Greater than the sum of its parts: multimodality imaging in adults with congenital heart disease
Introduction
As outcomes for children born with congenital heart disease have improved, the number of adults with congenital heart disease (ACHD) has continued to rise. In the past decade, the number of ACHD has surpassed the number of children (1). As such, the natural history of this population is being revealed in real time. Surveilling the course of residual disease requires continued and close study within our field. In this unique population, merely applying lessons learned from other populations, such as those with acquired adult cardiac disease, is an unacceptable solution, and one that would result in a mishandling of clinical data and improperly formed conclusions.
Indeed, a necessary goal in caring for the ACHD population is to identify signals of clinical deterioration and interventions that can minimize morbidity and mortality. Here we review key examples and lessons learned from imaging biomarkers in multimodal imaging, which has seen tremendous growth in the past two decades (2). The 6th special issue on “Current Management Aspects of Adult Congenital Heart Disease (ACHD)” arrives at a pivotal moment, underscoring the indispensable role of multimodality imaging in the comprehensive care of ACHD patients.
Multimodality imaging refers to the integration of various imaging techniques—such as echocardiography, magnetic resonance imaging (MRI), and computed tomography (CT)—to provide a detailed and holistic view of cardiac anatomy and function. Each modality offers unique strengths and, when used synergistically, they enhance diagnostic accuracy, guide therapeutic interventions, and improve long-term management strategies. Following a brief review of these imaging modalities, key examples and practical considerations will be reviewed.
The role of echocardiography
Echocardiography is the cornerstone of ACHD imaging, primarily due to its accessibility, cost-effectiveness, and ability to provide real-time functional and anatomical information. Transthoracic echocardiography (TTE) is particularly useful for routine follow-ups, offering critical insights into ventricular function, valvular abnormalities, and hemodynamic parameters. Its value as a screening test, though it is limited by poor acoustic windows in some patients, operator dependency, less detailed spatial resolution compared to MRI and CT. Often, cross-sectional imaging is needed to further refine and quantify abnormalities suspected on echocardiography.
Advancements in cardiac MRI (CMR)
CMR has emerged as a gold standard for detailed anatomical and functional assessment in ACHD. Its ability to provide high-resolution images without ionizing radiation makes it ideal for serial evaluations and lifelong monitoring of ACHD patients. CMR is particularly valuable in quantifying ventricular volumes and vascular flow, and myocardial tissue characterization offering comprehensive insights that are critical for pre-surgical planning and post-surgical follow-up. Stress CMR can detect perfusion differences in populations such as those with sequelae of Kawasaki disease, anomalous origin of the coronary arteries, or surgeries requiring coronary translocation. Four-dimensional (4D) flow is an advanced CMR sequence where blood flow is mapped in three orthogonal directions to produce a three-dimensional (3D) flow vector at each voxel (vector field) throughout the cardiac cycle (time is the 4th dimension). Flow can be measured post-hoc in any plane rather than limiting flow analysis to pre-defined planes designated during the MRI scan. Additionally, flowlines can be assessed for qualitative or quantitative assessment of nonlaminar and stenotic areas. This is particularly useful in the complex flow patterns, flow restriction, and energy loss seen in anatomies such as in a Fontan conduit or a systemic venous baffle (3-6).
The precision of cardiac CT (CCT)
CCT, with its excellent spatial resolution, plays a vital role in the anatomical delineation of complex congenital anomalies, valvar structure and coronary artery anatomy, and pre-interventional planning. Technological advancements have significantly reduced radiation exposure, making it a more viable option for repeated imaging when necessary. Likewise, significant metallic hardware compromises CMR image quality, requiring CCT for anatomic and functional assessment. Additionally, the superior spatial resolution of CCT is ideal for virtual and 3D model creation (7).
Nuclear imaging
Nuclear imaging techniques, including single-photon emission CT (SPECT) and positron emission tomography (PET), provide functional information that complements anatomical imaging. These modalities are particularly useful in assessing myocardial perfusion and viability, but also the detection of infective endocarditis (8).
Cardiac catheterization and angiography
Invasive catheterization-based angiography, once the primary tool for cardiac imaging, remains a vital tool in specific scenarios in ACHD, particularly in cases where a hemodynamic assessment and interventional procedure are concurrently needed. The spatial and temporal resolution of invasive angiography surpasses that of even CCT (spatial resolution 0.16 mm; temporal resolution 1–10 ms) and provides imaging of an isolated vessel segment within the limits of orthographic projection (9).
Intracardiac echocardiography (ICE)
ICE is used in specific interventional settings for ACHD. ICE offers real-time, high-resolution images of intracardiac structures from within the heart, providing superior guidance during catheter-based interventions. It allows for precise visualization of device placements, assessment of valve function, and detection of complications during and after procedures. Also, it can be used for detection of endocarditis when transesophageal echo is inadequate or contraindicated (10).
Select cardiac defects
Tetralogy of Fallot (TOF)
CMR is the standard of care for quantification of right ventricular size and function in patients with TOF, with current guidelines recommending serial CMR every 3 years in stable patients (11). Bokma et al. are the first to show a mortality benefit in pulmonary valve replacement, particularly in patients who underwent proactive pulmonary valve replacement guided by CMR volume and function data (12). A combination of CMR and CT provides complementary data in preparation for transcatheter pulmonary valve replacement (13). CMR provides functional information, flow data, and prognostic value as to the long-term benefit of valve replacement. For example, higher burden of right ventricular late gadolinium enhancement prior to pulmonary valve replacement imparts a higher risk of deleterious post-valve replacement volume changes (14). Whereas 4D CT (full cardiac cycle CT images) provides detailed anatomy of the landing zone within the right ventricular outflow tract (15) as well as anatomic detail of the coronary arteries to assess the risk of coronary artery compression, as shown in the example in Figure 1 (13).
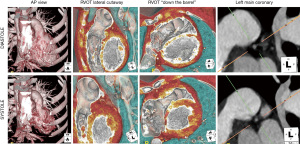
Fontan circulation
A growing population of patients with Fontan physiology require meticulous follow-up to monitor the function of their unique circulatory system. In addition to an annual echocardiogram, CMR every 2–3 years is recommended to provide detailed assessment of the Fontan pathway for obstructions and baffle leaks, ventricular volumes, and function (16). In the past decade, there has been increased interest in noninvasive assessment of collateral burden by CMR (17), with particular attention toward short-term morbidity and mortality relative to high collateral burden. As long-term data of this analysis accumulates in the ACHD Fontan population, it is anticipated that prognostic data may be drawn from this imaging biomarker. Alongside the circulatory system, lymphatic imaging has been an area of investigation and imparts prognostic information (18). More complex flow data with 4D flow energetics have found to correlate with exercise capacity (5). Figure 2 demonstrates the variety of Fontan circuit morphologies that can be seen with CMR.
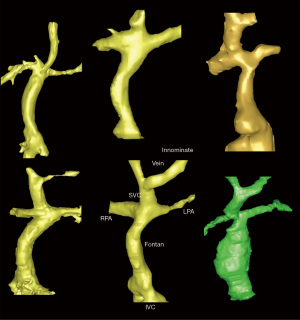
Aortic coarctation
The thoracic anatomy of ACHD patients imparts limited echocardiographic windows, obliging cross-sectional imaging for detailed anatomic assessment. CMR angiography and CCT are both useful for monitoring the aorta for aneurysm formation and re-coarctation, with the latter providing better spatial resolution. In addition to an anatomic assessment at rest, the dynamic changes that occur at increased cardiac output provide a better understanding of the hemodynamic effect of residual coarctation on a patient’s daily activities. Stress CMR of the aortic arch flow individually assesses a patient’s hemodynamic response to exercise that is nearly physiologic (19-21). CMR with 4D flow is being investigated to noninvasively detect difference in flow geometry, wall stress, and pressure gradient to predict which patients may benefit from intervention (21-23).
Systemic right ventricle (RV)
For patients with a systemic RV, as seen in conditions like congenitally corrected transposition of the great arteries (ccTGA) or after atrial switch procedures for D-transposition of the great arteries (D-TGA), CMR provides reliable quantification of ventricular size and function (Figure 3A), as well as myocardial fibrosis, which provides prognostic information to the care team. An indexed RV end-diastolic volume of ≥130 vs. <130 mL/m2 imparts a 10-year survival of 70% vs. 100% in atrial switch patients and 62% vs. 100% (24). In patients with ccTGA, repair or replacement of a regurgitant systemic atrioventricular (AV) valve before the right ventricular ejection fraction falls below 40% better preserves systemic RV function at 1-year post-repair (25). Additionally, systemic RV function by MRI can inform the timing of cardiac resynchronization therapy in ccTGA patients with heart failure (26). Both CMR and CT can be used for the assessment of venous baffles (Figure 3B,3C) (27).
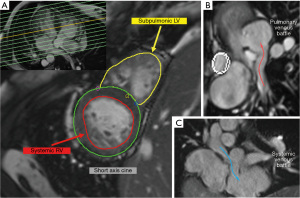
Transposition of the great arteries (TGA) with arterial switch operation
In patients with D-TGA following the arterial switch operation, regular surveillance with echocardiography provides information on ventricular function and valvar regurgitation. Following the Lecompte maneuver, echocardiographic imaging of the pulmonary arteries is limited and CMR is shown to have increased sensitivity for the detection of focal stenoses (28). Likewise, the neo-aortic root is prone to dilation resulting in neo-aortic regurgitation over time (29). In additional to the assessment of pulmonary arteries and the neo-aortic root, CMR can be used to assess re-implanted coronary arteries (see Figure 4) and perfusion defects relating to coronary anomalies in select individuals (30). CCT can be used for higher resolution anatomic evaluation of the coronary arteries, neo-aortic root, and pulmonary arteries.
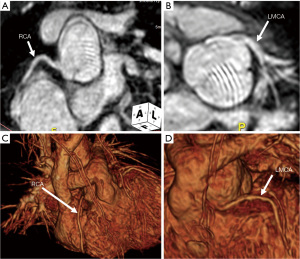
Sinus venosus defect
Sinus venosus defects require detailed imaging to evaluate the anomalous pulmonary venous connections. Echocardiography provides qualitative assessment of right heart size and function but is inadequate to fully map the venous system. CCT and CMR provide precise anatomical details needed for surgical planning, with CMR providing the additional advantage of direct measurement of the shunt fraction [pulmonary-to-systemic flow (Qp:Qs) ratio] (31). In poor surgical candidates for open heart repair of superior sinus venosus repair, transcatheter-covered stent closure is an alternative method (32). This innovative approach requires precise anatomic mapping and often 3D printing or virtual reality examination of the atrial and venous anatomy for pre-procedural planning (33,34). Figure 5 shows an example of a CT and surface-rendered 3D model used for pre-procedural planning.
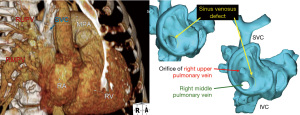
The future of multimodal imaging
Though the ACHD population is growing, the population size is modest relative to adult patients with acquired heart disease. Multicenter registries hold promise to drive research forward in a complex and diverse population (35). As such, attention needs to be paid to the inclusion of imaging variables in multicenter registries. The inclusion of more granular imaging variables with consistent definitions into large-scale databases will make the detection of potent imaging biomarkers more realizable.
Each of the modalities discussed is reliant on operator experience and comfortability of technologists, sonographers, post-processors, and clinicians. A critical mass of ACHD pathology is needed at a given center to produce expertise over time. Advanced education and institutional partnerships may help raise the level of performance by concentrating care at more competitive centers (36).
Computational fluid dynamics (CFD) is a mathematical technique to quantify and characterize fluid flow though anatomy using sophisticated software. CFD has been used to simulate blood flow through patient-specific 3D virtual models predominantly on a research basis (37-39), though viable commercial systems have emerged in recent years. For example, CT fractional flow reserve is an established method of simulating coronary flow using CFD an adult coronary artery disease (40). This highlights the potential for practical clinical tools for assessing patient-specific blood flow in the ACHD population (7). For example, CFD has been used to simulate the blood flow in complex repairs such as in Fontan palliation, identifying modifiable areas of inefficient flow that may result in exercise differences (41,42).
Extended reality (XR; encompassing virtual, augmented, and mixed reality) is a method to transform real-world imaging data into a “digital twin” model for interaction in a virtual environment. XR has been shown to improve surgical planning in patient undergoing workup for complex congenital heart disease repairs by creating a more intuitive experience for operators interacting with imaging data, as shown in Figure 6 (43-47). Additionally, XR has been shown to aid in patient understanding of their own anatomy, particularly in patients transitioning from pediatric to adult congenital cardiology care (48). Lastly, artificial intelligence (AI) in imaging is a central area of interest and research in radiology and cardiology, using sophisticated computer models trained on large datasets to perform tasks at or above human capacity (7). AI technology has been deployed into MRI scanners to enable more rapid imaging and post-processing software for automated image segmentation with a primary benefit of reducing analysis burden (49). AI models in adult cardiology have been created and successfully tested on multiple publicly available datasets of non-congenital heart disease (CHD) patients containing large numbers of scans, whereas the only publicly available MR dataset for CHD patients contains less than 100 subjects (50). As AI products are integrated into clinical workflows, special attention should be paid toward the false negative and positive disease detection seen in this population that may not be meaningfully represented in the training data.
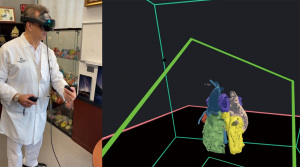
Conclusions
Multimodality imaging in ACHD is an imperative toolkit for patient management, offering a multifaceted view not attainable from single imaging techniques. A multimodal imaging approach provides information into the underlying physiology that, when synthesized by the cardiologist provides value that exceeds the sum of each constituent modality. An understanding of the advantages and disadvantages of each modality is needed to guide a diagnostic approach for each patient. As we advance into an era of personalized medicine, the integration of these imaging modalities will be pivotal in optimizing the care and outcomes of ACHD patients.
Acknowledgments
Funding: None.
Footnote
Provenance and Peer Review: This article was commissioned by the Guest Editor (Harald Kaemmerer) for the series “Current Management Aspects of Adult Congenital Heart Disease (ACHD): Part VI Trial” published in Cardiovascular Diagnosis and Therapy. The article has undergone external peer review.
Peer Review File: Available at https://cdt.amegroups.com/article/view/10.21037/cdt-24-363/prf
Conflicts of Interest: The author has completed the ICMJE uniform disclosure form (available at https://cdt.amegroups.com/article/view/10.21037/cdt-24-363/coif). The series “Current Management Aspects of Adult Congenital Heart Disease (ACHD): Part VI” was commissioned by the editorial office without any funding or sponsorship. The author has no other conflicts of interest to declare.
Ethical Statement: The author is accountable for all aspects of the work in ensuring that questions related to the accuracy or integrity of any part of the work are appropriately investigated and resolved.
Open Access Statement: This is an Open Access article distributed in accordance with the Creative Commons Attribution-NonCommercial-NoDerivs 4.0 International License (CC BY-NC-ND 4.0), which permits the non-commercial replication and distribution of the article with the strict proviso that no changes or edits are made and the original work is properly cited (including links to both the formal publication through the relevant DOI and the license). See: https://creativecommons.org/licenses/by-nc-nd/4.0/.
References
- Stout KK, Daniels CJ, Aboulhosn JA, et al. 2018 AHA/ACC Guideline for the Management of Adults With Congenital Heart Disease: A Report of the American College of Cardiology/American Heart Association Task Force on Clinical Practice Guidelines. Circulation 2019;139:e698-800. [PubMed]
- Patel MR, Spertus JA, Brindis RG, et al. ACCF proposed method for evaluating the appropriateness of cardiovascular imaging. J Am Coll Cardiol 2005;46:1606-13. [Crossref] [PubMed]
- van der Palen RL, Westenberg JJ, Hazekamp MG, et al. Four-dimensional flow cardiovascular magnetic resonance for the evaluation of the atrial baffle after Mustard repair. Eur Heart J Cardiovasc Imaging 2016;17:353. [Crossref] [PubMed]
- Rizk J. 4D flow MRI applications in congenital heart disease. Eur Radiol 2021;31:1160-74. [Crossref] [PubMed]
- Rijnberg FM, Westenberg JJM, van Assen HC, et al. 4D flow cardiovascular magnetic resonance derived energetics in the Fontan circulation correlate with exercise capacity and CMR-derived liver fibrosis/congestion. J Cardiovasc Magn Reson 2022;24:21. [Crossref] [PubMed]
- Ait Ali L, Martini N, Listo E, et al. Impact of 4D-Flow CMR Parameters on Functional Evaluation of Fontan Circulation. Pediatr Cardiol 2024;45:998-1006. [Crossref] [PubMed]
- Chessa M, Van De Bruaene A, Farooqi K, et al. Three-dimensional printing, holograms, computational modelling, and artificial intelligence for adult congenital heart disease care: an exciting future. Eur Heart J 2022;43:2672-84. [PubMed]
- Walter C, Zuccarino F, Carretero Bellón JM. PET/CT role in the diagnosis of infective endocarditis in patients with congenital heart disease. An Pediatr (Engl Ed) 2022;96:260-3.
- Lin E, Alessio A. What are the basic concepts of temporal, contrast, and spatial resolution in cardiac CT? J Cardiovasc Comput Tomogr 2009;3:403-8. [Crossref] [PubMed]
- Sanchez-Nadales A, Cedeño J, Sonnino A, et al. Utility of Intracardiac Echocardiography for Infective Endocarditis and Cardiovascular Device-Related Endocarditis: A Contemporary Systematic Review. Curr Probl Cardiol 2023;48:101791. [Crossref] [PubMed]
- Sánchez Ramírez CJ, Pérez de Isla L. Tetralogy of Fallot: cardiac imaging evaluation. Ann Transl Med 2020;8:966. [Crossref] [PubMed]
- Bokma JP, Geva T, Sleeper LA, et al. Improved Outcomes After Pulmonary Valve Replacement in Repaired Tetralogy of Fallot. J Am Coll Cardiol 2023;81:2075-85. [Crossref] [PubMed]
- Canan A, Ocazionez-Trujillo D, Vargas D, et al. Pre- and Postprocedure Imaging of Transcatheter Pulmonary Valve Implantation. Radiographics 2022;42:991-1011. [Crossref] [PubMed]
- Monti CB, Zanardo M, Capra D, et al. The predictive role of right ventricular late gadolinium enhancement in patients with tetralogy of Fallot undergoing pulmonary valve replacement. Eur Radiol Exp 2023;7:9. [Crossref] [PubMed]
- Hendriks BMF, Martens B, Mihl C. Pre-procedural computed tomography in transcatheter pulmonary valve replacement: The first steps towards standardization of image quality. Int J Cardiol Heart Vasc 2020;29:100542. [Crossref] [PubMed]
- Rychik J, Atz AM, Celermajer DS, et al. Evaluation and Management of the Child and Adult With Fontan Circulation: A Scientific Statement From the American Heart Association. Circulation 2019;140:e234-84. [Crossref] [PubMed]
- Glatz AC, Rome JJ, Small AJ, et al. Systemic-to-pulmonary collateral flow, as measured by cardiac magnetic resonance imaging, is associated with acute post-Fontan clinical outcomes. Circ Cardiovasc Imaging 2012;5:218-25. [Crossref] [PubMed]
- Castellanos DA, Ahmad S, St Clair N, et al. Magnetic resonance three-dimensional steady-state free precession imaging of the thoracic duct in patients with Fontan circulation and its relationship to outcomes. J Cardiovasc Magn Reson 2023;25:28. [Crossref] [PubMed]
- Mandell JG, Loke YH, Mass PN, et al. Altered hemodynamics by 4D flow cardiovascular magnetic resonance predict exercise intolerance in repaired coarctation of the aorta: an in vitro study. J Cardiovasc Magn Reson 2021;23:99. [Crossref] [PubMed]
- Schubert C, Brüning J, Goubergrits L, et al. Assessment of hemodynamic responses to exercise in aortic coarctation using MRI-ergometry in combination with computational fluid dynamics. Sci Rep 2020;10:18894. [Crossref] [PubMed]
- Frydrychowicz A, Markl M, Hirtler D, et al. Aortic hemodynamics in patients with and without repair of aortic coarctation: in vivo analysis by 4D flow-sensitive magnetic resonance imaging. Invest Radiol 2011;46:317-25. [Crossref] [PubMed]
- van Ooij P, Farag ES, Blanken CPS, et al. Fully quantitative mapping of abnormal aortic velocity and wall shear stress direction in patients with bicuspid aortic valves and repaired coarctation using 4D flow cardiovascular magnetic resonance. J Cardiovasc Magn Reson 2021;23:9. [Crossref] [PubMed]
- Yurpolskaya LA, Shlyappo MA, Makarenko VN, et al. 4D FLOW Magnetic Resonance Imaging in the Study of Blood Flow in Patients With Aortic Coarctation in the Long-Term After Surgery. Kardiologiia 2020;60:54-64. [Crossref] [PubMed]
- Lewis MJ, Van Dissel A, Kochav J, et al. Cardiac MRI predictors of adverse outcomes in adults with a systemic right ventricle. ESC Heart Fail 2022;9:834-41. [Crossref] [PubMed]
- Mongeon FP, Connolly HM, Dearani JA, et al. Congenitally corrected transposition of the great arteries ventricular function at the time of systemic atrioventricular valve replacement predicts long-term ventricular function. J Am Coll Cardiol 2011;57:2008-17. [Crossref] [PubMed]
- Bevilacqua F, Pasqualin G, Ferrero P, et al. Overview of Long-Term Outcome in Adults with Systemic Right Ventricle and Transposition of the Great Arteries: A Review. Diagnostics (Basel) 2023;13:2205. [Crossref] [PubMed]
- Arthur L, Schoeneberg L, Angtuaco M, et al. Advanced imaging improves detection of baffle leaks and stenoses after atrial switch compared with transthoracic echocardiography. Int J Cardiovasc Imaging 2021;37:2767-72. [Crossref] [PubMed]
- Blakenberg F, Rhee J, Hardy C, et al. MRI vs echocardiography in the evaluation of the Jatene procedure. J Comput Assist Tomogr 1994;18:749-54. [Crossref] [PubMed]
- Michalak KW, Sobczak-Budlewska K, Moll JJ, et al. Neoaortic Regurgitation in Patients with Transposition Long Term After an Arterial Switch Operation and Its Relation to the Root Diameters and Surgical Technique Used. Pediatr Cardiol 2020;41:31-7. [Crossref] [PubMed]
- Tobler D, Motwani M, Wald RM, et al. Evaluation of a comprehensive cardiovascular magnetic resonance protocol in young adults late after the arterial switch operation for d-transposition of the great arteries. J Cardiovasc Magn Reson 2014;16:98. [Crossref] [PubMed]
- Celona A, Caruso E, Farruggio S, et al. Anomalous venoatrial connections - CT and MRI assessment. Heliyon 2023;9:e18462. [Crossref] [PubMed]
- Baruteau AE, Hascoet S, Malekzadeh-Milani S, et al. Transcatheter Closure of Superior Sinus Venosus Defects. JACC Cardiovasc Interv 2023;16:2587-99. [Crossref] [PubMed]
- Sivakumar K, Sagar P, Thejaswi P. Virtual Reality Software Aids Precise Transcatheter Closure of Sinus Venosus Defect Using Covered Stent Without 3D Printing. Pediatr Cardiol 2024;45:939-41. [Crossref] [PubMed]
- Thakkar AN, Chinnadurai P, Breinholt JP, et al. Transcatheter closure of a sinus venosus atrial septal defect using 3D printing and image fusion guidance. Catheter Cardiovasc Interv 2018;92:353-7. [Crossref] [PubMed]
- Alsaied T, Allen KY, Anderson JB, et al. The Fontan outcomes network: first steps towards building a lifespan registry for individuals with Fontan circulation in the United States - CORRIGENDUM. Cardiol Young 2020;30:1381. [Crossref] [PubMed]
- Johnson AJ, McGrath LB, Khan AM, et al. Hospital Care for Adult Patients with Congenital Heart Diseases. Heart Surg Forum 2023;26:E842-54. [Crossref] [PubMed]
- Rigatelli G, Chiastra C, Pennati G, et al. Applications of computational fluid dynamics to congenital heart diseases: a practical review for cardiovascular professionals. Expert Rev Cardiovasc Ther 2021;19:907-16. [Crossref] [PubMed]
- Gerrah R, Haller SJ. Computational fluid dynamics: a primer for congenital heart disease clinicians. Asian Cardiovasc Thorac Ann 2020;28:520-32. [Crossref] [PubMed]
- Pennati G, Corsini C, Hsia TY, et al. Computational fluid dynamics models and congenital heart diseases. Front Pediatr 2013;1:4. [Crossref] [PubMed]
- Rajiah P, Cummings KW, Williamson E, et al. CT Fractional Flow Reserve: A Practical Guide to Application, Interpretation, and Problem Solving. Radiographics 2022;42:340-58. [Crossref] [PubMed]
- Bossers SS, Cibis M, Gijsen FJ, et al. Computational fluid dynamics in Fontan patients to evaluate power loss during simulated exercise. Heart 2014;100:696-701. [Crossref] [PubMed]
- Whitehead KK, Pekkan K, Kitajima HD, et al. Nonlinear power loss during exercise in single-ventricle patients after the Fontan: insights from computational fluid dynamics. Circulation 2007;116:I165-71. [Crossref] [PubMed]
- Priya S, La Russa D, Walling A, et al. From Vision to Reality: Virtual Reality's Impact on Baffle Planning in Congenital Heart Disease Pediatr Cardiol 2024;45:165-74. [Crossref] [PubMed]
- Galeczka M, Smerdzinski S, Tyc F, et al. Virtual reality for transcatheter procedure planning in congenital heart disease. Kardiol Pol 2023;81:1026-7. [Crossref] [PubMed]
- Krasemann T, Branstetter J. Virtual Reality Treatment Planning for Congenital Heart Disease. JACC Case Rep 2021;3:1584-5. [Crossref] [PubMed]
- Kim B, Loke YH, Mass P, et al. A Novel Virtual Reality Medical Image Display System for Group Discussions of Congenital Heart Disease: Development and Usability Testing. JMIR Cardio 2020;4:e20633. [Crossref] [PubMed]
- Goo HW, Park SJ, Yoo SJ. Advanced Medical Use of Three-Dimensional Imaging in Congenital Heart Disease: Augmented Reality, Mixed Reality, Virtual Reality, and Three-Dimensional Printing. Korean J Radiol 2020;21:133-45. [Crossref] [PubMed]
- Kieu V, Sumski C, Cohen S, et al. The Use of Virtual Reality Learning on Transition Education in Adolescents with Congenital Heart Disease. Pediatr Cardiol 2023;44:1856-60. [Crossref] [PubMed]
- Morales MA, Manning WJ, Nezafat R. Present and Future Innovations in AI and Cardiac MRI. Radiology 2024;310:e231269. [Crossref] [PubMed]
- Pace DF, Contreras HTM, Romanowicz J, et al. HVSMR-2.0: A 3D cardiovascular MR dataset for whole-heart segmentation in congenital heart disease. Sci Data 2024;11:721. [Crossref] [PubMed]