Total | – | – | 5 |
D-CS, deterioration of cardiogenic shock; OR, odds ratio; CI, confidence interval; BP, blood pressure; LVEF, left ventricular ejection fraction; TIMI, Thrombolysis in Myocardial Infarction.
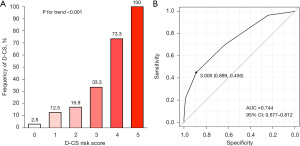
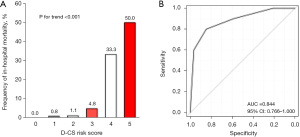
Further analyses were conducted to compare evaluate clinical characteristics and D-CS risk between SCAI stage A and B. Patients with SCAI stage B presented with higher heart rate (99.3±24.3 vs. 75.1±13.9 bpm, P<0.001), increase levels of arterial lactate [1.8 (IQR, 1.6, 1.9) vs. 1.3 (IQR, 1.0, 1.4) mmol/L, P=0.04] and lower LVEF [45% (IQR, 35%, 55%) vs. 55% (IQR, 45%, 60%), P=0.001] as well as a greater frequency of catecholamines, IABP, and mechanical ventilation use (Table S2). Furthermore, the occurrence of D-CS was significantly higher in patients with SCAI stage B (31.7% vs. 15.4%, P=0.01). Multivariate logistic regression analysis emerged D-CS risk score ≥3 (OR, 4.37; 95% CI: 2.11–9.02; P<0.001) is an independent predictor of D-CS, but not SCAI stage B (OR, 1.38; 95% CI: 0.56–3.39; P=0.48) (Table S3). Figure 4 illustrates the frequency of D-CS in patients with SCAI stage A and B, respectively. The occurrence of D-CS increased in association with D-CS risk score in both groups (Figure 5A,5B). Of note, D-CS risk score ≥3 had higher ability to predict the occurrence of D-CS compared to SCAI shock stage B (AUC, 0.669 vs. 0.560, P=0.004) (Figure 5C).
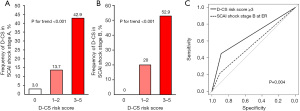
Clinical characteristics were similar in the derivation and validation cohorts (Table S4). In the validation cohort, 133 (81.6%) and 30 (18.4%) patients presented stage A and B, respectively (Table S4). In addition, the incidence of D-CS was 17.2% (28/163) (Table S4). The remaining 163 patients who developed CS stage A or B in the CICU did not have any subsequent deterioration of SCAI CS stage. A lower in-hospital survival rate was observed in patients with D-CS (88.7% vs. 100.0%, log-rank P=0.001) (Figure S2). The D-CS risk score was associated with greater frequency of D-CS during hospitalization and higher in-hospital mortality (Figure S3). In addition, this score satisfactorily discriminated the occurrence of D-CS (AUC, 0.749, 95% CI: 0.651–0.848) and in-hospital mortality (AUC, 0.961, 95% CI: 0.914–1.000), respectively (Figure S4).
Discussion
In the current study, 17.3% of patients with AMI initially presenting with SCAI shock stage A or B progressed to higher SCAI shock stages after hospitalization. Worsening SCAI shock stage was associated with higher in-hospital mortality. Moreover, our newly developed risk score predicted worsening SCAI shock stage (Figure S5). Our findings indicated that the progressive nature of shock stage in the setting of AMI was predictable according to D-CS risk score, which included systolic BP, LVEF, and initial TIMI flow grade.
Pathophysiologically, reduced myocardial contractility and cardiac output could result in systemic vasoconstriction and activation of inflammation in patients with AMI (1,15). These changes attributable to AMI progress to the stage of CS (1). In the current study, we observed that 17.3% of patients with AMI in hemodynamically stable condition did not present with obvious deteriorating characteristics in the ER, but later developed higher CS stages during PCI or in the CICU. In addition, patients with D-CS were more likely to have damage in other organs and metabolic disorders, reflected by a greater frequency of acute kidney injury and lower levels of pH and bicarbonate. These observations suggest that patients with D-CS might be in the “initial stage” of progressive CS status after AMI. A similar clinical finding occurred in the SHOCK trial registry, which enrolled patients with AMI complicated by CS (5). While 9% presented with CS upon arrival, 46.6% developed CS during hospitalization, especially within 6 hours from CS onset. Given the lower survival rate of AMI with in-hospital development of CS, it is required to appropriately stratify patients by ongoing risk of developing CS.
The ORBI and RICO Working Groups have developed the ORBI risk score, which includes 11 clinical variables in patients with ST-elevation myocardial infarction receiving PCI (16). In another study, the IABP-shock II risk score contained seven variables, with six values evaluated in the ER and one variable evaluated after PCI (17). While several approaches have been shown to predict higher in-hospital mortality, limitations of these risk scores include the need to collect data on many variables, including after PCI (18,19). The D-CS risk score includes three clinical variables, two of which (systolic BP and LVEF) are evaluable in the ER. The other variable (initial TIMI flow grade) is obtained during coronary angiography in the catheterization laboratory. These features of our D-CS risk score can help promptly identify patients with AMI at high risk for worsening CS.
We observed an association of D-CS risk score with deteriorating SCAI stage and in-hospital mortality in patients with SCAI shock stage A and B, respectively. Of note, D-CS risk score was better for predicting the occurrence of D-CS compared to SCAI shock stage B. These observations indicate the clinical applicability of D-CS risk score for stratifying a future risk of deteriorating shock stages in both SCAI shock stage A and B.
Favorable clinical outcomes in patients without D-CS suggest the importance of appropriate management for preventing this deteriorating shock status. A stronger approach that includes MCS is needed in patients presenting with rapidly worsening CS stage (2). However, a clinical approach for identifying patients at high risk of worsening CS for MCS at the right time has not been established yet (20). Given that our D-CS risk score predicts in-hospital D-CS stage prior to PCI, appropriate adoption of anti-CS management prior to CS development might be achievable with our scoring approach in hemodynamically stable patients with AMI. Recent studies have reported that the use of Impella prior to reperfusion therapy reduces myocardial infarct size (21,22). Future studies are warranted to elucidate whether the use of mechanical support according to our D-CS risk score could mitigate the risk of deteriorating SCAI CS stages and thereby improve in-hospital outcomes.
Several caveats should be considered. First, this was an observational, single-center, and retrospective study that included a relatively small number of patients with AMI and CS. Therefore, our findings could be considered hypothesis generating. Second, the number of patients in the validation cohort was small. Future studies will be needed to clarify the association of our D-CS risk score with the deterioration of SCAI CS stages in a larger study with adequate statistical power. Third, the use and selection of inotropes and MCS was not randomized. Fourth, due to lack of available invasive hemodynamic data, evaluation of CS stage in the current analysis did not include Swan-Ganz catheter-derived measures. Further studies are required to investigate whether invasive hemodynamic monitoring could improve clinical outcomes of patients with D-CS. Fifth, the current analysis included patients with AMI only. Whether our findings can be applied to those with other cardiovascular diseases remains uncertain. Sixth, the averaged hospital stay was 13.9±9.4 days and over 2 hours were required to complete PCI, which is different from the experience in foreign countries. These features of our study patients might affect the generalizability of our findings.
Conclusions
Worsening SCAI CS stage after hospitalization was observed in 17.3% of patients with AMI who initially presented with SCAI shock stage A or B. This D-CS status was associated with higher in-hospital mortality. Our newly developed risk score integrating three clinical features in the ER and catheterization laboratory satisfactorily predicted D-CS. These findings underscore the need to promptly identify patients with AMI at high risk for worsening CS. Our D-SC risk score might help intensify anti-shock therapies appropriately and improve CS outcomes.
Acknowledgments
We thank Miss Yuko Yoshioka, Miss Yoko Imanaka, and Miss Emi Kanai for their excellent assistance.
Funding: This project was supported by
Footnote
Reporting Checklist: The authors have completed the STROBE reporting checklist. Available at https://cdt.amegroups.com/article/view/10.21037/cdt-24-226/rc
Data Sharing Statement: Available at https://cdt.amegroups.com/article/view/10.21037/cdt-24-226/dss
Peer Review File: Available at https://cdt.amegroups.com/article/view/10.21037/cdt-24-226/prf
Conflicts of Interest: All authors have completed the ICMJE uniform disclosure form (available at https://cdt.amegroups.com/article/view/10.21037/cdt-24-226/coif). Y.K. serves as an unpaid editorial board member of Cardiovascular Diagnosis and Therapy from September 2023 to August 2025. Y.K. has received research support from Kowa, Nipro and Abbott, and honoraria from Nipro, Abbott, Kowa, Amgen, Sanofi, Astellas, Takeda and Daiichi-Sankyo. K.M. has received honoraria from Abbot, Terumo, Amgen and Zeon Medical, and support for attending meetings from OrbusNeich. The other authors have no conflicts of interest to declare.
Ethical Statement: The authors are accountable for all aspects of the work in ensuring that questions related to the accuracy or integrity of any part of the work are appropriately investigated and resolved. The research protocol was approved by the ethics committee of National Cerebral & Cardiovascular Center (No. M24-055-9). Informed consent was not required due to the observational analysis of hospitalized patients. The study was conducted in accordance with the Declaration of Helsinki (as revised in 2013).
Open Access Statement: This is an Open Access article distributed in accordance with the Creative Commons Attribution-NonCommercial-NoDerivs 4.0 International License (CC BY-NC-ND 4.0), which permits the non-commercial replication and distribution of the article with the strict proviso that no changes or edits are made and the original work is properly cited (including links to both the formal publication through the relevant DOI and the license). See: https://creativecommons.org/licenses/by-nc-nd/4.0/.
References
- Samsky MD, Morrow DA, Proudfoot AG, et al. Cardiogenic Shock After Acute Myocardial Infarction: A Review. JAMA 2021;326:1840-50. [Crossref] [PubMed]
- Arrigo M, Price S, Baran DA, et al. Optimising clinical trials in acute myocardial infarction complicated by cardiogenic shock: a statement from the 2020 Critical Care Clinical Trialists Workshop. Lancet Respir Med 2021;9:1192-202. [Crossref] [PubMed]
- Zweck E, Thayer KL, Helgestad OKL, et al. Phenotyping Cardiogenic Shock. J Am Heart Assoc 2021;10:e020085. [Crossref] [PubMed]
- Thayer KL, Zweck E, Ayouty M, et al. Invasive Hemodynamic Assessment and Classification of In-Hospital Mortality Risk Among Patients With Cardiogenic Shock. Circ Heart Fail 2020;13:e007099. [Crossref] [PubMed]
- Webb JG, Sleeper LA, Buller CE, et al. Implications of the timing of onset of cardiogenic shock after acute myocardial infarction: a report from the SHOCK Trial Registry. SHould we emergently revascularize Occluded Coronaries for cardiogenic shocK? J Am Coll Cardiol 2000;36:1084-90. [Crossref] [PubMed]
- Obling L, Frydland M, Hansen R, et al. Risk factors of late cardiogenic shock and mortality in ST-segment elevation myocardial infarction patients. Eur Heart J Acute Cardiovasc Care 2018;7:7-15. [Crossref] [PubMed]
- Qiao S, Zhang J, Kong Z, et al. Comparison of the prognosis for different onset stage of cardiogenic shock secondary to ST-segment elevation myocardial infarction. BMC Cardiovasc Disord 2020;20:302. [Crossref] [PubMed]
- Baran DA, Grines CL, Bailey S, et al. SCAI clinical expert consensus statement on the classification of cardiogenic shock: This document was endorsed by the American College of Cardiology (ACC), the American Heart Association (AHA), the Society of Critical Care Medicine (SCCM), and the Society of Thoracic Surgeons (STS) in April 2019. Catheter Cardiovasc Interv 2019;94:29-37. [Crossref] [PubMed]
- Naidu SS, Baran DA, Jentzer JC, et al. SCAI SHOCK Stage Classification Expert Consensus Update: A Review and Incorporation of Validation Studies: This statement was endorsed by the American College of Cardiology (ACC), American College of Emergency Physicians (ACEP), American Heart Association (AHA), European Society of Cardiology (ESC) Association for Acute Cardiovascular Care (ACVC), International Society for Heart and Lung Transplantation (ISHLT), Society of Critical Care Medicine (SCCM), and Society of Thoracic Surgeons (STS) in December 2021. J Am Coll Cardiol 2022;79:933-46. [Crossref] [PubMed]
- Kapur NK, Kanwar M, Sinha SS, et al. Criteria for Defining Stages of Cardiogenic Shock Severity. J Am Coll Cardiol 2022;80:185-98. [Crossref] [PubMed]
- Jentzer JC, van Diepen S, Barsness GW, et al. Cardiogenic Shock Classification to Predict Mortality in the Cardiac Intensive Care Unit. J Am Coll Cardiol 2019;74:2117-28. [Crossref] [PubMed]
- Baran DA, Long A, Badiye AP, et al. Prospective validation of the SCAI shock classification: Single center analysis. Catheter Cardiovasc Interv 2020;96:1339-47. [Crossref] [PubMed]
- Thygesen K, Alpert JS, Jaffe AS, et al. Fourth Universal Definition of Myocardial Infarction (2018). Circulation 2018;138:e618-51. [Crossref] [PubMed]
- Kimura K, Kimura T, Ishihara M, et al. JCS 2018 Guideline on Diagnosis and Treatment of Acute Coronary Syndrome. Circ J 2019;83:1085-196. [Crossref] [PubMed]
- Vahdatpour C, Collins D, Goldberg S. Cardiogenic Shock. J Am Heart Assoc 2019;8:e011991. [Crossref] [PubMed]
- Auffret V, Cottin Y, Leurent G, et al. Predicting the development of in-hospital cardiogenic shock in patients with ST-segment elevation myocardial infarction treated by primary percutaneous coronary intervention: the ORBI risk score. Eur Heart J 2018;39:2090-102. [Crossref] [PubMed]
- Pöss J, Köster J, Fuernau G, et al. Risk Stratification for Patients in Cardiogenic Shock After Acute Myocardial Infarction. J Am Coll Cardiol 2017;69:1913-20. [Crossref] [PubMed]
- Wang J, Shen B, Feng X, et al. A Review of Prognosis Model Associated With Cardiogenic Shock After Acute Myocardial Infarction. Front Cardiovasc Med 2021;8:754303. [Crossref] [PubMed]
- Miller PE, Jentzer J, Katz JN. Are Unselected Risk Scores in the Cardiac Intensive Care Unit Needed? J Am Heart Assoc 2021;10:e021940. [Crossref] [PubMed]
- Thiele H, Ohman EM, de Waha-Thiele S, et al. Management of cardiogenic shock complicating myocardial infarction: an update 2019. Eur Heart J 2019;40:2671-83. [Crossref] [PubMed]
- Esposito ML, Zhang Y, Qiao X, et al. Left Ventricular Unloading Before Reperfusion Promotes Functional Recovery After Acute Myocardial Infarction. J Am Coll Cardiol 2018;72:501-14. [Crossref] [PubMed]
- Meyns B, Stolinski J, Leunens V, et al. Left ventricular support by catheter-mounted axial flow pump reduces infarct size. J Am Coll Cardiol 2003;41:1087-95. [Crossref] [PubMed]