Deep vein thrombosis: pathogenesis, diagnosis, and medical management
Introduction
Deep vein thrombosis (DVT), a subset of venous thromboembolism (VTE), is a major preventable cause of morbidity and mortality worldwide. The incidence of VTE is estimated to be 1 per 1,000 people annually (1,2), with DVT accounting for approximately two-thirds of these events (3). Pulmonary embolism (PE), a dreaded complication of DVT, occurs in up to one-third of cases and is the primary contributor to mortality (4). Much of the morbidity of DVT results from the development of post-thrombotic syndrome, which occurs in up to 50% of patients within 2 years of DVT and encompasses a number of symptoms including leg pain, swelling, and in severe cases, venous ulcers (5,6). Anticoagulation is the mainstay of therapy for DVT, with the goal of preventing progression to PE and recurrence of thrombosis. The 30-day mortality rate exceeds 3% in patients with DVT who are not anticoagulated, and this mortality risk increases 10-fold in patients who develop PE (7). The advent of direct oral anticoagulants (DOACs) has generated a need to compare these newer agents with the more conventional vitamin K-antagonists (VKAs) for the treatment of DVT. Several recent clinical trials have addressed this question and demonstrated a similar safety and efficacy profile between the two drug classes. With more therapeutic options, clinicians are now better able to incorporate disease- and patient-specific considerations into the medical management of DVT.
Pathogenesis
Virchow’s Triad, first described in 1856, implicates three contributing factors in the formation of thrombosis: venous stasis, vascular injury, and hypercoagulability. Venous stasis is the most consequential of the three factors, but stasis alone appears to be insufficient to cause thrombus formation (8). However, the concurrent presence of venous stasis and vascular injury or hypercoagulability greatly increases the risk for clot formation (9). The clinical conditions most closely associated with DVT are fundamentally related to the elements of Virchow’s Triad; these include surgery or trauma, malignancy, prolonged immobility, pregnancy, congestive heart failure, varicose veins, obesity, advancing age, and a history of DVT (10).
Venous thrombosis tends to occur in areas with decreased or mechanically altered blood flow such as the pockets adjacent to valves in the deep veins of the leg (11). While valves help to promote blood flow through the venous circulation, they are also potential locations for venous stasis and hypoxia. Multiple postmortem studies have demonstrated the propensity for venous thrombi to form in the sinuses adjacent to venous valves (12-14). As blood flow slows, oxygen tension declines with a coincident increase in hematocrit (15). The hypercoagulable micro-environment that ensues may downregulate certain antithrombotic proteins that are preferentially expressed on venous valves including thrombomodulin and endothelial protein C receptor (EPCR) (16). In addition to reducing important anticoagulant proteins, hypoxia drives the expression of certain procoagulants. Among these is P-selectin, an adhesion molecule which attracts immunologic cells containing tissue factor to the endothelium (17,18). Debate remains regarding the precise location of tissue factor in this process, whether expressed on the endothelium or by cells within the extravascular tissue, but there is general agreement that tissue factor serves as the primary nidus for thrombus formation (19). Thrombus formation appears to require both tissue factor and P-selectin (17,18).
A venous thrombus has essentially two components, an inner platelet rich white thrombus forming the so-called lines of Zahn surrounded by an outer red cell dense fibrin clot (12). Fibrin and extracellular DNA complexed with histone proteins forms the outer scaffold, which may be important in determining thrombus susceptibility to tissue plasminogen activator (TPA) and thrombolysis (20). As the ratio of procoagulants to anticoagulants increases, so does the risk of thrombus formation. The proportion of proteins is in part determined by the ratio of endothelial cell surface to blood volume. A decreased cell surface to blood volume ratio (i.e., large vessels) favors procoagulants (21). Factor VIII, von Willebrand factor, factor VII and prothrombin seem to be particularly influential in tipping the scale towards coagulation (22). In addition to promoting thrombin generation, prothrombin inhibits the anticoagulant properties of activated protein C, thereby dampening a natural anticoagulant pathway. There are three such pathways: the protein C anticoagulant pathway (protein C, protein S, thrombomodulin, and perhaps EPCR), heparin-antithrombin pathway, and tissue factor inhibitor pathway. Defects in these pathways are associated with an increased risk for thrombus formation. In humans, less is known regarding the role of tissue factor inhibitor pathway (19,22,23). There are also a number of familial variants that predispose to thrombus formation by increasing the levels of factor VII, VIII, IX, von Willebrand factor, and prothrombin. In factor V Leiden, which affects up to 5% of Caucasians and increases the risk of thrombosis 7-fold, activated factor Va is resistant to the inhibitory influence of protein C (19). Other risk factors for clot formation include cancer, oral contraceptives, obesity, and advancing age. Malignancy can exert a compressive effect on veins contributing to stasis. It also leads to shedding of procoagulants such as tissue factor on membrane particles that promotes thrombosis (24). Obesity and oral contraceptive use are independent risk factors for thrombosis. Together, they increase thrombosis risk synergistically (25). Finally, advancing age is associated with an increased risk for thrombosis. While the cause for this remains unsettled, several factors related to aging have been observed: greater prevalence of obesity, increased frequency of illness and periods of prolonged immobility, comorbid medical conditions, and an increase in the level of procoagulants without a commensurate increase in anticoagulants such as protein C (19). Taken together, thrombosis formation is a dynamic, multicausal process that hinges on a fine balance of physical and biochemical factors.
Diagnosis
The clinical presentation of DVT varies with the extent and location of a thrombus. The cardinal signs and symptoms of DVT include asymmetrical swelling, warmth, or pain in an extremity, and a high index of suspicion should be present in patients with the aforementioned risk factors. A number of scoring systems have been devised to estimate the pre-test probability of DVT. In the United States, the most widely used scoring system is the Wells criteria (26). In its original form, patients were stratified into three categories, high, intermediate, or low risk, based on the presence or absence of 9 clinical criteria. DVT prevalence was estimated to be 5% and 53% in the low- and high-risk groups, respectively (27). Several years later, the Wells scoring system was revised to include a “previously documented DVT” criterion and extend the post-operative duration from 4 to 12 weeks. The risk categories were also trimmed to “unlikely” or “likely”, with the prevalence of DVT estimated to be 6% and 28%, respectively (28). In 2012, the National Institute for Health and Care Excellence (NICE) guidelines estimated the sensitivity and specificity for DVT of the Wells criteria to be 77–98% and 38–58%, respectively (29). While the sensitivity of the Wells criteria can be quite high, it is clear from this data that the scoring system cannot be used as the sole diagnostic modality for DVT. Nevertheless, it is clinically useful in stratifying patients and determining the most appropriate sequence for further testing.
Like the Wells scoring criteria, the D-dimer assay has a high sensitivity and relatively lower specificity for the diagnosis of DVT, estimated by the NICE guidelines to be 75–100% and 26–83%, respectively (29). The test measures D-dimer, a prominent fibrin degradation product, which is generated by the fibrinolytic response to thrombus formation in the body. Elevation in D-dimer is not unique to thrombosis however, as it can be increased in a variety of pathologic states including malignancy, inflammatory conditions, pregnancy, and liver disease. It is also increased during the post-operative period and after trauma. Given its high sensitivity, the D-dimer assay can help to rule out DVT in low-risk patients, particularly when combined with the Wells scoring criteria or ultrasound (US).
Diagnostic imaging is often employed to confirm the presence of DVT. US is the first-line imaging modality for diagnosis of proximal DVT because it is safe, easily accessible, cost-effective, and reliable (30-32). It can accurately determine the size, chronicity, and degree of occlusion of a thrombus and therefore better inform the decision to pursue medical management or interventional techniques. During the examination, an US probe is used to gently compress the vein of interest. Inability to compress the vein is considered diagnostic for DVT. The clot can be further characterized with real-time imaging such as duplex and color-flow Doppler (Figure 1). The primary limitation of US is its diminished ability to detect distal DVT. A meta-analysis by Goodacre et al. estimated the pooled sensitivity of US for proximal and distal DVT to be 94.2% and 63.5%, respectively (33). However, proximal compressive US examinations are often favored over whole-leg US in the clinical setting because distal DVT rarely results in clinically significant sequelae (34). US is augmented by the Wells scoring criteria and D-dimer assay. In patients with a negative D-dimer who are “unlikely” to have DVT, US is not necessary. In similarly stratified patients with a positive D-dimer, US is recommended. Finally, in patients with a comorbid condition associated with an elevated D-dimer, US is preferred in place of D-dimer (34). Other diagnostic imaging modalities used for DVT include conventional contrast venography, computed tomography (CT) venography, and magnetic resonance (MR) venography. Contrast venography is the gold standard for lower extremity DVT, but it is limited by a number of factors including availability, patient discomfort, user-dependence, inadequate visualization, and patient-specific variables such as contrast allergy and renal insufficiency (32,34). The exam is performed by cannulating a dorsal vein in the foot and applying a compression tourniquet to the proximal thigh. Contrast media is injected and serial radiographs are taken to visualize the deep venous system of the leg. A persistent filling defect in multiple views is considered diagnostic for DVT (35) (Figure 2). In CT venography, contrast media is injected into the arm and imaging is timed with opacification of the deep venous system in the lower extremities (Figure 3). The exam is non-invasive, readily available, highly sensitive and specific for DVT, and provides the added benefit of cross-sectional imaging. It may be particularly useful for identifying proximal DVT in patients with suspected PE (36). Like conventional venography, it carries the same exposure to ionizing radiation and contrast media and is limited by renal insufficiency and severe contrast allergy. MR venography provides many of the same benefits as CT venography without the need for ionizing radiation. It has a similar sensitivity and specificity for DVT (37) (Figure 4) . In addition, a variety of pulse sequences can be applied to visualize the deep venous system without the need for contrast media. The disadvantages of MR venography are similar to other MR exams, namely patient intolerability, increased cost, and incompatible hardware. Although not yet well studied, MR venography is becoming an increasingly viable option when US is not feasible in cases of suspected DVT (37).
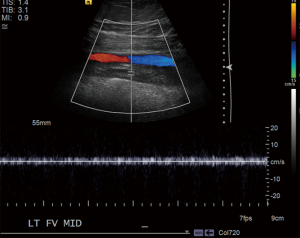
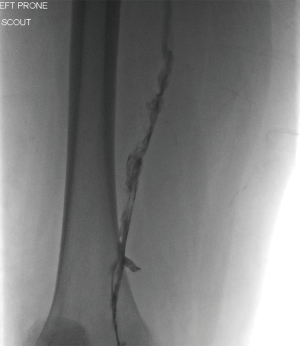
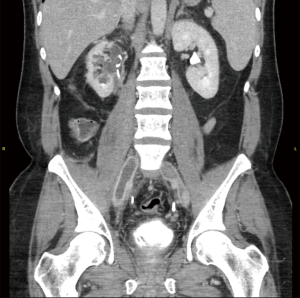
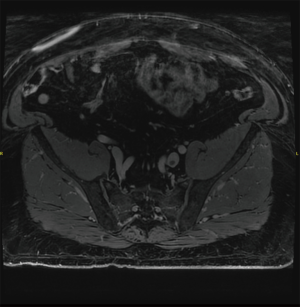
Medical management
Anticoagulation is an essential component of therapy for DVT. With a few notable exceptions, patients with DVT can be treated with oral anticoagulants alone. In cases of extensive thrombus burden involving proximal deep veins, mechanical- and catheter-directed thrombolysis (CDT) may be indicated in the acute phase to rapidly induce clot lysis and reduce the risk of post-thrombotic syndrome (38,39). These techniques are also being employed for the treatment of acute limb ischemia secondary to arterial thrombosis, although there is an increased risk for ischemia-reperfusion injury (40,41). However, thrombolytic therapy is associated with an increased risk of major bleeding and has shown no mortality benefit in patients with DVT (42-45). Further studies are underway to determine the proper patient selection and potential short- and long-term benefits of CDT as compared to systemic thrombolysis and/or anticoagulation therapy (45). In patients with an increased risk of bleeding or absolute contraindication to anticoagulation therapy, an inferior vena cava filter can be placed to prevent progression to PE.
Unfractionated heparin (UFH)/low molecular weight heparin (LMWH)
During the acute phase, which corresponds to the first 5–10 days of therapy, UFH or LMWH is utilized as a bridging agent when a VKA is planned. Rapid initiation of treatment helps to curb fibrin clot formation and augment the body’s fibrinolytic response, thereby reducing symptoms and risk of further thrombus formation or progression to PE. These agents are particularly useful in the hospital setting given their short half-life and relative convenience for perioperative management. UFH has several advantages over LMWH; it has a shorter elimination half-life (~1 hour); it is fully reversible, and it is preferred in patients with body mass index (BMI) >40 kg/m2 or weight <50 kg, or those who have creatinine clearance <30 mL/min (46). However, optimal therapeutic levels are difficult to achieve given substantial differences in dosing requirements among individuals (47), and UFH carries an 8–10-fold increased risk for heparin-induced thrombocytopenia (HIT) when compared to LMWH (48). For these reasons, LMWH such as enoxaparin is often the bridging therapy of choice. Fondaparinux, a synthetic pentasaccharide, can also be used for bridging purposes. Similar to UFH and LMWH, its anticoagulant effect is mediated through activation of antithrombin III, but it is selective for factor Xa and has no affinity for PF-4, conferring a substantial reduction in incidence of HIT. In clinical practice, fondaparinux is limited by its long half-life (17–21 hours with normal renal function) and lack of a reversal agent (48,49). Once therapeutic levels are achieved, as determined by activated partial thromboplastin time (aPTT) or anti-Xa levels, VKA therapy should begin. Parenteral anticoagulation with UFH or LMWH should be continued for at least 5 days and until the international normalized ratio (INR) is sustained >2 for 24 hours. In most circumstances, LMWH/VKA therapy is recommended for at least 3 months and can safely be completed on an outpatient basis (46,49-52).
VKAs versus DOACs
DOACs are an attractive alternative to VKAs such as warfarin for a number of reasons; they have fewer drug-drug interactions; they can be taken orally and in some cases do not require bridging; they do not require frequent laboratory monitoring, and they have been shown to be as effective as VKA therapy for DVT (46,50,52) (Table 1). The primary disadvantages of DOACs relate to their long half-life, making them less suitable for inpatient treatment and also contraindicated in patients with poor liver and/or renal function. Also, DOACs have not been well studied in all patient populations and therefore are not recommended in cases of active malignancy, thrombocytopenia, or high bleeding risk (46,50).
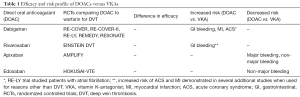
Full table
Dabigatran
The effectiveness of dabigatran for treatment of DVT has been confirmed in three recent double-blind randomized controlled trials: RE-COVER, REMEDY, and RESONATE. In the RE-COVER trial, more than 2,500 patients with documented proximal DVT were assigned to either dabigatran or standard LMWH/warfarin for the duration of therapy. There was no difference in mortality, major bleeding, acute coronary events, or recurrence of VTE (53). These results were confirmed in the RE-COVER II trial, with pooled data from both studies showing a hazard ratio of recurrent VTE of 1.09 (95% CI, 0.76–1.57) and major bleeding of 0.73 (95% CI, 0.48–1.11) (52). In the RE-LY study, there did appear to be an increased risk of gastrointestinal (GI) bleeding in patients with atrial fibrillation who were treated with dabigatran as compared to warfarin (54). This risk was not corroborated by the RE-COVER, RE-COVER II, or REMEDY trials, likely owing to differences in the patient population between the studies. In particular, patients with atrial fibrillation tend to be older, have other comorbid conditions, and are more likely to be on concomitant anti-platelet agents such as aspirin or Plavix. In a large meta-analysis that included the RE-LY and RE-COVER trials, as well as several other studies comparing the efficacy of dabigatran to warfarin for stroke prophylaxis, acute coronary syndrome, and DVT prophylaxis in joint replacement, dabigatran was associated with an increased risk of myocardial infarction or acute coronary syndrome with a pooled odds ratio of 1.33 (95% CI, 1.03–1.77) (55,56). Taken together, dabigatran appears to be as effective as warfarin in the short- and long-term treatment for DVT. Until further studies are conducted, caution should be used when prescribing dabigatran to elderly patients with atrial fibrillation or other cardiac risk factors.
Rivaroxaban
Rivaroxaban, a direct factor Xa inhibitor, has been shown to be as effective as warfarin for DVT in randomized controlled trials. In the EINSTEIN DVT trial, patients were randomized to either rivaroxaban or LMWH/VKA therapy within 48 hours of diagnosis. The hazard ratio for recurrent VTE was 0.68 (95% CI, 0.44–1.04), major bleeding was 0.65 (95% CI, 0.33–1.30), and non-major bleeding was 0.97 (95% CI, 0.76–1.22) (57). The EINSTEIN PE trial yielded similar results in terms of safety when rivaroxaban was compared to warfarin for treatment of symptomatic PE (58). However, based on a recent meta-analysis of 12 randomized controlled trials (RCTs) and a population-based cohort study, rivaroxaban does appear to be associated with an increased risk of GI bleeding in patients over the age of 75 (59,60). Therefore, it should be used judiciously in these patients.
Apixaban
Apixaban was compared to warfarin therapy for DVT in the AMPLIFY trial. Patients were randomized to either apixaban or LMWH/VKA therapy within 36 hours of diagnosis. There was no statistical difference in all-cause mortality or recurrent VTE. Major bleeding and clinically-relevant non-major bleeding was lower in the apixaban group compared to the warfarin group with a relative risk of 0.31 (95% CI, 0.17–0.55) and 0.44 (95% CI, 0.36–0.55), respectively (61). Apixaban has not been associated with an increased risk of GI bleeding or acute coronary syndrome in other studies (44,62). Therefore, apixaban is an attractive alternative to conventional therapy for DVT in appropriately selected patients.
Edoxaban
The HOKUSAI-VTE study compared edoxaban, an oral direct thrombin inhibitor, to warfarin for treatment of VTE. This large, randomized double-blind non-inferiority study included more than 8,200 patients from 37 countries across the world. Patients with DVT or PE were randomized to edoxaban or warfarin treatment arms after a median of 7 days of parenteral anticoagulant therapy. The hazard ratio for edoxaban (60 mg once daily) compared to warfarin for recurrent VTE was 0.89 (95% CI, 0.70–1.13), major bleeding was 0.84 (95% CI, 0.59–1.21), and clinically-relevant non-major bleeding was 0.81 (95% CI, 0.71–0.94). Similar to apixaban, there was no difference in all-cause mortality or major bleeding, and edoxaban was associated with a decreased risk of clinically-relevant non-major bleeding compared to warfarin (63). Based on these study results, edoxaban appears to be as effective as warfarin with a lower risk of non-major bleeding after an initial 5–10-day course of parenteral anticoagulant therapy.
Summary
DVT is a prevalent and vexing problem for clinicians. Normal blood physiology allows for coagulation in the appropriate setting, but a variety of disease states can alter the balance of pro- and anti-coagulant factors leading to pathologic thrombus formation. DVT is diagnosed with increasing precision using the Wells criteria, D-dimer assay, and an expanding array of imaging modalities including US, CT, and MR venography. The treatment of DVT has traditionally included VKAs such as warfarin with heparin or fractionated heparin bridging (47,50-52). With the arrival of DOACs came hope for more therapeutic options for DVT, but the safety and efficacy profile of these newer agents compared to conventional therapy has been of paramount importance. After more than a decade of research, multiple large-scale clinical trials have demonstrated comparable efficacy between the two drug classes (46,50,52,57,58,61,63). Although the safety profile for DOACs has been quite favorable, dabigatran and rivaroxaban have been associated with an increased risk of GI bleeding in select patients (54,59,60). Conversely, apixaban and edoxaban were associated with a lower risk of non-major bleeding in the AMPLIFY and HOKUSAI-VTE trials, respectively (61,63). In patients receiving dabigatran for other reasons including stroke and DVT prophylaxis, as well as acute coronary syndrome (ACS), there may be an increased risk of myocardial infarction (55,56). Taken together, DOACs are as effective as warfarin for treatment of DVT. DOACs should be used with caution in select patient populations including those of advanced age, with atrial fibrillation or other cardiac risk factors, or who have an increased risk of GI bleeding.
Acknowledgements
Funding: R Oklu gratefully acknowledges funding from the National Institutes of Health (EB021148, CA172738, EB024403, HL137193) and the Mayo Clinic.
Footnote
Conflicts of Interest: The authors have no conflicts of interest to declare.
References
- Beckman MG, Hooper WC, Critchley SE, et al. Venous thromboembolism: a public health concern. Am J Prev Med 2010;38:S495-501. [Crossref] [PubMed]
- White RH. The epidemiology of venous thromboembolism. Circulation 2003;107:I4-8. [Crossref] [PubMed]
- Silverstein MD, Heit JA, Mohr DN, et al. Trends in the incidence of deep vein thrombosis and pulmonary embolism: a 25-year population-based study. Arch Intern Med 1998;158:585-93. [Crossref] [PubMed]
- Kearon C. Natural history of venous thromboembolism. Circulation 2003;107:I22-30. [Crossref] [PubMed]
- Galanaud JP, Kahn SR. Postthrombotic syndrome: a 2014 update. Curr Opin Cardiol 2014;29:514-9. [Crossref] [PubMed]
- Kahn SR, Shrier I, Julian JA, et al. Determinants and time course of the postthrombotic syndrome after acute deep venous thrombosis. Ann Intern Med 2008;149:698-707. [Crossref] [PubMed]
- Søgaard KK, Schmidt M, Pedersen L, et al. 30-year mortality after venous thromboembolism: a population-based cohort study. Circulation 2014;130:829-36. [Crossref] [PubMed]
- Wessler S, Reimer SM, Sheps MC. Biologic assay of a thrombosis-inducing activity in human serum. J Appl Physiol 1959;14:943-6. [PubMed]
- Kumar DR, Hanlin E, Glurich I, et al. Virchow's contribution to the understanding of thrombosis and cellular biology. Clin Med Res 2010;8:168-72. [Crossref] [PubMed]
- Mammen EF. Pathogenesis of venous thrombosis. Chest 1992;102:640S-4S. [Crossref] [PubMed]
- Nicolaides AN, Kakkar VV, Field ES, et al. The origin of deep vein thrombosis: a venographic study. Br J Radiol 1971;44:653-63. [Crossref] [PubMed]
- Sevitt S. The structure and growth of valve-pocket thrombi in femoral veins. J Clin Pathol 1974;27:517-28. [Crossref] [PubMed]
- Lund F, Diener L, Ericsson JL. Postmortem intraosseous phlebography as an aid in studies of venous thromboembolism. With application on a geriatric clientele. Angiology 1969;20:155-76. [Crossref] [PubMed]
- Paterson JC, McLachlin J. Precipitating factors in venous thrombosis. Surg Gynecol Obstet 1954;98:96-102. [PubMed]
- Hamer JD, Malone PC, Silver IA. The PO2 in venous valve pockets: its possible bearing on thrombogenesis. Br J Surg 1981;68:166-70. [Crossref] [PubMed]
- Brooks EG, Trotman W, Wadsworth MP, et al. Valves of the deep venous system: an overlooked risk factor. Blood 2009;114:1276-9. [Crossref] [PubMed]
- Myers DD, Hawley AE, Farris DM, et al. P-selectin and leukocyte microparticles are associated with venous thrombogenesis. J Vasc Surg 2003;38:1075-89. [Crossref] [PubMed]
- Closse C, Seigneur M, Renard M, et al. Influence of hypoxia and hypoxia-reoxygenation on endothelial P-selectin expression. Thromb Res 1997;85:159-64. [Crossref] [PubMed]
- Esmon CT. Basic mechanisms and pathogenesis of venous thrombosis. Blood Rev 2009;23:225-9. [Crossref] [PubMed]
- Oklu R, Albadawi H, Watkins MT, et al. Detection of extracellular genomic DNA scaffold in human thrombus: implications for the use of deoxyribonuclease enzymes in thrombolysis. J Vasc Interv Radiol 2012;23:712-8. [Crossref] [PubMed]
- Busch C, Cancilla PA, DeBault LE, et al. Use of endothelium cultured on microcarriers as a model for the microcirculation. Lab Invest 1982;47:498-504. [PubMed]
- Bertina RM. Elevated clotting factor levels and venous thrombosis. Pathophysiol Haemost Thromb 2003;33:395-400. [Crossref] [PubMed]
- Rosendaal FR. Venous thrombosis: a multicausal disease. Lancet 1999;353:1167-73. [Crossref] [PubMed]
- Rao LV. Tissue factor as a tumor procoagulant. Cancer Metastasis Rev 1992;11:249-66. [Crossref] [PubMed]
- Abdollahi M, Cushman M, Rosendaal FR. Obesity: risk of venous thrombosis and the interaction with coagulation factor levels and oral contraceptive use. Thromb Haemost 2003;89:493-8. [PubMed]
- Wells PS, Anderson DR, Bormanis J, et al. Value of assessment of pretest probability of deep-vein thrombosis in clinical management. Lancet 1997;350:1795-8. [Crossref] [PubMed]
- Wells PS, Owen C, Doucette S, et al. Does this patient have deep vein thrombosis? JAMA 2006;295:199-207. [Crossref] [PubMed]
- Wells PS, Anderson DR, Rodger M, et al. Evaluation of D-dimer in the diagnosis of suspected deep-vein thrombosis. N Engl J Med 2003;349:1227-35. [Crossref] [PubMed]
- Langford Nj, Stansby G, Avital L. The management of venous thromboembolic diseases and the role of thrombophilia testing: summary of NICE Guideline CG144. Acute Med 2012;11:138-42. [PubMed]
- Kearon C, Julian JA, Newman TE, et al. Noninvasive diagnosis of deep venous thrombosis. McMaster Diagnostic Imaging Practice Guidelines Initiative. Ann Intern Med 1998;128:663-77. [Crossref] [PubMed]
- Ho VB, van Geertruyden PH, Yucel EK, et al. ACR Appropriateness Criteria(®) on suspected lower extremity deep vein thrombosis. J Am Coll Radiol 2011;8:383-7. [Crossref] [PubMed]
- Min SK, Kim YH, Joh JH, et al. Diagnosis and Treatment of Lower Extremity Deep Vein Thrombosis: Korean Practice Guidelines. Vasc Specialist Int 2016;32:77-104. [Crossref] [PubMed]
- Goodacre S, Sampson F, Thomas S, et al. Systematic review and meta-analysis of the diagnostic accuracy of ultrasonography for deep vein thrombosis. BMC Med Imaging 2005;5:6. [Crossref] [PubMed]
- Guyatt GH, Norris SL, Schulman S, et al. Methodology for the development of antithrombotic therapy and prevention of thrombosis guidelines: Antithrombotic Therapy and Prevention of Thrombosis, 9th ed: American College of Chest Physicians Evidence-Based Clinical Practice Guidelines. Chest 2012;141:53S-70S.
- Rabinov K, Paulin S. Roentgen diagnosis of venous thrombosis in the leg. Arch Surg 1972;104:134-44. [Crossref] [PubMed]
- Thomas SM, Goodacre SW, Sampson FC, et al. Diagnostic value of CT for deep vein thrombosis: results of a systematic review and meta-analysis. Clin Radiol 2008;63:299-304. [Crossref] [PubMed]
- Sampson FC, Goodacre SW, Thomas SM, et al. The accuracy of MRI in diagnosis of suspected deep vein thrombosis: systematic review and meta-analysis. Eur Radiol 2007;17:175-81. [Crossref] [PubMed]
- Oklu R, Wicky S. Catheter-directed thrombolysis of deep venous thrombosis. Semin Thromb Hemost 2013;39:446-51. [Crossref] [PubMed]
- Ganguli S, Kalva S, Oklu R, et al. Efficacy of lower-extremity venous thrombolysis in the setting of congenital absence or atresia of the inferior vena cava. Cardiovasc Intervent Radiol 2012;35:1053-8. [Crossref] [PubMed]
- Oklu R, Albadawi H, Jones JE, et al. Reduced hind limb ischemia-reperfusion injury in Toll-like receptor-4 mutant mice is associated with decreased neutrophil extracellular traps. J Vasc Surg 2013;58:1627-36. [Crossref] [PubMed]
- Wicky S, Pinto EG, Oklu R. Catheter-directed thrombolysis of arterial thrombosis. Semin Thromb Hemost 2013;39:441-5. [Crossref] [PubMed]
- Enden T, Haig Y, Kløw NE, et al. Long-term outcome after additional catheter-directed thrombolysis versus standard treatment for acute iliofemoral deep vein thrombosis (the CaVenT study): a randomised controlled trial. Lancet 2012;379:31-8. [Crossref] [PubMed]
- Watson LI, Armon MP. Thrombolysis for acute deep vein thrombosis. Cochrane Database Syst Rev 2004.CD002783. [PubMed]
- Bashir R, Zack CJ, Zhao H, et al. Comparative outcomes of catheter-directed thrombolysis plus anticoagulation vs anticoagulation alone to treat lower-extremity proximal deep vein thrombosis. JAMA Intern Med 2014;174:1494-501. [Crossref] [PubMed]
- Behravesh S, Hoang P, Nanda A, et al. Pathogenesis of Thromboembolism and Endovascular Management. Thrombosis 2017;2017:3039713. [PubMed]
- Streiff MB, Agnelli G, Connors JM, et al. Guidance for the treatment of deep vein thrombosis and pulmonary embolism. J Thromb Thrombolysis 2016;41:32-67. [Crossref] [PubMed]
- Hylek EM, Regan S, Henault LE, et al. Challenges to the effective use of unfractionated heparin in the hospitalized management of acute thrombosis. Arch Intern Med 2003;163:621-7. [Crossref] [PubMed]
- Linkins LA, Dans AL, Moores LK, et al. Treatment and prevention of heparin-induced thrombocytopenia: Antithrombotic Therapy and Prevention of Thrombosis, 9th ed: American College of Chest Physicians Evidence-Based Clinical Practice Guidelines. Chest 2012;141:e495S-530S.
- Garcia DA, Baglin TP, Weitz JI, et al. Parenteral anticoagulants: Antithrombotic Therapy and Prevention of Thrombosis, 9th ed: American College of Chest Physicians Evidence-Based Clinical Practice Guidelines. Chest 2012;141:e24S-43S.
- Holbrook A, Schulman S, Witt DM, et al. Evidence-based management of anticoagulant therapy: Antithrombotic Therapy and Prevention of Thrombosis, 9th ed: American College of Chest Physicians Evidence-Based Clinical Practice Guidelines. Chest 2012;141:e152S-84S.
- Segal JB, Bolger DT, Jenckes MW, et al. Outpatient therapy with low molecular weight heparin for the treatment of venous thromboembolism: a review of efficacy, safety, and costs. Am J Med 2003;115:298-308. [Crossref] [PubMed]
- Konstantinides S, Torbicki A. Management of venous thrombo-embolism: an update. Eur Heart J 2014;35:2855-63. [Crossref] [PubMed]
- Schulman S, Kearon C, Kakkar AK, et al. Dabigatran versus warfarin in the treatment of acute venous thromboembolism. N Engl J Med 2009;361:2342-52. [Crossref] [PubMed]
- Schulman S, Kakkar AK, Goldhaber SZ, et al. Treatment of acute venous thromboembolism with dabigatran or warfarin and pooled analysis. Circulation 2014;129:764-72. [Crossref] [PubMed]
- Connolly SJ, Ezekowitz MD, Yusuf S, et al. Dabigatran versus warfarin in patients with atrial fibrillation. N Engl J Med 2009;361:1139-51. [Crossref] [PubMed]
- Uchino K, Hernandez AV. Dabigatran association with higher risk of acute coronary events: meta-analysis of noninferiority randomized controlled trials. Arch Intern Med 2012;172:397-402. [Crossref] [PubMed]
- EINSTEIN Investigators, Bauersachs R, Berkowitz SD, et al. Oral rivaroxaban for symptomatic venous thromboembolism. N Engl J Med 2010;363:2499-510. [Crossref] [PubMed]
- EINSTEIN–PE Investigators, Büller HR, Prins MH, et al. Oral rivaroxaban for the treatment of symptomatic pulmonary embolism. N Engl J Med 2012;366:1287-97. [Crossref] [PubMed]
- Chai-Adisaksopha C, Crowther M, Isayama T, et al. The impact of bleeding complications in patients receiving target-specific oral anticoagulants: a systematic review and meta-analysis. Blood 2014;124:2450-8. [Crossref] [PubMed]
- Abraham NS, Singh S, Alexander GC, et al. Comparative risk of gastrointestinal bleeding with dabigatran, rivaroxaban, and warfarin: population based cohort study. BMJ 2015;350:h1857. [Crossref] [PubMed]
- Agnelli G, Buller HR, Cohen A, et al. Oral apixaban for the treatment of acute venous thromboembolism. N Engl J Med 2013;369:799-808. [Crossref] [PubMed]
- Loke YK, Pradhan S, Yeong JK, et al. Comparative coronary risks of apixaban, rivaroxaban and dabigatran: a meta-analysis and adjusted indirect comparison. Br J Clin Pharmacol 2014;78:707-17. [Crossref] [PubMed]
- Hokusai-VTE Investigators, Büller HR, Décousus H, et al. Edoxaban versus warfarin for the treatment of symptomatic venous thromboembolism. N Engl J Med 2013;369:1406-15. [Crossref] [PubMed]