The role of advanced reconstruction algorithms in cardiac CT
Advanced non-linear iterative reconstruction (IR) algorithms have been introduced by all vendors as an alternative to traditional, linear filtered back projection (FBP) reconstruction (Table 1). FBP is a simple reconstruction technique that requires relatively little computational processing, but is associated with higher noise and poor contrast resolution. Noise can be reduced in FBP only by increasing the radiation dose. IR algorithms operate by initially generating an expected dataset based on modeling, which is then compared to the actual, acquired dataset. Noise is detected and removed by using the difference between the anticipated and actual datasets using a process of iterative steps. All commercial IR algorithms employ statistics-model based de-noising in the image domain, raw (projection) data domain, or both. Statistics model-based algorithms operate by assigning a lower value to data with higher statistical uncertainty (noise). Some IR algorithms, described as partial model-based, additionally employ models of scanner geometry to estimate the acquired data. Full model-based IR algorithms, employ complete system models that include simulation of X-ray interaction with the imaged object. Statistics-model based algorithms can be utilized to either improve image quality at the same radiation dose or maintain image quality at a lower radiation dose compared to FBP. However, combining dose management with image quality improvements simultaneously is limited. Model-based IR can provide image quality improvements at lower doses. Model-based algorithms are also more likely to reduce other artifacts in addition to noise as well as improve spatial resolution.
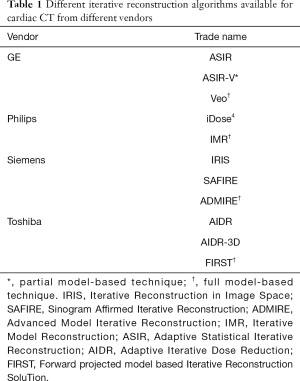
Full table
Although IR algorithms have been utilized in nuclear medicine for several decades, widespread use in CT is recent and facilitated by advances in computational processing. Cardiovascular CT imagers were early adopters of IR algorithms because most cardiovascular clinical targets are robust to changes in noise. Rigorous validation has come from both retrospective and prospective studies demonstrating non-inferiority of coronary CT data acquired at lower doses and reconstructed with IR techniques compared to standard dose data reconstructed with FBP. Other clinical studies have validated the diagnostic utility of low-dose coronary CT angiograms (CTA) reconstructed with IR algorithms using invasive coronary angiography (ICA) as a standard of reference (1-3).
In this article, we review various applications of IR techniques to cardiac imaging and illustrate how they have changed practice.
Clinical utility of iterative reconstruction
Decreased radiation dose
One of the important clinical utility of IR for cardiovascular CT is radiation dose reduction. Note IR algorithms do not directly lower dose but rather enable a lowering of tube current and/or tube potential by the user. IR may also be used in conjunction with other dose reduction tools such as anatomic based tube-current modulation, ECG-based tube current modulation, prospective ECG-triggered axial or high-pitch helical scanning. Multiple studies have demonstrated the ability of various IR algorithms to maintain image quality at lower doses. An early, prospective, multi-center study, the ERASIR study, found comparable image quality between CTA reconstructed with ASIR and FBP, with the best results at 40–60% ASIR and 44% radiation dose reduction (4). Other retrospective studies showed lower noise, equivalent image quality and dose savings of 24–54% for ASIR (5-7), 82% for VEO (8), 50% for AIDR-3D (5,9), 62% for IRIS (10), 52–80% for SAFIRE (11-14), 52% for ADMIRE (15,16), 39–63% for iDose4 (17-21) and 80% for IMR (22). FIRST showed 28% dose reduction compared to AIDR-3D (23). The PROTECTION V study, a large, prospective, multi-center, multi-vendor, randomized-control trial of 400 patients found that image quality of coronary CTA performed at 30% reduced tube current and reconstructed with multiple IR algorithms (ASIR, SAFIRE, iDose4, AIDR-3D) matched standard tube current coronary CTA reconstructed with FBP (24). Improved image quality for low-dose (sub-millisievert) coronary CTA was observed with a model-based algorithm, IMR, compared to standard FBP (25). Sub-millisievert CTAs were also observed in other studies, including one on a 320-detector row CT scanner with the use of AIDR-3D, automatic exposure control and fast gantry rotation time (26).
The utility of IR algorithms to reduced radiation exposure in pediatric patients for whom radiation dose is of a greater concern due to their higher radio sensitivity and longer life span has also been demonstrated. Several studies in children have shown that IR algorithms enable reduced radiation doses with maintained or improved image quality. CT studies in pediatric population, including those with congenital heart disease (CHD) have shown higher image quality, lower noise, and maintained diagnostic accuracy in ultra-low dose studies (using 70 kVp/half tube current/high pitch helical mode) reconstructed with SAFIRE or IRIS compared to FBP reconstructions, with up to 54% radiation dose savings and effective radiation doses <0.1 mSv (27-30). Other pediatric CT studies using ASIR demonstrated improved conspicuity of various pediatric cardiovascular structures in newborns and children compared to FBP with 36% dose reduction as long as ASIR levels were low (20–40%) (31); ASIR at higher (>50%) levels resulted in lower image quality with image conspicuity very poor due to noise free appearance of structures at 100% ASIR (31). The model-based algorithm IMR, showed improved detection of congenital anomalies in infants <3 months compared to FBP and iDose4, with pooled accuracy of 0.91 (0.84, 0.81 for iDose4 & FBP) and pooled sensitivity of 0.82 (0.67 and 0.58 for iDose4 & FBP) and fewer non-diagnostic studies (2 vs. 15 vs. 39 for IMR, iDose4, FBP) (32). However, in a separate study of infants with CHD, IMR provided superior image quality (noise, margin sharpness), but did not improve the diagnostic accuracy or visualization of coronary arteries beyond what was achieved with iDose4 (33).
IR techniques have also proven useful in simultaneously decreasing radiation and contrast burden. A prospective, multi-center, randomized coronary CTA trial demonstrated comparable image quality, noise, SNR, and CNR for SAFIRE images reconstructed from low tube voltage (100 kVp) and iodine concentration (270 mg/mL) data and FBP images reconstructed from high iodine concentration (370 mg/mL) and tube voltage (120 kVp) data (34). In a pediatric study on infants with complex CHD, good image quality and high diagnostic accuracy compared to surgery was demonstrated using 70% ASIR, with low tube potential (80 kVp) and low iodine concentration contrast agent (270 mg/mL) (35). Reconstruction of data obtained with low tube potential (80 kVp) and low contrast volume (35 mL at a flow rate of 3.5–4.5 mL/s) with IMR demonstrated a total radiation dose reduction of 52% and iodine delivery rate reduction of 52% for coronary CTA (36).
Improving image quality
Improvements in image quality at a given radiation dose can also be achieved with IR. In the evaluation of coronary arteries, iDose4 demonstrated improved visualization of distal vessels, grading of proximal vessels and CNR along with improved inter-observer agreement compared to FBP (18). The attenuation numbers were similar for FBP and IR algorithms at the same radiation dose (18). IMR showed an 88% reduction in intravascular noise and improved image quality compared to both standard FBP and iDose4 when radiation dose levels were unchanged (22). Improved image quality for the evaluation of coronary arterial plaques was seen with MBIR, compared to FBP for reconstruction of the same data (37). IR algorithms have also demonstrated improved image quality in specific settings such as obesity, heavy coronary artery calcification and stenting as described below. IR algorithms, particularly those operating partially or fully in the raw (projection) data domain may be used to reduce several artifacts (38). In a study of 50 patients referred for evaluation of the thoracic aorta, iDose4 reduced artifacts in the shoulder caused by photon starvation and improved image quality compared to FBP (38).
Cardiac CT applications of iterative reconstruction
Coronary artery stenosis assessment
Several studies have shown good image quality and accurate quantification of coronary stenosis using low radiation dose techniques enabled by IR (11). SAFIRE reconstruction of low radiation dose (<1 mSv) data had high diagnostic accuracy (Sensitivity, 96%; specificity, 94%) for detection of >50% stenosis in comparison with ICA (3). Another study comparing full dose FBP and half-dose SAFIRE images from the same patient showed better image quality, lower noise, and higher accuracy of stenosis detection with SAFIRE (accuracy, 97% vs. 94%; sensitivity, 100% vs. 100%; specificity 95% vs. 89%; NPV, 100 vs. 100%; PPV, 93% vs. 88% (1). Increasing strengths of SAFIRE (levels 1, 3, 5) were associated with progressively lower noise and increasing SNR and CNR. However, image quality and diagnostic accuracy at a per segment level peaked at level 3 (SAFIRE level 1, 94%; level 3 95%; level 5, 95%) due to a “plastic” appearance at level 5.
An important limitation of coronary artery stenosis assessment with CTA is low specificity in patients with significant calcium. Calcium blooming results in overestimation of luminal stenosis, which can lead to unnecessary invasive angiograms or stress tests. Calcium blooming can be reduced by creating high-energy virtual monoenergetic images if dual energy data are available or, possibly, by using higher tube potential (140 kVp). Higher resolution (aka, sharper) reconstruction kernels/filters can sometimes reduce calcium blooming, but at the cost of increased noise. Window settings in the image display can be also be adjusted to reduce the appearance of calcium blooming.
The results of IR on assessment of coronary arteries with extensive calcifications suggest decreased calcium blooming with IR (Figure 1). In one study of patients with Agatston calcium scores >400, IRIS provided lower noise, improved image quality, lower calcium volume and improved diagnostic accuracy in stenosis detection compared to FBP (accuracy, 96% vs. 92%; specificity, 96% vs. 91%; PPV, 77% vs. 61%) (39). Using SAFIRE 3 images, nine coronary arterial segments containing severe calcification (out of 126 segments in 36 patients with stenosis of 50% of greater) were reclassified from false positive in FBP images to true negative with ICA as reference standard, suggesting decreased calcium blooming with IR (2). However, another study revealed that the accuracy of coronary artery assessment decreases with increasing calcium content even with IR. Coronary CTAs reconstructed using iDose4 demonstrated good overall image quality and diagnostic accuracy but accuracy was lower in patients with a greater calcium burden (CAC >400) than those with a lower burden when ICA was used as a reference standard (specificity, 92% vs. 98%, PPV, 76% vs. 87%; accuracy, 92% vs. 97%) (40).
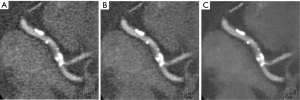
Coronary plaque evaluation
In addition to stenosis evaluation and quantification, assessment of plaque in the coronary artery wall is a major advantage of CT compared to luminography techniques and functional imaging. Plaque burden and composition have significant prognostic and predictive value. Plaque features such as positive remodeling, lipid rich necrotic core, thin fibrous cap, spotty calcification and napkin-ring sign are high risk features of vulnerable plaque that predict future acute coronary syndrome (41). An ex vivo study of three human hearts showed improved image quality for coronary artery plaque evaluation with lower noise for Veo than ASIR and FBP (37). A separate study demonstrated no change in plaque volume measured from Veo, ASIR, and FBP images in comparison to IVUS measurements (42). There was a strong correlation between CTA and IVUS but with overestimation of smaller, eccentric and calcified plaques with all CT image types.
Clinical evaluation of coronary plaques with statistics-model based iterative reconstruction techniques typically shows improvements in image quality but no change in quantitative metrics. In a comparison study with FBP, multiple levels of iDose4 showed objective image quality improvements but no change in plaque volume, plaque composition lipid/fibrous/calcified components, or luminal area (43). Similar results were obtained with multiple levels of ASIR showing no impact on plaque volume, plaque composition (soft, intermediate, calcified), luminal diameter or luminal stenosis; however, with higher levels of ASIR, the number of plaque components between 401–500 HU decreased significantly (44).
Model-based reconstruction yielded different results. Patient images reconstructed with Veo showed lower plaque volume and burden compared to ASIR images (45). A similar study showed IMR yielded lower overall plaque volume, particularly for calcified and high-attenuation non-calcified (90–129 HU) plaques, compared to FBP and iDose4 (46). This result was attributed to the higher spatial resolution of IMR and improved delineation of calcified plaques. Further, Veo CT images showed increased sensitivity for the detection of lipid core (plaque attenuation <60 HU) compared to ASIR and FBP (47) with histology as the gold standard.
In total, the evaluation of coronary plaque seems minimally affected by noise improvements characteristic of reconstruction techniques employing only statistical models but positively impacted by spatial resolution improvements inherent in techniques additionally employing full system models. Model-based IR may also offer workflow advantages for plaque evaluation. An ex vivo study of three human hearts demonstrated Veo facilitates automated plaque assessment by reducing the need for vessel-wall boundary corrections compared to ASIR and FBP, particularly at the site of moderate or severe calcifications (48).
Coronary stent assessment
Imaging coronary stents is also challenging due to blooming and subsequent underestimation of luminal size and overestimation of in-stent stenosis. This is more relevant in stents <3.0 mm and high density metal alloys such as gold or tantalum rather than magnesium or cobalt-chromium alloys (49). Current solutions are the same as those for reducing calcium blooming. Multiple phantom and human studies using IR algorithms (ASIR, IRIS, iDose4) in combination with sharper kernels/filters that improve spatial resolution have shown lower noise, improved image quality, decreased stent-strut volume, improved intra-stent luminal area and diameter visualization and improved detection of in-stent restenosis compared to FBP algorithms (10,50-53) (Figure 2). A high resolution protocol using ASIR at 40% and 60% showed improvement in image quality and in-stent luminal area and diameter (50). Low-dose imaging (0.32 mSv) with SAFIRE reconstruction demonstrated improved image quality and accuracy in the detection of in-stent restenosis with ICA as the reference standard (sensitivity, 100% vs. 85%; specificity, 75% vs. 69%; PPV, 44% vs. 32%; NPV, 100% vs. 96%) (54).
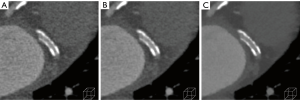
Calcium quantification
Coronary calcium scoring is used in clinical cardiology for risk stratification, particularly in the intermediate risk population, due to its ability to predict future cardiovascular events. Although the clinical utility of IR has been established for many cardiovascular CT imaging tasks, appropriate use has not been extended to calcium scoring. Reconstruction of coronary calcium scan data with non-linear IR algorithms enables reduced dose scanning and/or reduced noise, but has wider implications on diagnostic utility due to the known impact of these algorithms on calcium quantification. Some studies show good correlation between calcium scores derived from standard dose FBP and low dose IR techniques (iDose4, IRIS, SAFIRE) (55,56), with reclassification seen in only <3% of individuals (56). Multiple other studies have demonstrated a reduction in Agatston, volume and, to a lesser extent, mass scores quantified from IR versus FBP images (57-59). Lower calcium values with ASIR compared to FBP resulted in reclassification of one-third of patients to a lower risk category with an additional 13% of patients moving from a non-zero calcium score to a calcium score of zero (58). Increasing levels of ASIR were associated with progressive decreases in Agatston and volume scores but not mass scores compared to FBP (60). Studies with IRIS and SAFIRE showed similar results: Agatston, volume, and mass scores were lower changing non-zero scores to zero in 4% of patients (61). Similarly, iDose4 and IMR images each yielded 7% lower calcium score compared to FBP images with a risk reclassification rate of 2.4% (57).
A study of ex vivo human hearts in an anthropomorphic phantom using multiple vendors IR solutions (SAFIRE, iDose4, ASIR, AIDR-3D) at lower doses compared to FBP, showed no significant difference in calcium scores with FBP at radiation doses as much as 80% lower than normal; however, for IR algorithms, there was a trend towards lower calcium scores and volumes with minimal reclassification, with variable influence on mass and no effect on reproducibility (59). The impact of IR algorithms on calcium scoring stems from their de-noising effects. Lower noise results in fewer pixels with attenuation above the standard 130 HU threshold applied to calcium scoring images to identify calcium. This tends to lower all scores since fewer pixels are counted as calcium and partial volume averaging is reduced; the impact is less on mass scoring which is inherently less sensitive to partial volume averaging. For those pixel values above 130 HU, the reduced contribution from noise leads to fewer higher attenuation pixels. The consequence is further lowering of Agatston scores since computation is based on weighting factors that increase with pixel attenuation.
It is possible that calcium quantification from IR images is more accurate than quantification from FBP images as some studies suggest (57), but since the clinical value of an individual patient’s score rests on comparison to historical data in age, gender, and race-matched patients, such a change in practice needs more thorough investigation and community consensus. Having IR algorithms is not always necessary for calcium scoring.
Non-coronary calcifications in chest
Although coronary artery calcium quantification may be compromised with IR, quantification of larger cardiovascular calcifications may be adequate. Thoracic and aortic valve calcifications, in addition to coronary calcifications, are associated with coronary artery disease and stroke (62). Aortic calcium poses a particular threat to patients undergoing cardiac surgery. Post-operative strokes are seen in 1.4–9.7% of these patients, mainly as a result of emboli provoked by atherothrombotic material and calcifications displaced from the aortic wall during manipulation and clamping of the aorta.
Studies suggest aortic calcium can be reliably quantified from low dose CT reconstructed with IR techniques. Changes in the appearance and quantification of aortic valve calcification and thoracic aortic calcification on images reconstructed from sub-mSv CT data with multiple IR techniques were found to be minor (62). Cardiac CT at 0.35 mSv (60% dose reduction) at all iDose4 levels and chest CT at 0.48 mSv (75% dose reduction) at iDose4 levels 4 and 6 and IMR levels 1 and 2 showed comparable image quality to full dose FBP reconstructions, with equivalent calcium mass scores and false positive/false negative studies in only 1 of 28 patients (62). Another study also found no significant difference in aortic calcification (thoracic aortic calcium + aortic valve calcium) score or mass score at low dose (50% lower mAs) iDose4 level 7 images compared to standard dose iDose4 level 3 images; however, there was significantly lower calcium volume at low dose studies (55). Mass scores have been suggested to be more reproducible than Agatston scores and less influenced by IR at lower doses (62). These results are promising for aortic calcium quantification in the setting of low dose CT. This could include quantification from low dose, lung cancer screening chest CT or low dose, pre-op screening for the primary purpose of assessing aortic calcium. A prospective, multicenter randomized clinical trial, the CRICKET study, is ongoing to evaluate if non-contrast chest CT before cardiac surgery can decrease post-operative stroke rate, change surgical approach based on CT findings and cost effectiveness, as compared to conventional chest X-ray (63).
Obese patients
Obtaining good quality CT angiograms is challenging in obese/overweight individuals due to excessive image noise which results in poor delineation of small, distal coronary arteries and non-calcified plaques. There is an increase in non-evaluable segments (2.4% vs. 1.4%) and lower per-patient specificity in diagnostic accuracy of CAD (84% vs. 87%) in patients with a BMI >26 vs. <26 kg/m2 (64). The approach to improving image quality in larger patients is typically to increase tube output (tube current and/or tube potential) as well as increase the volume and/or flow rate of intravenous contrast. The result is increased radiation and contrast dose but all too often still insufficient image quality. IR algorithms provide an additional tool for improving image quality in these patients by decreasing image noise at a given dose level (Figure 3). Higher levels of IR are sometimes used for obese compared to non-obese patients. In a study of obese patients, image quality, noise, SNR and CNR were maintained in low radiation dose (50% reduced) images reconstructed using SAFIRE, compared to full dose FBP images (65). Another study showed a low radiation dose, low contrast dose protocol is feasible even in obese patients (BMI >30 kg/m2) using AIDR; image quality was maintained compared to standard radiation and contrast dose protocols (56% radiation dose, 23% iodine intake and 24% iodine injection rate decrease) (66). Note that radiation dose savings relative to FBP progressively decreases with increasing BMI because of the need to improve image quality (rather than maintain) for the heaviest patients (67).
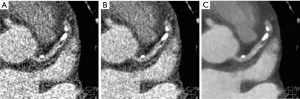
Achieving sufficient image quality in obese patients is particularly challenging with techniques that improve spatial resolution since these inherently increase noise. A high in-plane spatial resolution (230 µm) CT ASIR protocol showed improved image quality in obese patients with visualization of distal coronary arterial branches and comparable noise, SNR, CNR and radiation dose compared to standard resolution FBP protocol (68).
Other applications
Several studies have demonstrated the utility of IR algorithms for other cardiovascular CT applications. In a phantom study of four different prosthetic heart valves, lower radiation dose protocols using iDose4 showed comparable image quality, noise and artifacts compared to a full-dose protocol using FBP (69). Improved diagnostic quality and accuracy was observed in CT perfusion studies using IR (70,71). In a myocardial perfusion patient study using regadenoson, improved image quality and thus 3D quantification of myocardial perfusion (compared with perfusion abnormalities predicted by coronary CTA stenosis >50%) was seen with iDose4 compared to FBP (70) (Figure 4). In a porcine dynamic myocardial perfusion study, iDose4 yielded improved image quality (noise, CNR, SNR) compared to FBP when data for reconstruction was acquired with the same radiation dose and equivalent image quality when data for IR were acquired at a lower dose (71).
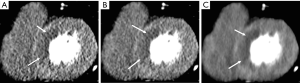
Compared to FBP and iDose4, IMR has demonstrated improved image quality and higher diagnostic performance of late iodine enhancement images for detecting MI (72). Late iodine enhancement images reconstructed with IMR also showed high sensitivity for detecting cardiac sarcoidosis compared to MRI (73). IMR also showed utility for epicardial fat quantification which is sensitive to image noise; IMR demonstrated improved image quality and reduced inter-observer variability for epicardial fat quantification (74). Veo has been used successfully in combination with low radiation dose protocols in patients for pulmonary vein isolation (75,76).
Challenges and limitations
Some challenges and limitations exist for the use of IR algorithms in cardiovascular CT practice. Image reconstruction times can be significantly longer for some IR algorithms, especially model-based algorithms; however, reconstruction times have progressively decreased over the years with improvements in computational power and processing times are not a major limiting issue in the current generation systems. An often-heard complaint about IR is that the images look “different”, “artificial”, “funny”, “plasticky”, “blotchy”, “pixilated”, or “waxy”, especially at higher IR levels (77-79). This artificial-look with smooth borders is due to lower noise levels and different noise textures (visual representation of noise). This requires adjustment and may limit diagnostic confidence of inexperienced observers; however, with time and more objective data illustrating the equivalency/superiority of IR, adoption is expected to increase.
Successful deployment of IR algorithms requires a balance between reducing radiation dose and maintaining diagnostic image quality. Implementation of IR algorithms should be approached in a stepwise manner. Initially IR algorithms should be applied at the lowest levels to data acquired using an institution’s standard doses while cardiologists/radiologists become accustomed to the new look. Dose can be lowered gradually while maintaining the IR level and the level can be progressively increased, maintaining image quality throughout the stepwise process. It should be recognized that the appropriate combination of dose and IR level is dependent on patient size (65). Although there is a growing body of evidence to support the utility of IR algorithms for cardiovascular CT, conclusions from phantom or clinical investigation of a specific algorithm should not be extended to other algorithms, even algorithms from the same manufacturer, because of algorithm-dependent differences in noise patterns and artifacts in reconstructed images.
Conclusions
Iterative reconstruction algorithms by multiple CT vendors are now routinely utilized in clinical cardiovascular CT. These algorithms have several applications with the primary goal to enable lower radiation dose protocols by reducing image noise. Iterative reconstruction techniques, particularly model-based techniques, are also useful in improving image quality and decreasing some artifacts. Calcium scores derived from IR images should not be relied on clinically per current standards because of the likelihood of risk reclassification. Implementation of IR algorithms should be accomplished gradually through a stepwise process.
Acknowledgements
None.
Footnote
Conflicts of Interest: Dr. SS Halliburton is employee of Philips Healthcare. The other authors have no conflicts of interest to declare.
References
- Moscariello A, Takx RA, Schoepf UJ, et al. Coronary CT angiography: Image quality, diagnostic accuracy, and potential for radiation dose reduction using a novel iterative image reconstruction technique-comparison with traditional filtered back projection. Eur Radiol 2011;21:2130-8. [Crossref] [PubMed]
- Wang R, Schoepf UJ, Wu R, et al. Diagnostic accuracy of coronary CT angiography: Comparison of filtered back projection and iterative reconstruction with different strengths. J Comput Assist Tomogr 2014;38:179-84. [Crossref] [PubMed]
- Yin WH, Lu B, Hou ZH, et al. Detection of coronary artery stenosis with sub-milliSievert radiation dose by prospectively ECG-triggered high-pitch spiral CT angiography and iterative reconstruction. Eur Radiol 2013;23:2927-33. [Crossref] [PubMed]
- Leipsic J, LaBounty TM, Heilbron B, et al. Estimated radiation dose reduction using adaptive statistical iterative reconstruction in coronary CT angiography: The ERASIR study. AJR Am J Roentgenol 2010;195:655-60. [Crossref] [PubMed]
- Williams MC, Weir NW, Mirsadraee S, et al. Iterative reconstruction and individualized automatic tube current selection reduce radiation dose while maintaining image quality in 320-multidetector computed tomography coronary angiography. Clin Radiol 2013;68:e570-7. [Crossref] [PubMed]
- Tumur O, Soon K, Brown F, et al. New scanning technique using Adaptive Statistical Iterative Reconstruction (ASIR) significantly reduced the radiation dose of cardiac CT. J Med Imaging Radiat Oncol 2013;57:292-6. [Crossref] [PubMed]
- Gosling O, Loader R, Venables P, et al. A comparison of radiation doses between state-of-the-art multislice CT coronary angiography with iterative reconstruction, multislice CT coronary angiography with standard filtered back-projection and invasive diagnostic coronary angiography. Heart 2010;96:922-6. [Crossref] [PubMed]
- Fuchs TA, Stehli J, Bull S, et al. Coronary computed tomography angiography with model-based iterative reconstruction using a radiation exposure similar to chest X-ray examination. Eur Heart J 2014;35:1131-6. [Crossref] [PubMed]
- Tomizawa N, Nojo T, Akahane M, et al. AdaptiveIterative Dose Reduction in coronary CT angiography using 320-row CT: assessment of radiation dose reduction and image quality. J Cardiovasc Comput Tomogr 2012;6:318-24. [Crossref] [PubMed]
- Renker M, Ramachandra A, Schoepf UJ, et al. Iterative image reconstruction techniques: Applications for cardiac CT. J Cardiovasc Comput Tomogr 2011;5:225-30. [Crossref] [PubMed]
- Yin WH, Lu B, Li N, et al. Iterative reconstruction to preserve image quality and diagnostic accuracy at reduced radiation dose in coronary CT angiography: An intraindividual comparison. JACC Cardiovasc Imaging 2013;6:1239-49. [Crossref] [PubMed]
- Takx RA, Schoepf UJ, Moscariello A, et al. Coronary CT angiography: Comparison of a novel iterative reconstruction with filtered back projection for reconstruction of low-dose CT - Initial experience. Eur J Radiol 2013;82:275-80. [Crossref] [PubMed]
- Wang R, Schoepf UJ, Wu R, et al. CT coronary angiography: Image quality with sinogram-affirmed iterative reconstruction compared with filtered back-projection. Clin Radiol 2013;68:272-8. [Crossref] [PubMed]
- Layritz C, Schmid J, Achenbach S, et al. Accuracy of prospectively ECG-triggered very low-dose coronary dual-source CT angiography using iterative reconstruction for the detection of coronary artery stenosis: Comparison with invasive catheterization. Eur Heart J Cardiovasc Imaging 2014;15:1238-45. [Crossref] [PubMed]
- Hell MM, Bittner D, Schuhbaeck A, et al. Prospectively ECG-triggered high-pitch coronary angiography with third-generation dual-source CT at 70 kVp tube voltage: Feasibility, image quality, radiation dose, and effect of iterative reconstruction. J Cardiovasc Comput Tomogr 2014;8:418-25. [Crossref] [PubMed]
- Meyer M, Haubenreisser H, Schoepf UJ, et al. Radiation Dose Levels of Retrospectively ECG-Gated Coronary CT Angiography Using 70-kVp Tube Voltage in Patients with High or Irregular Heart Rates. Acad Radiol 2017;24:30-7. [Crossref] [PubMed]
- Hou Y, Zheng J, Wang Y, et al. Optimizing radiation dose levels in prospectively electrocardiogram-triggered coronary computed tomography angiography using iterative reconstruction techniques: a phantom and patient study. PLoS One 2013;8:e56295. [Crossref] [PubMed]
- Utsunomiya D, Weigold WG, Weissman G, et al. Effect of hybrid iterative reconstruction technique on quantitative and qualitative image analysis at 256-slice prospective gating cardiac CT. Eur Radiol 2012;22:1287-94. [Crossref] [PubMed]
- Carrascosa P, Rodriguez-Granillo GA, Capunay C, et al. Low-dose CT coronary angiography using iterative reconstruction with a 256-slice CT scanner. World J Cardiol 2013;5:382-6. [PubMed]
- Hou Y, Liu X, Xv S, et al. Comparisons of image quality and radiation dose between iterative reconstruction and filtered back projection reconstruction algorithms in 256-MDCT coronary angiography. AJR Am J Roentgenol 2012;199:588-94. [Crossref] [PubMed]
- Laqmani A, Buhk JH, Henes FO, et al. Impact of a 4th generation iterative reconstruction technique on image quality in low-dose computed tomography of the chest in immunocompromised patients. Rofo 2013;185:749-57. [Crossref] [PubMed]
- Halpern EJ, Gingold EL, White H, et al. Evaluation of Coronary Artery Image Quality with Knowledge-based Iterative Model Reconstruction. Acad Radiol 2014;21:805-11. [Crossref] [PubMed]
- Maeda E, Tomizawa N, Kanno S, et al. The feasibility of Forward-projected model-based Iterative Reconstruction SoluTion (FIRST) for coronary 320-row computed tomography angiography: A pilot study. J Cardiovasc Comput Tomogr 2017;11:40-5. [Crossref] [PubMed]
- Deseive S, Chen MY, Korosoglou G, et al. Prospective Randomized Trial on Radiation Dose Estimates of CT Angiography Applying Iterative Image Reconstruction: The PROTECTION V Study. JACC Cardiovasc Imaging 2015;8:888-96. [Crossref] [PubMed]
- Iyama Y, Nakaura T, Kidoh M, et al. Submillisievert Radiation Dose Coronary CT Angiography: Clinical Impact of the Knowledge-Based Iterative Model Reconstruction. Acad Radiol 2016;23:1393-401. [Crossref] [PubMed]
- Chen MY, Shanbhag SM, Arai AE. Submillisievert median radiation dose for coronary angiography with a second-generation 320-detector row CT scanner in 107 consecutive patients. Radiology 2013;267:76-85. [Crossref] [PubMed]
- Zheng M, Zhao H, Xu J, et al. Image quality of ultra-low-dose dual-source CT angiography using high-pitch spiral acquisition and iterative reconstruction in young children with congenital heart disease. J Cardiovasc Comput Tomogr 2013;7:376-82. [Crossref] [PubMed]
- Han BK, Grant KL, Garberich R, et al. Assessment of an iterative reconstruction algorithm (SAFIRE) on image quality in pediatric cardiac CT datasets. J Cardiovasc Comput Tomogr 2012;6:200-4. [Crossref] [PubMed]
- Nie P, Li H, Duan Y, et al. Impact of sinogram affirmed iterative reconstruction (SAFIRE) algorithm on image quality with 70 kVp-tube-voltage dual-source CT angiography in children with congenital heart disease. PLoS One 2014;9:e91123. [Crossref] [PubMed]
- Tricarico F, Hlavacek AM, Schoepf UJ, et al. Cardiovascular CT angiography in neonates and children: image quality and potential for radiation dose reduction with iterative image reconstruction techniques. Eur Radiol 2013;23:1306-15. [Crossref] [PubMed]
- Mieville FA, Gudinchet F, Rizzo E, et al. Paediatric cardiac CT examinations: impact of the iterative reconstruction method ASIR on image quality--preliminary findings. Pediatr Radiol 2011;41:1154-64. [Crossref] [PubMed]
- Kligerman S, Bolster F, Mitchell J, et al. Use of Model-based Iterative Reconstruction to Improve Detection of Congenital Cardiovascular Anomalies in Infants Undergoing Free-breathing Computed Tomographic Angiography. J Thorac Imaging 2017;32:127-35. [Crossref] [PubMed]
- Jia Q, Zhuang J, Jiang J, et al. Image quality of ct angiography using model-based iterative reconstruction in infants with congenital heart disease: Comparison with filtered back projection and hybrid iterative reconstruction. Eur J Radiol 2017;86:190-7. [Crossref] [PubMed]
- Yin WH, Lu B, Gao JB, et al. Effect of reduced x-ray tube voltage, low iodine concentration contrast medium, and sinogram-affirmed iterative reconstruction on image quality and radiation dose at coronary CT angiography: Results of the prospective multicenter REALISE trial. J Cardiovasc Comput Tomogr 2015;9:215-24. [Crossref] [PubMed]
- Wang SY, Gao W, Zhong YM, et al. Prospective ECG-triggering cardiac CT for infants with complex congenital heart disease using low-dose contrast medium, low tube voltage, and adaptive statistical iterative reconstruction. Clin Radiol 2017;72:502-7. [Crossref] [PubMed]
- Zhang F, Yang L, Song X, et al. Feasibility study of low tube voltage (80 kVp) coronary CT angiography combined with contrast medium reduction using iterative model reconstruction (IMR) on standard BMI patients. Br J Radiol 2016;89:20150766. [Crossref] [PubMed]
- Scheffel H, Stolzmann P, Schlett CL, et al. Coronary artery plaques: Cardiac CT with model-based and adaptive-statistical iterative reconstruction technique. Eur J Radiol 2012;81:e363-9. [Crossref] [PubMed]
- Rajiah P, Schoenhagen P, Mehta D, et al. Low-dose, wide-detector array thoracic aortic CT angiography using an iterative reconstruction technique results in improved image quality with lower noise and fewer artifacts. J Cardiovasc Comput Tomogr 2012;6:205-13. [Crossref] [PubMed]
- Renker M, Nance JW Jr, Schoepf UJ, et al. Evaluation of heavily calcified vessels with coronary CT angiography: Comparison of iterative and filtered back projection image reconstruction. Radiology 2011;260:390-9. [Crossref] [PubMed]
- Hou Y, Ma Y, Fan W, et al. Diagnostic accuracy of low-dose 256-slice multi-detector coronary CT angiography using iterative reconstruction in patients with suspected coronary artery disease. Eur Radiol 2014;24:3-11. [Crossref] [PubMed]
- Motoyama S, Sarai M, Harigaya H, et al. Computed tomographic angiography characteristics of atherosclerotic plaques subsequently resulting in acute coronary syndrome. J Am Coll Cardiol 2009;54:49-57. [Crossref] [PubMed]
- Stolzmann P, Schlett CL, Maurovich-Horvat P, et al. Variability and accuracy of coronary CT angiography including use of iterative reconstruction algorithms for plaque burden assessment as compared with intravascular ultrasound-an ex vivo study. Eur Radiol 2012;22:2067-75. [Crossref] [PubMed]
- Takx RA, Willemink MJ, Nathoe HM, et al. The effect of iterative reconstruction on quantitative computed tomography assessment of coronary plaque composition. Int J Cardiovasc Imaging 2014;30:155-63. [Crossref] [PubMed]
- Fuchs TA, Fiechter M, Gebhard C, et al. CT coronary angiography: Impact of adapted statistical iterative reconstruction (ASIR) on coronary stenosis and plaque composition analysis. Int J Cardiovasc Imaging 2013;29:719-24. [Crossref] [PubMed]
- Precht H, Kitslaar PH, Broersen A, et al. First experiences with model based iterative reconstructions influence on quantitative plaque volume and intensity measurements in coronary computed tomography angiography. Radiography (Lond) 2017;23:77-9. [Crossref] [PubMed]
- Karolyi M, Szilveszter B, Kolossvary M, et al. Iterative model reconstruction reduces calcified plaque volume in coronary CT angiography. Eur J Radiol 2017;87:83-9. [Crossref] [PubMed]
- Puchner SB, Ferencik M, Maurovich-Horvat P, et al. Iterative image reconstruction algorithms in coronary CT angiography improve the detection of lipid-core plaque--a comparison with histology. Eur Radiol 2015;25:15-23. [Crossref] [PubMed]
- Puchner SB, Ferencik M, Karolyi M, et al. The effect of iterative image reconstruction algorithms on the feasibility of automated plaque assessment in coronary CT angiography. Int J Cardiovasc Imaging 2013;29:1879-88. [Crossref] [PubMed]
- Kerl JM, Schoepf UJ, Vogl TJ, et al. In vitro evaluation of metallic coronary artery stents with 64-MDCT using an ECG-gated cardiac phantom: relationship between in-stent visualization, stent type, and heart rate. AJR Am J Roentgenol 2010;194:W256-62. [Crossref] [PubMed]
- Gebhard C, Fiechter M, Fuchs TA, et al. Coronary artery stents: Influence of adaptive statistical iterative reconstruction on image quality using 64-HDCT. Eur Heart J Cardiovasc Imaging 2013;14:969-77. [Crossref] [PubMed]
- Zhou Q, Jiang B, Dong F, et al. Computed tomography coronary stent imaging with iterative reconstruction: A trade-off study between medium kernel and sharp kernel. J Comput Assist Tomogr 2014;38:604-12. [Crossref] [PubMed]
- Funama Y, Oda S, Utsunomiya D, et al. Coronary Artery Stent Evaluation by Combining Iterative Reconstruction and High-resolution Kernel at Coronary CT Angiography. Acad Radiol 2012;19:1324-31. [Crossref] [PubMed]
- Oda S, Utsunomiya D, Funama Y, et al. Improved coronary in-stent visualization using a combined high-resolution kernel and a hybrid iterative reconstruction technique at 256-slice cardiac CT - Pilot study. Eur J Radiol 2013;82:288-95. [Crossref] [PubMed]
- Eisentopf J, Achenbach S, Ulzheimer S, et al. Low-dose dual-source CT angiography with iterative reconstruction for coronary artery stent evaluation. JACC Cardiovasc Imaging 2013;6:458-65. [Crossref] [PubMed]
- Hecht HS, de Siqueira ME, Cham M, et al. Low- vs. standard-dose coronary artery calcium scanning. Eur Heart J Cardiovasc Imaging 2015;16:358-63. [Crossref] [PubMed]
- Schindler A, Vliegenthart R, Schoepf UJ, et al. Iterative image reconstruction techniques for CT coronary artery calcium quantification: Comparison with traditional filtered back projection in vitro and in vivo. Radiology 2014;270:387-93. [Crossref] [PubMed]
- Szilveszter B, Elzomor H, Karolyi M, et al. The effect of iterative model reconstruction on coronary artery calcium quantification. Int J Cardiovasc Imaging 2016;32:153-60. [Crossref] [PubMed]
- Van Osch JA, Mouden M, Van Dalen JA, et al. Influence of iterative image reconstruction on CT-based calcium score measurements. Int J Cardiovasc Imaging 2014;30:961-7. [PubMed]
- Willemink MJ, Takx RA, De Jong PA, et al. The impact of CT radiation dose reduction and iterative reconstruction algorithms from four different vendors on coronary calcium scoring. Eur Radiol 2014;24:2201-12. [Crossref] [PubMed]
- Gebhard C, Fiechter M, Fuchs TA, et al. Coronary artery calcium scoring: Influence of adaptive statistical iterative reconstruction using 64-MDCT. Int J Cardiol 2013;167:2932-7. [Crossref] [PubMed]
- Kurata A, Dharampal A, Dedic A, et al. Impact of iterative reconstruction on CT coronary calcium quantification. Eur Radiol 2013;23:3246-52. [Crossref] [PubMed]
- van Hamersvelt RW, den Harder AM, Willemink MJ, et al. Aortic Valve and Thoracic Aortic Calcification Measurements: How Low Can We Go in Radiation Dose? J Comput Assist Tomogr 2017;41:148-55. [Crossref] [PubMed]
- den Harder AM, de Heer LM, Maurovich-Horvat P, et al. Ultra low-dose chest ct with iterative reconstructions as an alternative to conventional chest x-ray prior to heart surgery (CRICKET study): Rationale and design of a multicenter randomized trial. J Cardiovasc Comput Tomogr 2016;10:242-5. [Crossref] [PubMed]
- Alkadhi H, Scheffel H, Desbiolles L, et al. Dual-source computed tomography coronary angiography: influence of obesity, calcium load, and heart rate on diagnostic accuracy. Eur Heart J 2008;29:766-76. [Crossref] [PubMed]
- Wang R, Schoepf UJ, Wu R, et al. Image quality and radiation dose of low dose coronary CT angiography in obese patients: sinogram affirmed iterative reconstruction versus filtered back projection. Eur J Radiol 2012;81:3141-5. [Crossref] [PubMed]
- Pan YN, Li AJ, Chen XM, et al. Coronary Computed Tomographic Angiography at Low Concentration of Contrast Agent and Low Tube Voltage in Patients with Obesity:: A Feasibility Study. Acad Radiol 2016;23:438-45. [Crossref] [PubMed]
- Hara AK, Paden RG, Silva AC, et al. Iterative reconstruction technique for reducing body radiation dose at CT: feasibility study. AJR Am J Roentgenol 2009;193:764-71. [Crossref] [PubMed]
- Gebhard C, Fuchs TA, Fiechter M, et al. Image quality of low-dose CCTA in obese patients: impact of high-definition computed tomography and adaptive statistical iterative reconstruction. Int J Cardiovasc Imaging 2013;29:1565-74. [Crossref] [PubMed]
- Habets J, Symersky P, de Mol BA, et al. A novel iterative reconstruction algorithm allows reduced dose multidetector-row CT imaging of mechanical prosthetic heart valves. Int J Cardiovasc Imaging 2012;28:1567-75. [Crossref] [PubMed]
- Bhave NM, Mor-Avi V, Kachenoura N, et al. Analysis of myocardial perfusion from vasodilator stress computed tomography: does improvement in image quality by iterative reconstruction lead to improved diagnostic accuracy? J Cardiovasc Comput Tomogr 2014;8:238-45. [Crossref] [PubMed]
- Gramer BM, Muenzel D, Leber V, et al. Impact of iterative reconstruction on CNR and SNR in dynamic myocardial perfusion imaging in an animal model. Eur Radiol 2012;22:2654-61. [Crossref] [PubMed]
- Tanabe Y, Kido T, Kurata A, et al. Impact of knowledge-based iterative model reconstruction on myocardial late iodine enhancement in computed tomography and comparison with cardiac magnetic resonance. Int J Cardiovasc Imaging 2017. [Epub ahead of print]. [Crossref] [PubMed]
- Aikawa T, Oyama-Manabe N, Naya M, et al. Delayed contrast-enhanced computed tomography in patients with known or suspected cardiac sarcoidosis: A feasibility study. Eur Radiol 2017. [Epub ahead of print]. [Crossref] [PubMed]
- Oda S, Utsunomiya D, Funama Y, et al. Effect of iterative reconstruction on variability and reproducibility of epicardial fat volume quantification by cardiac CT. J Cardiovasc Comput Tomogr 2016;10:150-5. [Crossref] [PubMed]
- Fahlenkamp UL, Diaz Ramirez I, Wagner M, et al. Image quality of low-radiation dose left atrial CT using filtered back projection and an iterative reconstruction algorithm: intra-individual comparison in unselected patients undergoing pulmonary vein isolation. Acta Radiol 2017.284185117708472. [Epub ahead of print]. [PubMed]
- Annoni AD, Andreini D, Pontone G, et al. Ultra-low-dose CT for left atrium and pulmonary veins imaging using new model-based iterative reconstruction algorithm. Eur Heart J Cardiovasc Imaging 2015;16:1366-73. [Crossref] [PubMed]
- Geyer LL, Glenn GR, De Cecco CN, et al. CT Evaluation of Small-Diameter Coronary Artery Stents: Effect of an Integrated Circuit Detector with Iterative Reconstruction. Radiology 2015;276:706-14. [Crossref] [PubMed]
- Marin D, Nelson RC, Schindera ST, et al. Low-tube-voltage, high-tube-current multidetector abdominal CT: improved image quality and decreased radiation dose with adaptive statistical iterative reconstruction algorithm--initial clinical experience. Radiology 2010;254:145-53. [Crossref] [PubMed]
- Singh S, Kalra MK, Hsieh J, et al. Abdominal CT: comparison of adaptive statistical iterative and filtered back projection reconstruction techniques. Radiology 2010;257:373-83. [Crossref] [PubMed]