LncRNA-5829: a novel inhibitor of cardiac fibrosis
Highlight box
Key findings
• In this study, lncRNA-5829 was downregulated in the myocardial fibrosis model, confirming that lncRNA-5829 plays an important regulatory role in the pathological process of myocardial fibrosis.
What is known and what is new?
• LncRNA is involved in regulating the progression of various cardiovascular diseases, but its role in myocardial fibrosis has not yet been fully elucidated.
• This study discovered a new lncRNA with anti-myocardial fibrosis effects, lncRNA5829.
What is the implication, and what should change now?
• We have confirmed the protective role of lncRNA5829 in myocardial fibrosis, providing a new potential drug target for myocardial fibrosis.
Introduction
Cardiovascular diseases (CVDs) are the leading cause of morbidity and mortality worldwide (1,2), thus there is a need for a deeper understanding of their underlying pathogenic mechanisms. Myocardial fibrosis encompasses the diverse alterations, both quantitative and qualitative, in the collagenous framework of the myocardium in response to ischemia, pharmacological agents, or other deleterious factors that adversely impact the cardiovascular system or the heart directly (3). Multiple heart diseases such as ischemic heart disease, hypertrophy and heart failure are accompanied by myocardial fibrosis, which is one of the crucial pathological manifestations in the heart (4). At present, the prevention and cure of myocardial fibrosis have become the main treatment for many kinds of heart disease. Due to the wide range of sources of fibroblasts and the lack of specific markers, the research on the molecular mechanism and regulatory network of myocardial fibrosis is not clear, and there is a lack of effective strategies on its treatment. This problem has become the focus of medical attention and research. Therefore, finding a new regulatory factor and elucidating the mechanism is new research fields of myocardial fibrosis.
Long non-coding RNAs (lncRNAs) are a class of non-coding RNAs molecules longer than 200 bp, including enhancer RNAs, intergenic transcripts and transcripts that overlap with other transcripts in the same direction or reverse direction (5). Abnormal expression of lncRNAs may affect cell proliferation, tumor progression or metastasis, ranging from integrating chromatin remodeling complex to regulating transcriptional and post-transcriptional processes (6). LncRNAs act as signal molecules, decoy molecules, guide molecules or skeleton molecules to interact with other RNAs or proteins, directly affecting the cell signal cascade (7). A large number of studies have confirmed the feasibility of lncRNAs as a tool for the diagnosis, prognosis and prediction of different types of CVDs including myocardial fibrosis (8-11). Ge et al. confirmed that lncRNANeat1 was up-regulated in myocardial tissue of mice treated with aortic coarctation surgery and in myocardial fibroblasts treated with transforming growth factor-β1 (TGF-β1), and inhibiting the expression of Smad7 by binding with EZH2, promoted the occurrence and development of myocardial fibrosis and exacerbated cardiac function injury (12). Zhang et al. established CFAR as a new profibrotic factor acting through a novel miR-449a-5p/LOXL3/mTOR axis in the heart and therefore might be considered as a potential molecular target for the treatment of cardiac fibrosis and associated heart diseases (13). The study of lncRNAs as a new regulatory factor in CVDs is still in its infancy, and most of the functions of lncRNAs have not been revealed. Recently, a large number of peptides encoded by lncRNAs have been validated, and it is noteworthy that these peptides have been shown to play a significant role in the pathological processes of various diseases (14).
On the basis of these facts, from the Mouse LncRNA Array V3.0 Chip, we found out that there was a difference in the expression of the new lncRNA-5829 in the model of myocardial fibrosis, so we conducted a deep study on whether lncRNA-5829 was involved in the regulation of myocardial fibrosis in order to provide theoretical basis for the clinical diagnosis and treatment of myocardial fibrosis.
In this study, we used ligation of the left anterior descending coronary artery (LAD) surgery to establish a mouse model of myocardial infarction (MI), the LAD ligation surgery is well-established and convenient, with the site of obstruction being clear and easily identifiable, corresponding to the actual pathological process of MI. This makes it suitable for experimental research. Consequently, this method is primarily used to establish a MI model. This model represents patients who have experienced MI without optimal reperfusion, placing them at a higher risk of developing heart failure and myocardial fibrosis. The MI model produced by LAD ligation surgery results in a very large area of MI, making it suitable for understanding the cellular events and molecular pathways involved in the healing and remodeling process of MI. We present this article in accordance with the ARRIVE reporting checklist (available at https://cdt.amegroups.com/article/view/10.21037/cdt-24-462/rc).
Methods
Experimental animals
Thirty-two C57BL/6 (20–25 g) 8-week-old male mice were procured from Liaoning Changsheng Biotechnology Co. The use of these animals has been approved by the Institutional Review Board (IRB) of the College of Pharmacy, Harbin Medical University (approval No. IRB3002620), and complies with the regulations of Harbin Medical University and the National Research Council (NRC) Guide for Care and Use of Laboratory Animals (8th edition). Mice were placed in cages, with four mice per cage, and the temperature was maintained at 22±2 ℃ with a humidity of 55%±5%. A 12-hour light/dark cycle was implemented, and ventilation occurred 10 to 20 times per hour. Non-toxic plastic cages with stainless steel wire lids and metal racks were utilized. Standard laboratory water was provided for drinking and was replaced two to three times per week. A sufficient amount of fresh, dry feed was added to the feeders in the cages, with feed supplementation occurring on two fixed days each week.
Animal model
Mice were anesthetized with avertine (0.2 mL/kg, intraperitoneally injected). Then mice were randomly (There are 32 mice; first, label each mouse with a number from 1 to 32 as its identifier. Next, utilize the Microsoft Excel function RANDBETWEEN() to generate random numbers ranging from 0 to 99. Copy and paste these generated random numbers, then sort them from smallest to largest. Finally, divide the mice into groups of 8 based on the sorted order.) divided into 4 groups (each with 8 mice), including sham group, MI group, adeno-associated virus 9 (AAV9)-lncRNA5829+MI group, and AAV9-negative control (NC) + MI group. The LAD was ligated for 4 weeks to model the myocardial fibrosis induced by MI. The surgery was performed under sterile conditions. MI was marked by S-T segment of lead II. A recombinant serum type 9 adeno-associated virus (rAAV9) system was used to construct lncRNA-5829 gene overexpression vector and NC, which was purchased from Guangzhou Aizhe Biotechnology Co., Ltd. (Guangzhou, China). The titer of rAAV9 was 3*10e11 GC/mL, which was injected into mice by tail vein with an injection volume of about 100–200 µL. Four weeks after injection, MI intervention was carried out. The heart was rapidly isolated 4 weeks after MI. Myocardial specimens near the area of MI were dissected for experimental analysis. The sham-operated group underwent open-heart surgery without blocking the LAD. Different stages of the experiment (allocation cycle, experiment implementation) are handled by different people. Anyone who only passed the labeled digital randomized experiment did not know what kind of treatment each group received. Results were analyzed by mouse ear tag evaluation data.
Cell culture
No exclusion criteria was set during the experiment. Cardiac fibroblasts (CFs) and cardiomyocytes (CMs) were isolated from Kunming neonatal mice aged 1–3 days. The hearts were then excised and subjected to a washing process with DMEM (Hyclone, USA), post which they were cubed into uniform segments. Subsequently, these segments were incubated with 0.25% trypsin (Solarbio, China) for cell digestion. After centrifugation to obtain a cell suspension, the resulting cells were resuspended in DMEM supplemented with 10% fetal bovine serum (FBS, Biological Industries, Israel), 100 U/mL of penicillin, and 100 mg/mL of streptomycin (Solarbio, China). According to the characteristics of different adhesion speed between CFs and CMs, the culture flask was cultured in the incubator for 1 to 1.5 hours and then taken out. At this time, most of the CFs would adhere and grow in the culture flask while the CMs were suspended in the culture medium. The CFs in the culture flask were placed in the incubator overnight. The suspension containing CMs was mixed and inoculated for 48 hours in a 6-well plate.
Cell transfection
The CFs were cultured in serum-free medium for a duration of 4 hours prior to the transfection process. This involved the introduction of various constructs, which included lncRNA-5829 siRNA, siRNA-NC synthesized by RIBOBIO (Guangzhou, China), and a plasmid encoding overexpression of lncRNA-5829, which was synthesized by Aiyou Huagene Biosciences, Guangzhou, China. Lipo2000 (Lipofectamine 2000, Invitrogen, USA) was combined with Opti-MEM I (Invitrogen, USA), a reduced serum medium, for a duration of 5 minutes. Subsequently, these two mixtures were combined and incubated at room temperature for an additional 15 minutes. Thereafter, this mixture was added to the cell culture plate, which was then incubated at a temperature of 37 ℃ with an atmosphere of 5% CO2 for a period of 48 hours to facilitate subsequent experimental procedures.
Mouse lncRNA Array V3.0 Chip
Mouse LncRNA Array V3.0 Chip was produced and analyzed by Aksomics (Shanghai, China). The expression of lncRNAs was detected from the fibrotic tissue of the marginal zone of MI in mice. Total RNA was extracted from the heart tissues of three mice from both the MI group and the control group. The RNA was converted into cDNA using reverse transcriptase, and the cDNA was labeled with fluorescent markers. The labeled cDNA was then hybridized with pre-designed microarray chips (Aksomics). These chips have many different probes, each corresponding to a specific gene or transcript. The chips were scanned with a scanner to obtain the fluorescence signal intensity of each probe, which reflects the expression level of the corresponding gene or transcript in the sample. Data analysis revealed that more than 1200 lncRNAs were found to have differential expressions, in which 602 lncRNAs were up-regulated and 603 lncRNAs were down-regulated (P<0.05, fold change >2 or <0.5).
Masson’s trichrome staining
The mouse hearts were rapidly dissected and subsequently immersed in 4% neutral formalin solution for a duration of 24 hours. Thereafter, they were subjected to Masson’s trichrome staining (manufactured by Solarbio, China). The alterations in myocardial collagen content were examined under a light microscope (Olympus, Tokyo, Japan), with the blue stain indicating the distribution of collagen fibers. The analysis of Masson staining is usually based on the calculation of collagen volume fraction (CVF), which is the percentage of blue area of collagen positive to total tissue area. The CVF was analyzed by Image-Pro Plus 6.0.
Hematoxylin-eosin (HE) staining
Paraffin section dewaxing to water, stain with hematoxylin solution (Solarbio, China) for 2–5 minutes, rinse with distilled water to remove excess dye. Differentiate with differentiation solution (Solarbio, China) for 10–60 seconds, wash with tap water twice, each time for 3–5 minutes. Apply eosin staining solution (Solarbio, China) for 30 seconds to 2 minutes, pour off excess dye and dehydrate rapidly. Dehydrate, clear, and mount: (I) immerse in gradient ethanol [75%, 85%, 95%, and 100% ethanol (i)] for 2–3 seconds each, immerse in 100% ethanol (ii) for 1 minute; (II) clear with xylene twice, each time for 1 minute, mount with neutral resin, observe under the light microscope (Olympus). Under the light microscope, the hematoxylin stain is alkaline, which mainly makes the chromatin in the nucleus and nucleic acid in the cytoplasm purplish blue, while eosin is an acid dye, which mainly makes the components in the cytoplasm and extracellular matrix (ECM) red.
Echocardiography
Four weeks after MI surgery, mice were anesthetized with avetin (0.2 mL/kg, intraperitoneally injected) and subsequently positioned on a testing platform. Their cardiac function was evaluated utilizing transthoracic echocardiography facilitated by a visual ultrasound system (Vevo2100). Changes in the ejection fraction (EF%) and fractional shortening (FS%) were quantitatively measured.
Western blot analysis
Total protein samples were extracted from heart tissue or CFs, and the total protein concentration was measured by BCA Protein Assay Kit (Beyotime, China). The samples were separated by SDS-PAGE at 100 µg tissue (in vivo experiments) or 40 µg cell (in vitro experiments) per well. The protein bands from the gel were transferred to the NC membrane (Millipore, USA), then the membrane was sealed in 5% skim milk (BD Pharmingen, USA) for 1.5 h at room temperature and incubated with the primary antibody at 4 ℃ overnight. The antibodies used were anti-FN1 (1:500, Proteintech, USA), anti-collagen1 (1:1,000, Wanleibio, China), anti-TGF-β1 (1:1,000, Proteintech, USA), anti-β-actin (1:1,000, Proteintech, USA). Then, the membranes were incubated with mouse or rabbit polyclonal secondary antibodies (1:10,000, Abcam, USA) for 1 h. Image Studio Ver 5.2 was used to analyze the gray scale (density) of protein bands.
Quantitative real-time polymerase chain reaction (qRT-PCR)
Total RNA samples were extracted from myocardium or CFs using Trizol reagent (Invitrogen, USA). The obtained RNA samples were reverse transcribed after the concentration was measured. Refer to the High-Capacity cDNA reverse transcription reagent kits manual (Toyobo, Japan). RNA samples were quantified by Nano drop 8000. The expression of RNAs including lncRNA-5829, and fibrotic markers fibronectin 1 (FN1), collagen type I alpha 1 (Col1α1) and collagen type III alpha 1 (Col3α1) mRNAs were detected by ABI 7500 fast real-time polymerase chain reaction (PCR) system (Applied Biosystems, USA) with SYBR Green I (Applied Biosystems, USA). β-actin was used as an inter-tube control for sample input. Primer pairs used in this study were commercially designed (Table 1).
Table 1
Target gene | Forward primer (5'-3') | Reverse primer (5'-3') |
---|---|---|
lncRNA-5829 | TGGTTTGGGCTAACGAAAAG | TGTGTGAAGGTTCCTGACCA |
Col1α1 | AAGAAGACATCCCTGAAGTCA | TTGTGGCAGATACAGATCAAG |
Col3α1 | TTGGGATGCAGCCACCTTG | CGCAAAGGACAGATCCTGAG |
FN1 | ATGTGGACCCCTCCTGATAGT | GCCCAGTGATTTCAGCAAAGG |
β-actin | GTGCTATGTTGCTCTAGACTTCG | ATGCCACAGGATTCCATACC |
qRT-PCR, quantitative real-time polymerase chain reaction.
5-ethynyl-2′-deoxyuridine (EdU) fluorescence staining
The newly synthesized DNA reflecting the proliferative activity of CFs was detected by the EdU fluorescent staining method as described in the EdU assay kit (RiboBio, Guangzhou, China). Changes in proliferation were observed by light microscope. The red points represent newly synthesized DNA, and blue dots represent the nucleus.
Cell counting kit-8 (CCK8) assay
CFs were seeded into 96-well plates, and supplemented with 100 µL of complete culture medium per well. The cells were maintained in a 5% CO2 incubator for a duration of 48 hours, post which, 10 µL of the CCK8 assay reagent was introduced into each well. One hour subsequent to this addition, the absorbance values at 450 nm were determined using a microplate reader (CMax Plus, Molecular Devices, USA).
RNA fluorescence in situ hybridization
Fluorescent in situ hybridization (FISH) was conducted with a FISH Kit (RiboBio, Guangzhou, China) (15). Briefly, cells were fixed in 4% formaldehyde and permeabilized in PBS with 0.5% Triton-100. After washing with PBS solution, the cells were prehybridized at 37 ℃ for 30 minutes. Then, cells were incubated with hybridization solution containing the specific probe for lncRNA-5829 at 37 ℃ overnight. Finally, the nucleus was counterstained with DAPI (Biosharp, Hefei, China). Fluorescent staining was examined under a confocal laser scanning microscope (Zeiss, Jena, Germany).
Immunofluorescence
Cells were fixed with 4% paraformaldehyde (Biosharp, Hefei, China) in cell culture plates and let for 20 minutes at room temperature. Fibroblasts were initially incubated with PBS for cleansing followed by a 20-minute treatment at room temperature with 0.5% Triton X-100. The preparation was subjected to an overnight incubation with α smooth muscle actin (α-SMA) antibody (1:250, Proteintech, USA) at 4 ℃. Thereafter, the slides were sequentially incubated with DAPI (1:500, Biosharp, Hefei, China) working solution in the dark at room temperature. After a 5-minute interval, nuclear staining was executed on the cell slides. The cells were observed under a fluorescence microscope and captured photographically.
Statistical analysis
Data were analyzed employing GraphPad Prism 8.0 and were represented as mean ± standard deviation (SD). Student’s t-test was utilized to ascertain the discrepancy in expression levels between two groups. One-way analysis of variance (ANOVA) was adopted for the comparison of these groups. Should the ANOVA reveal substantial dissimilarities among the groups, the Bonferroni post-test was conducted to determine the specific group disparities. Statistical significance was inferred at P<0.05, the P value was two-sided test.
Results
Establishment of myocardial fibrosis models in vivo and in vitro
The in vivo model of myocardial fibrosis was established by ligation of the left anterior descending branch of the coronary artery for 4 weeks. The results of echocardiography in mice showed that the percentage of EF% and FS% in MI group was significantly lower than that in sham group (Figure 1A-1C). Masson staining showed that collagen deposition and fibrotic area in MI group were significantly higher than those in sham group (Figure 1D). The results of HE staining showed that compared with the sham group, the myocardial tissue around the infarcted area in the MI group was disordered, with a large number of inflammatory cell necrosis and infiltration (Figure 1E). The results of real-time PCR showed that the mRNA expression levels of Col1α1 and Col3α1 were significantly increased in MI group (Figure 1F,1G). Western blot results showed that the expression of fibrosis protein markers FN1 and Collagen1 increased significantly in MI group (Figure 1H). These results proved that the in vivo model of myocardial fibrosis in mice was successfully established. At the same time, we conducted in vitro experiments for verification. The primary CFs of neonatal mice were treated with TGF-β1 for 48 hours to establish the cellular model of myocardial fibrosis. Real-time PCR results showed that the mRNA expression of Col1α1 and Col3α1 increased significantly in TGF-β1 group (Figure 1I,1J). Western blot results showed that the expression of fibrosis protein markers FN1 and Collagen1 increased significantly in TGF-β1 group (Figure 1K). The above results indicate that the in vitro model of myocardial fibrosis induced by TGF-β1 has been established successfully.
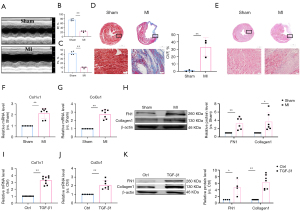
Down-regulated expression of lncRNA-5829 in myocardial fibrosis
The expression of lncRNAs in the fibrotic tissue of the marginal zone of MI in mice was determined by Mouse LncRNA Array V3.0 Chip. Using the Chip combined with potential encoding ability, we found 26 significantly up-regulated and down-regulated lncRNAs. We screened these 26 lncRNAs with significant changes and coding functions, among which ENSMUST00000175829 has a moderate length, good coding function, good conservation, and the validation results are consistent with the chip results, which has attracted our special attention. During the occurrence of myocardial fibrosis, the expression level of lncRNA-5829 was decreased significantly and it was highly conservative (Figure 2A). The expression of lncRNA-5829 in myocardial fibrosis models were verified by real-time PCR technique. It was found that the expression of lncRNA-5829 in MI group was significantly lower than that in sham group, and the expression of lncRNA-5829 in TGF-β1 group was significantly lower than that in control group (Figure 2B,2C). Furthermore, the expression of lncRNA-5829 in CFs was significantly higher than that in CMs (Figure 2D). In addition, the results of FISH experiment showed that lncRNA-5829 existed in both nucleus and cytoplasm (Figure 2E).
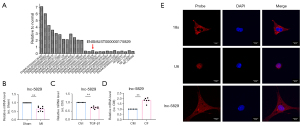
Knockdown of lncRNA-5829 induces cardiac fibrosis
To explore the role of lncRNA-5829 in myocardial fibrosis in vitro, we transfected lncRNA-5829 siRNA (0.05 nmol/mL) into neonatal mouse CFs to silence the expression of lncRNA-5829. As illustrated in Figure 3A, lncRNA-5829 siRNA1 markedly knocked down the expression of lncRNA-5829. Real-time PCR results showed that knockdown of lncRNA-5829 could significantly increase the mRNA expression of FN1, Col1α1 and Col3α1 (Figure 3B-3D). Western blot results showed that knockdown of lncRNA-5829 could significantly up-regulate the expression of fibrosis markers FN1 and Collagen1 (Figure 3E). The proliferation of CFs was observed by EdU staining by means of the number of EdU-positive cells. The results of EdU experiment showed that knockdown of lncRNA-5829 could significantly promote the proliferation of CFs (Figure 3F,3G), and the CCK8 experiment also confirmed this result (Figure 3H). The expression of α-SMA in cells was measured by immunofluorescence technique, and the results showed that knockdown of lncRNA-5829 could promote the transformation of CFs to myofibroblasts (Figure 3I). However, there were no such changes in all si-NC groups. The above experimental results show that knockdown of lncRNA-5829 can promote myocardial fibrosis.
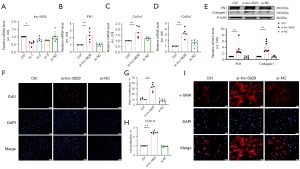
Overexpression of lncRNA-5829 inhibits TGF-β1 induced myocardial fibrosis
After overexpression of lncRNA-5829 in neonatal mouse CFs, we further detected its effect on myocardial fibrosis. Figure 4A showed the overexpression efficiency of lncRNA-5829 plasmid (2 µg/mL). Overexpression of lncRNA-5829 reduced the increase of mRNA expression of FN1, Col1α1 and Col3α1 induced by TGF-β1 but not in oe-NC groups (Figure 4B-4D). Western blot results showed that overexpression of lncRNA-5829 could significantly reduce the TGF-β1-induced increased expression of FN1 and Collagen1, as well as no changes in oe-NC group (Figure 4E). These results suggest that overexpression of lncRNA-5829 in vitro can reduce the increase of myocardial fibrosis protein markers and collagen deposition induced by TGF-β1. Similarly, overexpression of lncRNA-5829 could reverse the CFs proliferation induced by TGF-β1 with EdU staining (Figure 4F,4G). CCK8 experiment results showed that overexpression of lncRNA-5829 could reverse the increase of cell viability induced by TGF-β1 (Figure 4H). The effect of TGF-β1 on the expression of α-SMA was determined by immunofluorescence. Overexpression of lncRNA-5829 could reverse the high expression of α-SMA induced by TGF-β1 (Figure 4I). However, there was no such changes in oe-NC group. These results suggest that overexpression of lncRNA-5829 can inhibit the over-proliferation of CFs and the transformation from fibroblasts to myofibroblasts induced by TGF-β1. Taken together, the above experimental results show that overexpression of lncRNA-5829 can inhibit myocardial fibrosis.
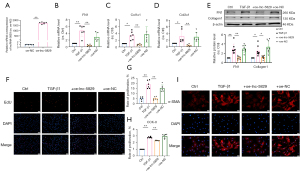
Overexpression of lncRNA-5829 improves cardiac function in mice with myocardial fibrosis
In order to study the function of lncRNA-5829 on myocardial fibrosis model in vivo, we constructed a lncRNA-5829 overexpression plasmid carried by AAV9 and injected it into mice through the tail vein. The results of echocardiography showed that the EF% and FS% of MI group were significantly lower than those of sham group. Compared with MI group, the heart EF% and FS% of lncRNA-5829 overexpression mice were significantly increased, but no such changes were found in AAV9-NC mice (Figure 5A-5C). Masson staining showed that collagen deposition in myocardium of lncRNA-5829 overexpression mice was significantly lower than that of MI mice (Figure 5D). The results of HE staining showed that lncRNA-5829 overexpression mice significantly improved the disorder of myocardial tissue arrangement and a large number of inflammatory cell infiltration around the infarcted area in MI group (Figure 5E). This observation was further supported by the notion that, the mRNA expressions of FN1, Col1α1 and Col3α1 were restored in lncRNA-5829 overexpression mice as compared with those treated with AAV9-NC (Figure 5F-5H). Similar trends in changing the expressions of FN1 and collagen1 were also confirmed by Western blot (Figure 5I). These results suggest that overexpression of lncRNA-5829 can significantly improve cardiac function and inhibit myocardial fibrosis in MI mice.
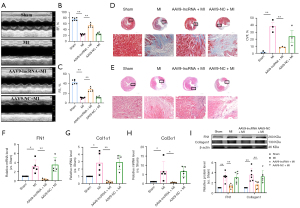
Discussion
Key finding
Here, for the first time, we found that a novel lncRNA-5829 was downregulated in the model of myocardial fibrosis. By in vivo and in vitro experiments, we proved that lncRNA-5829 participated in regulating myocardial fibrosis and played a protective function in this pathological process. Therefore, our study could provide a corresponding experimental basis for new molecular drug targets for cardiac fibrosis and related heart diseases.
Comparison with similar researches
More and more studies have found that lncRNAs play a regulatory role in CVDs and have developed a new direction for more effective treatment of CVDs. Stefan et al. found that lncRNAs may become a new target for vascular inflammation in acute and chronic disease states (16). Ning et al. found through bioinformatics analysis that six lncRNAs, SDCBP2-AS1, FENDRR, PART1, LINC00641, SNHG1, TTN-AS1, and IQCH-AS1, have diagnostic effects on patients with CVDs (17). Xiao et al. found that lncRNA (CIRKIL), a highly conserved long-stranded non-coding RNA, is a harmful factor of ischemia/reperfusion injury by regulating Ku70 nuclear translocation and DNA double-strand break repair (18). Zhang et al. found that the expression of lncRNA-RMRP was significantly up-regulated in myocardial fibrosis, and the mechanism was that RMRP absorbed miR-613 as a competitive endogenous RNA to promote the proliferation of CFs, thus played a regulatory role in the process of myocardial fibrosis (19). Parisa et al. found that LncRNA FIXER expression is significantly reduced in myofibroblasts. FIXER can regulate the expression of RUNX1 by binding with SUMO modifications and the transcription factor CBX4, controlling the differentiation of fibroblasts into myofibroblasts, matrix production, and cardiac fibrosis, thereby modulating the entire fibrosis gene program (20). Ma et al. found that the expression of RMST in the heart significantly increases after MI and is closely related to cardiac fibrosis. Further mechanistic studies confirmed that RMST regulates the function of CFs through the RMST/miR-24-3p/LOX signaling pathway (21). The above studies have shown that there are differences in the expression of lncRNAs in CVDs, especially in myocardial fibrosis. LncRNAs may be related to the occurrence and development of myocardial fibrosis and regulate the process of myocardial fibrosis through a variety of complex mechanisms. We have found that lncRNAs participate in the pathological process of myocardial fibrosis in various ways, and according to our results, lncRNA-5829 is involved in regulating myocardial fibrosis by alleviating the degree of fibrosis. As a new biomarker of myocardial fibrosis, it can provide new strategies for evaluating potential targets for clinical diagnosis and treatment of myocardial fibrosis.
Explanations of findings
Myocardial fibrosis is one of the most common pathological changes in CVDs. Under the stimulation of ischemia or chronic pressure, by secreting collagen and increasing the expression of α-SMA, it promotes the release of other ECM molecules, resulting in the imbalance of the internal composition of ECM, and pathological changes such as decreased myocardial compliance (22,23), assessing subclinical deterioration of myocardial strain parameters, cardiac remodeling, and changes in cardiac function through speckle tracking echocardiography (24,25), cardiac remodeling and cardiac function changes, and consequently fatal heart diseases such as malignant arrhythmias and heart failure (26,27). Therefore, it is particularly important to actively search for the treatment of myocardial fibrosis. In recent years, a large number of studies have shown that lncRNAs have a wide range of biological functions, not only can orchestrate physiological processes such as cell proliferation, differentiation, and metabolism but also to engage in the modulation of a multitude of pathological processes in vivo, including cancer (28), ischemic brain injury (29), diabetes (30) and so on.
In this study, the expression of lncRNAs in the fibrotic tissue of the marginal zone of MI was detected by Mouse LncRNA Array V3.0 Chip. It was found that the expression of more than 1200 lncRNAs was changed, of which 602 were up-regulated and 603 were down-regulated. Combined with stability, conservatism and prediction of encoding ability, almost 30 differentially expressed lncRNAs with significant changes were verified, and the significant down-regulation of lncRNA-5829 expression level attracted our special attention. LncRNA-5829, which is 940 nt in length and located on chromosome 10, is a new lncRNA and has not been reported in any field. LncRNA-5829 is highly conserved in mouse and human genomes and is abundantly expressed in the heart. Subsequently, we verified the chip results by real-time PCR technique, which showed that the expressions of lncRNA-5829 in myocardial fibroblasts were significantly down-regulated in in vivo and in vitro models of myocardial fibrosis, and the expression of CFs was significantly higher than that of CMs.
As the object of our study, we further discussed the role of lncRNA-5829 in myocardial fibrosis. First of all, at the cellular level, it is verified that lncRNA-5829 is involved in the regulation of myocardial fibrosis from two aspects. Functional studies showed that knockdown of lncRNA-5829 simulating the state of low expression in myocardial fibrosis model could significantly promote the occurrence of myocardial fibrosis. On the contrary, overexpression of lncRNA-5829 was observed to reverse fibrosis damage induced by TGF-β1. These results strongly imply that gain- and loss-of-functions of lncRNA-5829 may play a crucial role in myocardial fibrosis. To confirm these in vitro observations, we constructed the lncRNA-5829 overexpression plasmid and control plasmid packaged by AAV9 vector. The mouse model of myocardial fibrosis was established 4 weeks after tail vein injection of plasmids. In vivo experiments showed that lncRNA-5829 overexpression mice could significantly mitigate the degree of myocardial fibrosis induced by MI operation. The results indicate that lncRNA-5829 may play an important role in the regulation of myocardial fibrosis. This study provides a new theoretical basis for the systematic elucidation of the pathological mechanism of myocardial fibrosis and its effective prevention and treatment in the future.
Future directions
As we all know, lncRNAs can play an important regulatory role in target gene transcription and post-transcriptional expression by binding to DNA, RNA and proteins (31). LncRNAs can indirectly affect the expression level of downstream targets through sponge adsorption of microRNAs, thus participating in the occurrence and development of various heart diseases (32). The traditional view is that lncRNA, named as non-coding RNA, has no function of encoding protein, but recent studies have revealed that some lncRNAs can encode small peptides, subverting the definition of non-coding RNA. Li et al. identified three peptides encoded by lncRNAs (SEP6, SEP16, SEP18) by analyzing the transcriptome and single-cell RNA sequencing data from arterial samples of atherosclerosis patients. SEP6 and SEP16, to some extent, inhibit the transition of VSMCs to a contractile state through the MAP kinase and p53 signaling pathways, respectively, while SEP18 is involved in regulating intimal hyperplasia (33). The MLN peptide encoded by LINC00948 inhibits cardiac SR Ca2+ by suppressing SERCA and regulates Ca2+ entry into the SR to control muscle relaxation (34). The regulating mechanism of lncRNA by encoding small peptides has also become a recent research hotspot. Through CPCWeb bioinformatics software (http://cpc2.cbi.pku.edu.cn), we predicted the possibility and amino acid sequence of peptide encoded by lncRNA-5829. Excitingly, the possibility of lncRNA-5829 to encode peptide was 98.666%, the length of encoded peptide was 173AA, and the open reading frame (ORF) was complete (Figure 6A). Based on the analysis of FICKETT testcode score, putative peptide length and isoelectric point, we think that there is a very high probability of lncRNA-5829 to encode peptide (Figure 6B-6D). Then we predicted the possible ORF region of lncRNA-5829 to encode peptide through the ExPASyWeb bioinformatics software (https://web.expasy.org/translate) (Figure 6E). The longer red sequence is the most likely to encode. In subsequent experiments, we plan to first verify whether lncRNA5829 is actively expressed and whether it is in a translational state, by assessing the expression of lncRNA5829, which encodes a micropeptide, using real time PCR; at the same time, we will isolate and purify ribosomes from tissues or cells, and perform translatome quantitative detection. The encoding function of lncRNA-5829 will be verified by inserting the Flag/His sequence after the predicted ORF to construct the tag fusion protein vector plasmid. In the following study, we will continue to elucidate the underlying mechanism of action of lncRNA-5829. It is conceivable that the role of lncRNA-5829 in regulating myocardial fibrosis through a spectrum of mechanisms, warranting future investigation to unravel these potentialities. However, despite mass spectrometry’s proficiency in detecting peptides, it has limitations in detecting peptides encoded by LncRNAs due to their relatively small size (35). However, with the further optimization of mass spectrometry, RNA sequencing, ribosomal map analysis and the development and progress of other disciplines, it is believed that more small peptides will be identified in the future, which will provide a new direction for the study of many diseases.
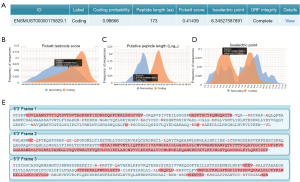
Limitations
There are certain limitations in this study. In the present study, we focused on the function of lncRNA-5829 involved in regulating myocardial fibrosis but did not explore the potential mechanism in this process. Additionally, there were certain issues in data analysis as well, due to the differences in the results of each repeated experiment, there was no uniform normalization process. This will also be an aspect that we need to focus on and improve in subsequent experiments.
Implications and actions needed
This study indicates that the expression of lncRNA-5829 is downregulated during the process of myocardial fibrosis, and overexpression of lncRNA-5829 can rescue myocardial fibrosis. The ultimate goal of lncRNAs research is to translate it into clinical applications. LncRNAs are relatively stable in blood and less sensitive to degradation mediated by nucleases (36). Therefore, serum lncRNAs can serve as diagnostic markers for diseases. According to our research, human serum lncRNA-5829 has the potential to be used as a diagnostic marker for myocardial fibrosis. Although the secondary structure of lncRNAs makes the exogenous delivery of lncRNAs difficult, limiting the development of lncRNA drugs. However, with further exploration of lncRNAs, drug delivery systems such as lentiviral vectors and lipid nanoparticles can be applied to the delivery of lncRNAs, and have entered the clinical trial stage (37). Based on our research, overexpression of lncRNA5829 can inhibit myocardial fibrosis, so whether lncRNA5829 can be translated into clinical drugs and delivered into the human body is worth further research.
Conclusions
This study found that the expression of lncRNA829 was downregulated in a myocardial fibrosis model, and lncRNA5829 is involved in regulating the pathological process of myocardial fibrosis, playing an important protective role in it.
Acknowledgments
We thank Dr. Baiyan Li for his help in polishing our paper.
Footnote
Reporting Checklist: The authors have completed the ARRIVE reporting checklist. Available at https://cdt.amegroups.com/article/view/10.21037/cdt-24-462/rc
Data Sharing Statement: Available at https://cdt.amegroups.com/article/view/10.21037/cdt-24-462/dss
Peer Review File: Available at https://cdt.amegroups.com/article/view/10.21037/cdt-24-462/prf
Funding: This work was supported in part by
Conflicts of Interest: All authors have completed the ICMJE uniform disclosure form (available at https://cdt.amegroups.com/article/view/10.21037/cdt-24-462/coif). All authors report that this work was supported by National Natural Science Foundation of China (Nos. 82070236 and 81470523), Excellent Young Talents Funding of College of Pharmacy in Harbin Medical University (2019-YQ-15) and Heilongjiang Province Traditional Chinese Medicine Research Project (2018-146). The authors have no other conflicts of interest to declare.
Ethical Statement: The authors are accountable for all aspects of the work in ensuring that questions related to the accuracy or integrity of any part of the work are appropriately investigated and resolved. The utilization of these animals was sanctioned by the Ethics Committee of Harbin Medical University (approval No. IRB3002620), in compliance with the Ethics Committee of Harbin Medical University and the NRC Guide for the Care and Use of Laboratory Animals (8th edition).
Open Access Statement: This is an Open Access article distributed in accordance with the Creative Commons Attribution-NonCommercial-NoDerivs 4.0 International License (CC BY-NC-ND 4.0), which permits the non-commercial replication and distribution of the article with the strict proviso that no changes or edits are made and the original work is properly cited (including links to both the formal publication through the relevant DOI and the license). See: https://creativecommons.org/licenses/by-nc-nd/4.0/.
References
- Zhao D, Liu J, Wang M, et al. Epidemiology of cardiovascular disease in China: current features and implications. Nat Rev Cardiol 2019;16:203-12. [Crossref] [PubMed]
- Lozano-Vidal N, Bink DI, Boon RA. Long noncoding RNA in cardiac aging and disease. J Mol Cell Biol 2019;11:860-7. [Crossref] [PubMed]
- González A, Schelbert EB, Díez J, et al. Myocardial Interstitial Fibrosis in Heart Failure: Biological and Translational Perspectives. J Am Coll Cardiol 2018;71:1696-706. [Crossref] [PubMed]
- Benjamin EJ, Muntner P, Alonso A, et al. Heart Disease and Stroke Statistics-2019 Update: A Report From the American Heart Association. Circulation 2019;139:e56-e528. [Crossref] [PubMed]
- Mattick JS, Amaral PP, Carninci P, et al. Long non-coding RNAs: definitions, functions, challenges and recommendations. Nat Rev Mol Cell Biol 2023;24:430-47. [Crossref] [PubMed]
- Chi Y, Wang D, Wang J, et al. Long Non-Coding RNA in the Pathogenesis of Cancers. Cells 2019;8:1015. [Crossref] [PubMed]
- Sanchez Calle A, Kawamura Y, Yamamoto Y, et al. Emerging roles of long non-coding RNA in cancer. Cancer Sci 2018;109:2093-100. [Crossref] [PubMed]
- Cao M, Luo H, Li D, et al. Research advances on circulating long noncoding RNAs as biomarkers of cardiovascular diseases. Int J Cardiol 2022;353:109-17. [Crossref] [PubMed]
- Abdulle LE, Hao JL, Pant OP, et al. MALAT1 as a Diagnostic and Therapeutic Target in Diabetes-Related Complications: A Promising Long-Noncoding RNA. Int J Med Sci 2019;16:548-55. [Crossref] [PubMed]
- Azat M, Huojiahemaiti X, Gao R, et al. Long noncoding RNA MIAT: A potential role in the diagnosis and mediation of acute myocardial infarction. Mol Med Rep 2019;20:5216-22. [Crossref] [PubMed]
- Hua X, Wang YY, Jia P, et al. Multi-level transcriptome sequencing identifies COL1A1 as a candidate marker in human heart failure progression. BMC Med 2020;18:2. [Crossref] [PubMed]
- Ge Z, Yin C, Li Y, et al. Long noncoding RNA NEAT1 promotes cardiac fibrosis in heart failure through increased recruitment of EZH2 to the Smad7 promoter region. J Transl Med 2022;20:7. [Crossref] [PubMed]
- Zhang M, Zhang B, Wang X, et al. LncRNA CFAR promotes cardiac fibrosis via the miR-449a-5p/LOXL3/mTOR axis. Sci China Life Sci 2023;66:783-99. [Crossref] [PubMed]
- Yi Q, Feng J, Lan W, et al. CircRNA and lncRNA-encoded peptide in diseases, an update review. Mol Cancer 2024;23:214. [Crossref] [PubMed]
- Luo S, Zhang M, Wu H, et al. SAIL: a new conserved anti-fibrotic lncRNA in the heart. Basic Res Cardiol 2021;116:15. [Crossref] [PubMed]
- Haemmig S, Simion V, Feinberg MW. Long Non-Coding RNAs in Vascular Inflammation. Front Cardiovasc Med 2018;5:22. [Crossref] [PubMed]
- Yang N, Song Y, Li Y, et al. Characterization of lncRNA-associated ceRNA network to uncover novel potential biomarkers in coronary artery disease. Medicine (Baltimore) 2023;102:e35913. [Crossref] [PubMed]
- Xiao H, Zhang M, Wu H, et al. CIRKIL Exacerbates Cardiac Ischemia/Reperfusion Injury by Interacting With Ku70. Circ Res 2022;130:e3-e17. [Crossref] [PubMed]
- Zhang SY, Huang SH, Gao SX, et al. Upregulation of lncRNA RMRP promotes the activation of cardiac fibroblasts by regulating miR-613. Mol Med Rep 2019;20:3849-57. [Crossref] [PubMed]
- Aghagolzadeh P, Plaisance I, Bernasconi R, et al. Assessment of the Cardiac Noncoding Transcriptome by Single-Cell RNA Sequencing Identifies FIXER, a Conserved Profibrogenic Long Noncoding RNA. Circulation 2023;148:778-97. [Crossref] [PubMed]
- Ma T, Qiu F, Gong Y, et al. Therapeutic silencing of lncRNA RMST alleviates cardiac fibrosis and improves heart function after myocardial infarction in mice and swine. Theranostics 2023;13:3826-43. [Crossref] [PubMed]
- Liu L, Luo F, Lei K. Exosomes Containing LINC00636 Inhibit MAPK1 through the miR-450a-2-3p Overexpression in Human Pericardial Fluid and Improve Cardiac Fibrosis in Patients with Atrial Fibrillation. Mediators Inflamm 2021;2021:9960241. [Crossref] [PubMed]
- Broekmans K, Giesen J, Menges L, et al. Angiotensin II-Induced Cardiovascular Fibrosis Is Attenuated by NO-Sensitive Guanylyl Cyclase1. Cells 2020;9:2436. [Crossref] [PubMed]
- Sonaglioni A, Albini A, Fossile E, et al. Speckle-Tracking Echocardiography for Cardioncological Evaluation in Bevacizumab-Treated Colorectal Cancer Patients. Cardiovasc Toxicol 2020;20:581-92. [Crossref] [PubMed]
- Mondillo S, Cameli M, Caputo ML, et al. Early detection of left atrial strain abnormalities by speckle-tracking in hypertensive and diabetic patients with normal left atrial size. J Am Soc Echocardiogr 2011;24:898-908. [Crossref] [PubMed]
- Long Y, Niu Y, Liang K, et al. Mechanical communication in fibrosis progression. Trends Cell Biol 2022;32:70-90. [Crossref] [PubMed]
- Al-Hasani J, Sens-Albert C, Ghosh S, et al. Zyxin protects from hypertension-induced cardiac dysfunction. Cell Mol Life Sci 2022;79:93. [Crossref] [PubMed]
- Li SY, Zhu Y, Li RN, et al. LncRNA Lnc-APUE is Repressed by HNF4α and Promotes G1/S Phase Transition and Tumor Growth by Regulating MiR-20b/E2F1 Axis. Adv Sci (Weinh) 2021;8:2003094. [Crossref] [PubMed]
- Chen J, Jin J, Zhang X, et al. Microglial lnc-U90926 facilitates neutrophil infiltration in ischemic stroke via MDH2/CXCL2 axis. Mol Ther 2021;29:2873-85. [Crossref] [PubMed]
- Tang X, Luo Y, Yuan D, et al. Long noncoding RNA LEENE promotes angiogenesis and ischemic recovery in diabetes models. J Clin Invest 2023;133:e161759. [Crossref] [PubMed]
- Zhang S, Ji WW, Wei W, et al. Long noncoding RNA Meg3 sponges miR-708 to inhibit intestinal tumorigenesis via SOCS3-repressed cancer stem cells growth. Cell Death Dis 2021;13:25. [Crossref] [PubMed]
- Mei J, Lin W, Li S, et al. Long noncoding RNA TINCR facilitates hepatocellular carcinoma progression and dampens chemosensitivity to oxaliplatin by regulating the miR-195-3p/ST6GAL1/NF-κB pathway. J Exp Clin Cancer Res 2022;41:5. [Crossref] [PubMed]
- Li K, Li B, Zhang D, et al. The translational landscape of human vascular smooth muscle cells identifies novel short open reading frame-encoded peptide regulators for phenotype alteration. Cardiovasc Res 2023;119:1763-79. [Crossref] [PubMed]
- Zhang L, Tang M, Diao H, et al. LncRNA-encoded peptides: unveiling their significance in cardiovascular physiology and pathology-current research insights. Cardiovasc Res 2023;119:2165-78. [Crossref] [PubMed]
- Zhang Y. LncRNA-encoded peptides in cancer. J Hematol Oncol 2024;17:66. [Crossref] [PubMed]
- Badowski C, He B, Garmire LX. Blood-derived lncRNAs as biomarkers for cancer diagnosis: the Good, the Bad and the Beauty. NPJ Precis Oncol 2022;6:40. [Crossref] [PubMed]
- Han S, Chen X, Huang L. The tumor therapeutic potential of long non-coding RNA delivery and targeting. Acta Pharm Sin B 2023;13:1371-82. [Crossref] [PubMed]