Interlinking pathways: a narrative review on the role of IL-6 in cancer and atherosclerosis
Introduction
Interleukin-6 (IL-6), a multifaceted cytokine, assumes a central role in the pathophysiology of various diseases, particularly cancer and atherosclerosis. These conditions represent major societal, public health, and economic challenges in the 21st century due to their high mortality and morbidity worldwide (1-3).
Over time, its known role has expanded beyond being a regulator in immune responses and inflammation, encompassing diverse physiological and pathological domains (4,5).
In oncology, IL-6 has been identified as a crucial element in tumor progression and metastasis. It promotes the proliferation and survival of cancer cells, aiding in the development of a conducive microenvironment for tumor growth. Its signaling through the Janus kinase (JAK)/signal transducer and activator of transcription (STAT) and mitogen-activated protein (MAP) kinase pathways demonstrates its importance in cancer cell survival and proliferation (6,7).
Furthermore, elevated IL-6 levels have been linked to poor prognosis in various types of cancer, marking it as a potential target for therapeutic intervention (8).
Atherosclerosis, a leading cause of cardiovascular diseases, also exhibits a significant involvement of IL-6 in its pathogenesis (9). IL-6 contributes to endothelial dysfunction and fosters a pro-inflammatory state within the vascular system (10). Its role in promoting human endothelial cell and monocyte adhesion further elucidates its contribution to the development of atherosclerotic lesions. Recent studies have highlighted the therapeutic potential of IL-6R antagonism in mitigating atherosclerosis, particularly in cases linked to clonal hematopoiesis associated with Tet2 deficiency (11).
The interplay between IL-6 pathways in both cancer and atherosclerosis suggests shared mechanisms underlying these diseases. Both conditions are characterized by chronic inflammation, with IL-6 playing a pivotal role as a mediator. This commonality points to potential overlapping pathways in their pathogenesis, offering a rationale for targeted strategies that modulate IL-6 activity.
Moreover, due to significant advances in oncological diagnostics and therapy, the number of cancer survivors has grown substantially (12). However, this positive trend is tempered by an increasing risk of cardiovascular complications among cancer patients. Today, the majority of cancer patients die from non-cancer causes, with cardiovascular disease significantly contributing to mortality and morbidity in cancer survivors (12-14). Additionally, cancer chemotherapy has been associated with the induction of atherosclerosis, further exacerbating cardiovascular risks (12-14).
Exploring the role of IL-6 in these conditions also involves understanding its regulatory mechanisms. IL-6 signaling is modulated by various factors, including soluble IL-6 receptors (IL-6R) and the trans-signaling system. The balance between classic signaling and trans-signaling pathways determines the cytokine’s functional outcomes in different contexts. Moreover, the genetic and epigenetic regulation of IL-6 expression adds another layer of complexity to its role in disease. Gene polymorphisms and epigenetic modifications can influence IL-6 levels, thereby affecting disease susceptibility and progression.
Rationale and knowledge gap
The multifaceted nature of IL-6 in disease pathogenesis highlights several existing research gaps. Despite significant advances, many aspects of IL-6 signaling, its interactions with other molecular pathways, and the shared mechanisms in atherosclerosis and cancer remain poorly understood.
Objective
This review comprehensively analyzes the multifaceted roles of IL-6, a versatile cytokine, in the contexts of cancer and atherosclerosis. It explores the shared pathogenic mechanisms mediated by IL-6 in both diseases, emphasizing their common root in chronic inflammation. Additionally, the review examines the regulatory dynamics of IL-6 signaling, including the impact of gene polymorphisms and epigenetic modifications. Finally, it underscores the therapeutic implications of modulating IL-6 in treating both conditions, advocating for personalized treatment approaches that integrate targeted therapies with lifestyle modifications. We present this article in accordance with the Narrative Review reporting checklist (available at https://cdt.amegroups.com/article/view/10.21037/cdt-24-344/rc).
Methods
In this paper, literature retrieval was conducted using Google Scholar, Scopus, and PubMed to find articles published in English that are related to IL-6 and report on the shared pathogenic mechanisms of IL-6 in cancer and atherosclerosis, covering studies from their inception through June 2024.
The search strategy is summarized in Table 1.
Table 1
Items | Specification |
---|---|
Date of search | 1 June 2024 |
Databases and other sources searched | PubMed, Scopus, and Google Scholar |
Search terms used | ((IL-6 OR “interleukin-6” OR “IL6” OR “IL6 pathways” OR “IL-6 signaling”) AND (atherosclerosis OR “vascular disease” OR “arteriosclerosis” OR “plaque formation” OR “endothelial dysfunction” OR “Atherosclerosis”(MeSH))) OR ((IL-6 OR “interleukin-6” OR “IL6” OR “IL6 pathways” OR “IL-6 signaling”) AND (cancer OR “malignant neoplasm” OR “tumor” OR “carcinoma” OR “oncology” OR “neoplasia” OR “Neoplasms”(MeSH))) |
Timeframe | From inception until June 2024 |
Inclusion and exclusion criteria | Inclusion criteria: original research, technical notes, review articles, and guidelines/expert written in English |
Exclusion criteria: documents not written in English, case reports, and publications from non-academic sources (such as websites, newspapers, and magazines) | |
Selection process | R.C. analyzed the scientific literature to extract relevant data for this narrative review |
Section 1: IL-6 and its biological significance
IL-6 is a cytokine that plays a significant role in immune regulation and inflammation, acting as both a pro-inflammatory and anti-inflammatory mediator (15) (Table 2).
Table 2
Aspect | Description | Cell types and factors involved | Mechanisms and pathways | Biological and clinical implications |
---|---|---|---|---|
Role in immune system | Central in mediating responses in both innate and adaptive immunity | Macrophages, T-cells, B-cells, endothelial cells | Responds to infections, tissue injuries | Facilitates communication between innate and adaptive immune systems; critical in immune response modulation |
Innate immunity functions | Pivotal in acute phase inflammatory response | Liver cells | Produces acute phase proteins like CRP and fibrinogen | Enhances pathogen defense, regulates inflammation, aids in tissue repair and healing |
Adaptive immunity functions | Modulates differentiation and function of B and T cells | B cells, T cells | Promotes B cell differentiation; affects T cell subsets | Key in antibody production, influences balance between pro-inflammatory and regulatory T cell responses |
Role in inflammation | Acts as a pro-inflammatory cytokine in various diseases | Macrophages, T-cells | Induces VEGF, stimulates cytokines like TNF-α, IL-1 | Involved in chronic inflammatory diseases pathogenesis; impacts disease progression and severity |
Transcriptional regulation | Controls IL-6 gene expression at transcriptional level | NF-κB, AP-1 | Activated by stress, cytokines, antigens | Critical in rapid IL-6 production in response to inflammatory stimuli; influences cellular responses to inflammation |
Post-transcriptional regulation | Involves mRNA stability and miRNA-mediated regulation | – | Affects mRNA degradation, translation inhibition | Allows for nuanced control of IL-6 levels; adapts IL-6 expression to specific cellular environments |
Regulatory feedback mechanisms | Involves complex feedback loops for homeostasis | Various immune cells, endothelial cells | Interactions between IL-6, its receptor, and signaling pathways | Maintains immune homeostasis; prevents overactivation of immune responses |
Role in autoimmune diseases | Contributory factor in autoimmune pathologies | Immune cells | Promotes autoantibody production, T cell activation | Linked to diseases like rheumatoid arthritis, systemic lupus erythematosus; potential target for therapeutic interventions |
Impact on tissue repair and regeneration | Influences healing processes | Fibroblasts, endothelial cells | Stimulates growth factors, collagen synthesis | Important in wound healing, tissue regeneration; excessive levels can lead to fibrosis |
Interactions with other cytokines | Works in conjunction with or in opposition to other cytokines | Immune cells | Synergistic or antagonistic interactions | Determines the nature of immune response, e.g., synergizing with IL-1 or antagonizing IL-10 |
Clinical implications in cancer | Role in cancer progression and metastasis | Cancer cells, immune cells | Promotes tumor growth, immune evasion | Target for cancer therapy; IL-6 blockers may reduce tumor progression and improve survival |
CRP, C-reactive protein; AP-1, activator protein 1; IL, interleukin; NF-κB, nuclear factor kappa B; TNF-α, tumor necrosis factor alpha; VEGF, vascular endothelial growth factor.
Its role in the immune system is complex and multifaceted, influencing various aspects of innate and adaptive immunity.
IL-6 in immune system regulation
IL-6 is important in regulating the immune system, and its functions are diverse and complex. Produced by a variety of cell types, including macrophages, T-cells, and endothelial cells (16), IL-6 is a key responder to physiological stimuli such as infections or tissue injuries. This multifunctional cytokine activates several immune response mechanisms, illustrating its central function in both innate and adaptive immunity.
In the context of innate immunity, IL-6 is pivotal during the acute phase response (17), a rapid inflammatory reaction to tissue injury or infection. This response involves the liver’s production of acute phase proteins, such as C-reactive protein (CRP), which are critical for enhancing the body’s ability to prevent infection and promote healing. IL-6 acts as a signaling molecule that alerts the liver to increase the synthesis of these proteins, thus serving a vital role in modulating the inflammatory response.
Regarding adaptive immunity, IL-6 significantly influences the differentiation and function of B and T cells (18). It promotes the differentiation of B cells into plasma cells, the antibody-producing cells crucial for humoral immunity (19). This process involves IL-6 binding to its receptor on B cells, triggering signaling pathways that lead to their proliferation and differentiation. The antibodies produced by plasma cells are essential for targeting and neutralizing pathogens (20).
IL-6 also aids in T-cell activation and differentiation (NO_PRINTED_FORM). It influences the fate of T cells, particularly affecting the differentiation of naïve T cells into various subsets, including Th17 cells and regulatory T cells (Tregs) (21). Th17 cells, known for their role in promoting inflammation, are involved in the defense against certain pathogens and in the pathogenesis of autoimmune diseases. Tregs, on the other hand, are crucial for maintaining immune tolerance and preventing excessive immune responses. The balance between these different T cell subsets, influenced by IL-6, is vital for the immune system’s proper functioning and for preventing autoimmune conditions.
IL-6 in inflammation
In the context of inflammation, IL-6 functions as a pro-inflammatory cytokine (22). It is crucial in the pathogenesis of several inflammatory diseases, including rheumatoid arthritis and inflammatory bowel disease. One of the pivotal mechanisms through which IL-6 enhances inflammation is by inducing VEGF (22), a signaling protein instrumental in angiogenesis—the formation of new blood vessels. In inflammatory settings, IL-6-induced VEGF expression serves two primary purposes: it increases vascular permeability, facilitating the migration of immune cells to the inflamed site, and it promotes angiogenesis, which can sustain and exacerbate chronic inflammation by supplying necessary nutrients and oxygen to the inflamed tissues (18,23).
Furthermore, IL-6 acts as a catalyst in the inflammatory response by stimulating the production of other pro-inflammatory cytokines. It activates cells like macrophages and T-cells, which then release additional cytokines such as TNF-α and IL-1 (18,24). This results in a cascade of cytokine activity, amplifying the inflammatory response and contributing to the persistence and severity of chronic inflammatory diseases.
IL-6 signaling
IL-6 signaling can proceed through either the classical or trans-signaling pathways. In the classical pathway, IL-6 binds to membrane-bound IL-6R, leading to the formation of a trimeric complex with gp130, which forms dimers and triggers intracellular signaling through pathways including JAK/STAT, rat sarcoma proto-oncogene (RAS)/mitogen-activated protein kinase (MAPK), and phosphoinositide-3 kinase (PI3K). Conversely, the trans pathway involves soluble IL-6R (sIL-6R), which activates cells lacking membrane-bound IL-6R, initiating downstream IL-6 signaling and inducing inflammatory responses in these cells (20).
Importantly, the IL-6 trans-signaling pathway is responsible for many of IL-6’s detrimental effects in chronic inflammatory diseases and cancer (25).
Recently, a third IL-6 signaling pathway was described, namely IL-6 cluster signaling. In this pathway, IL-6 forms complexes with membrane-bound IL-6R α-subunits on transmitting cells, which subsequently activate gp130 subunits on adjacent receiving cells (23) (Figure 1).
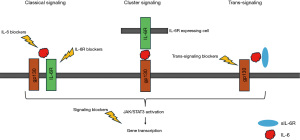
Regulation of IL-6 and epigenetic changes
The intricate regulation of IL-6 production involves a multifaceted interplay of various signaling pathways and transcription factors. At the transcriptional level, NF-κB and AP-1 emerge as pivotal players orchestrating the fine-tuning of IL-6 gene expression. Triggered by diverse stimuli like stress, cytokines, and environmental factors, NF-κB translocates to the nucleus, binding to specific sequences in the IL-6 gene promoter and initiating the transcriptional cascade (26-28).
Simultaneously, AP-1, responsive to growth factors and inflammatory cytokines, collaborates with NF-κB to enhance the transcription of the IL-6 gene (29). This collaborative effort forms part of a larger regulatory network, where these transcription factors interact with upstream signaling pathways and other transcriptional regulators, creating a sophisticated system for IL-6 expression (20,23,30,31).
Aberrant epigenetic modifications can dysregulate genes involved in chronic inflammation. The IL-6 pathway is affected by DNA methylation, which alters the patterns of related genes and promotes tumorigenesis (32-34).
Moving beyond transcriptional control, post-transcriptional mechanisms add another layer of complexity to IL-6 regulation. The stability of IL-6 mRNA emerges as a critical factor determining the quantity of IL-6 protein produced. Various cellular signals come into play, influencing the delicate balance between mRNA degradation and stabilization, thereby modulating the overall IL-6 protein synthesis.
Along with DNA methylation, microRNA (miRNA)-mediated regulation introduces another epigenetic mechanism implicated in the control of gene expression. These small, non-coding RNAs target the mRNA of the IL-6 gene, leading to either degradation or inhibition of translation. This dynamic interplay of miRNAs provides an additional layer of fine-tuning, allowing for precise modulation of IL-6 expression in response to a diverse array of internal and external stimuli (35-37).
In essence, the regulation of IL-6 is a dynamic and sophisticated process, influenced by an extensive range of factors. This complexity not only enables precise control of IL-6 levels in various physiological and pathological conditions but also underscores the adaptability of the regulatory mechanisms governing this crucial cytokine.
Section 2: IL-6 in cancer
The role of IL-6 in the pathogenesis of cancer has garnered significant attention in recent years (38). IL-6 is involved in various aspects of cancer development, including cell proliferation, survival, angiogenesis, and metastasis, and has been linked to a poor prognosis in several cancer types (8,31,39-44) (Table 3).
Table 3
References | Aspect | Relevant cancer | Signaling pathways involved | Detailed description | Clinical observations |
---|---|---|---|---|---|
Kumari et al., 2016 (8), Guo et al., 2012 (43) | Role in tumor growth | Breast and lung cancer | JAK/STAT pathway | IL-6 promotes cancer cell proliferation and survival | Linked to tumor progression in various cancers, particularly breast and lung cancer |
Kumari et al., 2016 (8), Masjedi et al., 2018 (44) | Tumor microenvironment | Breast and lung cancer | – | IL-6 levels are elevated in the tumor microenvironment | High IL-6 levels are associated with resistance to chemo- and radiotherapy |
Kumari et al., 2016 (8), Chen et al., 2010 (42) | Cell resistance | Breast, lung, and pharyngeal cancer | Counter-signalling pathways (anti-apoptotic/pro-survival) | IL-6 protects cancer cells from therapy-induced damage | IL-6 linked to reduced sensitivity of cancer cells to conventional anticancer therapies |
Masjedi et al., 2018 (44), Manore et al., 2022 (31) | Cell survival and invasiveness | Breast and lung cancer | – | High IL-6 levels in breast cancer contribute to tumor growth and therapeutic resistance | Targeting IL-6 and/or its receptor may potentiate anticancer therapies in breast cancer treatment |
Chen et al., 2010 (42) | Tumor microenvironment and cell resistance | Pharyngeal cancer | p-STAT3 pathway, EGFR nuclear translocation | IL-6 signaling contributes to resistance to irradiation and EGFR inhibitors | Blocking IL-6 signaling sensitizes cancer cells to treatments |
Berger et al., 2023 (40) | Tumor microenvironment and cell resistance | Breast and lung cancer | Sortilin-progranulin axis | IL-6 promotes breast cancer stem cell propagation | Targeting IL-6 could inhibit breast cancer progression, especially in estrogen receptor-negative models |
Nenu et al., 2023 (41) | Cell survival and invasiveness | Hepatocellular cancer | – | Dual role in promoting and inhibiting HCC progression | IL-6’s complex role in HCC underlines the need for tailored therapeutic strategies |
Florescu et al., 2023 (39) | Cell survival and invasiveness | Colorectal cancer | – | Correlation of IL-6 with diagnosis and prognosis of colorectal cancer | Elevated IL-6 levels associated with advanced tumor stages and short survival in patients |
EFGR, epidermal growth factor receptor; HCC, hepatocellular carcinoma; IL, interleukin; JAK/STAT, Janus kinase/signal transducers and activators of transcription; pSTAT3, phosphorylated transducer and activator of transcription-3.
In particular, classical IL-6 signaling is regarded as crucial for maintaining homeostatic processes, while trans-signaling has been shown to specifically amplify inflammation and promote inflammation-induced carcinogenesis (18,45).
One of the mechanisms through which IL-6 exerts this effect is by activating the JAK/STAT pathway. This pathway is crucial in transmitting signals from the cell surface to the nucleus, leading to the expression of genes that drive cell growth and survival. Excessive activation of STAT3 due to an overabundance of IL-6, coupled with oncogenic driver mutations, facilitates the development of malignant tumors (46-48).
IL-6 also plays a role in angiogenesis, the process of new blood vessel formation, which is essential for tumor growth and metastasis (38). It does so by upregulating vascular endothelial growth factor (VEGF) and other angiogenic factors (49). Furthermore, IL-6 can promote metastasis by enhancing the invasive capabilities of cancer cells and by modifying the tumor microenvironment to favor cancer cell migration and invasion (24).
Therapeutic implications
Research using IL-6-deficient mice has demonstrated that IL-6 plays a significant role in the pathogenesis of various inflammatory conditions (50,51).
Consequently, inhibiting IL-6 has become a promising therapeutic approach worthy of clinical translation. In principle, IL-6 signaling can be achieved by using antibodies targeting any component of the receptor signaling complex—whether IL-6 itself, IL-6R, or gp130. The initial clinical trial of an IL-6-specific inhibitor was conducted in patients with plasma cell leukemia, demonstrating suppression of myeloma cell proliferation in the bone marrow and a decrease in CRP levels (52).
Subsequently, humanized monoclonal antibodies to IL-6R, such as tocilizumab, sarilumab, and satralizumab, were developed to prevent IL-6 from binding to its receptor. Notably, none of these IL-6 signaling inhibitors can differentiate between classical signaling and trans-signaling; they block both pathways simultaneously. To address this, a selective inhibitor of IL-6 trans-signaling that does not interfere with classical signaling, sgp130Fc (olamkicept), was developed. Another therapeutic strategy for targeting IL-6 is linked to JAK/STAT pathway (53-56). In cancer, IL-6’s role in tumor growth, survival, and immune evasion makes it a compelling therapeutic target (57-69).
Previous studies focusing on IL-6 inhibitors are summarized in Table 4.
Table 4
Authors, year | Number of patients | Country | Relevant cancer | Study design | Phase | Study focus | Role of IL-6 | Outcome data | Key findings related to IL-6 |
---|---|---|---|---|---|---|---|---|---|
Kim et al., 2020 (63) | 39 | USA | Biliary tract cancer | Multi-center | Phase II | Efficacy of regorafenib | Association with overall survival | Overall survival, progression-free survival, and objective response rates | Elevated baseline IL-6 was associated with shorter overall survival |
Heath et al., 2019 (65) | 58 | USA | Prostate cancer | Multi-center | Phase II | Efficacy of cediranib in combination with docetaxel and prednisone | Predictive of progression | Overall survival and progression-free survival | Increased baseline levels of IL-6 were significantly associated with an increased risk of progression |
Haldar et al., 2018 (67) | 38 | Israel | Breast cancer | Multi-center | Phase II | Perioperative inhibition of β-adrenergic and COX2 signaling | Serum cytokine levels | Level of systemic inflammation and pro-metastatic/pro-growth biomarkers | Treatment reduced pro-inflammatory cytokines including IL-6 in the serum |
Puchalski et al., 2010 (66) | 68 | UK | Metastatic renal cell carcinoma | Single-center | Phase I/II | Pharmacodynamics of siltuximab | Pharmacokinetic and pharmacodynamic modeling | Level of systemic inflammation biomarkers | IL-6 levels were used as a pharmacodynamic marker to assess the efficacy of siltuximab |
Meyerhardt et al., 2012 (64) | 27 | USA | Metastatic colorectal cancer | Multi-center | Phase I | Efficacy of vandetanib combined with cetuximab and irinotecan | Biomarker analysis | Overall survival and progression-free survival | IL-6 was monitored as a potential biomarker for treatment efficacy, although no significant changes in IL-6 levels were observed during therapy |
Henry et al., 2006 (62) | 19 | USA | Prostate cancer | Multi-center | Phase II | Copper depletion with tetrathiomolybdate | Serum cytokine levels | Level of systemic inflammation and angiogenesis factors | IL-6 levels were elevated compared to normal controls prior to therapy but did not change significantly during treatment |
COX2, cyclooxygenase-2; IL, interleukin.
Generally, the primary adverse effects of anti-IL-6/IL-6R antibodies are associated with bacterial infections, likely because IL-6 coordinates innate and adaptive immune responses and activating the acute phase response. Inhibiting IL-6 trans-signaling could offer significant advantages as it does not interfere with the classical IL-6 signaling pathway, thus preserving the immune response against infections. Additionally, the major detrimental pro-inflammatory effects of IL-6 are mediated through trans-signaling (20,70).
Effectively treating cancer and enhancing the efficacy of current therapies, including immunotherapy, requires overcoming the immunosuppression caused by cancer-promoting inflammation. Immunotherapy often shows limited success in certain solid tumors due to the immune system’s suppression driven by chronic inflammation. This inflammation is highly complex, regulated by multiple interactive pathways, and involves numerous compensatory mechanisms, making it a difficult target. These challenges likely explain why cytokine-targeting drugs have not been effective as monotherapies in halting tumor progression. However, robust preclinical evidence suggests that combining inhibitors of IL-6 family cytokine signaling with immune checkpoint blockade (ICB) could be a promising therapeutic strategy (7).
Several clinical trials are testing the efficacy of combining immunotherapy and blockade of IL-6 family cytokines (e.g., NCT03866239, NCT03821246, NCT04191421, etc.).
Section 3: IL-6 in atherosclerosis
Atherosclerosis is the primary cause of cardiovascular and cerebrovascular diseases and a major contributor to human mortality and morbidity (71-75). Numerous studies have shown that atherosclerosis is a chronic inflammatory condition with IL-6 has a crucial role (76). The pathogenesis of atherosclerosis involves multiple steps, beginning with the deposition of low-density lipoproteins (LDLs) in the arterial intima, along with local oxidative stress and immune and inflammatory activation. IL-6 sustains vascular inflammation by promoting smooth muscle cell (SMC) proliferation and migration, endothelial dysfunction, and the recruitment and activation of inflammatory mediators (76). These processes lead to the development and destabilization of atherosclerotic plaques (77).
Endothelial dysfunction represents the primary stage of atherosclerosis formation. IL-6 promotes the expression of adhesion molecules on endothelial cells, such as vascular cell adhesion molecule-1 (VCAM-1), intercellular adhesion molecule-1 (ICAM-1), and E-selectin. This expression facilitates not only endothelial permeability but also the attachment and infiltration of the vascular wall by circulating immune cells, including neutrophils, lymphocytes, and macrophages, thereby enhancing the level of inflammatory molecules (76,78).
Schuett et al. confirmed this data in atherosclerosis-prone mice by using a fusion protein of the IL-6 trans-signaling inhibitor soluble gp130 to block trans-signaling in LDL receptor-deficient mice, demonstrating that treatment with this fusion protein was effective. They found that treatment with this fusion protein reduced the progression of atherosclerotic lesions, which was accompanied by decreased expression of adhesion molecules on the endothelium and reduced macrophage infiltration in the aorta, underscoring the significance of trans-signaling in the pro-atherogenic properties of IL-6 (79).
In addition to its various effects on the endothelium, IL-6 significantly impacts vascular smooth muscle by inducing proliferation, migration, and hypertrophy (76). Both endothelial and SMCs respond to these conditions by expressing chemical mediators and recruiting macrophages. These macrophages accumulate intracellular lipids, leading to pathologic intimal thickening with lipid pools. Exogenously administered IL-6 significantly accelerated the development of fatty lesions in mice, increasing the size of the fatty streak by up to 5.1-fold (80).
In the prospective population-based Cardiovascular Health Study (CHS), the role of circulating IL-6 levels in the prediction carotid plaque severity, vulnerability, and progression was evaluated in 4,334 patients with carotid atherosclerosis. The authors demonstrated that IL-6 predicted plaque severity (β=0.09, P=1.3×10−3), vulnerability [odds ratio (OR), 1.21; 95% confidence interval (CI), 1.05–1.40; P=7.4×10−3, E-value =1.71], and progression (OR, 1.44; 95% CI, 1.23–1.69; P=9.1×10−6, E-value =2.24). Moreover, a cut-off of 2.0 pg/mL may suggest the selection of individuals that would benefit from anti-IL-6 drugs (81).
The association between plaque progression and IL-6 was demonstrated in a study by Okazaki et al., which prospectively followed 210 patients with carotid atherosclerosis over a median of 9.0±1.0 years. Baseline levels and progression of carotid mean-maximal intima-media thickness were positively correlated with IL-6 levels (P<0.001 for both). This significant association remained even after adjusting for age, sex, traditional risk factors, and baseline carotid mean-maximal intima-media thickness, suggesting that chronic elevation of serum IL-6 is associated with the progression of atherosclerosis (82).
IL-6 not only accelerates the formation of atherosclerotic plaques but also contributes to plaque instability and cardiovascular events. Mossmann et al. investigated the role of increased serum IL-6 as a predictive marker of cardiovascular events in 100 consecutive patients undergoing coronary angiography, with a median follow-up of 297 weeks (95% CI: 266.95–327.16). They found that an IL-6 level higher than 0.44 pg/mL was associated with a worse prognosis (83).
Moreover, IL-6 contributes to vascular calcifications in chronic inflammation (84,85), a well-known imaging biomarker of plaque instability (86-90).
Given the significant and growing global burden of atherosclerosis, it is essential to identify the cellular mechanisms underlying its pathogenesis. This knowledge can lead to the discovery of novel therapeutic targets for preventing or mitigating its clinical consequences.
Therapeutic implications
In 2017, the Canakinumab Anti-Inflammatory Thrombosis Outcomes Study (CANTOS) provided compelling evidence supporting the inflammation hypothesis of atherothrombosis. This study, involving 10,000 participants, demonstrated that targeting IL-1β significantly reduces rates of major cardiovascular events, independent of effects on cholesterol, blood pressure, or coagulation. Importantly, the degree of risk reduction observed in CANTOS correlated directly with the reduction in IL-6 levels achieved by participants. Among those treated with canakinumab who experienced greater than median reductions in IL-6 after the first dose, a 36% decrease in major adverse cardiovascular events was observed with long-term therapy [hazard ratio (HR) 0.64, 95% CI: 0.54–0.77; P<0.0001] (91). Conversely, among patients who did not experienced IL-6 reduction, Canakinumab showed marginal improvement over placebo (92). This trial emphasized IL-6 as a potential therapeutic target.
Therapeutic strategies for targeting IL-6 can be categorized into three interconnected approaches: direct inhibition of IL-6, targeting receptors like IL-6R or gp130, and focusing on downstream kinases or transcription factors within the JAK-STAT pathway (93).
Existing clinical trials focusing on IL-6 inhibitors in atherosclerosis are summarized in Table 5.
Table 5
Clinical trial (NCT number) | Country | Study design | Target | Conditions | Interventions | Phase | Outcome measure |
---|---|---|---|---|---|---|---|
NCT03926117 | USA | Multicenter | IL-6 ligand | Individuals with elevated high-sensitivity CRP and chronic kidney disease | Ziltivekimab | II | Level of systemic inflammation biomarkers |
NCT05485961 | United States, Canada, Belgium, Germany and Australia | Multicenter | IL-6 ligand | Adults with cardiovascular disease and/or diabetes receiving maintenance dialysis With high-sensitivity CRP ≥2 mg/L at baseline | Clazakizumab | IIb | Level of systemic inflammation biomarkers |
NCT04626505 | Japan | Multicenter | IL-6 ligand | Individuals with elevated high-sensitivity CRP and chronic kidney disease | Ziltivekimab | II | Level of systemic inflammation biomarkers |
NCT06447701 | China | Multicenter | IL-6 receptor | Patients having an ischemic stroke or a TIA prior to randomization with symptomatic intracranial atherosclerosis (50–99%) | Tocilizumab | I | Newly diagnosed ischemic stroke |
NCT05021835 | USA | Multicenter | IL-6 ligand | Individuals with elevated high-sensitivity CRP and chronic kidney disease | Ziltivekimab | III | Major adverse cardiovascular event |
NCT03004703 | Norway | Multicenter | IL-6 receptor | Patients with ST-segment elevation myocardial infarction | Tocilizumab | II | Myocardial salvage index |
NCT01491074 | Norway | Multicenter | IL-6 receptor | Patients with acute non-ST-segment elevation myocardial infarction | Tocilizumab | II | Level of systemic inflammation biomarkers |
NCT05350592 | Denmark | Single-center | IL-6 receptor | Patients with acute myocardial infarction | Tocilizumab | II | Level of systemic inflammation biomarkers and adverse cardiovascular outcomes |
CRP, C-reactive protein; IL-6, interleukin 6; TIA, transient ischemic attack.
Section 4: common pathways and mechanisms in IL-6-driven cancer and atherosclerosis
Cancer and atherosclerosis are interconnected through shared risk factors and a fundamental pathophysiological mechanism involving chronic inflammation, also mediated through IL-6 signaling. The intersection of these two diseases is crucial, as it highlights not only their biological similarities but also the possibility of overlapping therapeutic strategies. This section delves into the common pathways and mechanisms through which IL-6 influences both cancer and atherosclerosis, underscoring the potential for overlapping therapeutic strategies.
Cancer and atherosclerosis: common risk factors
Diabetes, smoking, dyslipidemia, obesity, hypertension, sedentary behavior, unhealthy diets, physical inactivity, alcohol abuse, impaired immune response are risk factors common to both atherosclerosis development and progression and cancer. The presence of shared risk factors between cancer and atherosclerosis implies the existence of common pathogenetic mechanisms, prominently involving chronic inflammation (94).
Cancer and atherosclerosis: IL-6 a common mediator
Several biological processes have been identified that share mechanisms between cancer and atherosclerosis, with IL-6 serving a common role in both (95,96).
IL-6’s role as a pro-inflammatory cytokine is crucial in both diseases. In cancer, inflammation promotes tumor initiation, progression, and metastasis, while in atherosclerosis, chronic inflammation drives the development and progression of atherosclerotic plaques (95).
One of the primary mechanisms through which IL-6 exerts its effects in both cancer and atherosclerosis is the JAK/STAT pathway. Upon binding to its receptor, IL-6 activates JAK, which in turn phosphorylates and activates STAT proteins. These activated STATs translocate to the nucleus and modulate the expression of genes involved in cell proliferation, survival, and inflammation. Dysregulation of the JAK/STAT pathway is a hallmark of several cancers, contributing to uncontrolled cell growth and survival. Similarly, in atherosclerosis, the JAK/STAT pathway promotes the inflammatory processes that underpin plaque development. Activation of this pathway sustains inflammation, facilitating both tumor growth in cancer and plaque formation in atherosclerosis (97).
The IL-6 amplifier (IL-6 Amp) is another mechanism by which IL-6 perpetuates chronic inflammation in both diseases. This positive feedback loop involves the synergistic activation of STAT3 and NF-κB, which leads to the production of various pro-inflammatory cytokines and chemokines. In cancer, this mechanism sustains an inflammatory tumor microenvironment, promoting tumor growth and metastasis. In atherosclerosis, the IL-6 Amp enhances the recruitment of immune cells to the arterial wall, driving plaque progression and instability (50).
Angiogenesis, the formation of new blood vessels, is another shared process between cancer and atherosclerosis, with IL-6 playing a critical role. By stimulating the production of VEGF and other angiogenic factors, IL-6 enhances blood vessel formation. In cancer, angiogenesis is essential for tumor growth and metastasis, providing the tumor with the blood supply needed to sustain rapid cell proliferation. In atherosclerosis, angiogenesis contributes to the formation of vulnerable plaques, which are prone to rupture and lead to acute cardiovascular events (55,98,99).
Both cancer and atherosclerosis are characterized by oxidative stress and endothelial dysfunction, with IL-6 playing a central role in exacerbating these processes. In cancer, oxidative stress contributes to DNA damage and mutation, promoting carcinogenesis. In atherosclerosis, it leads to the dysfunction of endothelial cells, a critical early step in plaque formation. IL-6, through its pro-inflammatory effects, exacerbates oxidative stress and endothelial dysfunction in both diseases (100-102).
IL-6 modulates the immune system, impacting both cancer and atherosclerosis. In cancer, IL-6 contributes to the creation of an immunosuppressive tumor microenvironment, which allows tumors to evade immune surveillance. By promoting the differentiation of M2 macrophages and myeloid-derived suppressor cells (MDSCs), IL-6 enhances tumor growth and metastasis by suppressing anti-tumor immune responses (50).
In atherosclerosis, IL-6 influences the immune response within plaques, affecting the balance between pro-inflammatory and anti-inflammatory cells. By promoting the recruitment and activation of macrophages, IL-6 drives the inflammatory processes that lead to plaque formation and progression (96).
Recent research has highlighted genomic instability, such as clonal hematopoiesis of indeterminate potential, as a potential linking mechanism between cancer and atherosclerosis, with IL-6 playing a central role. Clonal hematopoiesis, the expansion of blood cell clones with certain genetic mutations, is associated with an increased risk of both hematological malignancies and atherosclerotic cardiovascular disease (95,103).
Discussion
The exploration of IL-6’s mechanism in cancer and atherosclerosis shows the complexity and interconnectedness of inflammatory processes in chronic diseases (18,70). The function of IL-6 in both cancer and atherosclerosis highlights the cytokine’s centrality in disease pathology. Its involvement in inflammation, immune modulation, and cellular proliferation provides a common thread linking these two seemingly disparate conditions (7).
The exploration of IL-6 as a therapeutic target in the realms of cancer and atherosclerosis is marked by its significant potential, albeit accompanied by intricate challenges and implications for future research and clinical practice.
The systemic nature of IL-6’s role in the body implies that its modulation as a treatment strategy must be approached with a nuanced understanding of its widespread effects. Particularly, there is a need to balance the therapeutic benefits of targeting IL-6 against the potential risks, such as impaired immune responses or heightened susceptibility to infections (7,38). This consideration is crucial, given IL-6’s integral role in immune regulation and inflammation.
Looking ahead, the path to fully leveraging IL-6’s therapeutic potential is paved with several key research directions. A deeper molecular understanding of IL-6’s role across different diseases is essential. This knowledge will facilitate the development of more effective, targeted treatments, particularly by shedding light on IL-6’s interactions with other significant signaling pathways in cancer and atherosclerosis. Alongside this, the identification of biomarkers predictors of treatment response to IL-6 targeted therapies represents a critical frontier (104,105).
Such biomarkers could guide patient selection, ensuring that those most likely to benefit from these treatments are accurately identified, thereby enhancing the precision and efficacy of therapy.
In terms of treatment strategies, the exploration of combination therapies involving IL-6 targeting agents is particularly promising. These combinations, which might include traditional chemotherapy, targeted agents, or immunotherapies in cancer, and statins or other anti-inflammatory drugs in atherosclerosis, as well as lifestyle changes, hold the potential for synergistic effects. Such strategies could not only amplify therapeutic efficacy but also counteract the development of resistance (106).
From a clinical perspective, the insights into IL-6’s function have immediate and significant implications. They herald new approaches to diagnosis and treatment, emphasizing the need for comprehensive, holistic management of chronic diseases. This approach should ideally integrate targeted IL-6 therapies with lifestyle interventions and traditional treatments, addressing the multifaceted nature of these conditions. Furthermore, patient education about IL-6’s role and the importance of comprehensive management strategies is pivotal. This includes a focus on adherence to therapy and lifestyle changes.
Clinicians must also be proactive in managing potential adverse effects of IL-6 targeted therapies and vigilant in monitoring for signs of immune suppression or other systemic impacts. Regular patient monitoring is essential to ensure the safety and effectiveness of these treatments (107).
Strengths and limitations
The main strength of this narrative review lies in its comprehensive scope. It offers a detailed technical background on IL-6 pathophysiology and highlights its shared pathogenic mechanisms through chronic inflammation in both cancer and atherosclerosis. Additionally, it synthesizes potential therapeutic options targeting IL-6 pathways in both diseases.
The primary limitation is that, despite our best efforts to include the most relevant research, the field of IL-6 is continuously evolving, as evidenced by several ongoing clinical trials. As a result, newer findings may not be reflected in this review. Other limitations include potential biases from the selection of included studies and the reliance on subjective interpretations of the literature. Nonetheless, every effort has been made to present a balanced, informative, and rigorous perspective on the topic.
Conclusions
While targeting IL-6 offers a promising avenue for treating cancer and atherosclerosis, it requires a comprehensive, individualized approach that considers the unique challenges and intricacies inherent in its modulation. Ongoing research in this area is vital, not only to enhance our understanding of IL-6’s diverse roles but also to develop more effective and personalized treatment strategies.
Acknowledgments
Funding: None.
Footnote
Reporting Checklist: The authors have completed the Narrative Review reporting checklist. Available at https://cdt.amegroups.com/article/view/10.21037/cdt-24-344/rc
Peer Review File: Available at https://cdt.amegroups.com/article/view/10.21037/cdt-24-344/prf
Conflicts of Interest: Both authors have completed the ICMJE uniform disclosure form (available at https://cdt.amegroups.com/article/view/10.21037/cdt-24-344/coif). L.S. serves as an unpaid editorial board member of Cardiovascular Diagnosis and Therapy from September 2023 to August 2025. The other author has no conflicts of interest to declare.
Ethical Statement: The authors are accountable for all aspects of the work in ensuring that questions related to the accuracy or integrity of any part of the work are appropriately investigated and resolved.
Open Access Statement: This is an Open Access article distributed in accordance with the Creative Commons Attribution-NonCommercial-NoDerivs 4.0 International License (CC BY-NC-ND 4.0), which permits the non-commercial replication and distribution of the article with the strict proviso that no changes or edits are made and the original work is properly cited (including links to both the formal publication through the relevant DOI and the license). See: https://creativecommons.org/licenses/by-nc-nd/4.0/.
References
- Bray F, Laversanne M, Sung H, et al. Global cancer statistics 2022: GLOBOCAN estimates of incidence and mortality worldwide for 36 cancers in 185 countries. CA Cancer J Clin 2024;74:229-63. [Crossref] [PubMed]
- Chen W, Li Z, Zhao Y, et al. Global and national burden of atherosclerosis from 1990 to 2019: trend analysis based on the Global Burden of Disease Study 2019. Chin Med J (Engl) 2023;136:2442-50. [Crossref] [PubMed]
- Saba L, Antignani PL, Gupta A, et al. International Union of Angiology (IUA) consensus paper on imaging strategies in atherosclerotic carotid artery imaging: From basic strategies to advanced approaches. Atherosclerosis 2022;354:23-40. [Crossref] [PubMed]
- Rose-John S, Waetzig GH, Scheller J, et al. The IL-6/sIL-6R complex as a novel target for therapeutic approaches. Expert Opin Ther Targets 2007;11:613-24. [Crossref] [PubMed]
- Rose-John S, Schooltink H. Cytokines are a therapeutic target for the prevention of inflammation-induced cancers. Recent Results Cancer Res 2007;174:57-66. [Crossref] [PubMed]
- Orange ST, Leslie J, Ross M, et al. The exercise IL-6 enigma in cancer. Trends Endocrinol Metab 2023;34:749-63. [Crossref] [PubMed]
- Soler MF, Abaurrea A, Azcoaga P, et al. New perspectives in cancer immunotherapy: targeting IL-6 cytokine family. J Immunother Cancer 2023;11:e007530. [Crossref] [PubMed]
- Kumari N, Dwarakanath BS, Das A, et al. Role of interleukin-6 in cancer progression and therapeutic resistance. Tumour Biol 2016;37:11553-72. [Crossref] [PubMed]
- Prapiadou S, Živković L, Thorand B, et al. Proteogenomic Data Integration Reveals CXCL10 as a Potentially Downstream Causal Mediator for IL-6 Signaling on Atherosclerosis. Circulation 2024;149:669-83. [Crossref] [PubMed]
- Kang S, Kishimoto T. Interplay between interleukin-6 signaling and the vascular endothelium in cytokine storms. Exp Mol Med 2021;53:1116-23. [Crossref] [PubMed]
- Huynh K. IL-6R antagonism ameliorates atherosclerosis linked to Tet2-related clonal haematopoiesis. Nat Rev Cardiol 2023;20:579. [Crossref] [PubMed]
- Willems RAL, Winckers K, Biesmans C, et al. Evolving data on cardiovascular complications in cancer. Thromb Res 2022;213:S87-94. [Crossref] [PubMed]
- Raposeiras Roubín S, Cordero A. The Two-way Relationship Between Cancer and Atherosclerosis. Rev Esp Cardiol (Engl Ed) 2019;72:487-94. [Crossref] [PubMed]
- Zhou F, Zhu X, Liu Y, et al. Coronary atherosclerosis and chemotherapy: From bench to bedside. Front Cardiovasc Med 2023;10:1118002. [Crossref] [PubMed]
- Uciechowski P, Dempke WCM. Interleukin-6: A Masterplayer in the Cytokine Network. Oncology 2020;98:131-7. [Crossref] [PubMed]
- Tanaka T, Narazaki M, Masuda K, et al. Regulation of IL-6 in Immunity and Diseases. Adv Exp Med Biol 2016;941:79-88. [Crossref] [PubMed]
- Rose-John S, Winthrop K, Calabrese L. The role of IL-6 in host defence against infections: immunobiology and clinical implications. Nat Rev Rheumatol 2017;13:399-409. [Crossref] [PubMed]
- Hunter CA, Jones SA. IL-6 as a keystone cytokine in health and disease. Nat Immunol 2015;16:448-57. [Crossref] [PubMed]
- Tanaka T, Narazaki M, Kishimoto T. IL-6 in inflammation, immunity, and disease. Cold Spring Harb Perspect Biol 2014;6:a016295. [Crossref] [PubMed]
- Rose-John S. Interleukin-6 signalling in health and disease. F1000Res 2020;9:F1000 Faculty Rev-1013.
- Korn T, Hiltensperger M. Role of IL-6 in the commitment of T cell subsets. Cytokine 2021;146:155654. [Crossref] [PubMed]
- Aliyu M, Zohora FT, Anka AU, et al. Interleukin-6 cytokine: An overview of the immune regulation, immune dysregulation, and therapeutic approach. Int Immunopharmacol 2022;111:109130. [Crossref] [PubMed]
- Rose-John S, Jenkins BJ, Garbers C, et al. Targeting IL-6 trans-signalling: past, present and future prospects. Nat Rev Immunol 2023;23:666-81. [Crossref] [PubMed]
- Fisher DT, Appenheimer MM, Evans SS. The two faces of IL-6 in the tumor microenvironment. Semin Immunol 2014;26:38-47. [Crossref] [PubMed]
- Briukhovetska D, Dörr J, Endres S, et al. Interleukins in cancer: from biology to therapy. Nat Rev Cancer 2021;21:481-99. [Crossref] [PubMed]
- Brasier AR. The nuclear factor-kappaB-interleukin-6 signalling pathway mediating vascular inflammation. Cardiovasc Res 2010;86:211-8. [Crossref] [PubMed]
- Akhtar M, Guo S, Guo YF, et al. Upregulated-gene expression of pro-inflammatory cytokines (TNF-α, IL-1β and IL-6) via TLRs following NF-κB and MAPKs in bovine mastitis. Acta Trop 2020;207:105458. [Crossref] [PubMed]
- Guo D, Wang Q, Li A, et al. Liquiritin targeting Th17 cells differentiation and abnormal proliferation of keratinocytes alleviates psoriasis via NF-κB and AP-1 pathway. Phytother Res 2024;38:174-86. [Crossref] [PubMed]
- Deichaite I, Sears TJ, Sutton L, et al. Differential regulation of TNFα and IL-6 expression contributes to immune evasion in prostate cancer. J Transl Med 2022;20:527. [Crossref] [PubMed]
- Grove CA, Walhout AJ. Transcription factor functionality and transcription regulatory networks. Mol Biosyst 2008;4:309-14. [Crossref] [PubMed]
- Manore SG, Doheny DL, Wong GL, et al. IL-6/JAK/STAT3 Signaling in Breast Cancer Metastasis: Biology and Treatment. Front Oncol 2022;12:866014. [Crossref] [PubMed]
- Tekpli X, Landvik NE, Anmarkud KH, et al. DNA methylation at promoter regions of interleukin 1B, interleukin 6, and interleukin 8 in non-small cell lung cancer. Cancer Immunol Immunother 2013;62:337-45. [Crossref] [PubMed]
- D'Anello L, Sansone P, Storci G, et al. Epigenetic control of the basal-like gene expression profile via Interleukin-6 in breast cancer cells. Mol Cancer 2010;9:300. [Crossref] [PubMed]
- Candido S, Tomasello BMR, Lavoro A, et al. Novel Insights into Epigenetic Regulation of IL6 Pathway: In Silico Perspective on Inflammation and Cancer Relationship. Int J Mol Sci 2021;22:10172. [Crossref] [PubMed]
- Yao Q, Chen Y, Zhou X. The roles of microRNAs in epigenetic regulation. Curr Opin Chem Biol 2019;51:11-7. [Crossref] [PubMed]
- Jansson MD, Lund AH. MicroRNA and cancer. Mol Oncol 2012;6:590-610. [Crossref] [PubMed]
- Hussen BM, Hidayat HJ, Salihi A, et al. MicroRNA: A signature for cancer progression. Biomed Pharmacother 2021;138:111528. [Crossref] [PubMed]
- Rašková M, Lacina L, Kejík Z, et al. The Role of IL-6 in Cancer Cell Invasiveness and Metastasis-Overview and Therapeutic Opportunities. Cells 2022;11:3698. [Crossref] [PubMed]
- Florescu DN, Boldeanu MV, Șerban RE, et al. Correlation of the Pro-Inflammatory Cytokines IL-1β, IL-6, and TNF-α, Inflammatory Markers, and Tumor Markers with the Diagnosis and Prognosis of Colorectal Cancer. Life (Basel) 2023;13:2261. [Crossref] [PubMed]
- Berger K, Persson E, Gregersson P, et al. Interleukin-6 Induces Stem Cell Propagation through Liaison with the Sortilin-Progranulin Axis in Breast Cancer. Cancers (Basel) 2023;15:5757. [Crossref] [PubMed]
- Nenu I, Toadere TM, Topor I, et al. Interleukin-6 in Hepatocellular Carcinoma: A Dualistic Point of View. Biomedicines 2023;11:2623. [Crossref] [PubMed]
- Chen CC, Chen WC, Lu CH, et al. Significance of interleukin-6 signaling in the resistance of pharyngeal cancer to irradiation and the epidermal growth factor receptor inhibitor. Int J Radiat Oncol Biol Phys 2010;76:1214-24. [Crossref] [PubMed]
- Guo Y, Xu F, Lu T, et al. Interleukin-6 signaling pathway in targeted therapy for cancer. Cancer Treat Rev 2012;38:904-10. [Crossref] [PubMed]
- Masjedi A, Hashemi V, Hojjat-Farsangi M, et al. The significant role of interleukin-6 and its signaling pathway in the immunopathogenesis and treatment of breast cancer. Biomed Pharmacother 2018;108:1415-24. [Crossref] [PubMed]
- Atsumi T, Singh R, Sabharwal L, et al. Inflammation amplifier, a new paradigm in cancer biology. Cancer Res 2014;74:8-14. [Crossref] [PubMed]
- Ernst M, Najdovska M, Grail D, et al. STAT3 and STAT1 mediate IL-11-dependent and inflammation-associated gastric tumorigenesis in gp130 receptor mutant mice. J Clin Invest 2008;118:1727-38. [Crossref] [PubMed]
- Hu B, Elinav E, Huber S, et al. Microbiota-induced activation of epithelial IL-6 signaling links inflammasome-driven inflammation with transmissible cancer. Proc Natl Acad Sci U S A 2013;110:9862-7. [Crossref] [PubMed]
- Caetano MS, Zhang H, Cumpian AM, et al. IL6 Blockade Reprograms the Lung Tumor Microenvironment to Limit the Development and Progression of K-ras-Mutant Lung Cancer. Cancer Res 2016;76:3189-99. [Crossref] [PubMed]
- Zegeye MM, Andersson B, Sirsjö A, et al. IL-6 trans-Signaling Impairs Sprouting Angiogenesis by Inhibiting Migration, Proliferation and Tube Formation of Human Endothelial Cells. Cells 2020;9:1414. [Crossref] [PubMed]
- Hirano T. IL-6 in inflammation, autoimmunity and cancer. Int Immunol 2021;33:127-48. [Crossref] [PubMed]
- Roohi E, Jaafari N, Hashemian F. On inflammatory hypothesis of depression: what is the role of IL-6 in the middle of the chaos? J Neuroinflammation 2021;18:45. [Crossref] [PubMed]
- Klein B, Wijdenes J, Zhang XG, et al. Murine anti-interleukin-6 monoclonal antibody therapy for a patient with plasma cell leukemia. Blood 1991;78:1198-204. [Crossref] [PubMed]
- Wang Y, Wang Z, Li S, et al. Deciphering JAK/STAT signaling pathway: A multifaceted approach to tumorigenesis, progression and therapeutic interventions. Int Immunopharmacol 2024;131:111846. [Crossref] [PubMed]
- Brooks AJ, Putoczki T. JAK-STAT Signalling Pathway in Cancer. Cancers (Basel) 2020;12:1971. [Crossref] [PubMed]
- Wang SW, Sun YM. The IL-6/JAK/STAT3 pathway: potential therapeutic strategies in treating colorectal cancer Int J Oncol 2014;44:1032-40. (Review). [Crossref] [PubMed]
- Jia J, Zhou X, Chu Q. Mechanisms and therapeutic prospect of the JAK-STAT signaling pathway in liver cancer. Mol Cell Biochem 2024; Epub ahead of print. [Crossref] [PubMed]
- Chalaris A, Rabe B, Paliga K, et al. Apoptosis is a natural stimulus of IL6R shedding and contributes to the proinflammatory trans-signaling function of neutrophils. Blood 2007;110:1748-55. [Crossref] [PubMed]
- Becker C, Fantini MC, Schramm C, et al. TGF-beta suppresses tumor progression in colon cancer by inhibition of IL-6 trans-signaling. Immunity 2004;21:491-501. [Crossref] [PubMed]
- Saad MI, Alhayyani S, McLeod L, Yu L, Alanazi M, Deswaerte V, et al. ADAM 17 selectively activates the IL‐6 trans‐signaling/ERK MAPK axis in KRAS‐addicted lung cancer. EMBO Mol Med. 2019;11:e9976. [Crossref] [PubMed]
- Lesina M, Kurkowski MU, Ludes K, et al. Stat3/Socs3 activation by IL-6 transsignaling promotes progression of pancreatic intraepithelial neoplasia and development of pancreatic cancer. Cancer Cell 2011;19:456-69. [Crossref] [PubMed]
- Rosenberg N, Van Haele M, Lanton T, et al. Combined hepatocellular-cholangiocarcinoma derives from liver progenitor cells and depends on senescence and IL-6 trans-signaling. J Hepatol 2022;77:1631-41. [Crossref] [PubMed]
- Henry NL, Dunn R, Merjaver S, et al. Phase II trial of copper depletion with tetrathiomolybdate as an antiangiogenesis strategy in patients with hormone-refractory prostate cancer. Oncology 2006;71:168-75. [Crossref] [PubMed]
- Kim RD, Sanoff HK, Poklepovic AS, et al. A multi-institutional phase 2 trial of regorafenib in refractory advanced biliary tract cancer. Cancer 2020;126:3464-70. [Crossref] [PubMed]
- Meyerhardt JA, Ancukiewicz M, Abrams TA, et al. Phase I study of cetuximab, irinotecan, and vandetanib (ZD6474) as therapy for patients with previously treated metastastic colorectal cancer. PLoS One 2012;7:e38231. [Crossref] [PubMed]
- Heath E, Heilbrun L, Mannuel H, et al. Phase II, Multicenter, Randomized Trial of Docetaxel plus Prednisone with or Without Cediranib in Men with Chemotherapy-Naive Metastatic Castrate-Resistant Prostate Cancer. Oncologist 2019;24:1149-e807. [Crossref] [PubMed]
- Puchalski T, Prabhakar U, Jiao Q, et al. Pharmacokinetic and pharmacodynamic modeling of an anti-interleukin-6 chimeric monoclonal antibody (siltuximab) in patients with metastatic renal cell carcinoma. Clin Cancer Res 2010;16:1652-61. [Crossref] [PubMed]
- Haldar R, Shaashua L, Lavon H, et al. Perioperative inhibition of β-adrenergic and COX2 signaling in a clinical trial in breast cancer patients improves tumor Ki-67 expression, serum cytokine levels, and PBMCs transcriptome. Brain Behav Immun 2018;73:294-309. [Crossref] [PubMed]
- Johnson DE, O'Keefe RA, Grandis JR. Targeting the IL-6/JAK/STAT3 signalling axis in cancer. Nat Rev Clin Oncol 2018;15:234-48. [Crossref] [PubMed]
- Schreiber S, Aden K, Bernardes JP, et al. Therapeutic Interleukin-6 Trans-signaling Inhibition by Olamkicept (sgp130Fc) in Patients With Active Inflammatory Bowel Disease. Gastroenterology 2021;160:2354-2366.e11. [Crossref] [PubMed]
- Jones SA, Jenkins BJ. Recent insights into targeting the IL-6 cytokine family in inflammatory diseases and cancer. Nat Rev Immunol 2018;18:773-89. [Crossref] [PubMed]
- Falk E. Pathogenesis of atherosclerosis. J Am Coll Cardiol 2006;47:C7-12. [Crossref] [PubMed]
- Song P, Fang Z, Wang H, et al. Global and regional prevalence, burden, and risk factors for carotid atherosclerosis: a systematic review, meta-analysis, and modelling study. Lancet Glob Health 2020;8:e721-9. [Crossref] [PubMed]
- Gerosa C, Faa G, Fanni D, et al. Fetal programming of COVID-19: may the barker hypothesis explain the susceptibility of a subset of young adults to develop severe disease? Eur Rev Med Pharmacol Sci 2021;25:5876-84. [PubMed]
- Muscogiuri G, Guglielmo M, Serra A, et al. Multimodality Imaging in Ischemic Chronic Cardiomyopathy. J Imaging 2022;8:35. [Crossref] [PubMed]
- Muscogiuri G, Volpato V, Cau R, et al. Application of AI in cardiovascular multimodality imaging. Heliyon 2022;8:e10872. [Crossref] [PubMed]
- Didion SP. Cellular and Oxidative Mechanisms Associated with Interleukin-6 Signaling in the Vasculature. Int J Mol Sci 2017;18:2563. [Crossref] [PubMed]
- Ross R. Atherosclerosis--an inflammatory disease. N Engl J Med 1999;340:115-26. [Crossref] [PubMed]
- Botts SR, Fish JE, Howe KL. Dysfunctional Vascular Endothelium as a Driver of Atherosclerosis: Emerging Insights Into Pathogenesis and Treatment. Front Pharmacol 2021;12:787541. [Crossref] [PubMed]
- Schuett H, Oestreich R, Waetzig GH, et al. Transsignaling of interleukin-6 crucially contributes to atherosclerosis in mice. Arterioscler Thromb Vasc Biol 2012;32:281-90. [Crossref] [PubMed]
- Huber SA, Sakkinen P, Conze D, et al. Interleukin-6 exacerbates early atherosclerosis in mice. Arterioscler Thromb Vasc Biol 1999;19:2364-7. [Crossref] [PubMed]
- Kamtchum-Tatuene J, Saba L, Heldner MR, et al. Interleukin-6 Predicts Carotid Plaque Severity, Vulnerability, and Progression. Circ Res 2022;131:e22-33. [Crossref] [PubMed]
- Okazaki S, Sakaguchi M, Miwa K, et al. Association of interleukin-6 with the progression of carotid atherosclerosis: a 9-year follow-up study. Stroke 2014;45:2924-9. [Crossref] [PubMed]
- Mossmann M, Wainstein MV, Mariani S, et al. Increased serum IL-6 is predictive of long-term cardiovascular events in high-risk patients submitted to coronary angiography: an observational study. Diabetol Metab Syndr 2022;14:125. [Crossref] [PubMed]
- Roy N, Rosas SE. IL-6 Is Associated with Progression of Coronary Artery Calcification and Mortality in Incident Dialysis Patients. Am J Nephrol 2021;52:745-52. [Crossref] [PubMed]
- López-Mejías R, González-Gay MA. IL-6: linking chronic inflammation and vascular calcification. Nat Rev Rheumatol 2019;15:457-9. [Crossref] [PubMed]
- Saba L, Chen H, Cau R, et al. Impact Analysis of Different CT Configurations of Carotid Artery Plaque Calcifications on Cerebrovascular Events. AJNR Am J Neuroradiol 2022;43:272-9. [Crossref] [PubMed]
- Cau R, Gupta A, Kooi ME, et al. Pearls and Pitfalls of Carotid Artery Imaging: Ultrasound, Computed Tomography Angiography, and MR Imaging. Radiol Clin North Am 2023;61:405-13. [Crossref] [PubMed]
- Saba L, Cau R, Murgia A, et al. Carotid Plaque-RADS: A Novel Stroke Risk Classification System. JACC Cardiovasc Imaging 2024;17:62-75. [Crossref] [PubMed]
- Cau R, Saba L, Balestrieri A, et al. Photon-Counting Computed Tomography in Atherosclerotic Plaque Characterization. Diagnostics (Basel) 2024;14:1065. [Crossref] [PubMed]
- Cademartiri F, Meloni A, Pistoia L, et al. Dual-Source Photon-Counting Computed Tomography-Part I: Clinical Overview of Cardiac CT and Coronary CT Angiography Applications. J Clin Med 2023;12:3627. [Crossref] [PubMed]
- Ridker PM, Everett BM, Thuren T, et al. Antiinflammatory Therapy with Canakinumab for Atherosclerotic Disease. N Engl J Med 2017;377:1119-31. [Crossref] [PubMed]
- Ridker PM, Libby P, MacFadyen JG, et al. Modulation of the interleukin-6 signalling pathway and incidence rates of atherosclerotic events and all-cause mortality: analyses from the Canakinumab Anti-Inflammatory Thrombosis Outcomes Study (CANTOS). Eur Heart J 2018;39:3499-507. [Crossref] [PubMed]
- Ridker PM, Rane M. Interleukin-6 Signaling and Anti-Interleukin-6 Therapeutics in Cardiovascular Disease. Circ Res 2021;128:1728-46. [Crossref] [PubMed]
- Libby P, Kobold S. Inflammation: a common contributor to cancer, aging, and cardiovascular diseases-expanding the concept of cardio-oncology. Cardiovasc Res 2019;115:824-9. [Crossref] [PubMed]
- Gallucci G, Turazza FM, Inno A, et al. Atherosclerosis and the Bidirectional Relationship between Cancer and Cardiovascular Disease: From Bench to Bedside-Part 1. Int J Mol Sci 2024;25:4232. [Crossref] [PubMed]
- Nettersheim FS, Picard FSR, Hoyer FF, et al. Immunotherapeutic Strategies in Cancer and Atherosclerosis-Two Sides of the Same Coin. Front Cardiovasc Med 2022;8:812702. [Crossref] [PubMed]
- Yang V, Kragstrup TW, McMaster C, et al. Managing Cardiovascular and Cancer Risk Associated with JAK Inhibitors. Drug Saf 2023;46:1049-71. [Crossref] [PubMed]
- Gopinathan G, Milagre C, Pearce OM, et al. Interleukin-6 Stimulates Defective Angiogenesis. Cancer Res 2015;75:3098-107. [Crossref] [PubMed]
- Cau R, Anzalone N, Mannelli L, et al. Pericarotid Fat as a Marker of Cerebrovascular Risk. AJNR Am J Neuroradiol 2024;45:1635-41. [Crossref] [PubMed]
- Ross JS, Stagliano NE, Donovan MJ, et al. Atherosclerosis: a cancer of the blood vessels? Am J Clin Pathol 2001;116:S97-107. [PubMed]
- Vidal-Vanaclocha F. Inflammation in the molecular pathogenesis of cancer and atherosclerosis. Reumatol Clin 2009;5:40-3. [Crossref] [PubMed]
- Morganti M, Carpi A, Nicolini A, et al. Atherosclerosis and cancer: common pathways on the vascular endothelium. Biomed Pharmacother 2002;56:317-24. [Crossref] [PubMed]
- Jaiswal S, Natarajan P, Silver AJ, et al. Clonal Hematopoiesis and Risk of Atherosclerotic Cardiovascular Disease. N Engl J Med 2017;377:111-21. [Crossref] [PubMed]
- Nouri B, Nair N, Barton A. Predicting treatment response to IL6R blockers in rheumatoid arthritis. Rheumatology (Oxford) 2020;59:3603-10. [Crossref] [PubMed]
- Liu C, Yang L, Xu H, et al. Systematic analysis of IL-6 as a predictive biomarker and desensitizer of immunotherapy responses in patients with non-small cell lung cancer. BMC Med 2022;20:187. [Crossref] [PubMed]
- Dimosiari A, Patoulias D, Kitas GD, et al. Do Interleukin-1 and Interleukin-6 Antagonists Hold Any Place in the Treatment of Atherosclerotic Cardiovascular Disease and Related Co-Morbidities? An Overview of Available Clinical Evidence. J Clin Med 2023;12:1302. [Crossref] [PubMed]
- Burmester GR. Interleukin 6 inhibitors: Biology, principles of use, and adverse effects. UpToDate.