Neutrophil extracellular traps are increased in cancer patients but does not associate with venous thrombosis
Introduction
The strong association between malignancy and venous thromboembolic disease has been known for decades (1-3). While several mechanisms have been described, including increased levels of blood clotting factors, increased expression of tissue factor, exposure of phosphatidylserine and release of circulating procoagulant microparticles (4,5), the pathophysiology of this phenomenon still remains largely unknown and may vary between cancer types and stage of disease.
Recently, it was shown that neutrophils, in response to activation, release their genomic DNA into the extracellular space, referred to as neutrophil extracellular traps (NETs). NETs, which are comprised of extracellular chromatin studded with cytoplasmic and nuclear proteins, are released through a process termed NETosis—a form of cell death distinct from apoptosis and necrosis (6). NETs are thought to be an important part of the innate immune response and function as mesh-like networks to trap pathogens in a highly concentrated antimicrobial microenvironment (6). NETs have also been shown to have pro-thrombotic properties, through the activation of both the intrinsic and the extrinsic clotting pathways (7,8). NETs can bind to red blood cells (RBCs) and platelets, thus acting as a scaffold for thrombosis (9,10). NETs have also been observed in human deep venous thrombosis (DVT) samples as a component of the thrombus matrix, supporting their role in thrombus formation (11,12).
Despite preclinical data associating NETs with malignancy and with thrombosis, the role of NETs in cancer-associated thrombosis in humans remains unexplored. In this study, we show that intravascular NETs in cancer patients are elevated due to decreased degradation by plasma nucleases. However, increased levels of NETs and cfDNA in plasma and in venous thrombus of cancer patients did not correlate with higher levels of thrombin-antithrombin (TAT) complex activation or prevalence of DVT. Thrombosis is a complex process; the exact mechanism of NETs as a procoagulant in humans remains to be elucidated.
Methods
Study design
Institutional review board (IRB) approval was obtained for this study. Plasma samples were collected from sequential patients who presented to the radiology department for a computed tomography (CT) imaging study. Following sample acquisition, demographic and clinical data were retrospectively analyzed. A total of 65 plasma samples were collected, and of these, 27 patients had a cancer diagnosis (Table 1). The control population comprised of non-cancer patients that presented for a CT scan for the evaluation of vascular disease (n=25; aneurysm, dissection, peripheral vascular disease), chest pain (n=4) and abdominal pain (n=9).
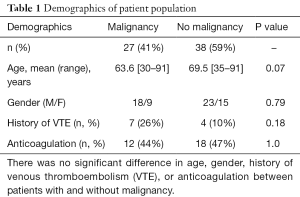
Full table
Circulating nucleosome levels in plasma
The plasma levels of circulating mono- and oligo-nucleosomes were measured using Cell Death Detection ELISA kit (Roche Diagnostics Corporation, Indianapolis, IN, USA), according to manufacturer instructions.
Circulating free DNA (cfDNA) levels in plasma
Levels of plasma cfDNA were quantified using Quant-iT™ PicoGreen® double stranded DNA Assay Kit (Thermo Fisher Scientific Inc., Grand Island, NY, USA), according to manufacturer’s instructions. Each sample was diluted ten-fold in 10 mM Tris-HCl, 1 mM EDTA, pH =7.5 DNase-free buffer, and loaded into a 96 well plate. Fluorescent signal of the plasma and the DNA standard samples were acquired at excitation of 485 nm and emission of 538 nm using fluorescent microplate reader (Molecular Devices, Sunnyvale, CA, USA). The plasma cfDNA concentration was extrapolated from the standard DNA values and expressed in nanogram/mL ± standard error of the mean.
DNase-1 and endonuclease-G protein levels in plasma
To test for differences in DNA degradation between cancer and non-cancer patients, DNase-1 and endonuclease-G protein levels were quantitated using Western blotting. Human plasma was mixed with four volumes of RIPA protein lysis buffer containing 10 µL/mL protease inhibitor cocktail (Sigma-Aldrich, St. Louis, MO, USA). The samples were mixed with Laemmli sample buffer and boiled at 95 °C for 5 min. Soluble plasma protein was loaded into 4–15% SDS-PAGE gradient gel (Bio-Rad, Hercules, CA, USA) and transferred to a nitrocellulose membrane (Bio-Rad). The membranes were treated with blocking solution (Sigma-Aldrich) for 1 h at room temperature. Membranes were incubated over night with polyclonal rabbit anti-DNase-1 (SC-30058) or anti-Endonuclease G (SC-32935, Santa Cruz Biotech, Santa Cruz, CA, USA) IgG at 1:500 dilution followed by 1:4000 diluted horse-radish-peroxidase-conjugated goat anti-rabbit IgG for 1 h at room temperature. The membranes were reacted using chemiluminescence system (ECL Advance Western Blotting Detection Kit, Amersham Bioscience, GE Healthcare, Pittsburgh, PA, USA) and the specific chemiluminescence signal was visualized using FluorChem HD2 imaging system (ProteinSimple, Santa Clara, CA, USA). The membranes were stained with Ponceau S to confirm equal sample loading and the band density of 60 kDa protein was obtained. The specific band densities of the DNase-1 and Endonuclease-G were calculated using the imager software and normalized to the band densities of the Ponceau S staining. Data was expressed as arbitrary units (AU).
Plasmid incision assay
In addition to testing plasma levels of endonucleases, we tested nuclease activity using a plasmid incision assay in the following manner. An aliquot of each plasma sample was diluted 20-fold in a buffer containing 50 mM Tris-HCl, 0.5% Triton X-100, 0.25 M sucrose and 10 µL of protease inhibitor cocktail (Sigma-Aldrich). Equal aliquots from each sample were added to a reaction buffer containing 1 µg pBR322 plasmid DNA (New England Biolabs, Beverly, MA, USA), 2 mM CaCl2, 5 mM MgCl2, 10 mM Tris-HCl (pH 7.4) and 0.5 mM dithiothreitol and incubated at 37 °C for 30 min. The reaction was stopped with a buffer containing 0.05% w/v Bromophenol blue, 40% sucrose, 0.1 M ethylenediaminetetraacetic acid (EDTA) pH 8.0 and 1% sodium dodecyl sulfate (SDS). Samples were loaded into 1% agarose gel containing 1 µg/mL ethidium bromide and run at 7 V/cm for 2 h. A digital image of the gel was acquired using FluorChem HD2 imaging system (ProteinSimple) and UV light source. The relative amount of nuclease activity was calculated based on the density of the three bands for plasmid DNA present in covalently closed circular DNA (Type I), open circular DNA (Type II), or linear DNA (Type III), as previously described (13,14).
TAT complex in plasma
Quantitative assessment of TAT complex concentration in the plasma was measured using a human ELISA kit (ab108907, Abcam, Cambridge, MA, USA). The absorbance was read on a microplate reader at a wavelength of 450 nm with wavelength correction at 570 nm. The concentration in the plasma was determined by extrapolating from a regression analysis of the log-log curve-fit of the standard values. Results were expressed as nanograms per milliliter ± standard error of the mean.
Analysis of NETs in human thrombus
The presence of NETs in fresh human venous thromboemboli was assessed in the following manner. Aspirated thrombus specimens were acquired from patients with malignancy (n=5) who presented to interventional radiology for management of acute ileocaval thrombosis and who underwent mechanical thrombectomy. Samples were acquired through 9–12 French sheaths. All patients carried a diagnosis of malignancy: lung cancer (n=3), pancreatic adenocarcinoma (n=1), and bladder cancer (n=1). Thrombus samples were fixed in formalin immediately after acquisition. Immunohistochemistry (IHC) for NETs was then performed in the following manner. Sections were incubated with mouse monoclonal anti-histone H2A/H2B/DNA complex antibody at 1 µg/mL dilution (gift of Dr. Marc Monestier, Temple University, PA, USA). Slides were incubated with biotin conjugated anti-mouse IgG (Vector Labs, Burlingame, CA, USA) followed by HRP-conjugated streptavidin. Specific signal was developed using cyanine-3 tyramide signal amplification kit (Perkin Elmer, Boston, MA, USA) according to the manufacturer’s instructions. An adjacent section was treated with human recombinant DNase-1 prior to immunostaining to demonstrate antibody specificity and illustrate the potential therapeutic implications. Digital images were acquired using a digital slide scanner (NanoZoomer2.0-RS, Hamamatsu Corporation, Middlesex, NJ, USA) and quantified using ImageJ software.
Immunohistochemical analysis of NETs from normal and malignant tissue
The presence of NETs in normal and malignant tissue was assessed in the following manner. Paraffin embedded tissue sections of both normal organs and malignant tissue were purchased from a commercial tissue bank (IMH-326/327/343, Imgenex, San Diego, CA, USA). Detailed information about each slide is available online. Briefly, the slides contain both normal tissues (skin, breast, spleen, muscle, lung, liver, stomach, colon, kidney, prostate, placenta, and brain) as well as malignant tissues from a range of organs (breast, lung, liver, esophagus, stomach, small bowel, colon, kidney, bladder, ovary, and skin). Slide processing and IHC staining was performed according to the manufacturer’s instructions. Paraffin embedded tissue sections were deparaffinized and rehydrated then incubated with mouse monoclonal anti-histone H2A/H2B/DNA complex antibody as above. The slides were covered with mounting medium (Vectashield, Vector Labs) containing 4',6-diamidino-2-phenylindole (DAPI). For negative control, the NETs antibody was omitted and substituted with 1µg/mL dilution of non-specific anti-mouse IgG.
Statistical analysis
Statistical analysis was performed using Prism-5 program (Graph pad, La Jolla, CA, USA) using parametric and non-parametric t-tests. The results are expressed as the mean ± standard error. Demographic and clinical characteristics were compared using Chi-square for parametric continuous variables and Kruskal-Wallis test for non-parametric data.
Results
Patient population
There was no significant difference in mean age (63.6 vs. 69.5 years, P>0.05) or gender (67% vs. 64% male, P>0.05) for the non-malignancy and malignancy groups (Table 1). Similarly, laboratory values between the two groups, including white blood cell count, neutrophil count, sedimentation rate, C-reactive protein, prothrombin time, international normalized ratio, activated partial thromboplastin time, hematocrit, and platelet levels, were not significantly different (P>0.05) (Table 2). There was no significant difference in the clinical history of previous venous thromboembolic events (Chi square =1.59, P>0.1), thromboembolic risk factors (smoking, immobilization, oral contraceptive use; Chi square =0.97, P>0.1), or anticoagulation use (heparin, warfarin, aspirin, dipyridamole and clopidogrel; Chi square =1.07, P>0.1) between the malignancy and non-malignancy groups. However, there was a significantly greater number of patients with thromboembolic risk factors in the malignancy group (Chi square =7.78, P<0.01).
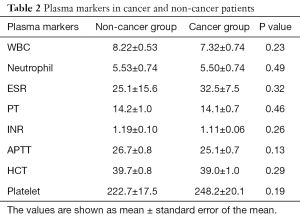
Full table
Plasma NETs analysis
Circulating nucleosome levels, indicating NETs in plasma, were markedly higher in the malignancy group compared to the non-malignancy group (0.188±0.046 vs. 0.062±0.004 AU, P=0.0009) (Figure 1). Similarly, cfDNA levels were higher in the malignancy group (950.34±88.40 vs. 625.02±56.87 ng/mL, P=0.0008) (Figure 1). Consistent with these results, plasma levels of DNase-1 were found to be lower in the malignancy group (5.2±0.36 vs. 5.9±0.19 AU, P=0.016) (Figure 2). Endonuclease-G plasma levels, however, were not found to be significantly different between the two groups (7.4±1.1 vs. 6.4±0.4 AU, P=0.95) (Figure 2). These data imply that diminished degradation of nucleic acids by plasma nucleases such as DNAse-1 could result in higher levels of NETs in cancer patients.
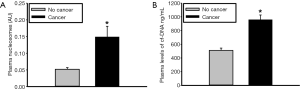
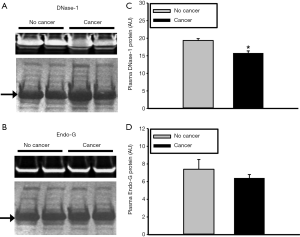
Since there are many nucleases present in plasma, overall nuclease activity was evaluated using a semi-quantitative plasmid incision assay. Plasma nuclease activity was found to be significantly lower in cancer patients compared to non-cancer patients (349±14 vs. 406±20 AU, P=0.029) (Figure 3). These data further substantiate the hypothesis that increased plasma levels of NETs and cfDNA in cancer patients are due, in part, to reduced nuclease levels and/or activity.
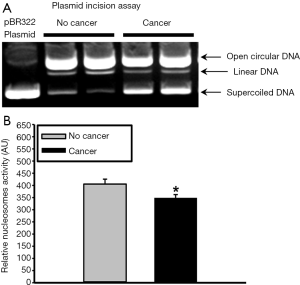
To assess for intravascular coagulation activity, TAT complex levels was quantified (15). TAT complex plasma concentration was slightly elevated in cancer patients (34.19±17.62 ng/mL), in comparison to non-cancer group (31.26±15.05 ng/mL) but this increase was not statistically significant (P=0.29) (Figure 4).
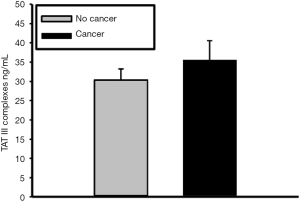
Analysis of NETs in human thrombus
Next, we immunostained aspirated thrombus tissues from five cancer patients that presented with acute venous thrombosis; a corresponding plasma analysis for NETs in this subset was not performed. IHC was notable for extensive extracellular staining, consistent with NETs, throughout the thrombus (Figure 5). Moreover, consecutive sections pretreated with DNase prior to immunostaining showed marked reduction in the level of NETs detected in the extracellular matrix demonstrating the specificity of the NETs antibody used in these experiments.
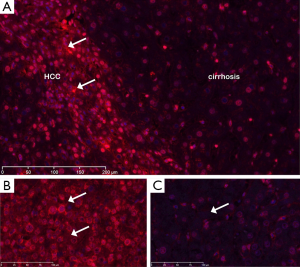
IHC analysis of NETs from normal and malignant tissues
The presence of NETs in an array of both normal and malignant tissue was evaluated by IHC. Examples are provided in Figures 6 and 7. Cirrhotic tissue had minimal NETs signal; however, hepatocellular carcinoma (HCC) tissue demonstrated higher cellularity and higher relative levels of NETs signal in the extracellular space (Figure 6). In lung cancer, scattered foci within the tumor demonstrated intense NETs signal in the extracellular matrix (Figure 7). In other tissue specimens included in the array, NETs signal was predominantly nuclear.
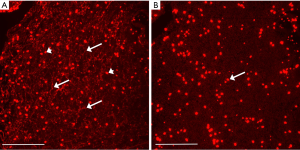
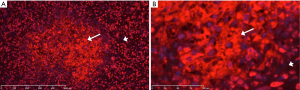
Discussion
NETs are complex extracellular networks of nucleic acids studded with histones and anti-microbial proteins that may promote thrombosis. Increased levels of intravascular NETs have been proposed as a mechanism for the high rate of venous thromboembolic disease in cancer patients (12,16-19). In this study, we tested the hypothesis that intravascular NETs are higher in cancer patients and associate with higher prevalence of venous thrombosis when compared to non-cancer patients. Blood sampled from cancer patients was found to have greater concentrations of NETs and cfDNA, as well as lower concentrations and activity of nucleases, compared to a demographically similar group of non-cancer patients. Extracellular DNA was shown to be a diffuse component of human thrombus samples from cancer patients, and treatment with DNase substantially degraded this scaffolding network to indicate the specificity of the antibody. Finally, NETs were shown to be present extensively within the extracellular matrix of certain malignant tissues.
NETs and cfDNA represent a conserved, common denominator among cancer, thrombosis, autoimmune diseases and infection, and their role in the pathogenesis of these diseases continues to expand. Patients with sepsis have higher plasma levels of nucleosomes compared to healthy controls (20), and elevated level of cfDNA has been suggested as a prognostic indicator of mortality in intensive care unit patients with severe sepsis. Decreased plasma nucleosome levels have been suggested as predictors for response to cancer chemotherapy and as a prognostic biomarker in various malignancies, including pancreatic, colorectal, lung, breast and liver cancer (21-26); following therapy, patients with progressive disease have been shown to have higher levels of circulating nucleosomes compared to patients with partial or complete response (22,27-29). NETs may also play a role in ischemia-reperfusion injury (7,30), capillary injury (31), and atherosclerosis (32).
Nucleosomes are a cell death by-product, and thus it follows logically that patients with aggressive tumors with high proliferative index would lead to greater nucleosome release especially by circulating tumor cells. Additionally, NETs and cfDNA can also accumulate in plasma due to reduced degradation process by nucleases. Blood nuclease activity is responsible for the degradation of circulating nucleosomes. Its plasma level is known to be decreased in systematic lupus erythematosus patients, causing longer persistence of nucleosomes and sustained autoimmune response stimulation (33). Although reduced in vitro activity of endonuclease (DNase) in various tumor cells has been known for more than 50 years, their plasma levels have not been studied in cancer patients until recently (34).
Beyond elucidating a fundamental mechanism for cancer-associated thromboembolic disease, NETs may represent a new therapeutic target for venous thromboembolic disease (12). NETs are a component of thrombus matrix and a scaffold for thrombus formation (11,12). Moreover, in this study DNase was shown to be an effective method of dismantling the NETs scaffold. Thus, NETs may be a potential drug target for cancer-induced thrombosis (35,36). A previous human plasma study suggested that DNase treatment results in increased thrombin generation, possibly due to breakdown of cfDNA scaffold, thus exposing histone protein molecules which possess potent pro-inflammatory and platelet activation properties (20). On the contrary, another study showed that DNase treatment protects mice from flow restriction-induced DVT (9,37). Therefore, any therapeutic potential of recombinant DNase for thrombosis remains to be further investigated.
Lung cancer and HCC tissues demonstrated intense IHC signal for NETs in the extracellular matrix of the tumor tissue. Although the source for these NETs is not known, it is either from cancer cell turn-over or from immune cells such as neutrophils. The significance of these NETs in tumor tissue is also not known; however, high levels of DNA in the extracellular matrix will likely impede chemotherapy distribution due to steric hindrance from the negatively charged DNA and reduce drug efficacy (38).
There are several limitations to this study. While no significant difference in prior venous thromboembolic event was found between the malignancy and non-malignancy cohorts in the plasma NETs experiment, these patients were not followed prospectively to identify subsequent thrombosis events. Additionally, we identified an extensive NETs infrastructure within thrombus aspirated from cancer patients, but we did not compare these results with thrombus obtained from non-cancer patients to assess relative levels of NETs. Furthermore, plasma from these patients with active venous thrombosis was not analyzed because the thrombectomy procedure often leads to cellular injury and this may potentially cause a rise in plasma NETs levels. The source of the NETs detected in the thrombus tissue and in cancer tissue was not investigated; immune cells and/or cancer cells maybe the source.
Conclusions
NETs are elevated in patients with cancer and comprise an extensive scaffolding network within thrombus and certain tumor tissue. These high levels of NETS and cfDNA in cancer patients are associated with reduced DNAse-1 levels and nuclease activity but did not associate with higher prevalence of venous thrombosis. While NETs may potentially become an important biomarker of malignancy, further studies are warranted to determine their role as a procoagulant in human thrombosis.
Acknowledgments
Funding: R Oklu gratefully acknowledges funding from the National Institutes of Health (EB021148, CA172738, EB024403, HL137193) and the Mayo Clinic.
Footnote
Conflicts of Interest: The authors have no conflicts of interest to declare.
Ethical statement: The study was approved by the Institutional review board (No. 2010P002234). Written consent form showed that all data could be used for publication.
References
- Zwicker JI, Furie BC, Furie B. Cancer-associated thrombosis. Crit Rev Oncol Hematol 2007;62:126-36. [Crossref] [PubMed]
- Langer F, Bokemeyer C. Crosstalk between cancer and haemostasis. Implications for cancer biology and cancer-associated thrombosis with focus on tissue factor. Hamostaseologie 2012;32:95-104. [Crossref] [PubMed]
- Connolly GC, Khorana AA. Emerging risk stratification approaches to cancer-associated thrombosis: risk factors, biomarkers and a risk score. Thromb Res 2010;125 Suppl 2:S1-7. [Crossref] [PubMed]
- Thaler J, Ay C, Pabinger I. Clinical significance of circulating microparticles for venous thromboembolism in cancer patients. Hamostaseologie 2012;32:127-31. [Crossref] [PubMed]
- Zwicker JI. Predictive value of tissue factor bearing microparticles in cancer associated thrombosis. Thromb Res 2010;125 Suppl 2:S89-91. [Crossref] [PubMed]
- Lu T, Kobayashi SD, Quinn MT, et al. A NET Outcome. Front Immunol 2012;3:365. [Crossref] [PubMed]
- Oklu R, Albadawi H, Jones JE, et al. Reduced hind limb ischemia-reperfusion injury in Toll-like receptor-4 mutant mice is associated with decreased neutrophil extracellular traps. J Vasc Surg 2013;58:1627-36. [Crossref] [PubMed]
- Massberg S, Grahl L. Reciprocal coupling of coagulation and innate immunity via neutrophil serine proteases. Nat Med 2010;16:887-96. [Crossref] [PubMed]
- Brill A, Fuchs TA, Savchenko AS, et al. Neutrophil extracellular traps promote deep vein thrombosis in mice. J Thromb Haemost 2012;10:136-44. [Crossref] [PubMed]
- Clark SR, Ma AC, Tavener SA, et al. Platelet TLR4 activates neutrophil extracellular traps to ensnare bacteria in septic blood. Nat Med 2007;13:463-9. [Crossref] [PubMed]
- Savchenko AS, Martinod K, Seidman MA, et al. Neutrophil extracellular traps form predominantly during the organizing stage of human venous thromboembolism development. J Thromb Haemost 2014;12:860-70. [Crossref] [PubMed]
- Oklu R, Albadawi H, Watkins MT, et al. Detection of extracellular genomic DNA scaffold in human thrombus: implications for the use of deoxyribonuclease enzymes in thrombolysis. J Vasc Interv Radiol 2012;23:712-8. [Crossref] [PubMed]
- Basnakian AG, Ueda N, Kaushal GP, et al. DNase I-like endonuclease in rat kidney cortex that is activated during ischemia/reperfusion injury. J Am Soc Nephrol 2002;13:1000-7. [PubMed]
- Buzder T, Yin X, Wang X, et al. Uptake of foreign nucleic acids in kidney tubular epithelial cells deficient in proapoptotic endonucleases. DNA Cell Biol 2009;28:435-42. [Crossref] [PubMed]
- Lippi G, Cervellin G, Franchini M, et al. Biochemical markers for the diagnosis of venous thromboembolism: the past, present and future. J Thromb Thrombolysis 2010;30:459-71. [Crossref] [PubMed]
- Demers M, Krause DS, Schatzberg D, et al. Cancers predispose neutrophils to release extracellular DNA traps that contribute to cancer-associated thrombosis. Proc Natl Acad Sci USA 2012;109:13076-81. [Crossref] [PubMed]
- Hawes MC, Wen F, Elquza E. Extracellular DNA: A Bridge to Cancer. Cancer Res 2015;75:4260-4. [Crossref] [PubMed]
- Demers M, Wagner DD. NETosis: a new factor in tumor progression and cancer-associated thrombosis. Semin Thromb Hemost 2014;40:277-83. [Crossref] [PubMed]
- Demers M, Wagner DD. Neutrophil extracellular traps: A new link to cancer-associated thrombosis and potential implications for tumor progression. Oncoimmunology 2013;2:e22946. [Crossref] [PubMed]
- Gould TJ, Vu TT, Swystun LL, et al. Neutrophil extracellular traps promote thrombin generation through platelet-dependent and platelet-independent mechanisms. Arterioscler Thromb Vasc Biol 2014;34:1977-84. [Crossref] [PubMed]
- Kohles N, Nagel D, Jüngst D, et al. Relevance of circulating nucleosomes and oncological biomarkers for predicting response to transarterial chemoembolization therapy in liver cancer patients. BMC Cancer 2011;11:202. [Crossref] [PubMed]
- Holdenrieder S, Nagel D, Schalhorn A, et al. Clinical relevance of circulating nucleosomes in cancer. Ann N Y Acad Sci 2008;1137:180-9. [Crossref] [PubMed]
- Wittwer C, Boeck S, Heinemann V, et al. Circulating nucleosomes and immunogenic cell death markers HMGB1, sRAGE and DNAse in patients with advanced pancreatic cancer undergoing chemotherapy. Int J Cancer 2013;133:2619-30. [PubMed]
- Kumar S, Guleria R, Singh V, et al. Plasma nucleosome levels might predict response to therapy in patients with advanced non-small-cell lung cancer. Clin Lung Cancer 2010;11:36-44. [Crossref] [PubMed]
- Kumar S, Guleria R, Singh V, et al. Efficacy of circulating plasma DNA as a diagnostic tool for advanced non-small cell lung cancer and its predictive utility for survival and response to chemotherapy. Lung Cancer 2010;70:211-7. [Crossref] [PubMed]
- Fahmueller YN, Nagel D, Hoffmann RT, et al. Predictive and prognostic value of circulating nucleosomes and serum biomarkers in patients with metastasized colorectal cancer undergoing Selective Internal Radiation Therapy. BMC Cancer 2012;12:5. [Crossref] [PubMed]
- Stoetzer OJ, Wittwer C, Lehner J, et al. Circulating nucleosomes and biomarkers of immunogenic cell death as predictive and prognostic markers in cancer patients undergoing cytotoxic therapy. Expert Opin Biol Ther 2012;12 Suppl 1:S217-24. [Crossref] [PubMed]
- Holdenrieder S, Stieber P. Clinical use of circulating nucleosomes. Crit Rev Clin Lab Sci 2009;46:1-24. [Crossref] [PubMed]
- Mueller S, Holdenrieder S, Stieber P, et al. Early prediction of therapy response in patients with acute myeloid leukemia by nucleosomal DNA fragments. BMC Cancer 2006;6:143. [Crossref] [PubMed]
- Albadawi H, Oklu R, Raacke Malley RE, et al. Effect of DNase I treatment and neutrophil depletion on acute limb ischemia-reperfusion injury in mice. J Vasc Surg 2016;64:484-93. [Crossref] [PubMed]
- Boneschansker L, Inoue Y, Oklu R, et al. Capillary plexuses are vulnerable to neutrophil extracellular traps. Integr Biol (Camb) 2016;8:149-55. [Crossref] [PubMed]
- Oklu R, Stone JR, Albadawi H, et al. Extracellular traps in lipid-rich lesions of carotid atherosclerotic plaques: implications for lipoprotein retention and lesion progression. J Vasc Interv Radiol 2014;25:631-4. [Crossref] [PubMed]
- Napirei M, Gültekin A, Kloeckl T, et al. Systemic lupus-erythematosus: deoxyribonuclease 1 in necrotic chromatin disposal. Int J Biochem Cell Biol 2006;38:297-306. [Crossref] [PubMed]
- Taper HS. Altered deoxyribonuclease activity in cancer cells and its role in non toxic adjuvant cancer therapy with mixed vitamins C and K3. Anticancer Res 2008;28:2727-32. [PubMed]
- Wicky S, Pinto EG, Oklu R. Catheter-directed thrombolysis of arterial thrombosis. Semin Thromb Hemost 2013;39:441-5. [Crossref] [PubMed]
- Oklu R, Wicky S. Catheter-directed thrombolysis of deep venous thrombosis. Semin Thromb Hemost 2013;39:446-51. [Crossref] [PubMed]
- von Brühl ML, Stark K, Steinhart A, et al. Monocytes, neutrophils, and platelets cooperate to initiate and propagate venous thrombosis in mice in vivo. J Exp Med 2012;209:819-35. [Crossref] [PubMed]
- Sheth RA, Hesketh R, Kong DS, et al. Barriers to drug delivery in interventional oncology. J Vasc Interv Radiol 2013;24:1201-7. [Crossref] [PubMed]