Computed tomography of cardiomyopathies
Introduction
Cardiomyopathies are a heterogeneous group of diseases of the myocardium associated with mechanical and/or electrical dysfunction typically exhibiting inappropriate ventricular hypertrophy or dilatation in the absence of underlying ischemia, hypertension, valvular or congenital heart disease (1). Heart failure and cardiomyopathy are common entities affecting approximately 6.5 million people in the United States and becoming increasingly prevalent in recent years (2). Ischemic heart disease is by far the most common etiology of heart failure, with dilated cardiomyopathy being the most common primary cardiomyopathy (3). Several non-invasive imaging modalities are used in cardiomyopathies to effectively and efficiently diagnose, categorize, and guide subsequent therapies, which are of critical importance. Computed tomography (CT) is playing an increasingly important role in the evaluation of cardiomyopathies. In this article, we review the role of CT in the evaluation of cardiomyopathies.
Role of imaging
Imaging plays a crucial role in the screening, detection, and characterization of cardiomyopathies. There are several available imaging modalities, each with its own strengths and limitations, particularly echocardiography, nuclear medicine, magnetic resonance imaging (MRI) and invasive coronary angiography (ICA).
Echocardiography
Echocardiography is the initial imaging modality used in the evaluation of cardiac failure and cardiomyopathy as it is a widely available, portable technique that allows for real-time evaluation of chamber size and morphology, systolic and diastolic function, and valvular abnormalities as well as blood flow, all without the use of ionizing radiation (4). Limitations of echocardiography include inadequate soft tissue characterization and suboptimal field-of-view in the setting of poor acoustic windows as in obesity, obstructive lung disease, or chest wall deformities. While transesophageal echocardiography (TEE) can overcome some limitations of poor surface windows, it is invasive with more limited availability.
Nuclear medicine
Nuclear medicine is also valuable in evaluation of cardiomyopathy (5,6), especially allowing for evaluation of myocardial perfusion with single-photon emission CT (SPECT). SPECT has high accuracy in evaluation of coronary artery disease (CAD) and also provides prognostic information (7,8). It also helps in distinguishing ischemic from non-ischemic etiologies (9). Nuclear imaging techniques have also shown useful in other areas such as left ventricle (LV) analysis (10), myocardial viability utilizing positron emission tomography (PET) (11), and diagnosis of some cardiomyopathy subtypes (5). For example, in the evaluation of cardiac amyloidosis, technetium-pyrophosphate has shown potential in distinguishing light chain from transthyretin amyloidosis subtypes, while 123I-metaiodobenzylguanidine (MIBG) can be useful in functional evaluation of amyloid cardiomyopathy (12). 123I-MIBG and carbon-11 labeled hydroxyephedrine are utilized for sympathetic innervation evaluation as well as potential future applications for monitoring molecular interventions (13). Disadvantages of nuclear imaging include the use of ionizing radiation, limited spatial resolution, poor tissue characterization, and relatively long imaging times.
ICA
ICA is considered the gold standard in the evaluation of CAD with fractional flow reserve (FFR) proven to be superior to luminal assessment in guiding treatment and improving outcomes (14). It also allows for intervention in patients with significant coronary artery stenosis. However, the main disadvantage of angiography is radiation exposure and invasiveness of the procedure with the potential for uncommon, yet serious, complications (15).
MRI
MRI provides excellent information in the evaluation of cardiomyopathy without the need for radiation or contrast. It has good spatial and temporal resolution and provides accurate and reproducible measurements of cardiac volumes and function, along with good soft-tissue characterization (16,17), which is done with an array of sequences such as T1, T2, T2*, steady state free precession (SSFP), short tau inversion recovery (STIR), perfusion, delayed enhancement, and T1 mapping (18-20) enabling differentiation of several cardiomyopathy phenotypes (21,22). However, cardiac MRI is not widely available, typically requires higher levels of skills, and has several contraindications including some pacemakers/implantable cardioverter defibrillators (ICD), claustrophobia and severe renal dysfunction.
CT
CT is not typically considered a first-line imaging modality in the evaluation of cardiomyopathies. However, it is increasingly gaining importance in this population, particularly in patients who have contraindications for other imaging tests or have suboptimal results, for example due to poor acoustic window in echocardiogram or contraindications for MRI. CT is widely available and has a rapid turnaround, with most scans performed in a matter of seconds. CT has excellent spatial resolution for evaluation of even small structures such as coronary arteries and modern scanners have high temporal resolution up to 66 milliseconds. CT angiography (CTA) is excellent in the evaluation of coronary arteries, which is an important component of cardiomyopathy evaluation as discussed below. CT can also provide functional evaluation as well as some tissue characterization and can be used before and after treatment (23). The principle disadvantages of CT include the use of ionizing radiation and iodinated contrast material. Iodinated contrast should be used with caution in patients with renal dysfunction and contrast allergy.
CT techniques
Recent progress in CT technology has allowed for the effective and efficient imaging of the heart for numerous applications. Key approaches to selecting among different cardiac techniques are summarized in Table 1. Specific scan parameters for several techniques are listed in Table 2. Advances in hardware have enabled acquisition of images at high spatial and temporal resolutions, with smaller detectors and improved detector electronics and faster gantry rotation times. Most centers perform cardiac CT with 16 to 64 slice scanners with 64 slice multi-detector CT (MDCT) systems able to produce isotropic spatial resolution as high as 0.35 mm and a temporal resolution as good as 165 ms (24,25). Multi-detector scanners with up to 320 slices allows increased z-coverage of up to 16 cm, which allows single heart beat scanning. There are also dual source scanners, which have improved temporal resolution (up to 66 milliseconds) and have the option of high-pitch helical mode in which data can be obtained rapidly without data gaps due to the presence of a second tube (26). There are also several types of dual-energy scanners, such as dual-source, rapid kilovoltage peak (kVp) switching, dual spin and dual-layer technologies, which allow material characterization beyond what is possible with single energy CT (27).
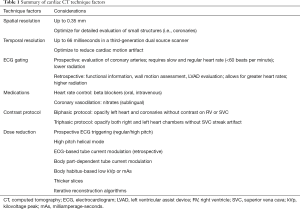
Full table
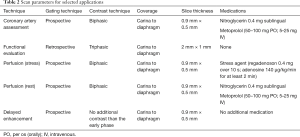
Full table
The CT protocol for the evaluation of the cardiomyopathies can be varied depending on the specific clinical question to be answered. Cardiac CT is performed with electrocardiogram (ECG) gating to avoid motion artifacts. For the evaluation of coronary arteries, prospective ECG-triggering is the default mode to minimize radiation dose, although this is possible only if the heart rate is slow and regular (less than 60–65 beats per minute, depending on the scanner). Retrospective ECG-gating is used in evaluation of coronary arteries if the heart rate is high or irregular. This mode is also used in specific scenarios such as in the evaluation of cardiac function and volumes, wall motion or valvular abnormalities, and LV assist device (LVAD) position and complications. ECG-based tube current modulation is used with retrospective mode in order to minimize radiation dose. Non-ECG gated/helical high-pitch techniques can be used for the evaluation of pulmonary veins (28). For evaluation of coronary arteries, beta-blockers are administered either orally or intravenously if the heart rate is high or irregular (29). Sublingual nitroglycerine is also administered to dilate the coronary arteries. However, these medications are not required for non-coronary arterial indications, such as evaluation of cardiac function or LVAD evaluation. Intravenous contrast protocols are also optimized depending on the clinical indication. Recommended contrast injection protocols according to the Society of Cardiovascular CT are as follows: for evaluation of coronary arteries, a biphasic protocol is used where injection of contrast (typically 50–120 mL at 5–7 mL/s) is followed by a saline bolus (typically 40–50 mL at 5–7 mL/s) to opacify the left heart and coronary arteries, with no contrast in the right ventricle (RV) or superior vena cava (SVC) (30,31). For evaluation of cardiac volumes and function, a triphasic protocol is used, where injection of contrast at 5–7 mL/s is followed by either a slower rate of contrast injection at 2 mL/s or a contrast-saline admixture at same rate (50:50 of contrast:saline at 5–7 mL/s), followed by a smaller volume saline bolus to opacify all the cardiac chambers without streak artifact in the SVC (30).
Several cardiac CT techniques allow for additional cardiac evaluation beyond anatomic and basic function assessment. Perfusion imaging relies on the distribution of contrast material during the first pass through the myocardium which is dependent on the myocardial arterial blood supply (32). CT myocardial perfusion exams have been described with both single and dual energy techniques. In the single energy technique, images are acquired in the early arterial phase (i.e., 8–16 s) during first pass through the myocardium with perfusion abnormalities presenting as areas of decreased myocardial attenuation secondary to reduced blood flow (Figure 1). Images are obtained at rest and after pharmacological stress, with variable order in which these are done (33). Using dual energy techniques, iodine perfusion maps displayed as color maps can evaluate myocardial perfusion differences with the value of quantified iodine serving as a marker of myocardial perfusion (34). Delayed myocardial enhancement (Delayed iodine enhancement) imaging is an indicator of myocardial fibrosis/scar analogous to late gadolinium enhanced MRI. This is done 5–15 min after intravenous administration of iodinated contrast and can be done either in the single or dual-energy mode. Single-energy technique is limited by poor signal-to-noise ratio (SNR) and contrast-to-noise ratio (CNR) as well as beam hardening artifact. Improved SNR and CNR can be seen in virtual monoenergetic images and iodine maps of dual-energy scanners. This technique can be used for the characterization of cardiomyopathies based on the pattern of enhancement, particularly in patients who have contraindications for MRI. This can also be used for the evaluation of myocardial viability in patients with infarction (35). Multiple studies have shown sensitivities of 88–92% and specificities of 72–77% in detection of chronic myocardial infarction (MI) (35,36). There is also potential for estimating the extracellular volume fraction (ECV), which is a biomarker for myocardial fibrosis. This technique is not widely used and requires data from larger patient cohorts (37).

Strain imaging is used for the evaluation of regional cardiac function in longitudinal, radial, circumferential and torsional directions by evaluating velocity gradients between two objects in space. This is usually performed using echocardiography or MRI; however, CT can also be used for evaluating myocardial strain. A recent study showed that quantitative LV strain is feasible using CT feature tracking, with comparable accuracy as that of echocardiography and has potential for evaluation of regional function in patients with poor echo windows or contraindications for MRI (38). This technique has potential in detecting regional functional abnormalities prior to the onset of overt cardiac failure. CT metrics such as changes in wall thickness, wall motion and volume over time can also be used to quantify LV dyssynchrony, which is useful for predicting response to cardiac resynchronization therapy (39).
Although radiation dose is a concern with the use of cardiac CT (40), it can be minimized using the principle of As Low As Reasonably Achievable (ALARA) by using a variety of technique such as prospective ECG-triggering including helical mode (41), ECG-based tube current modulation in retrospective acquisitions (42,43), automatic-tube current modulation based on body-part size (42), body-habitus based low kVp or milliamperage-seconds (mAs) (43,44), higher-pitch (45,46), thicker slices, and iterative reconstruction techniques (40,47).
Role of CT in cardiomyopathy assessment
There are several established roles for CT in the evaluation of cardiomyopathies. Broadly, these include: evaluation of coronary arteries, characterization cardiomyopathy type, evaluation of cardiac volumes and function, treatment planning, and post-treatment evaluation, which are summarized on Table 3.
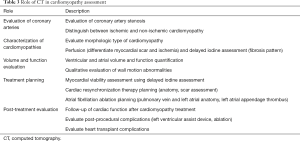
Full table
Evaluation of coronary arteries
Cardiac failure and cardiomyopathy is often suggested through history, physical exam, and echocardiography. However, accurate characterization is necessary for optimal patient management. The initial step in characterization of heart failure is to diagnose or exclude ischemic cardiomyopathy (48), since LV functional recovery can be achieved in these patients by coronary revascularization procedures (11). Although traditionally the work-up for ischemic cardiomyopathy was done by ICA (49), CTA is now considered the first-line non-invasive imaging test for exclusion of significant coronary artery stenosis, especially in the low to intermediate risk population (Figure 2). A particular advantage of coronary CTA (CCTA) in assessment of CAD is its high specificity (95–98%) and negative predictive value (95–100%) (50-52). In patients without previously known coronary disease presenting with and LV ejection fraction (LVEF) of less than 35%, CCTA demonstrated a sensitivity of 98% and specificity of 97% for the diagnosis of CAD (53). As a result of this and other studies, CCTA has been given a high appropriateness rating for the evaluation of ischemic etiology in patients presenting with heart failure and ischemic symptoms and may be even appropriate in imaging heart failure patients without ischemic symptoms (54). With latest technologies such as CT perfusion, CT-FFR or transcoronary attenuation gradient, the hemodynamic significance of coronary artery stenosis can also be characterized, which helps in triaging patients for ICA and selecting appropriate patients for revascularization, thus improving outcome (32,34,55-58). CT can also evaluate the plaque burden and vulnerability which also carry prognostic significance (59).
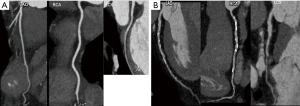
Characterization of cardiomyopathies
Characterization of the different phenotypes of cardiomyopathies is important as the treatment varies depending on the etiology. For example, sarcoidosis benefits from corticosteroids, whereas iron-overload cardiomyopathy requires chelation therapy. Although MRI is the imaging modality that is typically used for characterizing cardiomyopathies, CT can also be effective in patients who cannot have MRI. The conventional CT images obtained using biphasic or triphasic injection protocol can provide morphological information, which can be used for diagnosis of several cardiomyopathies. With retrospective ECG-gated techniques, the qualitative and quantitative analysis of the cardiac volumes and function can be performed, which is also useful in the diagnosis. Both of these can also be used to exclude other diseases such as congenital and valvular heart diseases. CT myocardial perfusion can be used for evaluation of myocardial ischemia and infarct, with the former showing perfusion defects only with stress and the latter showing defect in both stress and rest images. Delayed iodine enhancement (DIE) images can be obtained 5–15 min after administration of intravenous contrast (60), either in the single or dual-energy mode to evaluate for myocardial fibrosis and scar analogous to MRI, but with much lower CNR and associated radiation dose. Based on the pattern and distribution of enhancement, different types of cardiomyopathies can be distinguished (61,62). More recently, similar to cardiac MRI, cardiac CT has been shown to have the potential to quantify the myocardial ECV, which is a surrogate for myocardial fibrosis (63). Cardiac CT has shown good correlation in ECV calculation in several heart failure cohorts when compared with the cardiac MRI (64,65). Table 4 summarizes CT findings for various cardiomyopathies described in greater detail here.
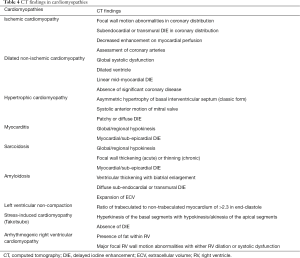
Full table
In ischemic cardiomyopathy, focal regional wall motion abnormalities in a vascular distribution can be evaluated using cine images. Wall thinning or fatty metaplasia may be seen in chronic infarcts (Figure 3A). On DIE images, areas of enhancement are seen in a sub-endocardial or transmural location corresponding to a vascular distribution. Myocardial perfusion techniques will demonstrate areas of decreased enhancement on first-pass images, both at stress and rest and may delineate a greater territory of ischemic myocardium (32). The percentage of stenosis of the supplying coronary arteries as well as plaque characterization can also be readily assessed. Complications of MI including aneurysm (Figure 3B), pseudoaneurysm, rupture and thrombus can also be detected in CT.
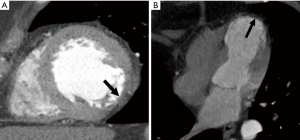
Dilated cardiomyopathy is characterized by a dilated LV and global systolic dysfunction without regional wall motion abnormalities (Figure 4). A linear mid-myocardial pattern of DIE may also be seen in idiopathic cases. CT has been shown to effectively distinguish patients with ischemic and non-ischemic dilated cardiomyopathies by the exclusion of significant coronary disease as well as absence of typical subendocardial or transmural DIE enhancement patterns (66). However, CT and other imaging modalities are often unable to differentiate between the various causative etiologies of dilated cardiomyopathy.
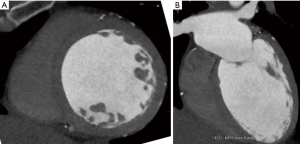
Hypertrophic cardiomyopathy is characterized most commonly by asymmetrical hypertrophy of the basal septum, with narrowing of the LV outflow tract and presence of systolic anterior motion of the mitral valve (Figure 5). Atypical forms include apical (Figure 6), mid ventricular, and concentric hypertrophy. Myocardial fibrosis has been shown in DIE images with either focal, typically located near the RV insertion points, or diffuse (61,62). The amount of fibrosis present in these patients has been shown to correlate with the presence of life-threatening arrhythmias and risk of sudden death (67).
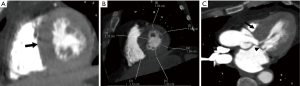
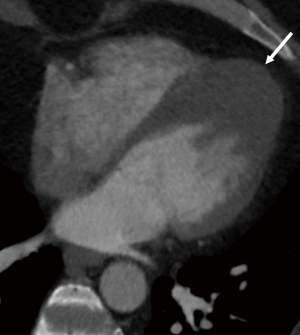
In myocarditis, cine images demonstrate global or regional wall motion abnormalities. On DIE images, sub-epicardial/mid-myocardial enhancement may be seen (68). Contrary to MRI, myocardial edema is poorly assessed at cardiac CT. Differentiating myocarditis from ischemic heart disease may be challenging given similar presentation of chest pain and elevated cardiac biomarkers but is crucial as myocarditis is often self-limiting with anti-inflammatory therapy and supportive care.
Infiltrative cardiomyopathies are characterized by concentric LV thickening and CT has been shown to correlate well with MRI in the characterization of these cardiomyopathies, including sarcoidosis and amyloidosis (69,70). Cardiac involvement in sarcoidosis has been shown to be present in up to 5% of patients with systemic sarcoidosis (71). CT may demonstrate focal wall thickening or thinning in the acute and chronic stages, respectively, with regional motion abnormalities. On DIE images, mid-myocardial or sub-epicardial DIE indicative of fibrosis may be seen similar to that seen in cases of myocarditis (Figure 7) (69). The presence of fibrosis has shown to predict adverse cardiac outcomes of these patients (72). In the acute phase, the myocardium is thickened and there may be focal areas of granulomas. In the chronic phase, the myocardium is thinned.
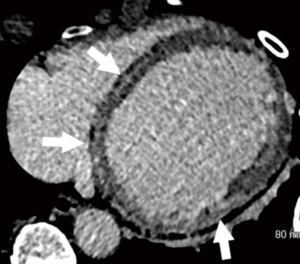
Amyloidosis is characterized by abnormal deposition of fibrillar amyloid proteins within the myocardial extracellular space with various clinical subtypes including light-chain (AL), familial transthyretin-related (ATTR), secondary, and senile systemic forms. Structurally, amyloidosis typically demonstrates ventricular thickening with biatrial enlargement. On DIE images, there is a distinctive diffuse sub-endocardial to transmural enhancement pattern (73). CT ECV techniques have also demonstrated expansion of ECV (74).
LV non-compaction is characterized by prominent and excessive LV trabeculations due to an arrest of myocardial compaction, which may be either familial or sporadic. At imaging, the diagnosis can be made by a ratio of trabeculated to non-trabeculated myocardium of greater than 2.3 in end-diastole (75) (Figure 8). Areas of trabecular and subendocardial delayed contrast enhancement can be seen (76). Thrombosis, LV dysfunction and arrhythmias are complications associated with LV noncompaction (LVNC).
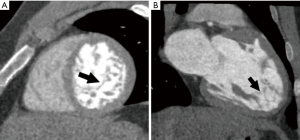
Stress induced cardiomyopathy (Takotsubo) is a reversible LV systolic dysfunction in the absence of coronary disease often seen after periods of severe emotional stress. Evaluation of CT cine images is key there is a characteristic pattern hyperkinesis of the basal segments with hypokinesis/akinesias of the apical segments, resulting in apical ballooning. An atypical form has also been reported with hyperkinesis of the apical segments and akinesis of basal segments (77,78). CT is particularly useful in excluding coronary disease in these patients. DIE images will often be normal.
Arrhythmogenic RV cardiomyopathy (ARVD) is characterized by fibrofatty replacement of the RV with variable involvement of the LV and presents clinically as arrhythmias, typically at a young age. While CT may show fat in the RV myocardium, contributing diagnostic findings at imaging according to Task Force criteria include the presence of major wall motion abnormalities (akinesia, dyskinesia, or dyssynchrony) with either a dilated RV (end-diastolic volume greater than 110 mL/m2 for male, greater than 100 mL/m2 for female) or RV dysfunction (ejection fraction less than 40%) (Figure 9) (79,80) .
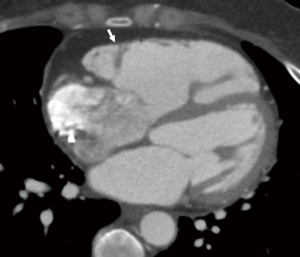
Quantification of cardiac volumes and function
CT provides accurate measurements of the cardiac volumes and functions, and is utilized when quantification is not possible with other imaging modalities such as echocardiogram due to poor acoustic windows or MRI due to presence of MRI-incompatible pacemakers/ICDs or other contraindications. For quantification, retrospective ECG-gated acquisition throughout the cardiac cycle is required, along with a tri-phasic contrast injection to visualize both the ventricles. Radiation dose is minimized by using ECG-based tube current modulation and thicker slices. Beta blockers and nitroglycerine are not needed for this type of indication. The retrospective data is typically reconstructed from 0% to 100%, at 10% intervals, with the 0% image usually correlating with end-diastole and 30–40% image correlating with end-systole. The CT images are reconstructed in the short-axis plane, usually at 2-mm thickness, although may be variable. Cine images can be used to qualitatively evaluate for morphology and function.
Cardiac volumes and function can be calculated by various methods, including the area-length method, Simpsons method, and threshold based segmentation. In the area-length method, the volume is based on assumption of ellipsoid shape of ventricle and measuring the area and length of the ventricle in the long axis. In Simpson’s method, volumes are determined by summing the areas of LV cavity at each short axis slice multiplied by the slice thickness (Figure 10A). Simpson’s method has been shown superior to the area-length technique, which overestimates both end-diastolic and end-systolic volumes and stroke volume (81). Threshold-based segmentation utilizes the differences in attenuation between the blood pool and myocardium. Ventricular volume is calculated by summing of all the contiguous voxels whose attenuation is above that of a predefined threshold. This technique has been shown to be accurate and reproducible with low interobserver variability with short processing time (82). Regional wall motion can also be evaluated including wall attenuation, systolic percentage wall thickening, wall motion/shortening and regional ejection fraction (Figure 10B). Lastly, while assessment of diastolic function is typically performed with echocardiography, it has also been shown to be feasible with CT. CT derived transmitral velocity, mitral septal tissue velocity, and estimation of LV filling pressures have shown good correlation with echocardiogram (83).
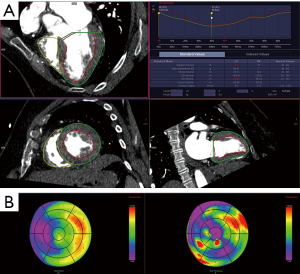
The LVEF and volumes obtained by CT have been shown to correlate with other imaging modalities such as echocardiography (81,84,85), SPECT (86) and MRI (87,88), although there has been mild but consistent overestimation of LVEF (81,84). The temporal resolution of CT is not as good as echocardiography and hence the measurements may be limited in the setting of elevated heart rates (89). Compared to MRI, the acquisition is fast and the images can be reconstructed even at thinner slices if more accurate quantification is desired (90).
Cardiac CT is also valuable in assessing the volumes and function of other cardiac chambers, including the RV (91). CT has been shown to be accurate compared to MRI in the evaluation of RV volumes and functions (79,92,93), particularly in patients who cannot have MRI, since echocardiography provides limited acoustic windows in adults for the evaluation of RV. Atrial volumes and function can also be quantified using CT (94). Excellent correlation has been shown between CT and MRI in patients on sinus rhythm, but lower accuracy is seen in patients with atrial fibrillation (94,95).
Treatment planning
CT is also increasingly used in the treatment planning of various cardiomyopathies, particularly for viability assessment, cardiac synchronization therapy (CRT) and radiofrequency ablation of pulmonary veins for atrial fibrillation. Myocardial viability is important in patients being planned for coronary revascularization, since the success of this procedure is less if there are no viable segments present. This assessment is traditionally performed using cardiac MRI or PET. But, in patients who cannot undergo imaging at either of these modalities, CT is a good alternative imaging test. Infarcted myocardium is seen as a perfusion defect that persists in rest and stress myocardial perfusion images. Scar shows high iodine uptake in DIE images, either with single or dual energy technique (60). Studies have good accuracy of DIE in evaluating myocardial viability compared to MRI, both for single-energy (sensitivity, 92%, specificity, 100%, and accuracy, 94% when correlated with infarct size) (60,96) and dual energy CT (36).
CRT is typically used in treatment of cardiac dysfunction the setting of wide complex rhythms. There is high failure rate of CRT with high rates of response failure and suboptimal lead implantation (30% and 10%, respectively) (97,98). Key elements required for successful CRT include: knowledge of precise area of latest mechanical activation, presence of viable myocardium, and presence of accessible venous tributaries draining this area. Knowledge of the venous anatomy prior to the procedure aids in evaluating if the procedure is feasible and to minimize the procedure time (99). Cardiac venous anatomy can be evaluated by delaying the acquisition of a coronary CTA protocol. Variations in the venous anatomy, such as absence of venous tributaries may result in a wasted procedure. A recent study showed a lower prevalence of coronary venous tributaries draining the lateral LV wall, i.e., posterolateral and left marginal veins in ischemic cardiomyopathy (68% and 48%), which potentially hinders access for optimal positioning of LV lead and causes suboptimal CT response (97). Presence of scar in the lateral LV wall in the region of LV lead placement has been associated with reduced effectiveness of CRT due to lesser response to electrical stimulation (100,101). Although this scar is typically assessed using cardiac MRI or PET, DIE can also show this and helps in selecting appropriate patients. CT could also potentially be used prospectively in CRT planning to map areas of LV scarring.
Ablation techniques for atrial fibrillation involve interrupting abnormal pathways of conduction between the pulmonary vein ostia and the left atrium. CT is used in the pre-procedural evaluation for assessment of pulmonary venous and left atrial anatomy and left atrial thrombus. Pulmonary vein mapping CT is usually acquired with prospective ECG triggering, but non-ECG gated techniques including high pitch helical mode can be used to minimize the radiation dose (102). CT provides information on the pulmonary vein ostial anatomy, particularly in noting the location, size, anatomic variants and adjacent structures that may be affected by the ablation. The ostial measurements are necessary for selecting the optimal catheter size and have been shown to be comparable to MRI and superior to TEE (103,104). Pre-procedural CT has been shown to reduce the procedure time, increase the success of sinus rhythm restoration, and reduce arrhythmia recurrence (105,106). Furthermore, CT can also be used to evaluate the complications of ablation including pulmonary vein stenosis (107). Left atrial volume and narrow left atrial ridges especially about the appendage and between venous ostia are also important factors, which if ignored may lead to incomplete ablation lines or damage of veins resulting in stenosis. Presence of narrow ridges on pre-procedural imaging may subsequently alter the site of ablation (108). CT-derived left atrial volume has been shown to be superior to that of echocardiography in predicting the success of ablation (109). CT is also used in the detection of left atrial appendage thrombus, which is an absolute contraindication for ablation procedure. CT has a high negative predictive value (up to 98%), but modest specificity (85–88%) compared to TEE (108). It may be challenging to distinguish left atrial appendage thrombus from contrast admixture artifact. Thrombus and contrast admixture can be distinguished by measuring the attenuation (lower in thrombus), ratio of attenuation in LAA and aorta (lower in thrombus), iodine quantification with dual energy CT scanner (lower in thrombus), delayed phase acquisition (thrombus persists in delayed phase) or a split-bolus contrast injection protocol. Similarly, CT is also useful in evaluation of left atrial appendage anatomy and thrombus in patients with atrial fibrillation patients prior to undergoing atrial appendage occlusion device implantation (110).
Post-treatment evaluation
In patients with contraindications to MRI, CT is also a valuable imaging modality in the follow up of treatment. CT can provide accurate estimates of the ventricular volumes and function, which typically show improvement with several treatments. CT can also be used in the follow-up of procedures such as atrial ablation to evaluate for complications such as pulmonary venous stenosis, thrombosis and fistula with adjacent organs. CT is also useful in the evaluation of the position and complications of LVAD for patients with heart failure. Retrospective ECG gated CT can identify the position of the LV inflow and outflow cannulas. CT is also useful in the evaluation of other complications of LVAD including thrombus, hemorrhage, tamponade, aortic valve lesions, infection and fluid collections (111). CT has role in the evaluation of patients with heart transplants. Specifically, CT has been used in the setting of cardiac allograft vasculopathy (CAV), which is an accelerated form of CAD characterized by areas of fibrosis and intimal hyperplasia within transplant coronary vessels and is a major determinant in long-term survival in cardiac transplant patients (112). Traditional non-invasive imaging method such as echocardiography and nuclear medicine studies have been shown to be ineffective in evaluation of CAV (113). However, cardiac CT is a promising modality for the evaluation of CAV as it simultaneously evaluates coronary luminal irregularities as well as the coronary artery wall. CT has high sensitivity, specificity, and negative and positive predictive values in evaluation of CAV compared to both ICA and intravascular ultrasound (114,115).
Conclusions
Cardiac CT provides a comprehensive assessment of many facets of cardiomyopathies including characterization of the phenotype, evaluation of coronary arteries, quantification of function, treatment planning and post-treatment evaluation. CT is mostly utilized in cardiomyopathies in specific situations where echocardiography or MRI is not possible (due to contraindications) or suboptimal (due to poor windows in echo or artifacts in MRI).
Acknowledgements
None.
Footnote
Conflicts of Interest: The authors have no conflicts of interest to declare.
References
- Maron BJ, Towbin JA, Thiene G, et al. Contemporary definitions and classification of the cardiomyopathies: an American Heart Association Scientific Statement from the Council on Clinical Cardiology, Heart Failure and Transplantation Committee; Quality of Care and Outcomes Research and Functional Genomics and Translational Biology Interdisciplinary Working Groups; and Council on Epidemiology and Prevention. Circulation 2006;113:1807-16. [Crossref] [PubMed]
- Benjamin EJ, Blaha MJ, Chiuve SE, et al. Heart Disease and Stroke Statistics-2017 Update: A Report From the American Heart Association. Circulation 2017;135:e146-603. [Crossref] [PubMed]
- Baldasseroni S, Opasich C, Gorini M, et al. Left bundle-branch block is associated with increased 1-year sudden and total mortality rate in 5517 outpatients with congestive heart failure: a report from the Italian network on congestive heart failure. Am Heart J 2002;143:398-405. [Crossref] [PubMed]
- Quinones MA, Douglas PS, Foster E, et al. ACC/AHA clinical competence statement on echocardiography: a report of the American College of Cardiology/American Heart Association/American College of Physicians-American Society of Internal Medicine Task Force on Clinical Competence. J Am Coll Cardiol 2003;41:687-708. [PubMed]
- Matsuo S, Nakajima K, Kinuya S. Clinical use of nuclear cardiology in the assessment of heart failure. World J Cardiol 2010;2:344-56. [Crossref] [PubMed]
- Harinstein ME, Soman P. Radionuclide Imaging Applications in Cardiomyopathies and Heart Failure. Curr Cardiol Rep 2016;18:23. [Crossref] [PubMed]
- Matsuo S, Matsumoto T, Nakae I, et al. Prognostic value of ECG-gated thallium-201 single-photon emission tomography in patients with coronary artery disease. Ann Nucl Med 2004;18:617-22. [Crossref] [PubMed]
- Berman DS, Abidov A, Kang X, et al. Prognostic validation of a 17-segment score derived from a 20-segment score for myocardial perfusion SPECT interpretation. J Nucl Cardiol 2004;11:414-23. [Crossref] [PubMed]
- Danias PG, Ahlberg AW, Clark BA 3rd, et al. Combined assessment of myocardial perfusion and left ventricular function with exercise technetium-99m sestamibi gated single-photon emission computed tomography can differentiate between ischemic and nonischemic dilated cardiomyopathy. Am J Cardiol 1998;82:1253-8. [Crossref] [PubMed]
- Sciagra R, Berti V, Genovese S, et al. Reliability of myocardial perfusion gated SPECT for the reproducible evaluation of resting left ventricular functional parameters in long-term follow-up. Eur J Nucl Med Mol Imaging 2010;37:1722-9. [Crossref] [PubMed]
- Allman KC, Shaw LJ, Hachamovitch R, et al. Myocardial viability testing and impact of revascularization on prognosis in patients with coronary artery disease and left ventricular dysfunction: a meta-analysis. J Am Coll Cardiol 2002;39:1151-8. [Crossref] [PubMed]
- Bokhari S, Shahzad R, Castano A, et al. Nuclear imaging modalities for cardiac amyloidosis. J Nucl Cardiol 2014;21:175-84. [Crossref] [PubMed]
- Boogers MJ, Fukushima K, Bengel FM, et al. The role of nuclear imaging in the failing heart: myocardial blood flow, sympathetic innervation, and future applications. Heart Fail Rev 2011;16:411-23. [Crossref] [PubMed]
- Task Force M, Montalescot G, Sechtem U, et al. 2013 ESC guidelines on the management of stable coronary artery disease: the Task Force on the management of stable coronary artery disease of the European Society of Cardiology. Eur Heart J 2013;34:2949-3003. [Crossref] [PubMed]
- Tavakol M, Ashraf S, Brener SJ. Risks and complications of coronary angiography: a comprehensive review. Glob J Health Sci 2012;4:65-93. [PubMed]
- Pennell DJ, Sechtem UP, Higgins CB, et al. Clinical indications for cardiovascular magnetic resonance (CMR): Consensus Panel report. J Cardiovasc Magn Reson 2004;6:727-65. [Crossref] [PubMed]
- Pohost GM, Hung L, Doyle M. Clinical use of cardiovascular magnetic resonance. Circulation 2003;108:647-53. [Crossref] [PubMed]
- Coelho-Filho OR, Rickers C, Kwong RY, et al. MR myocardial perfusion imaging. Radiology 2013;266:701-15. [Crossref] [PubMed]
- Mahrholdt H, Klem I, Sechtem U. Cardiovascular MRI for detection of myocardial viability and ischaemia. Heart 2007;93:122-9. [Crossref] [PubMed]
- Mewton N, Liu CY, Croisille P, et al. Assessment of myocardial fibrosis with cardiovascular magnetic resonance. J Am Coll Cardiol 2011;57:891-903. [Crossref] [PubMed]
- Cummings KW, Bhalla S, Javidan-Nejad C, et al. A pattern-based approach to assessment of delayed enhancement in nonischemic cardiomyopathy at MR imaging. Radiographics 2009;29:89-103. [Crossref] [PubMed]
- Francois CJ, Schiebler ML, Reeder SB. Cardiac MRI evaluation of nonischemic cardiomyopathies. J Magn Reson Imaging 2010;31:518-30. [Crossref] [PubMed]
- Taylor AJ, Cerqueira M, Hodgson JM, et al. ACCF/SCCT/ACR/AHA/ASE/ASNC/NASCI/SCAI/SCMR 2010 Appropriate Use Criteria for Cardiac Computed Tomography. A Report of the American College of Cardiology Foundation Appropriate Use Criteria Task Force, the Society of Cardiovascular Computed Tomography, the American College of Radiology, the American Heart Association, the American Society of Echocardiography, the American Society of Nuclear Cardiology, the North American Society for Cardiovascular Imaging, the Society for Cardiovascular Angiography and Interventions, and the Society for Cardiovascular Magnetic Resonance. J Cardiovasc Comput Tomogr 2010;4:407.e1-33. [Crossref] [PubMed]
- Schuleri KH, George RT, Lardo AC. Applications of cardiac multidetector CT beyond coronary angiography. Nat Rev Cardiol 2009;6:699-710. [Crossref] [PubMed]
- Budoff MJ, Dowe D, Jollis JG, et al. Diagnostic performance of 64-multidetector row coronary computed tomographic angiography for evaluation of coronary artery stenosis in individuals without known coronary artery disease: results from the prospective multicenter ACCURACY (Assessment by Coronary Computed Tomographic Angiography of Individuals Undergoing Invasive Coronary Angiography) trial. J Am Coll Cardiol 2008;52:1724-32. [Crossref] [PubMed]
- Achenbach S, Marwan M, Schepis T, et al. High-pitch spiral acquisition: a new scan mode for coronary CT angiography. J Cardiovasc Comput Tomogr 2009;3:117-21. [Crossref] [PubMed]
- McCollough CH, Leng S, Yu L, et al. Dual- and Multi-Energy CT: Principles, Technical Approaches, and Clinical Applications. Radiology 2015;276:637-53. [Crossref] [PubMed]
- Lu MT, Cai T, Ersoy H, et al. Comparison of ECG-gated versus non-gated CT ventricular measurements in thirty patients with acute pulmonary embolism. Int J Cardiovasc Imaging 2009;25:101-7. [Crossref] [PubMed]
- Cademartiri F, Mollet NR, Runza G, et al. Diagnostic accuracy of multislice computed tomography coronary angiography is improved at low heart rates. Int J Cardiovasc Imaging 2006;22:101-5; discussion 107-9. [Crossref] [PubMed]
- Abbara S, Blanke P, Maroules CD, et al. SCCT guidelines for the performance and acquisition of coronary computed tomographic angiography: A report of the society of Cardiovascular Computed Tomography Guidelines Committee: Endorsed by the North American Society for Cardiovascular Imaging (NASCI). J Cardiovasc Comput Tomogr 2016;10:435-49. [Crossref] [PubMed]
- Weininger M, Barraza JM, Kemper CA, et al. Cardiothoracic CT angiography: current contrast medium delivery strategies. AJR Am J Roentgenol 2011;196:W260-72. [Crossref] [PubMed]
- Varga-Szemes A, Meinel FG, De Cecco CN, et al. CT myocardial perfusion imaging. AJR Am J Roentgenol 2015;204:487-97. [Crossref] [PubMed]
- Techasith T, Cury RC. Stress myocardial CT perfusion: an update and future perspective. JACC Cardiovasc Imaging 2011;4:905-16. [Crossref] [PubMed]
- Kang DK, Schoepf UJ, Bastarrika G, et al. Dual-energy computed tomography for integrative imaging of coronary artery disease: principles and clinical applications. Semin Ultrasound CT MR 2010;31:276-91. [Crossref] [PubMed]
- Kerl JM, Deseive S, Tandi C, et al. Dual energy CT for the assessment of reperfused chronic infarction - a feasibility study in a porcine model. Acta Radiol 2011;52:834-9. [Crossref] [PubMed]
- Deseive S, Bauer RW, Lehmann R, et al. Dual-energy computed tomography for the detection of late enhancement in reperfused chronic infarction: a comparison to magnetic resonance imaging and histopathology in a porcine model. Invest Radiol 2011;46:450-6. [Crossref] [PubMed]
- Lee HJ, Im DJ, Youn JC, et al. Myocardial Extracellular Volume Fraction with Dual-Energy Equilibrium Contrast-enhanced Cardiac CT in Nonischemic Cardiomyopathy: A Prospective Comparison with Cardiac MR Imaging. Radiology 2016;280:49-57. [Crossref] [PubMed]
- Buss SJ, Schulz F, Mereles D, et al. Quantitative analysis of left ventricular strain using cardiac computed tomography. Eur J Radiol 2014;83:e123-30. [Crossref] [PubMed]
- Truong QA, Singh JP, Cannon CP, et al. Quantitative analysis of intraventricular dyssynchrony using wall thickness by multidetector computed tomography. JACC Cardiovasc Imaging 2008;1:772-81. [Crossref] [PubMed]
- Sabarudin A, Sun Z. Coronary CT angiography: Dose reduction strategies. World J Cardiol 2013;5:465-72. [PubMed]
- Shuman WP, Branch KR, May JM, et al. Prospective versus retrospective ECG gating for 64-detector CT of the coronary arteries: comparison of image quality and patient radiation dose. Radiology 2008;248:431-7. [Crossref] [PubMed]
- Mayo JR, Leipsic JA. Radiation dose in cardiac CT. AJR Am J Roentgenol 2009;192:646-53. [Crossref] [PubMed]
- Hausleiter J, Meyer T, Hadamitzky M, et al. Radiation dose estimates from cardiac multislice computed tomography in daily practice: impact of different scanning protocols on effective dose estimates. Circulation 2006;113:1305-10. [Crossref] [PubMed]
- Hollingsworth CL, Yoshizumi TT, Frush DP, et al. Pediatric cardiac-gated CT angiography: assessment of radiation dose. AJR Am J Roentgenol 2007;189:12-8. [Crossref] [PubMed]
- Hausleiter J, Bischoff B, Hein F, et al. Feasibility of dual-source cardiac CT angiography with high-pitch scan protocols. J Cardiovasc Comput Tomogr 2009;3:236-42. [Crossref] [PubMed]
- Leschka S, Stolzmann P, Desbiolles L, et al. Diagnostic accuracy of high-pitch dual-source CT for the assessment of coronary stenoses: first experience. Eur Radiol 2009;19:2896-903. [Crossref] [PubMed]
- Leipsic J, Labounty TM, Heilbron B, et al. Adaptive statistical iterative reconstruction: assessment of image noise and image quality in coronary CT angiography. AJR Am J Roentgenol 2010;195:649-54. [Crossref] [PubMed]
- Lloyd-Jones DM, Larson MG, Leip EP, et al. Lifetime risk for developing congestive heart failure: the Framingham Heart Study. Circulation 2002;106:3068-72. [Crossref] [PubMed]
- Yancy CW, Jessup M, Bozkurt B, et al. 2013 ACCF/AHA guideline for the management of heart failure: a report of the American College of Cardiology Foundation/American Heart Association Task Force on Practice Guidelines. J Am Coll Cardiol 2013;62:e147-239. [Crossref] [PubMed]
- Mowatt G, Cook JA, Hillis GS, et al. 64-Slice computed tomography angiography in the diagnosis and assessment of coronary artery disease: systematic review and meta-analysis. Heart 2008;94:1386-93. [Crossref] [PubMed]
- Guo SL, Guo YM, Zhai YN, et al. Diagnostic accuracy of first generation dual-source computed tomography in the assessment of coronary artery disease: a meta-analysis from 24 studies. Int J Cardiovasc Imaging 2011;27:755-71. [Crossref] [PubMed]
- de Graaf FR, Schuijf JD, van Velzen JE, et al. Diagnostic accuracy of 320-row multidetector computed tomography coronary angiography in the non-invasive evaluation of significant coronary artery disease. Eur Heart J 2010;31:1908-15. [Crossref] [PubMed]
- Bhatti S, Hakeem A, Yousuf MA, et al. Diagnostic performance of computed tomography angiography for differentiating ischemic vs nonischemic cardiomyopathy. J Nucl Cardiol 2011;18:407-20. [Crossref] [PubMed]
- Patel MR, White RD, Abbara S, et al. 2013 ACCF/ACR/ASE/ASNC/SCCT/SCMR appropriate utilization of cardiovascular imaging in heart failure: a joint report of the American College of Radiology Appropriateness Criteria Committee and the American College of Cardiology Foundation Appropriate Use Criteria Task Force. J Am Coll Cardiol 2013;61:2207-31. [Crossref] [PubMed]
- Osawa K, Miyoshi T, Koyama Y, et al. Additional diagnostic value of first-pass myocardial perfusion imaging without stress when combined with 64-row detector coronary CT angiography in patients with coronary artery disease. Heart 2014;100:1008-15. [Crossref] [PubMed]
- Wang R, Yu W, Wang Y, et al. Incremental value of dual-energy CT to coronary CT angiography for the detection of significant coronary stenosis: comparison with quantitative coronary angiography and single photon emission computed tomography. Int J Cardiovasc Imaging 2011;27:647-56. [Crossref] [PubMed]
- Ko SM, Choi JW, Song MG, et al. Myocardial perfusion imaging using adenosine-induced stress dual-energy computed tomography of the heart: comparison with cardiac magnetic resonance imaging and conventional coronary angiography. Eur Radiol 2011;21:26-35. [Crossref] [PubMed]
- Ko SM, Park JH, Hwang HK, et al. Direct comparison of stress- and rest-dual-energy computed tomography for detection of myocardial perfusion defect. Int J Cardiovasc Imaging 2014;30 Suppl 1:41-53. [Crossref] [PubMed]
- Saremi F, Achenbach S. Coronary plaque characterization using CT. AJR Am J Roentgenol 2015;204:W249-60. [Crossref] [PubMed]
- Gerber BL, Belge B, Legros GJ, et al. Characterization of acute and chronic myocardial infarcts by multidetector computed tomography: comparison with contrast-enhanced magnetic resonance. Circulation 2006;113:823-33. [Crossref] [PubMed]
- Langer C, Lutz M, Eden M, et al. Hypertrophic cardiomyopathy in cardiac CT: a validation study on the detection of intramyocardial fibrosis in consecutive patients. Int J Cardiovasc Imaging 2014;30:659-67. [Crossref] [PubMed]
- Zhao L, Ma X, Feuchtner GM, et al. Quantification of myocardial delayed enhancement and wall thickness in hypertrophic cardiomyopathy: multidetector computed tomography versus magnetic resonance imaging. Eur J Radiol 2014;83:1778-85. [Crossref] [PubMed]
- Kellman P, Wilson JR, Xue H, et al. Extracellular volume fraction mapping in the myocardium, part 1: evaluation of an automated method. J Cardiovasc Magn Reson 2012;14:63. [Crossref] [PubMed]
- Nacif MS, Kawel N, Lee JJ, et al. Interstitial myocardial fibrosis assessed as extracellular volume fraction with low-radiation-dose cardiac CT. Radiology 2012;264:876-83. [Crossref] [PubMed]
- Nacif MS, Liu Y, Yao J, et al. 3D left ventricular extracellular volume fraction by low-radiation dose cardiac CT: assessment of interstitial myocardial fibrosis. J Cardiovasc Comput Tomogr 2013;7:51-7. [Crossref] [PubMed]
- Andreini D, Pontone G, Pepi M, et al. Diagnostic accuracy of multidetector computed tomography coronary angiography in patients with dilated cardiomyopathy. J Am Coll Cardiol 2007;49:2044-50. [Crossref] [PubMed]
- Green JJ, Berger JS, Kramer CM, et al. Prognostic value of late gadolinium enhancement in clinical outcomes for hypertrophic cardiomyopathy. JACC Cardiovasc Imaging 2012;5:370-7. [Crossref] [PubMed]
- Dambrin G, Laissy JP, Serfaty JM, et al. Diagnostic value of ECG-gated multidetector computed tomography in the early phase of suspected acute myocarditis. A preliminary comparative study with cardiac MRI. Eur Radiol 2007;17:331-8. [Crossref] [PubMed]
- Aikawa T, Oyama-Manabe N, Naya M, et al. Delayed contrast-enhanced computed tomography in patients with known or suspected cardiac sarcoidosis: A feasibility study. Eur Radiol 2017. [Epub ahead of print]. [Crossref] [PubMed]
- Marwan M, Pflederer T, Ropers D, et al. Cardiac amyloidosis imaged by dual-source computed tomography. J Cardiovasc Comput Tomogr 2008;2:403-5. [Crossref] [PubMed]
- Baughman RP, Teirstein AS, Judson MA, et al. Clinical characteristics of patients in a case control study of sarcoidosis. Am J Respir Crit Care Med 2001;164:1885-9. [Crossref] [PubMed]
- Patel MR, Cawley PJ, Heitner JF, et al. Detection of myocardial damage in patients with sarcoidosis. Circulation 2009;120:1969-77. [Crossref] [PubMed]
- Deux JF, Mihalache CI, Legou F, et al. Noninvasive detection of cardiac amyloidosis using delayed enhanced MDCT: a pilot study. Eur Radiol 2015;25:2291-7. [Crossref] [PubMed]
- Treibel TA, Bandula S, Fontana M, et al. Extracellular volume quantification by dynamic equilibrium cardiac computed tomography in cardiac amyloidosis. J Cardiovasc Comput Tomogr 2015;9:585-92. [Crossref] [PubMed]
- Sidhu MS, Uthamalingam S, Ahmed W, et al. Defining left ventricular noncompaction using cardiac computed tomography. J Thorac Imaging 2014;29:60-6. [Crossref] [PubMed]
- Zuccarino F, Vollmer I, Sanchez G, et al. Left ventricular noncompaction: imaging findings and diagnostic criteria. AJR Am J Roentgenol 2015;204:W519-30. [Crossref] [PubMed]
- Hussain J, Ghandforoush A, Virk Z, et al. Viability assessment by multidetector computed tomography in Takotsubo cardiomyopathy. J Thorac Imaging 2011;26:W7-8. [Crossref] [PubMed]
- Otalvaro L, Zambrano JP, Fishman JE. Takotsubo cardiomyopathy: utility of cardiac computed tomography angiography for acute diagnosis. J Thorac Imaging 2011;26:W83-5. [Crossref] [PubMed]
- Rizvi A, Deano RC, Bachman DP, et al. Analysis of ventricular function by CT. J Cardiovasc Comput Tomogr 2015;9:1-12. [Crossref] [PubMed]
- Marcus FI, McKenna WJ, Sherrill D, et al. Diagnosis of arrhythmogenic right ventricular cardiomyopathy/dysplasia: proposed modification of the Task Force Criteria. Eur Heart J 2010;31:806-14. [Crossref] [PubMed]
- Lessick J, Ghersin E, Abadi S, et al. Accuracy of the long-axis area-length method for the measurement of left ventricular volumes and ejection fraction using multidetector computed tomography. Can J Cardiol 2008;24:685-9. [Crossref] [PubMed]
- Sayyed SH, Cassidy MM, Hadi MA. Use of multidetector computed tomography for evaluation of global and regional left ventricular function. J Cardiovasc Comput Tomogr 2009;3:S23-34. [Crossref] [PubMed]
- Boogers MJ, van Werkhoven JM, Schuijf JD, et al. Feasibility of diastolic function assessment with cardiac CT: feasibility study in comparison with tissue Doppler imaging. JACC Cardiovasc Imaging 2011;4:246-56. [Crossref] [PubMed]
- Ko SM, Kim YJ, Park JH, et al. Assessment of left ventricular ejection fraction and regional wall motion with 64-slice multidetector CT: a comparison with two-dimensional transthoracic echocardiography. Br J Radiol 2010;83:28-34. [Crossref] [PubMed]
- Lim SJ, Choo KS, Park YH, et al. Assessment of left ventricular function and volume in patients undergoing 128-slice coronary CT angiography with ECG-based maximum tube current modulation: a comparison with echocardiography. Korean J Radiol 2011;12:156-62. [Crossref] [PubMed]
- Abadi S, Brook OR, Rispler S, et al. Hybrid cardiac SPECT/64-slice CTA-derived LV function parameters: correlation and reproducibility assessment. Eur J Radiol 2010;75:154-8. [Crossref] [PubMed]
- Greupner J, Zimmermann E, Grohmann A, et al. Head-to-head comparison of left ventricular function assessment with 64-row computed tomography, biplane left cineventriculography, and both 2- and 3-dimensional transthoracic echocardiography: comparison with magnetic resonance imaging as the reference standard. J Am Coll Cardiol 2012;59:1897-907. [Crossref] [PubMed]
- Asferg C, Usinger L, Kristensen TS, et al. Accuracy of multi-slice computed tomography for measurement of left ventricular ejection fraction compared with cardiac magnetic resonance imaging and two-dimensional transthoracic echocardiography: a systematic review and meta-analysis. Eur J Radiol 2012;81:e757-62. [Crossref] [PubMed]
- Singh RM, Singh BM, Mehta JL. Role of cardiac CTA in estimating left ventricular volumes and ejection fraction. World J Radiol 2014;6:669-76. [Crossref] [PubMed]
- Mahnken AH, Spuentrup E, Niethammer M, et al. Quantitative and qualitative assessment of left ventricular volume with ECG-gated multislice spiral CT: Value of different image reconstruction algorithms in comparison to MRI. Acta Radiologica 2003;44:604-11. [Crossref] [PubMed]
- Gopalan D. Right heart on multidetector CT. Br J Radiol 2011;84:S306-23. [Crossref] [PubMed]
- Raman SV, Shah M, McCarthy B, et al. Multi-detector row cardiac computed tomography accurately quantifies right and left ventricular size and function compared with cardiac magnetic resonance. Am Heart J 2006;151:736-44. [Crossref] [PubMed]
- Guo YK, Gao HL, Zhang XC, et al. Accuracy and reproducibility of assessing right ventricular function with 64-section multi-detector row CT: comparison with magnetic resonance imaging. Int J Cardiol 2010;139:254-62. [Crossref] [PubMed]
- Kuhl JT, Lonborg J, Fuchs A, et al. Assessment of left atrial volume and function: a comparative study between echocardiography, magnetic resonance imaging and multi slice computed tomography. Int J Cardiovasc Imaging 2012;28:1061-71. [Crossref] [PubMed]
- Agner BF, Kuhl JT, Linde JJ, et al. Assessment of left atrial volume and function in patients with permanent atrial fibrillation: comparison of cardiac magnetic resonance imaging, 320-slice multi-detector computed tomography, and transthoracic echocardiography. Eur Heart J Cardiovasc Imaging 2014;15:532-40. [Crossref] [PubMed]
- Habis M, Capderou A, Ghostine S, et al. Acute myocardial infarction early viability assessment by 64-slice computed tomography immediately after coronary angiography: comparison with low-dose dobutamine echocardiography. J Am Coll Cardiol 2007;49:1178-85. [Crossref] [PubMed]
- Boonyasirinant T, Halliburton SS, Schoenhagen P, et al. Absence of coronary sinus tributaries in ischemic cardiomyopathy: An insight from multidetector computed tomography cardiac venographic study. J Cardiovasc Comput Tomogr 2016;10:156-61. [Crossref] [PubMed]
- Singh JP, Houser S, Heist EK, et al. The coronary venous anatomy: a segmental approach to aid cardiac resynchronization therapy. J Am Coll Cardiol 2005;46:68-74. [Crossref] [PubMed]
- Catanzaro JN, Makaryus JN, Jadonath R, et al. Planning and guidance of cardiac resynchronization therapy-lead implantation by evaluating coronary venous anatomy assessed with multidetector computed tomography. Clin Med Insights Cardiol 2015;8:43-50. [PubMed]
- Derval N, Steendijk P, Gula LJ, et al. Optimizing hemodynamics in heart failure patients by systematic screening of left ventricular pacing sites: the lateral left ventricular wall and the coronary sinus are rarely the best sites. J Am Coll Cardiol 2010;55:566-75. [Crossref] [PubMed]
- Nam G, Schwartz J, Girsky MJ, et al. Utilization of Computed Tomography for Left Ventricular Lead Placement to Optimize Cardiac Resynchronization Therapy. J Clin Exp Cardiolog 2016;7:431.
- Thai WE, Wai B, Lin K, et al. Pulmonary venous anatomy imaging with low-dose, prospectively ECG-triggered, high-pitch 128-slice dual-source computed tomography. Circ Arrhythm Electrophysiol 2012;5:521-30. [Crossref] [PubMed]
- To AC, Gabriel RS, Park M, et al. Role of Transesophageal Echocardiography Compared to Computed Tomography in Evaluation of Pulmonary Vein Ablation for Atrial Fibrillation (ROTEA study). J Am Soc Echocardiogr 2011;24:1046-55. [Crossref] [PubMed]
- Hamdan A, Charalampos K, Roettgen R, et al. Magnetic resonance imaging versus computed tomography for characterization of pulmonary vein morphology before radiofrequency catheter ablation of atrial fibrillation. Am J Cardiol 2009;104:1540-6. [Crossref] [PubMed]
- Kistler PM, Rajappan K, Jahngir M, et al. The impact of CT image integration into an electroanatomic mapping system on clinical outcomes of catheter ablation of atrial fibrillation. J Cardiovasc Electrophysiol 2006;17:1093-101. [Crossref] [PubMed]
- Martinek M, Nesser HJ, Aichinger J, et al. Impact of integration of multislice computed tomography imaging into three-dimensional electroanatomic mapping on clinical outcomes, safety, and efficacy using radiofrequency ablation for atrial fibrillation. Pacing Clin Electrophysiol 2007;30:1215-23. [Crossref] [PubMed]
- Packer DL, Keelan P, Munger TM, et al. Clinical presentation, investigation, and management of pulmonary vein stenosis complicating ablation for atrial fibrillation. Circulation 2005;111:546-54. [Crossref] [PubMed]
- Thai WE, Wai B, Truong QA. Preprocedural imaging for patients with atrial fibrillation and heart failure. Curr Cardiol Rep 2012;14:584-92. [Crossref] [PubMed]
- Parikh SS, Jons C, McNitt S, et al. Predictive capability of left atrial size measured by CT, TEE, and TTE for recurrence of atrial fibrillation following radiofrequency catheter ablation. Pacing Clin Electrophysiol 2010;33:532-40. [Crossref] [PubMed]
- Kwong Y, Troupis J. Cardiac CT imaging in the context of left atrial appendage occlusion. J Cardiovasc Comput Tomogr 2015;9:13-8. [Crossref] [PubMed]
- Carr CM, Jacob J, Park SJ, et al. CT of Left Ventricular Assist Devices. RadioGraphics 2010;30:429-44. [Crossref] [PubMed]
- Schmauss D, Weis M. Cardiac allograft vasculopathy: recent developments. Circulation 2008;117:2131-41. [Crossref] [PubMed]
- Smart FW, Ballantyne CM, Cocanougher B, et al. Insensitivity of noninvasive tests to detect coronary artery vasculopathy after heart transplant. Am J Cardiol 1991;67:243-7. [Crossref] [PubMed]
- Wever-Pinzon O, Romero J, Kelesidis I, et al. Coronary computed tomography angiography for the detection of cardiac allograft vasculopathy: a meta-analysis of prospective trials. J Am Coll Cardiol 2014;63:1992-2004. [Crossref] [PubMed]
- Mittal TK, Panicker MG, Mitchell AG, et al. Cardiac allograft vasculopathy after heart transplantation: electrocardiographically gated cardiac CT angiography for assessment. Radiology 2013;268:374-81. [Crossref] [PubMed]