Can thrombus age guide thrombolytic therapy?
Introduction
Venous thrombosis (VT) is a common clinical condition with a significant financial burden on the US healthcare system. Despite its prevalence, the diagnosis and management VT remains rudimentary (1-4). Anticoagulation and thrombolytic therapies are the mainstay of VT treatment despite a rapid decrease in their effectiveness as thrombus composition evolves with age, and are most effective in the acute phase. An understanding of the biology of thrombosis will allow for more effective tailored therapy for VT (5).
Cases of VT in the US exceed 1 million per year, most commonly occurring in the lower extremities (1,4). The etiology of VT is multifactorial, involving risk factors described by Virchow including venous endothelial injury (e.g., trauma or surgery), hypercoagulability (cancer, pregnancy, protein C mutations), and venous stasis (damage to venous valves, immobility, previous thrombus), as well as intrinsic factors such as genetics (2,5). VT can lead to a myriad of complications including swelling, erythema, neurovascular compromise, threatened loss of limb, acute respiratory symptoms, pulmonary hypertension, cardiovascular collapse, thromboembolism and death. While most cases of VT resolve with treatment, residual fibrotic changes persist in many patients (1,6). As a thrombus matures, fibrosis can cause post-thrombotic syndrome (PTS) and, when the venous valves are involved, it can result in valvular incompetence. This can lead to chronic pain, heaviness, leg cramping, limb edema, stasis dermatitis and, at end stage, venous ulcerations. No effective treatment exists for PTS, and incidence is as high as 60% at 2 years when acute VT is treated with anticoagulation therapy alone (6,7).
The morbidity and mortality following acute pulmonary embolism (PE) is likely even higher. A minority of patients can develop chronic thromboembolic pulmonary hypertension (CTEPH) following PE, defined by pre-capillary pulmonary hypertension from vascular scarring (8). While the incidence of CTEPH following acute PE is believed to be between 0.4–8.8% (9-15), it is estimated that nearly one half of patients with PE experience some form of chronic dyspnea (16), with roughly the same proportion of patients have persistent defects on pulmonary V/Q scan chronically after treatment (17,18). The morbidity with this chronic dyspnea is significant, with decreased exercise tolerance based on 6-minute walk tests and right ventricular dysfunction or hypokinesis in nearly 20% of patients (18,19).
Anticoagulation and thrombolytic therapies are the mainstay of treatment despite a rapid decrease in effectiveness as chronic thrombus transformation evolves (5). The anticoagulation therapy alone may prevent thrombus propagation and recurrence or PE; however, it does not always provide an active and complete thrombus resolution which might require additional therapeutic adjuncts (8-10). Accurate early diagnosis and effective intervention are essential for decreasing mortality and immediate or long-term associated morbidities (7,20). Due to the dynamic nature of thrombosis, the age and composition of a thrombus are critical to its management (20,21). Here, we discuss temporal thrombus modifications and how this transformation may mirror imaging characteristics, therapeutics performance, and clinical outcome.
Outcomes and current guidelines
Venous disease presentation can be variable from asymptomatic to subclinical to symptomatic, depending on the extent of thrombotic lesion, location, number of sufficient collaterals, and the severity of the vascular disease occlusion and associated inflammation. Acute thrombi usually respond well to anticoagulant and thrombolytic therapies and result in restored venous function. However, in certain pathologic conditions, the thrombolytic process gets thwarted, resulting in a refractory thrombus which is difficult to manage clinically (8). A large body of literature acknowledges the steady transformation of VT over time (2,4,22,23). As a thrombus matures it undergoes reorganization in a process that resembles wound healing (24-26). Leukocytes and other inflammatory cells infiltrate the thrombus; cellular components are replaced by collagen deposits, and a neovascular network is formed (27,28). These processes alter the composition and properties of the thrombus, provoking its resistance to thrombolytic therapies (2,8,20). Therefore, the earlier anticoagulation therapies are employed, the better the outcome for the patient. For example, one study showed that patients with deep vein thrombosis (DVT) who receive therapeutic levels of anticoagulation therapy within the first 24 hours of presentation had a 17–19% decrease in VT recurrence compared to those who received therapy after 24 hours (29). The role of early thrombolytics in the setting of VTE and PE is less clear and the focus of much research. The most recent Cochrane review article cited that the incidence of PTS after acute VT decreased by 1/3 with thrombolytic therapy, but found little difference between systemic versus catheter directed therapy (30). Unfortunately, early results from the RTC ATTRACT trial, which compared catheter directed therapy to anticoagulation alone, did not demonstrate a significant difference between these two therapies in the incidence of PTS (31). Several recent meta-analyses have concluded somewhat conflicting results on the use of thrombolytics in the setting of acute PE, with the most recent review with Gao et al. concluding a lower all-cause mortality in treatment of acute intermediate risk PE compared to anticoagulation alone, and more remote analyses found no significant difference between the two treatments (32,33).
Thrombus resolution with anticoagulation alone proceeds slowly (34). Studies show that ~50% of patients on long-term anticoagulation alone continue to have abnormal venous hemodynamics on ultrasound imaging 1 year after diagnosis and treatment (35). Compared to acute thrombi, which are at high risk for fragmentation and embolization, residual chronic thrombi are stable and resistant to both anticoagulation and thrombolytic treatment (29,36,37). Several studies have described the hardening of chronic thrombi due in large part to the cross linking of fibrin and replacement of cellular material by collagen (29,36,38). By 1 week, thrombus collagen content may reach approximately 20%, and after three weeks it may be as high as 80% (25). While the process of thrombus maturation is variable, it is approximated that thrombi 15 days of age or older will undergo significant modification and can be considered chronic (29,39). These chronic thrombi become increasingly resistant to both anticoagulation and thrombolytic therapies (5).
Barring any contraindications, anticoagulation is the current recommended first-line treatment for VT. Most anticoagulation therapy usually proceeds in two stages: initial treatment, followed by long-term maintenance therapy, traditionally with vitamin K antagonists (VKA) or low molecular weight heparin (LMWH) (20,40). In acute thrombi, anticoagulation prevents progressive fibrin cross-linking and thrombus propagation, allowing natural thrombolytic mechanisms to proceed. Early administration of anticoagulation therapy reduces average VT recurrence rate from 12.9% in the first year to 5.4% (1,4), with a potential 1.9% risk of bleeding (40,41). Despite its rapid effect on fibrin, anticoagulants do not affect the cellular or connective tissue components of thrombi (5). Thus, no consensus exists regarding how long anticoagulation therapy should be continued.
While therapeutic anticoagulation helps achieve venous patency and lowers the risk of recurrence, patients treated with anticoagulation alone remain at high risk for post thrombotic syndrome (PTS) (42,43). Previous studies have suggested that incidence of PTS is greatly decreased when anticoagulation is used in conjunction with mechanical or pharmaceutical thrombolysis however, early results from the ATTRACT trial did not demonstrate a significant difference between these two therapies (30,31). However, therapy with ultrasound assisted thrombolytic catheters have shown no difference compared to conventional catheter directed therapy in a recent RTC (44). While thrombolytic therapy has been shown to improve short term hemodynamic benefit, no studies to date have shown a decreased incidence of CTEPH or improvement in subsequent pulmonary V/Q scans with combined therapy of thrombolytics and anticoagulation versus anticoagulation alone (45-47). It is unclear if this relates to the unique immunologic and physiologic environment within the lungs and is an area that warrants further research.
Currently, thrombolytic therapy is only recommended in patients with neurovascular compromise and limb threatening ischemia (swollen and blue or white limb) in the setting of VT, or hemodynamic instability (hypotension and tachycardia) and/or evidence of right heart strain based on echocardiography in the setting of PE (48,49). Thrombolytic agents can be administered systemically or locally via a catheter based delivery technique to actively degrade cross-linked fibrin (50,51). In contrast, mechanical thrombolysis is not routinely used as a first-line for VT management due to increased risk of bleeding (8% when using tPA in combination with mechanical thrombosis) and lack of prospective randomized control studies (21,40). As with anti-coagulative therapies, increased connective tissue in the aging thrombus decreases the effectiveness of thrombolytic therapies significantly (36). Thus it is critical that the most appropriate VT treatment be considered in the context of thrombus age and thrombus tissue characteristics.
Four stages of thrombus evolution
Chronological transformation of the thrombus is a spectrum of continuous modification which progresses through four stages: induction, acute fibrin dominant, intermediate and chronic connective tissue dominant stages. While one phase may dominate, all four are likely to be present to varying degrees in any given thrombus (2,35). Currently, clinical history is the primary method of determining the age of a thrombus. However, collateral venous circulation may cause VT to be asymptomatic for days or weeks, making patient history an inconsistent tool for assessing thrombus age (35,40). Inherent patient-to-patient variation also creates difficulty in standardizing thrombus age chronologically. Therefore, it is useful to include thrombus composition in our definition of age (22,39). Ideally, as composition evolves over time, so should therapy. For each phase, the histological, molecular, biochemical, and clinical factors are discussed. Pharmaceutical and imaging strategies are examined separately.
Stage I: induction
The initiation of VT is multifactorial and poorly understood, but results in disruption of the homeostatic balance between thrombosis and thrombolysis, ultimately favoring thrombogenesis (1). Once a small nidus has formed, generally around a vascular defect, venous valve or area of stasis, thrombin rapidly converts fibrinogen to fibrin, and fibrin polymerization is potentiated via positive feedback to create a loose fibrin network (2,22). Initiation also triggers an inflammatory response resulting in a cascade of cytokines, further perpetuating coagulation as well as potentially initiating thrombus resolution (24,26). This process is generally rapid and subclinical but sets the stage for either thrombus propagation or resolution.
Stage II: fibrin-dominant, acute thrombus
Following initiation, acute thrombi are comprised primarily of two components, dense fibrin mesh and cell layers (Figure 1). The inflammatory cytokine cascade that began in the induction phase continues and increases. As activated fibrin polymerizes and begins to form crosslinks, red blood cells (RBCs) and, to a lesser degree, platelets become trapped within the thrombus (2). Continuous intravascular flow causes cells and fibrin to be deposited in alternating layers called Zahn’s lines, a classical finding in VT (2,52,53). The fibrin component of an acute thrombus is continuously being remodeled, undergoing both polymerization and degradation. This makes the acute thrombus highly sensitive to anticoagulation and thrombolytic therapies, which promote fibrin breakdown (35,54). However, fibrinolysis does not break down cellular components of the thrombus. RBCs continue to constitute the bulk of acute thrombi, while platelets, activated by tissue factor and other inflammatory signals, express P-selectin, helping to facilitate the infiltration of leukocytes. Neutrophils are the first leukocytes to get recruited, followed by monocytes and differentiated macrophages (25). As these cells infiltrate and become activated, the thrombus becomes increasingly resistant to anticoagulation and thrombolytic therapies (52,55).
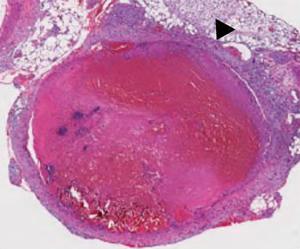
During this stage, an increasing number of neutrophils and monocytes can be seen in the thrombus, but no fibrotic changes are noted. The growing numbers of neutrophils augment and mediate both the plasmin system and deposition of matrix metalloproteinases (MMPs) which help in the remodeling of the extracellular matrix with the thrombus (56). The presence of monocytes also augments the plasmin system, and can degrade fibrin even in the absence of plasmin (57). Collagen is rarely visible, and the thrombus structure is dominated by fibrin and cellular materials, including a network of histone and extracellular DNA (8,58). Hemosiderin-positive macrophages, indicative of RBC breakdown, are also rarely detected in this stage. On average, the fibrin dominant thrombus persists for approximately 5 to 7 days (20).
Stage III: intermediate thrombus
The intermediate thrombus (Figure 2) is marked by the appearance of thrombus organization with the presence of poorly organized collagen deposits, initiation of neovascularization, and increased leukocyte infiltration (25,27). Circulating bone marrow progenitor stem cells migrate to the thrombus and differentiate into fibroblasts and vascular smooth muscle cells. The increased fibroblast presence in the thrombus during this stage mediates a fibroproliferative response. Monocyte chemoattractant-1 (MCP), lysyl oxidase, and transforming growth factor-β (TGF-β) are released, facilitating collagen synthesis and deposition. As connective tissue transformation increases, fibrinolysis gradually declines, which mirrors a progressive decrease in circulating D-dimer levels, the soluble products of degraded fibrin (24,59). Recruited neutrophils in the thrombus tissue actively release additional pro-inflammatory mediators as well as nuclear material to form neutrophil extracellular traps (NETs), which is suggested to contribute to thrombus stability and chronic remodeling (52,60). It has been shown that the presence of neutrophils during this stage is crucial to thrombus organization, and also appears to play a role in muscle fiber injury through a Toll-like receptor 4 pathway (61). Neutropenic animal models have demonstrated increased venous wall stiffness with collagen deposition, increased profibrotic growth factors during remodeling and altered release of MMPs and uPA when compared to control models (56). During this transitional stage, loosely organized collagen can be visible, and the number of leukocytes increases, predominantly monocytes and macrophages. Hemosiderin-stained macrophages can be readily identified, as significant RBC breakdown occurs (26). The thrombus appears more organized 7 to 10 days after presentation, classically beginning at the periphery of the thrombus and working inward with great variation (52).
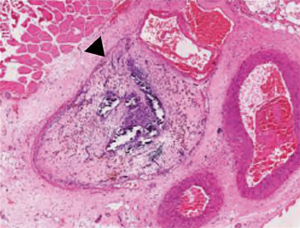
Stage IV: organized chronic thrombus
As the process of thrombus organization continues, collagen deposition becomes more visible and structured. Leukocyte infiltration shifts toward macrophages, and if present, the micro vessel network becomes well defined (25). At this point the bulk of the fibrin within the thrombus is cross-linked and resistant to lysis (51,62). Fibrinolysis occurs only at low levels, associated with low plasma levels of D-dimer. Only two-fifths of patients have measurable levels of D-dimer by the end of the first month, and this decreases to one-tenth of patients by the end of the third month. Persistent fibrinolysis beyond 3 months is usually associated with thrombus recurrence (2). In a recent study, Savchekno et al. provided evidence that NETs play a significant and previously obscure role in the stability of human thrombi during this stage of thrombus remodeling (52). Activated neutrophils within an aging thrombus release highly negatively charged genomic DNA tethered with histones or other thrombogenic nuclear or cytoplasmic proteins, which form web-like structures in response to inflammatory stimuli. Furthermore, in vivo studies have shown that NETs appear to bind to RBCs and promote platelet aggregation (60). This contributes to thrombus stability and produces a pro-thrombogenic environment independent of fibrin degradation, making older clots highly resistant to thrombolytic therapy (50).
In the organized thrombus, the thrombus becomes primarily acellular connective tissue, which is incorporated into the venous wall and become endothelialized. This stage of chronic thrombus resolution is slow, beginning with the end of fibrinolysis (1–3 months). Beyond seven months after thrombus formation, any residual venous occlusion will be a result of thrombus remodeling to a permanent post thrombotic scar (2,63). The incorporation of the scar tissue decreases the compliance of the normally thin walled vein, which can serve as a nidus for future thrombus formation.
Stage-specific therapy
Three main therapeutic goals exist in the treatment of VT: slowing the deposition of new fibrin, degrading the existing clot, and preventing distant thromboembolism. Therapeutic strategies are commonly used in combination to accomplish these goals (5,64,65).
Incipient clots are mostly asymptomatic, and this phase is the definitive target of prophylactic anticoagulation in high risk patients (66-71). Therapeutic anticoagulation is currently recommended for all stages of VT and works to inhibit propagation of the fibrin framework (5,20). Common anticoagulants such as unfractionated heparin (UFH), LMWH, and VKA are most effective on acute and subacute thrombi (41,72). As LMWHs and VKAs do not act on polymerized, clot-bound fibrin, they are less effective in chronic thrombi (31). Recently, novel non-vitamin-K-antagonist oral anticoagulants (NOACs), such as apixaban, dabigatran, edoxaban, and rivaroxaban have come into clinical practice, and studies have found all to be non-inferior to current standards (20,73-76). These new agents have a wider therapeutic window compared to VKAs and do not require frequent monitoring of INR given their comparative resistance to small dietary changes. These agents have become increasingly safer as more and more reversal agents enter the market, and a recent meta-analysis has suggested that patients on NOACs that present with bleeding events have a less severe presentation and similar course compared with VKAs (74,77).
In the case of patients at high risk for cardiopulmonary or neurovascular compromise, arterial thrombus or those with unique anatomic variations including atresia or agenesis of the IVC, either pharmaceutical or mechanical thrombolysis should be considered in combination with anticoagulation (20,21,78-81). Venous, catheter-directed thrombolysis (CDT) with exogenous tPA actively degrades fibrin crosslinks and decreases both the density and complexity of the thrombus as measured by fractal dimension (5,21,78). In thrombi less than 2 weeks old, CDT has shown some success at increasing venous patency and decreasing incidence of PTS than anticoagulation alone (20,21), although early results from the ATTRACT trial did not demonstrate a significant difference between these two therapies (31). Although the proportion of patients that experienced PTS between the two groups was not significantly different, smaller studies have demonstrated that mechanical thrombolysis shows better outcomes in chronic thrombi than CDT treatment alone. In one study, 90% of patients demonstrated a high degree of thrombolysis and venous patency as well as decreased recurrence at 1 year with CDT and mechanical thrombolysis together (21). While CDT and mechanical thrombolysis offer more options to physicians, especially for the treatment of chronic thrombi, they carry a high risk of bleeding complications, reported as high as 8% (21).
More novel therapies are being explored beyond standard anticoagulation and thrombolysis, particularly with the development of more precise in vitro 3D printed thrombus models (82). Given the expression of fibrinolytic promotors by monocytes in the aging thrombus, early animal models have suggested that thrombus organization can be accelerated with injection of monocyte chemotactic protein-1 (MCP-1) (83,84). It is possible that MCP-1 could play a role in future catheter directed therapy in the treatment of VT and prevention of PTS, and may supplement thrombolytic therapy should recanalization fail. Additionally, given the network of extracellular DNA and histones in acute thrombus, the use of deoxyribonuclease enzymes may be an additional future tool in catheter directed therapy (58).
Stage-specific imaging
Selection of effective VT therapy depends on many factors including the accurate determination of thrombus age and maturity. Numerous imaging modalities exist to diagnose and evaluate VT. However, to date no single method has been validated or standardized for clinical use.
Computed tomography venography (CTV) has historically been considered the gold standard in the diagnosis of most forms of VT (Figure 3). Due to high cost, invasiveness, and risk of contrast-related complications, this test is less frequently used clinically (78). Conversely, Doppler ultrasound is inexpensive, non-invasive, accurate, and does not utilize ionizing radiation, and has become the most widely used imaging technique in the diagnosis of peripheral VT (29,38,85). Although Doppler ultrasound can be used to differentiate between acute and chronic thrombus (Figure 4), patient habitus, acoustic windows and edema within the soft tissues make differentiate unrealizable. Furthermore, while CTV and Doppler ultrasound can identify a thrombus, they provide no clinical information about thrombus composition (38,40). Recently, ultrasound elastography has emerged as a possible modality for determining thrombus age by non-invasive imaging. As thrombi age, they become mechanically less compliant due to steady fibrin cross linking and progressive collagen deposition over time (29,86). Mature thrombi, which are rich in collagen and cross-linked fibrin, harden and show a progressive decrease in elasticity. Elastography, which relies on the Young modulus and strain measurements, can both qualitatively and semi-quantitatively characterize the elastic properties of tissues, and is useful for determining the pathologic age of a thrombus. While this has shown promise in animal models, the heterogeneity and significant variability within a thrombus may result in variable elastogram readings as portions of a thrombus may be at different stages of remodeling, a clear limitation should this be adapted for clinical use (86,87). As an additional adjunct, advances are being made with nuclear imaging to provide temporal information about thrombus composition (88,89). Uptake has been demonstrated in acute thrombus on F-18 FDG PET/CT scans due to the associated inflammatory response. As the thrombus organizes and the inflammation decreases, radiotracer uptake similarly decreases, allowing temporal information about the clot. Recently, shear wave magnetic resonance elastography has been tested for potentially assessing thrombus age by visualizing the heterogeneity and the mechanical properties of thrombi and predicting thrombi breakage, as well as in monitoring thrombolytic therapy (90,91).
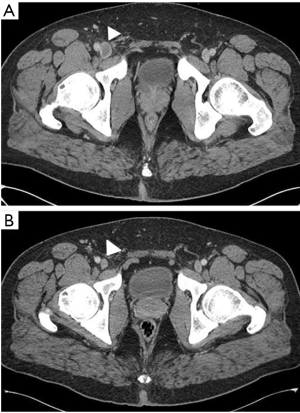
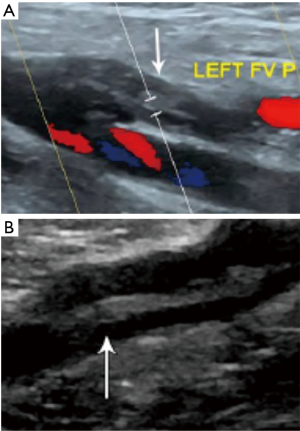
There is a growing need to develop better novel minimally invasive imaging techniques and diagnostic tools to accurately determine the age of the venous thrombus. New laboratory techniques must be developed, refined, and standardized in order to fulfil this goal (89). More studies are needed to validate proper imaging techniques and usher them into mainstream clinical practice. This will improve efficacy of current VT treatments and help eliminate adverse outcomes.
Conclusions
While our understanding of thrombosis is not complete, the concept that thrombus structural evolution during chronic transformation is not novel (5). Better clinical markers of thrombus maturity are necessary, as this understanding dictates whether anticoagulation, pharmacological thrombolysis, mechanical thrombolysis, or a combination of these is needed. Understanding the age of the thrombus will allow physicians to balance the benefits and risks of treatment, as well as the likelihood of treatment success. Pharmaceutical advances and recent evidence regarding the biology of thrombi are changing clinical practice. It is time to reassess our treatment of VT and move to an era of tailored therapy that integrates current knowledge and best practices and focus efforts on novels therapies to better address the collagen rich chronic thrombi that have proven more difficult to treat.
Acknowledgements
Funding: R Oklu gratefully acknowledge funding from the National Institutes of Health (EB021148, CA172738, EB024403, HL137193) and the Mayo Clinic.
Footnote
Conflicts of Interest: The authors have no conflicts of interest to declare.
References
- Heit JA. The epidemiology of venous thromboembolism in the community. Arterioscler Thromb Vasc Biol 2008;28:370-2. [Crossref] [PubMed]
- Morris TA. Natural history of venous thromboembolism. Crit Care Clin 2011;27:869-84. vi. [Crossref] [PubMed]
- Spyropoulos AC, Lin J. Direct medical costs of venous thromboembolism and subsequent hospital readmission rates: an administrative claims analysis from 30 managed care organizations. J Manag Care Pharm 2007;13:475-86. [Crossref] [PubMed]
- White RH. The epidemiology of venous thromboembolism. Circulation 2003;107:I4-8. [Crossref] [PubMed]
- Stanford SN, Sabra A, D'Silva L, et al. The changes in clot microstructure in patients with ischaemic stroke and the effects of therapeutic intervention: a prospective observational study. BMC Neurol 2015;15:35. [Crossref] [PubMed]
- Henke PK, Comerota AJ. An update on etiology, prevention, and therapy of postthrombotic syndrome. J Vasc Surg 2011;53:500-9. [Crossref] [PubMed]
- Kahn SR, Shrier I, Julian JA, et al. Determinants and time course of the postthrombotic syndrome after acute deep venous thrombosis. Ann Intern Med 2008;149:698-707. [Crossref] [PubMed]
- Fernandes T, Planquette B, Sanchez O, et al. From Acute to Chronic Thromboembolic Disease. Ann Am Thorac Soc 2016;13 Suppl 3:S207-14. [Crossref] [PubMed]
- Becattini C, Agnelli G, Pesavento R, et al. Incidence of chronic thromboembolic pulmonary hypertension after a first episode of pulmonary embolism. Chest 2006;130:172-5. [Crossref] [PubMed]
- Guerin L, Couturaud F, Parent F, et al. Prevalence of chronic thromboembolic pulmonary hypertension after acute pulmonary embolism. Prevalence of CTEPH after pulmonary embolism. Thromb Haemost 2014;112:598-605. [Crossref] [PubMed]
- Klok FA, van Kralingen KW, van Dijk AP, et al. Prospective cardiopulmonary screening program to detect chronic thromboembolic pulmonary hypertension in patients after acute pulmonary embolism. Haematologica 2010;95:970-5. [Crossref] [PubMed]
- Korkmaz A, Ozlu T, Ozsu S, et al. Long-term outcomes in acute pulmonary thromboembolism: the incidence of chronic thromboembolic pulmonary hypertension and associated risk factors. Clin Appl Thromb Hemost 2012;18:281-8. [Crossref] [PubMed]
- Pengo V, Lensing AW, Prins MH, et al. Incidence of chronic thromboembolic pulmonary hypertension after pulmonary embolism. N Engl J Med 2004;350:2257-64. [Crossref] [PubMed]
- Poli D, Grifoni E, Antonucci E, et al. Incidence of recurrent venous thromboembolism and of chronic thromboembolic pulmonary hypertension in patients after a first episode of pulmonary embolism. J Thromb Thrombolysis 2010;30:294-9. [Crossref] [PubMed]
- Surie S, Gibson NS, Gerdes VE, et al. Active search for chronic thromboembolic pulmonary hypertension does not appear indicated after acute pulmonary embolism. Thromb Res 2010;125:e202-5. [Crossref] [PubMed]
- Kim NH, Delcroix M, Jenkins DP, et al. Chronic thromboembolic pulmonary hypertension. J Am Coll Cardiol 2013;62:D92-9. [Crossref] [PubMed]
- Cosmi B, Nijkeuter M, Valentino M, et al. Residual emboli on lung perfusion scan or multidetector computed tomography after a first episode of acute pulmonary embolism. Intern Emerg Med 2011;6:521-8. [Crossref] [PubMed]
- Sanchez O, Helley D, Couchon S, et al. Perfusion defects after pulmonary embolism: risk factors and clinical significance. J Thromb Haemost 2010;8:1248-55. [Crossref] [PubMed]
- Stevinson BG, Hernandez-Nino J, Rose G, et al. Echocardiographic and functional cardiopulmonary problems 6 months after first-time pulmonary embolism in previously healthy patients. Eur Heart J 2007;28:2517-24. [Crossref] [PubMed]
- Hillis C, Crowther MA. Acute phase treatment of VTE: Anticoagulation, including non-vitamin K antagonist oral anticoagulants. Thromb Haemost 2015;113:1193-202. [Crossref] [PubMed]
- Oklu R, Wicky S. Catheter-directed thrombolysis of deep venous thrombosis. Semin Thromb Hemost 2013;39:446-51. [Crossref] [PubMed]
- van Rij AM, Hill G, Krysa J, et al. Prospective study of natural history of deep vein thrombosis: early predictors of poor late outcomes. Ann Vasc Surg 2013;27:924-31. [Crossref] [PubMed]
- White RH. Identifying risk factors for venous thromboembolism. Circulation 2012;125:2051-3. [Crossref] [PubMed]
- Henke PK, Wakefield T. Thrombus resolution and vein wall injury: dependence on chemokines and leukocytes. Thromb Res 2009;123 Suppl 4:S72-8. [Crossref] [PubMed]
- Nosaka M, Ishida Y, Kimura A, et al. Time-dependent appearance of intrathrombus neutrophils and macrophages in a stasis-induced deep vein thrombosis model and its application to thrombus age determination. Int J Legal Med 2009;123:235-40. [Crossref] [PubMed]
- Nosaka M, Ishida Y, Kimura A, et al. Time-dependent organic changes of intravenous thrombi in stasis-induced deep vein thrombosis model and its application to thrombus age determination. Forensic Sci Int 2010;195:143-7. [Crossref] [PubMed]
- Evans CE, Humphries J, Saha P, et al. Opinions on mouse models of thrombosis. Thromb Res 2012;130:285-6. [Crossref] [PubMed]
- Modarai B, Burnand KG, Humphries J, et al. The role of neovascularisation in the resolution of venous thrombus. Thromb Haemost 2005;93:801-9. [PubMed]
- Xie H, Kim K, Aglyamov SR, et al. Staging deep venous thrombosis using ultrasound elasticity imaging: animal model. Ultrasound Med Biol 2004;30:1385-96. [Crossref] [PubMed]
- Watson L, Broderick C, Armon MP. Thrombolysis for acute deep vein thrombosis. Cochrane Database Syst Rev 2016;11:CD002783. [PubMed]
- Vedantham S, Goldhaber SZ, Kahn SR, et al. Rationale and design of the ATTRACT Study: a multicenter randomized trial to evaluate pharmacomechanical catheter-directed thrombolysis for the prevention of postthrombotic syndrome in patients with proximal deep vein thrombosis. Am Heart J 2013;165:523-30.e3. [Crossref] [PubMed]
- Gao GY, Yang P, Liu M, et al. Thrombolysis for acute intermediate-risk pulmonary embolism: A meta-analysis. Thromb Res 2015;136:932-7. [Crossref] [PubMed]
- Dong BR, Hao Q, Yue J, et al. Thrombolytic therapy for pulmonary embolism. Cochrane Database Syst Rev 2015.CD004437. [PubMed]
- Rennenberg RJ. Oral anticoagulants, effect on thrombus resolution and post-thrombotic syndrome. Phlebology 2016;31:24-7. [Crossref] [PubMed]
- Kearon C. Natural history of venous thromboembolism. Circulation 2003;107:I22-30. [Crossref] [PubMed]
- Xie H, Kim K, Aglyamov SR, et al. Correspondence of ultrasound elasticity imaging to direct mechanical measurement in aging DVT in rats. Ultrasound Med Biol 2005;31:1351-9. [Crossref] [PubMed]
- Bilbao JI, Martinez-Cuesta A, Urtasun F, et al. Complications of embolization. Semin Intervent Radiol 2006;23:126-42. [Crossref] [PubMed]
- Emelianov SY, Chen X, O'Donnell M, et al. Triplex ultrasound: elasticity imaging to age deep venous thrombosis. Ultrasound Med Biol 2002;28:757-67. [Crossref] [PubMed]
- Fineschi V, Turillazzi E, Neri M, et al. Histological age determination of venous thrombosis: a neglected forensic task in fatal pulmonary thrombo-embolism. Forensic Sci Int 2009;186:22-8. [Crossref] [PubMed]
- Jaff MR, McMurtry MS, Archer SL, et al. Management of massive and submassive pulmonary embolism, iliofemoral deep vein thrombosis, and chronic thromboembolic pulmonary hypertension: a scientific statement from the American Heart Association. Circulation 2011;123:1788-830. [Crossref] [PubMed]
- Resnick SB, Resnick SH, Weintraub JL, et al. Heparin in interventional radiology: a therapy in evolution. Semin Intervent Radiol 2005;22:95-107. [Crossref] [PubMed]
- Akesson H, Brudin L, Dahlstrom JA, et al. Venous function assessed during a 5 year period after acute ilio-femoral venous thrombosis treated with anticoagulation. Eur J Vasc Surg 1990;4:43-8. [Crossref] [PubMed]
- Delis KT, Bountouroglou D, Mansfield AO. Venous claudication in iliofemoral thrombosis: long-term effects on venous hemodynamics, clinical status, and quality of life. Ann Surg 2004;239:118-26. [Crossref] [PubMed]
- Engelberger RP, Stuck A, Spirk D, et al. Ultrasound-assisted versus conventional catheter-directed thrombolysis for acute iliofemoral deep vein thrombosis: 1-year follow-up data of a randomized-controlled trial. J Thromb Haemost 2017;15:1351-60. [Crossref] [PubMed]
- Urokinase pulmonary embolism trial. Phase 1 results: a cooperative study. JAMA 1970;214:2163-72. [Crossref] [PubMed]
- Tibbutt DA, Davies JA, Anderson JA, et al. Comparison by controlled clinical trial of streptokinase and heparin in treatment of life-threatening pulmonay embolism. Br Med J 1974;1:343-7. [Crossref] [PubMed]
- Ly B, Arnesen H, Eie H, et al. A controlled clinical trial of streptokinase and heparin in the treatment of major pulmonary embolism. Acta Med Scand 1978;203:465-70. [Crossref] [PubMed]
- Vedantham S, Piazza G, Sista AK, et al. Guidance for the use of thrombolytic therapy for the treatment of venous thromboembolism. J Thromb Thrombolysis 2016;41:68-80. [Crossref] [PubMed]
- Casey ET, Murad MH, Zumaeta-Garcia M, et al. Treatment of acute iliofemoral deep vein thrombosis. J Vasc Surg 2012;55:1463-73. [Crossref] [PubMed]
- Beythien C, Terres W, Gutensohn K, et al. Thrombus age as a determinant of lysis efficacy of in vitro produced platelet-fibrin thrombi. Z Kardiol 1996;85:661-7. [PubMed]
- Ohman EM, Harrington RA, Cannon CP, et al. Intravenous thrombolysis in acute myocardial infarction. Chest 2001;119:253S-77S. [Crossref] [PubMed]
- Savchenko AS, Martinod K, Seidman MA, et al. Neutrophil extracellular traps form predominantly during the organizing stage of human venous thromboembolism development. J Thromb Haemost 2014;12:860-70. [Crossref] [PubMed]
- Bajd F, Vidmar J, Fabjan A, et al. Impact of altered venous hemodynamic conditions on the formation of platelet layers in thromboemboli. Thromb Res 2012;129:158-63. [Crossref] [PubMed]
- Undas A, Ariens RA. Fibrin clot structure and function: a role in the pathophysiology of arterial and venous thromboembolic diseases. Arterioscler Thromb Vasc Biol 2011;31:e88-99. [Crossref] [PubMed]
- Prandoni P, Lensing AW, Cogo A, et al. The long-term clinical course of acute deep venous thrombosis. Ann Intern Med 1996;125:1-7. [Crossref] [PubMed]
- Henke PK, Varma MR, Deatrick KB, et al. Neutrophils modulate post-thrombotic vein wall remodeling but not thrombus neovascularization. Thromb Haemost 2006;95:272-81. [PubMed]
- Simon DI, Ezratty AM, Francis SA, et al. Fibrin(ogen) is internalized and degraded by activated human monocytoid cells via Mac-1 (CD11b/CD18): a nonplasmin fibrinolytic pathway. Blood 1993;82:2414-22. [PubMed]
- Oklu R, Albadawi H, Watkins MT, et al. Detection of extracellular genomic DNA scaffold in human thrombus: implications for the use of deoxyribonuclease enzymes in thrombolysis. J Vasc Interv Radiol 2012;23:712-8. [Crossref] [PubMed]
- McDonald AP, Meier TR, Hawley AE, et al. Aging is associated with impaired thrombus resolution in a mouse model of stasis induced thrombosis. Thromb Res 2010;125:72-8. [Crossref] [PubMed]
- Varju I, Longstaff C, Szabo L, et al. DNA, histones and neutrophil extracellular traps exert anti-fibrinolytic effects in a plasma environment. Thromb Haemost 2015;113:1289-98. [Crossref] [PubMed]
- Oklu R, Albadawi H, Jones JE, et al. Reduced hind limb ischemia-reperfusion injury in Toll-like receptor-4 mutant mice is associated with decreased neutrophil extracellular traps. J Vasc Surg 2013;58:1627-36. [Crossref] [PubMed]
- Henke PK, Varma MR, Moaveni DK, et al. Fibrotic injury after experimental deep vein thrombosis is determined by the mechanism of thrombogenesis. Thromb Haemost 2007;98:1045-55. [PubMed]
- Brasselet C, Durand E, Addad F, et al. Collagen and elastin cross-linking: a mechanism of constrictive remodeling after arterial injury. Am J Physiol Heart Circ Physiol 2005;289:H2228-33. [Crossref] [PubMed]
- Burke DT. Prevention of deep venous thrombosis: overview of available therapy options for rehabilitation patients. Am J Phys Med Rehabil 2000;79:S3-8. [PubMed]
- East AT, Wakefield TW. What is the optimal duration of treatment for DVT? An update on evidence-based medicine of treatment for DVT. Semin Vasc Surg 2010;23:182-91. [Crossref] [PubMed]
- Hull RD, Liang J, Bergqvist D, et al. Benefit-to-harm ratio of thromboprophylaxis for patients undergoing major orthopaedic surgery. A systematic review. Thromb Haemost 2014;111:199-212. [Crossref] [PubMed]
- Zusman O, Paul M, Farbman L, et al. Venous thromboembolism prophylaxis with anticoagulation in septic patients: a prospective cohort study. QJM 2015;108:197-204. [Crossref] [PubMed]
- Hull RD, Hirsh J, Sackett DL, et al. Cost-effectiveness of primary and secondary prevention of fatal pulmonary embolism in high-risk surgical patients. Can Med Assoc J 1982;127:990-5. [PubMed]
- Nowak M, Krolak-Nowak K, Sobolewska-Wlodarczyk A, et al. Elevated risk of venous thromboembolic events in patients with inflammatory myopathies. Vasc Health Risk Manag 2016;12:233-8. [PubMed]
- Hull RD, Pineo GF. Extended prophylaxis against venous thromboembolism following total hip and knee replacement. Haemostasis 1999;29 Suppl S1:23-31. [PubMed]
- Hull RD, Pineo GF, Stein PD, et al. Extended out-of-hospital low-molecular-weight heparin prophylaxis against deep venous thrombosis in patients after elective hip arthroplasty: a systematic review. Ann Intern Med 2001;135:858-69. [Crossref] [PubMed]
- Gould MK, Dembitzer AD, Sanders GD, et al. Low-molecular-weight heparins compared with unfractionated heparin for treatment of acute deep venous thrombosis. A cost-effectiveness analysis. Ann Intern Med 1999;130:789-99. [Crossref] [PubMed]
- Piazza G, Mani V, Goldhaber SZ, et al. Magnetic resonance venography to assess thrombus resolution with edoxaban monotherapy versus parenteral anticoagulation/warfarin for symptomatic deep vein thrombosis: A multicenter feasibility study. Vasc Med 2016;21:361-8. [Crossref] [PubMed]
- Cohen AT, Hamilton M, Bird A, et al. Comparison of the Non-VKA Oral Anticoagulants Apixaban, Dabigatran, and Rivaroxaban in the Extended Treatment and Prevention of Venous Thromboembolism: Systematic Review and Network Meta-Analysis. PLoS One 2016;11:e0160064. [Crossref] [PubMed]
- Becattini C, Agnelli G. Treatment of Venous Thromboembolism With New Anticoagulant Agents. J Am Coll Cardiol 2016;67:1941-55. [Crossref] [PubMed]
- Bauersachs R. Non-vitamin K antagonist oral anticoagulants for the prevention of recurrent venous thromboembolism. Thromb Res 2016;144:12-20. [Crossref] [PubMed]
- Bleker SM, Brekelmans MP, Eerenberg ES, et al. Clinical impact of major bleeding in patients with venous thromboembolism treated with factor Xa inhibitors or vitamin K antagonists. An individual patient data meta-analysis. Thromb Haemost 2017;117:1944-51. [Crossref] [PubMed]
- Goktay AY, Senturk C. Endovascular Treatment of Thrombosis and Embolism. Adv Exp Med Biol 2017;906:195-213. [Crossref] [PubMed]
- Schrijver AM, Reijnen MM, van Oostayen JA, et al. Dutch randomized trial comparing standard catheter-directed thrombolysis versus ultrasound-accelerated thrombolysis for thromboembolic infrainguinal disease (DUET): design and rationale. Trials 2011;12:20. [Crossref] [PubMed]
- Ganguli S, Kalva S, Oklu R, et al. Efficacy of lower-extremity venous thrombolysis in the setting of congenital absence or atresia of the inferior vena cava. Cardiovasc Intervent Radiol 2012;35:1053-8. [Crossref] [PubMed]
- Wicky S, Pinto EG, Oklu R. Catheter-directed thrombolysis of arterial thrombosis. Semin Thromb Hemost 2013;39:441-5. [Crossref] [PubMed]
- Zhang YS, Davoudi F, Walch P, et al. Bioprinted thrombosis-on-a-chip. Lab Chip 2016;16:4097-105. [Crossref] [PubMed]
- Humphries J, McGuinness CL, Smith A, et al. Monocyte chemotactic protein-1 (MCP-1) accelerates the organization and resolution of venous thrombi. J Vasc Surg 1999;30:894-9. [Crossref] [PubMed]
- McGuinness CL, Humphries J, Waltham M, et al. Recruitment of labelled monocytes by experimental venous thrombi. Thromb Haemost 2001;85:1018-24. [PubMed]
- Rubin JM, Xie H, Kim K, et al. Sonographic elasticity imaging of acute and chronic deep venous thrombosis in humans. J Ultrasound Med 2006;25:1179-86. [Crossref] [PubMed]
- Geier B, Barbera L, Muth-Werthmann D, et al. Ultrasound elastography for the age determination of venous thrombi. Evaluation in an animal model of venous thrombosis. Thromb Haemost 2005;93:368-74. [PubMed]
- Linkins LA, Stretton R, Probyn L, et al. Interobserver agreement on ultrasound measurements of residual vein diameter, thrombus echogenicity and Doppler venous flow in patients with previous venous thrombosis. Thromb Res 2006;117:241-7. [Crossref] [PubMed]
- Brighton T, Janssen J, Butler SP. Aging of acute deep vein thrombosis measured by radiolabeled 99mTc-rt-PA. J Nucl Med 2007;48:873-8. [Crossref] [PubMed]
- Schaefer JK, Jacobs B, Wakefield TW, et al. New biomarkers and imaging approaches for the diagnosis of deep venous thrombosis. Curr Opin Hematol 2017;24:274-81. [Crossref] [PubMed]
- Schmitt C, Montagnon E, Henni AH, et al. Shear wave induced resonance elastography of venous thrombi: a proof-of-concept. IEEE Trans Med Imaging 2013;32:565-77. [Crossref] [PubMed]
- Bernal M, Gennisson JL, Flaud P, et al. Shear wave elastography quantification of blood elasticity during clotting. Ultrasound Med Biol 2012;38:2218-28. [Crossref] [PubMed]