Advances in cardiac CT contrast injection and acquisition protocols
Introduction
Technical developments have overcome the limitation of cardiac motion artifacts and made cardiac CT widely available. Coronary CT angiography (CTA) offers the tremendous benefit of obviating an invasive coronary angiography exam, specifically in patients with normal CTA results. With the additional features of functional cardiac and valvular assessment, and myocardial perfusion, CT has also become an alternative and complementary modality for specific questions traditionally reserved for cardiac ultrasound (echocardiography) or magnetic resonance imaging (MRI). However, a key component of cardiac CT imaging is based upon robust CT protocols adapted to available CT technique with reliable enhancement of iodine contrast medium (CM).
Optimal opacification
Contrast enhancement as the result of iodinated CM injection is a complex interaction of numerous factors which can be divided in patient-related, scanner-related and CM-related factors (1) (Figure 1).
Most important patient related factors are body size and cardiac output (1). An adaption of the amount of iodine to the patient’s body habitus, usually to weight or body-surface area, would be ideal (2). However, most cardiac CT studies report a consistent amount of CM. This makes sense as this is much easier to handle in clinical care.
Cardiac output affects contrast timing resulting in slower arrival of the CM bolus and a delayed enhancement when cardiac output is low (1,2). Sub-second acquisitions require precise scan timing for optimal opacification, at least of the left cardiac chambers, ascending aorta, and coronary arteries. Thus, knowledge of scan delay is crucial and needs to be determined individually. Optimal intravascular enhancement in coronary CTA is customarily considered between 250 and 300 Hounsfield Units (HU) to allow optimal differentiation of low-density coronary artery atherosclerotic lesions (approximately 40 HU), intermediate fibrous plaques (approximately 90 HU) and calcified plaques with greater than 130 HU (3-6).
Basics for CM injection
CM-related factors include injection duration, rate and bolus shaping, CM concentration and physicochemistry, and use of a saline chaser (1).
An 18-gauge IV catheter placed in the right antecubital vein is preferable, since the left arm might result in high-attenuation “streak” artifacts in the left subclavian vein and internal mammary artery. Hand veins should be avoided. In smaller patients, a 20-gauge catheter is also feasible. Central line can be used, when catheters are rated for IV injection.
Typically, injection protocols specify injection rate and duration (7). A high injection rate combined with a high iodine concentration CM is typically used in coronary CTA. An injection rate of at least 5 mL/s is preferable, with increased rates at higher cardiac outputs (8). An increase in injection rate results in an earlier and higher arterial enhancement (1). The injection duration should be at least as long as the estimated scan duration (8). With the availability of very short scan times, the injection duration is typically held to at least 10–12 seconds (acknowledging the minimum bolus needed to opacify the coronary circulation).
Multiphasic injection protocols
Multiphasic injection protocols are common in cardiac CT imaging. Dual-head power injectors progressively replace standard single-head pumps. These new injectors have separate reservoirs for iodine CM and saline and allow injection of CM, saline or a mixture of both. Biphasic protocols usually contain undiluted CM bolus with volumes ranging from 50 to 120 mL providing high contrast in the left cardiac chambers, ascending aorta and coronary arteries followed by a 20–30 mL saline chaser (8). Using modern power injector systems, minimized CM volumes, and sophisticated scan timing techniques, the saline chasing approach has become so effective that the right ventricle (RV) is almost entirely void of CM at the time of scan acquisition. This has the advantage to suppress streak artifacts from the RV. RV enhancement is discussed controversially. However, Kok et al. could not find benefits of RV enhancement during screening coronary CTA as they hardly detected any RV pathology (9). Therefore, it has been suggested that contrast bolus could be exclusively tailored to enhancement of the coronary arteries in at least some targeted scenarios (10).
Triphasic injection protocols contain an undiluted CM bolus, followed by a diluted contrast chaser and, finally, the saline chaser. The dilution of CM can be varied (e.g., 20% iodine with 80% saline) and provides diminished right heart cavity attenuation (Figure 2). That allows accurate and reproducible assessment of the RV volumes, anatomic structures, cardiac disease, and right ventricular function. Thus, appropriately tailored cardiac CT can be considered a reliable alternative for patients who are not suitable for either echocardiography or MRI.
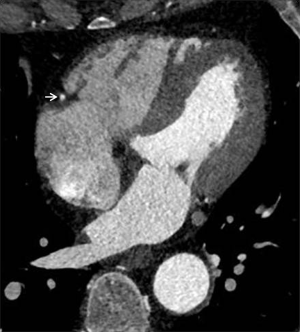
Test bolus and bolus tracking techniques
In times when cardiac CT scans can be performed within a single heartbeat, narrowed time window for optimal contrast enhancement needs exact timing with the opportunity to lower the amount of CM. A fixed delay is not recommended in cardiac CT (11). Time-to-peak enhancement differs for different target vessels (pulmonary artery, coronary artery, aorta, and peripheral arteries) and is dependent on the distance from venous access site, cardiac output, and possible vascular pathologies (1). Test bolus and bolus tracking are both techniques to calculate delay. In bolus tracking, attenuation is measured in a region of interest (ROI, e.g., aorta or pulmonary artery) while CM is injected. When a predefined threshold (typically 100–150 HU) is reached, breathing commands and CT scan is triggered automatically. In comparison, test bolus technique monitors a small amount (10–20 mL) of CM (followed by a saline flush) (Figure 3). In a second step, an ROI is placed by hand and the attenuation curve for this ROI is displayed (Figure 4). Based on this curve, additional time will be added before the actual CT scan starts. The advantage of the test bolus is that it serves as a “trial run” to ensure correct IV catheter function and those patients are getting used to the warmth and unusual taste sensation of CM before the actual CT scan. Drawback of the test bolus technique is the additional, minimal volume of CM. However, a 50% diluted CM bolus with only 5 mL of undiluted iodine is feasible (12). The risk of the bolus-tracking method is to miss optimal enhancement as breathing commands result in additional delay and, therefore, the threshold (usually 100–150 HU) is far below the optimal enhancement (250–300 HU). Further, an abort of the scan due to discomfort requires a repetition of the entire injection when bolus-tracking is used. Finally, in the test bolus technique, the total injection can be tailored more carefully to the planned scan length;
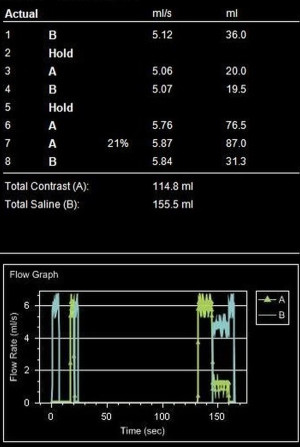
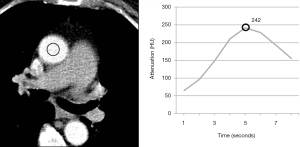
A new dual-ROI tracker technique has the potential to improve consistent opacification. After a first ROI with a threshold of 100 HU initiates breathing commands, a second ROI with a higher threshold closer to peak enhancement (300 HU) triggers the CT scan (13,14). This technique has shown more consistent aortic and coronary enhancement with reduced interpatient variability.
CT scanner technique
New scanner generations offer a combination of high spatial resolution, high-temporal resolution, and wide z-axis coverage with up to 320 detector rows or by using dual-source technique at very high pitch (>3.0) with shorter scan times, as low as a single heartbeat. The risk of slab-to-slab and motion artifacts is reduced. However, precise timing of CM bolus is crucial with the opportunity to tailor the CM bolus. Due to steadily new and improved CT scanners, adaptation of old and evaluation of new acquisition and contrast injection protocols are crucial.
Low tube voltage examinations
Multiple studies demonstrated the feasibility of CTA with reduced tube voltages. As 70- and 80-kVp is closer to the k-edge of iodine (33.2 keV) than conventional 120-kVp, iodine-containing CM attenuation is substantially higher in lower tube voltages (15). Drawback of low voltage scans is increased image noise. To maintain image quality, tube current should be increased, but this is limited to the tube current capacity (15). Late-generation scanners have higher capacities to counteract image noise in low voltage scans. Automated, patient’s habitus based tube voltage selection technique is favored to provide optimal noise levels (16). Despite of an increase in tube current, lower tube voltages result in less radiation exposure (15-17).
Iterative reconstruction (IR)
Each CT vendor provides its own IR algorithms. All of these reconstruction techniques have in common to reduce image noise based on a user-based setting independent of the CT acquisition and, therefore, without additional radiation penalty (18).
Advances in CT technique
The combination of high attenuation in low-kVp scans with low noise levels due to increased tube current output and additional IR result in increased contrast (14,18) (Figure 5). Thus, low tube-voltage scans combined with IR allow reduced iodine CM volumes or concentrations to achieve 120-kVp equal image noise and contrast (19). Reduced iodine concentration CM (270 mgl/mL) in 100-kVp scans result in similar vessel attenuation than standard iodine concentration (370 mgl/mL) in 120-kVp scans (20,21). The combination of 80-kVp, IR, and dual-ROI tracker technique allowed a 50% reduction of CM (140 instead of 280 mgI/kg) still providing homogenous enhancement in coronary CTA (14). However, an increase from low tube voltages to standard 120-kVp due to patient’s habitus with similar low amount of CM volume may result in a poor opacification. Therefore, the combination of automated tube voltage selection and reduced amount of CM might be critical. On the other side, a standard CM concentration and volume in low voltage 70-kVp coronary CTA resulting in very high lumen attenuation might make less apparent the visualization of calcified plaque, which may overlap the density of enhanced lumen (which can happen at any kVp). Further research should focus on the incorporation of individualized patient body habitus based automatic tube voltage adaptation and appropriate, tube-voltage based selection of iodine CM volume and concentration for optimal opacification. We believe that tube voltage based selection of CM volume and concentration has tremendous potential for more individual patient-adapted iodine volumes with beneficial net lower doses of iodine dispensed.
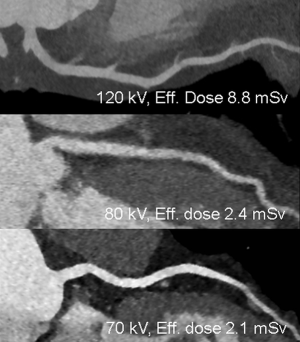
Spectral computed tomography (CT)
Spectral CT allows acquisition of data sets at different energy levels, usually 80- and 140-kVp (22). Acquisition of these data sets is vendor-specific. Independent of the acquisition technique, multiple post-processing techniques are available which focus on image quality improvement and material decomposition (23). Virtual monoenergetic imaging (VMI) allows imaging similar from a monoenergetic beam at a single kiloelectron voltage level with energy levels ranging from 40 to 200 keV (24). VMI at low energy levels (closer to the k-edge of iodine) have higher attenuation values and are used to improve contrast (22). Forty to sixty keV showed best balance between vessel attenuation and image noise in pre-transcatheter aortic valve replacement (TAVR) CTA depending on the used VMI algorithm (25). This can be used to optimize suboptimal opacification or lower the amount of iodine CM, e.g., in patients with impaired renal function (10,26,27) (Figure 6). VMI at high energy levels are useful to reduce artifacts (28). Material decomposition has been shown to have potential benefits for mapping of iodine distribution in the myocardium as a quantitative marker for perfusion and blood volume (29) or reconstruction of virtual unenhanced images (30) (Figure 7), but is still under intense debate and ongoing research.
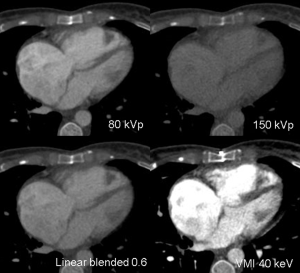
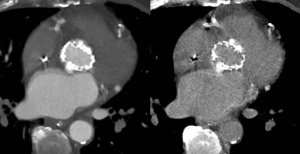
Cardiac function analysis
Regional left ventricular (LV) function assessment in CT is highly concordant with cardiac MRI (31,32) and improves diagnostic accuracy for acute coronary syndrome in patients with acute chest pain (33). Further, it allows imaging of valve replacements with functional assessment. However, radiation exposure increases substantially in cardiac function analysis if coronary-appropriate radiation doses are applied during the entire acquisition. Therefore, a short cardiac cycle phase with high tube current is usually combined with low tube current over the remainder of the cardiac cycle. Triphasic injection protocol is recommended to provide right ventricular enhancement to allow for septal thickness and motion evaluation.
Stress myocardial CTP
Stress myocardial CTP has shown good diagnostic accuracy in comparison to established invasive angiography and non-invasive perfusion imaging (SPECT, MRI) (34-40), in cohorts at higher pre-test risk for obstructive CAD than is routinely appropriate for traditional coronary CTA. CTP is composed of a stress and a rest phase acquisition. When the pharmacological stress agent (e.g., adenosine, regadenoson) reaches maximum effect, 60 to 70 mL of undiluted CM is injected. As beta-blockade influences myocardial perfusion (via blunted peak stress response), rest phase imaging is performed after stress phase, and includes visualization of the coronary arteries. However, some newer CT scanner generations can obviate the need for beta-blocker administration even for coronary assessment (16). Additional CM dye is necessary in the rest phase acquisition resulting in a total volume of approximately 130 to 150 mL CM (41). Potential radiation dose and CM savings might be possible when coronary arteries could be safely evaluated in stress CTP. Similar to SPECT imaging, rest phase acquisition might then be only performed when pathologies are visible in the myocardial stress perfusion images.
CM attenuation is proportional to the concentration of iodine. Thus, hypo-attenuated myocardial areas represent hypoperfusion and/or reduced intravascular blood volume (42). Artifacts due to dense contrast in the cardiac chambers or the aorta could mimic perfusion defects and might limit interpretation (41). Spectral imaging with VMI and iodine mapping might have additional benefit for myocardial perfusion interpretation.
Image acquisition for myocardial CTP can be performed as a standard, static scan starting after a pre-defined delay or as a dynamic scan using two alternating table positions (“shuttle-mode”). This “shuttle-mode” technique allows visualization of CM bolus over a longer time period (43). Further research is necessary to elucidate the additional impact of this increased radiation exposing technique.
Atrial fibrillation (AF)
Patients with AF were commonly scanned with retrospective ECG-gating with an optimal reconstruction phase at end diastole (44). End-systolic phases are often superior in patients with higher heart rates. To reduce the increased radiation exposure of retrospectively ECG-gated scans, these patients could benefit from low voltage 70-kVp coronary CTA with radiation doses as low as 4.5 mSv (45). However, prospectively ECG-triggered acquisitions, particularly with additional phases, and arrhythmia rejection, have been shown to offer robust, simple, and low-radiation-dose alternatives to traditional retrospective ECG-gating in the setting of arrhythmia (46,47).
Risks of iodine CM
Contrast-induced nephropathy (CIN) and prehydration
The risk of CIN is closely associated with the iodine dose used and preexisting renal function (1). CIN is defined as a serum creatinine concentration increase of at least 25% or 44.2 µmol/L within 3 days after CM injection when there is no other etiology for the increase (48). However, fluctuation of plasma creatinine concentration after CM injection has been reported (49). Nevertheless, persistent renal dysfunction after coronary CTA due to iodine CM is rare (49). In comparison, risk of CIN in multimorbid patients referred for TAVR planning is increased with higher amount of acute kidney injury after the procedure (50). Intravenous prehydration as a prophylaxis has shown no benefit compared to no prophylaxis in the prevention of CIN in high-risk patients (eGFR 30–60 mL/min/1.73m2). Thus, patients with eGFR >29 mL/min/1.73m2 do not benefit from prehydration nor suffer compromised patient safety. However, the amount of iodine should be minimized.
Gadolinium as an alternative?
Gadolinium is an alternative contrast agent in patients with severe iodine allergic reaction (51,52). Due to a necessary high volume with less consistent attenuation compared to iodine and the concerns about gadolinium deposition into the brain, gadolinium is currently no alternative to iodine (51,53-55).
Protocols in focus
Pre-TAVR imaging
TAVR has emerged as an effective treatment alternative for severe aortic stenosis in patients with high-risk for open surgery (56). Pre-procedural CT imaging is integral for TAVR planning and includes two parts: ECG-triggered coronary CTA and non-ECG-gated thoracic-abdominal-pelvis CTA for evaluation of peripheral vascular access (Figure 8) (57). Furthermore, pre-procedural imaging is crucial for device size selection (57). Patients are often multimorbid with consisting nephropathy which makes a careful adjustment of the CM volume all the more crucial. Several trials have shown the feasibility of low-volume CM in TAVR planning with a minimum of 20 mL iodine CM (12,58). Five mL iodine CM were used for a 50% diluted test bolus; biphasic injection protocol with 21 mL 70% diluted CM (15 mL of 370 mgI/mL) followed by saline chaser for CTA acquisition (12). The same CM bolus which were used to visualize coronary arteries were additionally used to visualize the complete aorta and iliac vessels. Careful adjustment of CT settings, especially the pitch, is necessary as a missed bolus or a too fast table movement in patients with low cardiac output easily results in poor enhancement in distal arteries. Other studies reported optimal opacification when using a total amount of 60 mL 350 mgI/mL CM (58). Options to increase lumen enhancement and contrast with simultaneous lowering CM volume would be low tube voltage or spectral CT imaging.

Triple rule-out
Triple-rule-out (TRO) protocols have been designed to evaluate the three major vascular causes of chest pain including CAD, acute aortic syndrome and pulmonary embolism in a single CTA acquisition. For an adequate opacification of three vascular territories including coronary arteries, aorta, and pulmonary arteries, a larger volume of CM was necessary. However, a TRO protocol containing non-ECG-gated chest CTA followed by ECG-gated coronary CTA using a triphasic injection protocol with only 60 mL of 370 mgI/mL CM on a 320-row detector scanner resulted in excellent image quality (59). In that study, bolus tracking technique with a ROI placed in the pulmonary trunk was used. The use of the previous mentioned dual-ROI bolus tracking technique with a first ROI triggering the chest CTA for pulmonary artery evaluation and the second ROI triggering coronary CTA might provide more consistent enhancement in both acquisitions.
Pulmonary vein (PV) imaging
To provide a road map of PV and left atrial (LA) variants in patients with AF, pre-procedural cardiac CT imaging is an alternative modality to MRI and transesophageal echocardiography. It provides assessment of the number of PV and branching pattern, individual PV ostial measurements, measurement of the LA dimensions, and identification of LA abnormalities and pathologies such as appendage thrombus (60) (Figure 9). Due to AF, retrospective gating is common with optimal opacification timed for the left atrium (61).
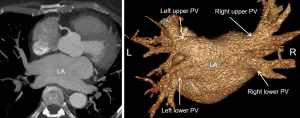
CHD
Improvement in medical care resulted in prolonged life expectancy of patients with CHD (62). These patients need lifelong follow-up with repetitive cardiac function imaging. However, these patients have challenging post-surgical anatomy and hemodynamics. Radiation-free echocardiography is the primary initial diagnostic modality with limitations in reproducibility and right ventricular systolic function imaging (63,64). MRI is an alternative modality, but contraindications due to implanted pacemakers and defibrillators are regularly in adult CHD patients (65). Similar to patients without CHD, adult CHD patients can also suffer from CAD including the emergency setting. Thus, CT imaging and interpretation of these patients are critical but challenging with complex pre- and post-surgical anatomy and hemodynamic. This makes an individual injection and scan protocol necessary (66). Expert consensus of cardiac CT imaging in patients with CHD were provided by the Society of Cardiovascular Computed Tomography (67,68). We recommend the presence of the interpreting physician at the time of scan.
Congenital coronary anomaly
Congenital coronary anomalies are present in 0.2–2% of the population (67). Coronary artery dominance, angulation from the aortic root, ostial narrowing and the presence of intramural course can be optimal visualized by coronary CTA without the adaptation of standard coronary CTA protocol and CM injection protocol. When echocardiographic detected coronary anomalies in children should be confirmed by CT, protocols with low radiation exposure and a CM volume adapted to the children’s size are recommended. Pediatric CTA is typically performed with 1–2 mL/kg total CM volume until standard adult volume is achieved (68). The combined volume load of the CM and saline flush is 2–3 mL/kg and is usually tolerated without hemodynamic consequence.
Septal defects
CT imaging in patients with atrial, ventricular or combined septal defects is rarely needed. Septal defects combined with systemic or pulmonary venous anomalies might need evaluation by CTA in addition to echocardiography (67).
Tetralogy of Fallot (TOF)
Assessment of TOF is usually multimodality based. Cardiac CT provides excellent visualization of the anatomic targets which include pulmonary trunk and branches, aortopulmonary collaterals, coronary arteries, and the aortic root as well as postoperative shunts (67). Therefore, bolus timing is crucial. Postoperative evaluation is usually performed with MRI.
Single ventricle anomaly
The amount of CHD patients with a functional single ventricle and multiple palliative surgical pathways are increasing (67). It is expected that 70% of patients with a systemic RV will survive to adulthood.
Pre-surgical imaging is mostly done by echocardiography. In complex cases, cardiac CT is an excellent modality to visualize crucial pulmonary venous anatomy (68). Several surgical steps are necessary to correct anatomy. After stage 1 (Norwood, hybrid) systemic and pulmonary artery stenoses are common (68). Further, patients with systemic to pulmonary artery shunts occasionally experience shunt thrombosis. If these pathologies can’t be sufficiently visualized with echocardiography, cardiac CT is an optimal alternative. Cardiac CT can also be an alternative to the routinely performed cardiac catheterization before stage 2 procedure (Glenn) with significantly lower radiation exposure and waiver for general anesthesia (68).
When CM injection is performed by an antecubital vein, the RV mixes opacified blood from the superior vena cava (SVC) and unopacified blood from the inferior vena cava (IVC). However, after Glenn procedure pulmonary arteries are only passively supplied by the SVC; blood from the IVC mixes with oxygen-rich blood from the PVs via the enlarged atrial septal defect in the right atrium and returns to the systemic circulation without passing pulmonary circulation. After stage 3 procedure (Fontan), pulmonary arteries are connected to the SVC and IVC (Figure 10). However, there can be discrepancies in this passively driven venous circulation. Commonly seen is the SVC directed towards the right lung and the IVC is directed towards the left lung. Patients with Fontan physiology have been described with an increased incidence of postoperative thromboembolic disease with thrombi commonly in the residual pulmonary artery stump or residual ventricle (67). Suboptimal contrast enhancement might result in non-diagnostic or misdiagnosis.
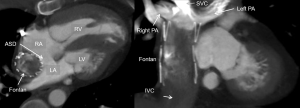
In addition to a bolus tracking technique, best pulmonary artery opacification is being achieved by splitting the CM bolus for combined upper and lower extremity venous injections, preferentially with two separate power injectors (68). However, the mixture of contrast-enhanced blood from the lower injection and unenhanced blood returned from the liver might still result in inhomogeneous opacification and mimic thrombus. A delayed second-phase CT might be necessary if suboptimal opacification on the first-phase CT scan limit interpretation. A dual-ROI bolus triggering might be advantageous in these cases.
Conclusions and outlook
This article gives an overview of the current standard of CM injection in cardiac CT imaging and contrast-enhancement related factors. The improvements in CT technology with increased z-coverage allow visualization of the complete heart during one heart beat which allows a very low contrast volume for précised timed contrast enhancement. Due to several different clinical indications and co-morbidities timing is crucial for optimal visualization. We expect ongoing improvements with intense interaction of CM injection protocols and CT scanner settings. We believe that on the bases of indication (e.g., evaluation of vascular structures), scout (to provide scan length), and a test-bolus, scan duration and automatic selection of tube current and potential will have more influence on an individual amount of iodine CM volume and concentration to minimize both radiation exposure and amount of iodine.
Acknowledgements
None.
Footnote
Conflicts of Interest: B Ghoshhajra is educational consultant for Siemens, Medtronic; JE Scholtz has no conflicts of interest to declare.
References
- Bae KT. Intravenous contrast medium administration and scan timing at CT: considerations and approaches. Radiology 2010;256:32-61. [Crossref] [PubMed]
- Bae KT, Seeck BA, Hildebolt CF, et al. Contrast enhancement in cardiovascular MDCT: effect of body weight, height, body surface area, body mass index, and obesity. AJR Am J Roentgenol 2008;190:777-84. [Crossref] [PubMed]
- Becker CR, Hong C, Knez A, et al. Optimal contrast application for cardiac 4-detector-row computed tomography. Invest Radiol 2003;38:690-4. [Crossref] [PubMed]
- Hutchison SJ, Merchant N. Principles of Cardiac and Vascular Computed Tomography: Expert Consult. Elsevier Health Sciences, 2014.
- Schroeder S, Kopp AF, Baumbach A, et al. Noninvasive detection and evaluation of atherosclerotic coronary plaques with multislice computed tomography. J Am Coll Cardiol 2001;37:1430-5. [Crossref] [PubMed]
- Nikolaou K, Sagmeister S, Knez A, et al. Multidetector-row computed tomography of the coronary arteries: predictive value and quantitative assessment of non-calcified vessel-wall changes. Eur Radiol 2003;13:2505-12. [Crossref] [PubMed]
- Fleischmann D. How to design injection protocols for multiple detector-row CT angiography (MDCTA). Eur Radiol 2005;15 Suppl 5:E60-5. [Crossref] [PubMed]
- Abbara S, Arbab-Zadeh A, Callister TQ, et al. SCCT guidelines for performance of coronary computed tomographic angiography: A report of the Society of Cardiovascular Computed Tomography Guidelines Committee. J Cardiovasc Comput Tomogr 2009;3:190-204. [Crossref] [PubMed]
- Kok M, Kietselaer BL, Mihl C, et al. Contrast Enhancement of the Right Ventricle during Coronary CT Angiography – Is It Necessary? PLoS ONE 2015;10:e0128625. [Crossref] [PubMed]
- Delesalle MA, Pontana F, Duhamel A, et al. Spectral optimization of chest CT angiography with reduced iodine load: experience in 80 patients evaluated with dual-source, dual-energy CT. Radiology 2013;267:256-66. [Crossref] [PubMed]
- Bae KT, Heiken JP, Brink JA. Aortic and hepatic contrast medium enhancement at CT. Part II. Effect of reduced cardiac output in a porcine model. Radiology 1998;207:657-62. [Crossref] [PubMed]
- Azzalini L, Abbara S, Ghoshhajra BB. Ultra-low contrast computed tomographic angiography (CTA) with 20-mL total dose for transcatheter aortic valve implantation (TAVI) planning. J Comput Assist Tomogr 2014;38:105-9. [Crossref] [PubMed]
- Tatsugami F, Awai K, Takada H, et al. Reduction of interpatient variability of arterial enhancement using a new bolus tracking system in 320-detector computed tomographic coronary angiography. J Comput Assist Tomogr 2013;37:79-83. [Crossref] [PubMed]
- Oda S, Utsunomiya D, Yuki H, et al. Low contrast and radiation dose coronary CT angiography using a 320-row system and a refined contrast injection and timing method. J Cardiovasc Comput Tomogr 2015;9:19-27. [Crossref] [PubMed]
- Hell MM, Bittner D, Schuhbaeck A, et al. Prospectively ECG-triggered high-pitch coronary angiography with third-generation dual-source CT at 70 kVp tube voltage: feasibility, image quality, radiation dose, and effect of iterative reconstruction. J Cardiovasc Comput Tomogr 2014;8:418-25. [Crossref] [PubMed]
- Meyersohn NM, Szilveszter B, Staziaki PV, et al. Coronary CT angiography in the emergency department utilizing second and third generation dual source CT. J Cardiovasc Comput Tomogr 2017;11:249-57. [Crossref] [PubMed]
- Scholtz JE, Wichmann JL, Hüsers K, et al. Automated tube voltage adaptation in combination with advanced modeled iterative reconstruction in thoracoabdominal third-generation 192-slice dual-source computed tomography: effects on image quality and radiation dose. Acad Radiol 2015;22:1081-7. [Crossref] [PubMed]
- Scholtz JE, Wichmann JL, Hüsers K, et al. Third-generation dual-source CT of the neck using automated tube voltage adaptation in combination with advanced modeled iterative reconstruction: evaluation of image quality and radiation dose. Eur Radiol 2016;26:2623-31. [Crossref] [PubMed]
- Wu Q, Wang Y, Kai H, et al. Application of 80-kVp tube voltage, low-concentration contrast agent and iterative reconstruction in coronary CT angiography: evaluation of image quality and radiation dose. Int J Clin Pract 2016;70 Suppl 9B:B50-5. [Crossref] [PubMed]
- Sinitsyn VE, Komarova MA, Mershina EA. Comparison of low- and high-concentration (270 and 320 mg I/ml) iso-osmolar iodinated contrast media in coronary CT angiography: a randomized prospective single-center blinded study. Vestn Rentgenol Radiol 2014.5-12. [PubMed]
- Yin WH, Lu B, Gao JB, et al. Effect of reduced x-ray tube voltage, low iodine concentration contrast medium, and sinogram-affirmed iterative reconstruction on image quality and radiation dose at coronary CT angiography: results of the prospective multicenter REALISE trial. J Cardiovasc Comput Tomogr 2015;9:215-24. [Crossref] [PubMed]
- Albrecht MH, Scholtz JE, Hüsers K, et al. Advanced image-based virtual monoenergetic dual-energy CT angiography of the abdomen: optimization of kiloelectron volt settings to improve image contrast. Eur Radiol 2016;26:1863-70. [Crossref] [PubMed]
- Rajiah P, Abbara S, Halliburton S. Spectral detector CT for cardiovascular applications. Ankara, Turkey: Diagnostic and interventional radiology, 2017.
- Marin D, Fananapazir G, Mileto A, et al. Dual-energy multi-detector row CT with virtual monochromatic imaging for improving patient-to-patient uniformity of aortic enhancement during CT angiography: an in vitro and in vivo study. Radiology 2014;272:895-902. [Crossref] [PubMed]
- Martin SS, Albrecht MH, Wichmann JL, et al. Value of a noise-optimized virtual monoenergetic reconstruction technique in dual-energy CT for planning of transcatheter aortic valve replacement. Eur Radiol 2017;27:705-14. [Crossref] [PubMed]
- Dong J, Wang X, Jiang X, et al. Low-contrast agent dose dual-energy CT monochromatic imaging in pulmonary angiography versus routine CT. J Comput Assist Tomogr 2013;37:618-25. [Crossref] [PubMed]
- Apfaltrer P, Sudarski S, Schneider D, et al. Value of monoenergetic low-kV dual energy CT datasets for improved image quality of CT pulmonary angiography. Eur J Radiol 2014;83:322-8. [Crossref] [PubMed]
- Bucher AM, Wichmann JL, Schoepf UJ, et al. Quantitative evaluation of beam-hardening artefact correction in dual-energy CT myocardial perfusion imaging. Eur Radiol 2016;26:3215-22. [Crossref] [PubMed]
- Danad I. Ó Hartaigh B, Min JK. Dual-energy computed tomography for detection of coronary artery disease. Expert Rev Cardiovasc Ther 2015;13:1345-56. [Crossref] [PubMed]
- Ananthakrishnan L, Rajiah P, Ahn R, et al. Spectral detector CT-derived virtual non-contrast images: comparison of attenuation values with unenhanced CT. Abdom Radiol (NY) 2017;42:702-9. [Crossref] [PubMed]
- Belge B, Coche E, Pasquet A, et al. Accurate estimation of global and regional cardiac function by retrospectively gated multidetector row computed tomography: comparison with cine magnetic resonance imaging. Eur Radiol 2006;16:1424-33. [Crossref] [PubMed]
- Takx RA, Moscariello A, Schoepf UJ, et al. Quantification of left and right ventricular function and myocardial mass: comparison of low-radiation dose 2nd generation dual-source CT and cardiac MRI. Eur J Radiol 2012;81:e598-604. [Crossref] [PubMed]
- Seneviratne SK, Truong QA, Bamberg F, et al. Incremental diagnostic value of regional left ventricular function over coronary assessment by cardiac computed tomography for the detection of acute coronary syndrome in patients with acute chest pain: from the ROMICAT trial. Circ Cardiovasc Imaging 2010;3:375-83. [Crossref] [PubMed]
- Feuchtner G, Goetti R, Plass A, et al. Adenosine stress high-pitch 128-slice dual-source myocardial computed tomography perfusion for imaging of reversible myocardial ischemia: comparison with magnetic resonance imaging. Circ Cardiovasc Imaging 2011;4:540-9. [Crossref] [PubMed]
- Ko BS, Cameron JD, Defrance T, et al. CT stress myocardial perfusion imaging using multidetector CT--A review. J Cardiovasc Comput Tomogr 2011;5:345-56. [Crossref] [PubMed]
- Okada DR, Ghoshhajra BB, Blankstein R, et al. Direct comparison of rest and adenosine stress myocardial perfusion CT with rest and stress SPECT. J Nucl Cardiol 2010;17:27-37. [Crossref] [PubMed]
- Ho KT, Chua KC, Klotz E, et al. Stress and rest dynamic myocardial perfusion imaging by evaluation of complete time-attenuation curves with dual-source CT. JACC Cardiovasc Imaging 2010;3:811-20. [Crossref] [PubMed]
- Tamarappoo BK, Dey D, Nakazato R, et al. Comparison of the extent and severity of myocardial perfusion defects measured by CT coronary angiography and SPECT myocardial perfusion imaging. JACC Cardiovasc Imaging 2010;3:1010-9. [Crossref] [PubMed]
- Patel AR, Lodato JA, Chandra S, et al. Detection of myocardial perfusion abnormalities using ultra-low radiation dose regadenoson stress multidetector computed tomography. J Cardiovasc Comput Tomogr 2011;5:247-54. [Crossref] [PubMed]
- Bamberg F, Becker A, Schwarz F, et al. Detection of hemodynamically significant coronary artery stenosis: incremental diagnostic value of dynamic CT-based myocardial perfusion imaging. Radiology 2011;260:689-98. [Crossref] [PubMed]
- Techasith T, Cury RC. Stress Myocardial CT PerfusionAn Update and Future Perspective. JACC Cardiovascular Imaging 2011;4:905-16. [Crossref] [PubMed]
- Rossi A, Merkus D, Klotz E, et al. Stress Myocardial Perfusion: Imaging with Multidetector CT. Radiology 2014;270:25-46. [Crossref] [PubMed]
- Baxa J, Hromádka M, Šedivý J, et al. Regadenoson-Stress Dynamic Myocardial Perfusion Improves Diagnostic Performance of CT Angiography in Assessment of Intermediate Coronary Artery Stenosis in Asymptomatic Patients. Biomed Res Int 2015;2015:105629. [Crossref] [PubMed]
- Oda S, Honda K, Yoshimura A, et al. 256-Slice coronary computed tomographic angiography in patients with atrial fibrillation: optimal reconstruction phase and image quality. Eur Radiol 2016;26:55-63. [Crossref] [PubMed]
- Meyer M, Haubenreisser H, Schoepf UJ, et al. Radiation Dose Levels of Retrospectively ECG-Gated Coronary CT Angiography Using 70-kVp Tube Voltage in Patients with High or Irregular Heart Rates. Acad Radiol 2017;24:30-7. [Crossref] [PubMed]
- Lee AM, Beaudoin J, Engel LC, et al. Assessment of image quality and radiation dose of prospectively ECG-triggered adaptive dual-source coronary computed tomography angiography (cCTA) with arrhythmia rejection algorithm in systole versus diastole: a retrospective cohort study. Int J Cardiovasc Imaging 2013;29:1361-70. [Crossref] [PubMed]
- Lee AM, Engel LC, Hui GC, et al. Coronary computed tomography angiography at 140 kV versus 120 kV: assessment of image quality and radiation exposure in overweight and moderately obese patients. Acta Radiologica 2014;55:554-62. [Crossref] [PubMed]
- Morcos SK, Thomsen HS, Webb JA. Contrast-media-induced nephrotoxicity: a consensus report. Contrast Media Safety Committee, European Society of Urogenital Radiology (ESUR). Eur Radiol 1999;9:1602-13. [Crossref] [PubMed]
- Maaniitty T, Stenström I, Uusitalo V, et al. Incidence of persistent renal dysfunction after contrast enhanced coronary CT angiography in patients with suspected coronary artery disease. Int J Cardiovasc Imaging 2016;32:1567-75. [Crossref] [PubMed]
- Belardi JA, Albertal M. Acute kidney injury after TAVI: Predict, detect, and prevent. Catheter Cardiovasc Interv 2016;87:532-3. [Crossref] [PubMed]
- Carrascosa P, Capunay C, Bettinotti M, et al. Feasibility of gadolinium-diethylene triamine pentaacetic acid enhanced multidetector computed tomography for the evaluation of coronary artery disease. J Cardiovasc Comput Tomogr 2007;1:86-94. [Crossref] [PubMed]
- Coche EE, Hammer FD, Goffette PP. Demonstration of pulmonary embolism with gadolinium-enhanced spiral CT. Eur Radiol 2001;11:2306-9. [Crossref] [PubMed]
- McDonald RJ, McDonald JS, Kallmes DF, et al. Intracranial Gadolinium Deposition after Contrast-enhanced MR Imaging. Radiology 2015;275:772-82. [Crossref] [PubMed]
- Kanda T, Matsuda M, Oba H, et al. Gadolinium Deposition after Contrast-enhanced MR Imaging. Radiology 2015;277:924-5. [Crossref] [PubMed]
- Radbruch A, Weberling LD, Kieslich PJ, et al. High-Signal Intensity in the Dentate Nucleus and Globus Pallidus on Unenhanced T1-Weighted Images: Evaluation of the Macrocyclic Gadolinium-Based Contrast Agent Gadobutrol. Invest Radiol 2015;50:805-10. [Crossref] [PubMed]
- Smith CR, Leon MB, Mack MJ, et al. Transcatheter versus Surgical Aortic-Valve Replacement in High-Risk Patients. N Engl J Med 2011;364:2187-98. [Crossref] [PubMed]
- Achenbach S, Delgado V, Hausleiter J, et al. SCCT expert consensus document on computed tomography imaging before transcatheter aortic valve implantation (TAVI)/transcatheter aortic valve replacement (TAVR). J Cardiovasc Comput Tomogr 2012;6:366-80. [Crossref] [PubMed]
- Bittner DO, Arnold M, Klinghammer L, et al. Contrast volume reduction using third generation dual source computed tomography for the evaluation of patients prior to transcatheter aortic valve implantation. Eur Radiol 2016;26:4497-504. [Crossref] [PubMed]
- Durmus T, Rogalla P, Lembcke A, et al. Low-dose triple-rule-out using 320-row-detector volume MDCT – less contrast medium and lower radiation exposure. Eur Radiol 2011;21:1416-23. [Crossref] [PubMed]
- Cury RC, Abbara S, Schmidt S, et al. Relationship of the esophagus and aorta to the left atrium and pulmonary veins: implications for catheter ablation of atrial fibrillation. Heart Rhythm 2005;2:1317-23. [Crossref] [PubMed]
- Lacomis JM, Wigginton W, Fuhrman C, et al. Multi–Detector Row CT of the Left Atrium and Pulmonary Veins before Radio-frequency Catheter Ablation for Atrial Fibrillation 1. Radiographics 2003;23:S35-48. [Crossref] [PubMed]
- Baumgartner H, Bonhoeffer P, De Groot NM, et al. ESC Guidelines for the management of grown-up congenital heart disease (new version 2010). Eur Heart J 2010;31:2915-57. [Crossref] [PubMed]
- Margossian R, Schwartz ML, Prakash A, et al. Comparison of Echocardiographic and Cardiac Magnetic Resonance Imaging Measurements of Functional Single Ventricular Volumes, Mass, and Ejection Fraction (from the Pediatric Heart Network Fontan Cross-Sectional Study). Am J Cardiol 2009;104:419-28. [Crossref] [PubMed]
- Puchalski MD, Williams RV, Askovich B, et al. Assessment of Right Ventricular Size and Function: Echo Versus Magnetic Resonance Imaging. Congenit Heart Dis 2007;2:27-31. [Crossref] [PubMed]
- Han BK, Hlavacek AM, Kay WA, et al. Multi-institutional evaluation of the indications and radiation dose of functional cardiovascular computed tomography (CCT) imaging in congenital heart disease. Int J Cardiovasc Imaging 2016;32:339-46. [Crossref] [PubMed]
- Ghoshhajra BB, Sidhu MS, El-Sherief A, et al. Adult congenital heart disease imaging with second-generation dual-source computed tomography: initial experiences and findings. Congenit Heart Dis 2012;7:516-25. [Crossref] [PubMed]
- Han BK, Rigsby CK, Hlavacek A, et al. Computed Tomography Imaging in Patients with Congenital Heart Disease Part I: Rationale and Utility. An Expert Consensus Document of the Society of Cardiovascular Computed Tomography (SCCT): Endorsed by the Society of Pediatric Radiology (SPR) and the North American Society of Cardiac Imaging (NASCI). J Cardiovasc Comput Tomogr 2015;9:475-92. [Crossref] [PubMed]
- Han BK, Rigsby CK, Leipsic J, et al. Computed Tomography Imaging in Patients with Congenital Heart Disease, Part 2: Technical Recommendations. An Expert Consensus Document of the Society of Cardiovascular Computed Tomography (SCCT): Endorsed by the Society of Pediatric Radiology (SPR) and the North American Society of Cardiac Imaging (NASCI). J Cardiovasc Comput Tomogr 2015;9:493-513. [Crossref] [PubMed]