Hereditary hemorrhagic telangiectasia and pulmonary arteriovenous malformations: clinical aspects
Introduction
Hereditary hemorrhagic telangiectasia (HHT) is an inherited disorder of blood vessel formation characterized by mucocutaneous and visceral vascular malformations resulting in direct communication between arterioles and venules without intervening capillary beds (1). These malformations lead to variable clinical manifestations that depend on where they occur and to what extent. Certain tissues are affected more often than others, but virtually any tissue can be involved. Recurrent epistaxis from nasal telangiectasias is present in nearly all patients by the age of 40 (2,3). Gastrointestinal telangiectasias and hepatic and cerebral vascular malformations are seen with varying frequency. The diagnosis of HHT is based on clinical criteria (4) whereby the presence of at least three of the following four criteria establishes a definite diagnosis of HHT: recurrent epistaxis, multiple characteristic telangiectasias, visceral vascular malformations, and a first-degree relative meeting diagnostic criteria for HHT (Table 1). Pulmonary arteriovenous malformations (PAVMs) are the most common pulmonary manifestation of HHT and only rarely occur sporadically in patients without HHT. It is essential, therefore, that physicians who provide care to patients with PAVMs be aware of the clinical manifestations of HHT in general and of HHT-related PAVMs in particular.
Pathogenesis
HHT is inherited in an autosomal dominant pattern, affecting more than 1 in 10,000 people worldwide (5), and results from mutations in genes that encode proteins critical to transforming growth factor beta (TGF-β) signaling. Most cases involve mutations in two such genes, endoglin and ACVRL1. Pathogenic mutations in endoglin, which encodes a type III TGF-β receptor, are associated with early HHT onset and a tendency for lung and brain AVMs (6-8). ACVRL1 encodes the type I TGF-β receptor activin-like kinase 1 (ALK1) (6,8,9). Pathogenic ACVRL1 mutations are associated with a higher prevalence of hepatic vascular malformations and a lower prevalence of pulmonary and brain AVMs compared to patients with endoglin mutations. Mutations in other TGF-β genes have been identified in a minority of cases (10,11) (Table 2).
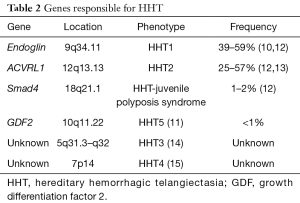
Full table
The mechanisms by which defects in TGF-β signaling lead to the vascular malformations of HHT remain incompletely understood. ALK1 and endoglin are highly expressed in vascular endothelial cells and play essential roles in angiogenesis and vascular stability (16). Reduced expression of endoglin or ALK1 is probably not sufficient by itself to result in defective angiogenesis since most vascular beds develop normally in patients with HHT. Instead, a two-hit hypothesis has been proposed postulating that a trigger for abnormal blood vessel formation must exist on top of the underlying genetic defect. Suggested triggers include context-specific loss of heterozygosity and reduced endoglin expression in areas of inflammation or hypoxia (17). Regardless of the specific trigger, in the proper genetic and environmental context, disordered angiogenesis leads to the vascular malformations observed in patients with HHT, including those in the lung. This process is thought to occur in a stepwise fashion starting with dilation of post-capillary venules followed by arteriolar dilation and then loss of the intervening capillary bed (18).
Clinical manifestations
PAVMs are found in up to half of patients with HHT (19,20) and can lead to fatal embolic and hemorrhagic complications. PAVMs occur in three general forms. Most PAVMs in HHT are morphologically simple, consisting of a feeding vessel that originates from a single segmental pulmonary artery and connects to a draining pulmonary vein via a single aneurysmal nidus (21,22). Complex PAVMs are fed by more than one segmental pulmonary artery and may have multiple draining veins (23). Less than 10% of PAVMs are diffuse, defined as diffusely involving at least one lung segment (24), and have been associated with higher rates of hypoxemia and embolic complications (25,26).
Blood that transits a PAVM does not participate in alveolar gas exchange, reducing the oxygen content of the blood returning to the left heart. Importantly, that blood will also not undergo physical filtration, a critical function of the pulmonary circulation by which the pulmonary capillary network prevents passage of thrombi and other blood-borne material into systemic circulation (27). The pulmonary capillary circulation may also play a role in innate immunity by removing circulating bacteria that enter the bloodstream. Recent evidence suggests that bacteria sequestered in pulmonary capillaries are efficiently removed by marginated neutrophils (28) and that bypassing this function might increase the risk of septic complications. Whether this mechanism contributes to the relatively high incidence of serious infections in patients with HHT (29) has not been established.
Symptoms
Most HHT-related PAVMs are asymptomatic. Dyspnea is the most commonly reported symptom but can be difficult to differentiate from that caused by other features of HHT such as iron deficiency anemia, high output heart failure in patients with extensive intrahepatic shunting, venous thromboembolism (VTE), or rare instances of HHT-associated heritable pulmonary arterial hypertension. The reported prevalence of hypoxemia varies. Older reports suggest most HHT patients with PAVMs will have a reduced PaO2 (30) while more recent series that include patients with smaller PAVMs place the prevalence considerably lower (31). Cyanosis and clubbing are likewise less common than suggested by early reports. Orthodeoxia may be present, but platypnea is rare. In a series of 257 patients with PAVMs, of the 75 that exhibited orthodeoxia none reported platypnea (32).
Paradoxical septic emboli
HHT patients with PAVMs are several hundred-fold more likely to develop brain abscess compared to the general population (33), and the associated morbidity is significant. In one cohort, 80% of those that developed brain abscess were unable to return to work due to persistent neurologic deficits (34). Male gender, presence of deep vein thrombosis, and low resting oxygen saturation were associated with increased abscess risk. Preceding dental procedure was the most commonly identified risk factor. Poor dental hygiene also increases risk, and in some cases a precipitating event cannot be identified. Encephalomalacia from prior embolic infarcts may also increase risk as the damaged cortical tissue can serve as a nidus for bacterial growth and abscess development (35). When culture data are available, periodontal microbes are responsible for most brain abscesses. In the same cohort study described above, 28 patients developed brain abscess with most cultures failing to grow any organism. Cultured organisms included those commonly found in endo- and periodontal infections, and half of the positive cultures were polymicrobial (34).
Stroke
Patients with HHT are at increased risk of cerebral ischemic events attributable to paradoxical embolization of thrombus through PAVMs. Reported rates vary depending on the study population and how the event is defined. In a cohort referred for PAVM embolotherapy, nearly 40% reported a prior transient ischemic attack and 18% had experienced a clinical stroke (36). The rate is lower when patients with smaller PAVMs are included (26,27,34). Conventional stroke risk factors are often not present. Instead, higher grade intra-pulmonary shunting on contrast echocardiography (37) and low serum iron levels have been linked to an increased stroke risk in this population (27). Low serum iron status has also been associated with increased incidence of VTE, which occurs at a rate at least two-fold higher in patients with HHT than in a general hospitalized population. Indeed, low serum iron was found to be the most significant predictor of VTE risk among patients with HHT even after adjustment for age, inflammation, and von Willebrand factor (38), suggesting that iron deficiency anemia may be a modifiable risk factor for both VTE and stroke in patients with HHT. Consensus guidelines call for annual evaluation of hemoglobin and/or hematocrit for patients with HHT older than 35 and closely monitoring trends in iron status irrespective of hemoglobin level (1). This approach is critical as hypoxemia secondary to shunting through PAVMs may lead to a hemoglobin that is higher than would otherwise be expected, potentially masking clinically important iron deficiency (31).
Hemorrhage
Spontaneous rupture of PAVMs can result in massive hemoptysis or hemothorax and has been estimated to affect between 1% and 8% (39-41) of HHT patients with PAVMs. Pregnant patients are particularly vulnerable to PAVM rupture due to physiologic changes resulting in increased flow through and enlargement of existing AVMs. Three of the seven women who experienced massive hemoptysis in one study were pregnant (39). In specific cohorts of pregnant women with HHT, between 1% and 2% experienced PAVM rupture, and the risk of rupture may remain elevated for some time after delivery (42,43). Clinical presentation depends on the site of rupture and extent of hemorrhage. Symptoms of spontaneous hemothorax can include pleuritic chest pain, dyspnea, hypoxemia, and hemodynamic compromise, if sufficient bleeding occurs. Intra-pulmonary hemorrhage may be heralded by minor hemoptysis prior to the onset of massive, life-threatening hemorrhage, potentially allowing an opportunity for therapeutic intervention (42). Not all hemoptysis in HHT is indicative of PAVM rupture (44), but hemoptysis in a patient with PAVMs, especially during pregnancy, should be evaluated promptly to exclude PAVM rupture prior to the onset of massive hemorrhage.
Screening for PAVMs
Effective screening is necessary to identify which HHT patients have PAVMs so that their dire clinical consequences can be avoided. Transthoracic contrast echocardiography (TTCE) is the preferred method for PAVM screening because of its high sensitivity and excellent negative predictive value (45-47). The absence of shunting on TTCE excludes a treatable PAVM on CT and requires no confirmatory imaging. Positive predictive value varies with the extent of right-to-left shunting. Quantitative and qualitative schemes have been used to grade right-to-left shunting, but regardless of the specific method, increasing shunt grades are associated with higher probabilities of confirming the presence of PAVMs on CT and of detecting treatment-amenable PAVMs (48-52) (Table 3). Shunt grade has also been shown to predict the prevalence of cerebral embolic manifestations including stroke, transient ischemic attack, or brain abscess (37). As with any assessment of shunting alone, TTCE cannot establish that the shunt is intrapulmonary and cannot define PAVM location or anatomy. A delay of 3–4 or more cardiac cycles and the appearance of bubbles in the pulmonary vein prior to entering the left ventricle are indicative of extra-cardiac shunting; however, shunting that arises between 1 and 3 cardiac cycles does not exclude an extra-cardiac shunt since other factors can influence shunt timing (48).
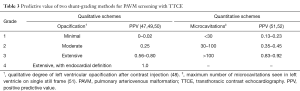
Full table
Although reports of adverse events from agitated saline echocardiography are reported too infrequently to accurately describe their incidence, a large survey of neurologists by the American Society of Echocardiography yielded an estimate of 0.062%, none of which resulted in durable effects (53). The side effects seen were all deemed consistent with gas embolization and were associated with excellent image quality, suggestive of a dose-response relationship. A more recent case series identified 5 adverse events out of 3,314 TTCE and transcranial Doppler studies (54). Mechanisms proposed were speculative but included introduction of large bubbles by insufficiently vertical syringe angle, large needle/catheter size, operator indiscretion, or migrainous vasospasm triggered by agitated saline. Precautions should be taken to minimize these risks, most importantly by avoiding the injection of a visible quantity of air, especially in patients with known shunt.
Alternate methods to assess right-to-left shunting are used less often for PAVM screening. An elevated shunt fraction is present in essentially all patients with PAVMs, and can be calculated after breathing 100% oxygen for 15–20 minutes. The most precise measurement of shunt fraction requires invasive sampling of mixed venous blood and is not routinely done. Instead, shunt fraction can be estimated from the arterial PaO2 (55). In a patient with otherwise normal gas exchange, a shunt fraction of greater than 5% is indicative of right to left shunting. Radionuclide perfusion lung scanning is another method to determine shunt fraction and may have utility when contrast echocardiography or 100% oxygen method cannot be obtained (55). In this technique, radiolabeled macro aggregated albumin microspheres 7–25 µm in size are injected intravenously (56). In individuals without a right to left shunt, the material is filtered as particles >8 µm are normally unable to pass through the pulmonary capillary bed. The shunt fraction is deduced from quantification of uptake, typically in the right kidney to minimize effect of overlying splenic activity, relative to the total dose of radiolabeled substrate administered. Overall, perfusion scanning is marginally less invasive, quicker, and more comfortable than the 100% oxygen method (57). Disadvantages include high cost and inability to distinguish intrapulmonary from intra-cardiac shunting.
PAVM screening is recommended for all patients at the initial HHT evaluation. If negative, repeat screening is recommended after puberty, within 5 years of planned pregnancy, after pregnancy, and otherwise every 5 to 10 years (1). The issue of whether and when ongoing screening can safely be discontinued after repeatedly negative results has not been settled, but it is unlikely that new PAVMs will arise after middle age. Chest imaging to confirm PAVM and to assess treatment feasibility is recommended after positive screening. CT can reliably diagnose PAVMs using established criteria and has replaced angiography for that purpose (58). Contrast is not required but can assist in better defining AVM architecture, precisely defining the origin of the feeding artery, and assessing whether an AVM remains perfused. Once diagnosed, long-term follow-up is essential since small, untreated PAVMs can grow, and previously treated PAVMs can reperfuse. Current recommendations advise CT at 1 to 5 year intervals to assess for interval growth. Relatively slow growth rates of most PAVMs and concern about cumulative radiation exposure have led many to favor a 5-year interval (59). Some degree of right-to-left shunting will usually persist after PAVM closure (60), limiting the role TTCE in long term follow up of treated PAVMs. Instead, CT chest is advised within 6–12 months of treatment and then every 5 years if successful closure is confirmed (Figure 1).
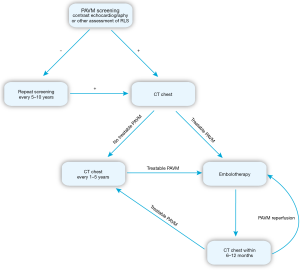
Treatment
The goals of PAVM management are to minimize embolic complications, prevent and treat hemorrhage from PAVM rupture, and improve functional capacity by reducing shunt-related hypoxemia. These objectives are accomplished through physical disruption of PAVMs when feasible, in conjunction with medical strategies to mitigate PAVM risk and treat complications.
Oxygen
Hypoxemia is often well tolerated in HHT patients with PAVMs. Compensatory erythrocytosis and increased cardiac output can maintain normal oxygen delivery to tissues as long as there are sufficient iron stores to allow hemoglobin synthesis (31). It is important, therefore, that iron deficiency be corrected and that hemorrhagic complications of HHT be addressed to limit further blood loss. In symptomatic patients, supplemental oxygen can reduce dyspnea and improve functional capacity but is not indicated to prevent pulmonary hypertension since PAVM-associated hypoxemia is not accompanied by alveolar hypoxia (25,31). Shunting through PAVMs can lead to pronounced hypoxemia in some cases (25), but patients often remain asymptomatic and highly functional for years. In a cohort of 36 patients with diffuse PAVMs, none of the 9 deaths over the follow up period were directly related to hypoxemia or its complications, and oxygen levels were not significantly lower among those who died (24). Formatting comment: alter line spacing to indicate end of paragraph.
Therapeutic embolization
Disruption of flow through PAVMs via transcatheter embolization has been the standard approach to management since the 1980s (22,23,36). Surgical resection of PAVMs, at one time the only definitive treatment option, is now rarely performed except when urgent treatment is required in settings where expertise in embolotherapy is lacking. Until recently, PAVMs with feeding vessels ≤3 mm in diameter were considered low risk, but emerging evidence suggests that paradoxical embolic complications can arise from PAVMs fed by smaller vessels (25,61) and embolic risk may not be directly related to feeding vessel diameter (34). For that reason, treatment decisions are increasingly being made on the basis of technical feasibility and safety considerations rather than feeding artery diameter. The benefits of embolotherapy in reducing PAVM-related complications are supported by the results of observational studies showing that successful PAVM closure reduces shunt fraction (62), improves oxygenation (30), and reduces the rate of brain abscess and stroke (27). Sustained PAVM closure can be achieved in most cases (63). Failures result most often from recanalization of the treated artery but can also occur by collateral perfusion from an adjacent PA branch, perfusion by untreated feeding vessels in complex PAVMs, and by systemic perfusion (30,63-66).
Prophylaxis
Steps to reduce embolic complications are recommended for all patients with PAVMs and for patients with confirmed or suspected HHT who have not yet been screened for PAVMs. To prevent septic embolic complications, antibiotics are recommended before procedures that may result in bacteremia (67). Choice of antibiotic follows American Heart Association guidelines for the prevention of infective endocarditis (68). To prevent embolization of air, in-line filters can be used during intravenous infusions should standard precautions fail. Avoidance of SCUBA diving has also been recommended over concern that gas embolism associated with rapid decompression may be particularly deleterious to patients with PAVMs (1).
Conclusions
PAVMs are a common feature of HHT and are associated with a range of potential clinical manifestations, from symptomatic hypoxemia to life-threatening septic embolic events, paradoxical thromboembolism, and hemorrhage from PAVM rupture. The management of HHT patients with PAVMs requires a comprehensive approach that addresses the PAVM directly and HHT generally. Contrast echocardiography is the preferred screening modality, followed by chest CT if shunting is detected. Specific management of PAVMs involves a combination of interventional, medical, and prophylactic modalities. Transcatheter embolization should be considered for amenable PAVMs. Long term PAVM monitoring and prophylactic measures are advocated to further reduce risk. Importantly, other HHT disease manifestations such as VTE and iron deficiency from recurrent blood loss may influence the rate and severity of PAVM-related complications and should be identified and treated, if present. Together, these strategies have the potential to prevent life-altering and fatal complications associated with PAVMs in patients with HHT.
Acknowledgements
None.
Footnote
Conflicts of Interest: The authors have no conflicts of interest to declare.
References
- Faughnan ME, Palda VA, Garcia-Tsao G, et al. International guidelines for the diagnosis and management of hereditary haemorrhagic telangiectasia. J Med Genet 2011;48:73-87. [Crossref] [PubMed]
- Shah RK, Dhingra JK, Shapshay SM. Hereditary hemorrhagic telangiectasia: a review of 76 cases. Laryngoscope 2002;112:767-73. [Crossref] [PubMed]
- Berg J, Porteous M, Reinhardt D, et al. Hereditary haemorrhagic telangiectasia: a questionnaire based study to delineate the different phenotypes caused by endoglin and ALK1 mutations. J Med Genet 2003;40:585-90. [Crossref] [PubMed]
- Shovlin CL, Guttmacher AE, Buscarini E, et al. Diagnostic criteria for hereditary hemorrhagic telangiectasia (Rendu-Osler-Weber syndrome). Am J Med Genet 2000;91:66-7. [Crossref] [PubMed]
- Marchuk DA, Guttmacher AE, Penner JA, Ganguly P. Report on the workshop on Hereditary Hemorrhagic Telangiectasia, July 10-11, 1997. Am J Med Genet 1998;76:269-73. [Crossref] [PubMed]
- McAllister KA, Grogg KM, Johnson DW, et al. Endoglin, a TGF-beta binding protein of endothelial cells, is the gene for hereditary haemorrhagic telangiectasia type 1. Nat Genet 1994;8:345-51. [Crossref] [PubMed]
- Letteboer TG, Mager JJ, Snijder RJ, et al. Genotype-phenotype relationship in hereditary haemorrhagic telangiectasia. J Med Genet 2006;43:371-7. [Crossref] [PubMed]
- Bayrak-Toydemir P, McDonald J, Markewitz B, et al. Genotype-phenotype correlation in hereditary hemorrhagic telangiectasia: mutations and manifestations. Am J Med Genet A 2006;140:463-70. [Crossref] [PubMed]
- Johnson DW, Berg JN, Baldwin MA, et al. Mutations in the activin receptor-like kinase 1 gene in hereditary haemorrhagic telangiectasia type 2. Nature genetics. 1996;13:189-95. [Crossref] [PubMed]
- Prigoda NL, Savas S, Abdalla SA, et al. Hereditary haemorrhagic telangiectasia: mutation detection, test sensitivity and novel mutations. J Med Genet 2006;43:722-8. [Crossref] [PubMed]
- Wooderchak-Donahue WL, McDonald J, O'Fallon B, et al. BMP9 mutations cause a vascular-anomaly syndrome with phenotypic overlap with hereditary hemorrhagic telangiectasia. Am J Hum Genet 2013;93:530-7. [Crossref] [PubMed]
- McDonald J, Pyeritz RE. Hereditary Hemorrhagic Telangiectasia. In: Adam MP, Ardinger HH, Pagon RA, et al. editors. GeneReviews(R). Seattle: University of Washington, 1993.
- Olivieri C, Pagella F, Semino L, et al. Analysis of ENG and ACVRL1 genes in 137 HHT Italian families identifies 76 different mutations (24 novel). Comparison with other European studies. J Hum Genet 2007;52:820-9. [Crossref] [PubMed]
- Cole SG, Begbie ME, Wallace GM, Shovlin CL. A new locus for hereditary haemorrhagic telangiectasia (HHT3) maps to chromosome 5. J Med Genet 2005;42:577-82. [Crossref] [PubMed]
- Bayrak-Toydemir P, McDonald J, Akarsu N, et al. A fourth locus for hereditary hemorrhagic telangiectasia maps to chromosome 7. Am J Med Genet A 2006;140:2155-62. [Crossref] [PubMed]
- Mahmoud M, Allinson KR, Zhai Z, et al. Pathogenesis of arteriovenous malformations in the absence of endoglin. Circ Res 2010;106:1425-33. [Crossref] [PubMed]
- Marchuk DA, Srinivasan S, Squire TL, Zawistowski JS. Vascular morphogenesis: tales of two syndromes. Hum Mol Genet 2003;12:R97-112. [Crossref] [PubMed]
- Braverman IM, Keh A, Jacobson BS. Ultrastructure and three-dimensional organization of the telangiectases of hereditary hemorrhagic telangiectasia. J Invest Dermatol 1990;95:422-7. [Crossref] [PubMed]
- van Gent MW, Post MC, Snijder RJ, et al. Real prevalence of pulmonary right-to-left shunt according to genotype in patients with hereditary hemorrhagic telangiectasia: a transthoracic contrast echocardiography study. Chest 2010;138:833-9. [Crossref] [PubMed]
- Cottin V, Plauchu H, Bayle JY, et al. Pulmonary arteriovenous malformations in patients with hereditary hemorrhagic telangiectasia. Am J Respir Crit Care Med 2004;169:994-1000. [Crossref] [PubMed]
- White RI Jr, Mitchell SE, Barth KH, et al. Angioarchitecture of pulmonary arteriovenous malformations: an important consideration before embolotherapy. AJR Am J Roentgenol 1983;140:681-6. [Crossref] [PubMed]
- White RI Jr, Pollak JS, Wirth JA. Pulmonary arteriovenous malformations: diagnosis and transcatheter embolotherapy. J Vasc Interv Radiol 1996;7:787-804. [Crossref] [PubMed]
- Sharma P, Kochar P, Sharma S, et al. A case of pulmonary arteriovenous malformation: role of interventional radiology in diagnosis and treatment. Ann Transl Med 2017;5:345. [Crossref] [PubMed]
- Pierucci P, Murphy J, Henderson KJ, et al. New definition and natural history of patients with diffuse pulmonary arteriovenous malformations: twenty-seven-year experience. Chest 2008;133:653-61. [Crossref] [PubMed]
- Faughnan ME, Lui YW, Wirth JA, et al. Diffuse pulmonary arteriovenous malformations: characteristics and prognosis. Chest 2000;117:31-8. [Crossref] [PubMed]
- Moussouttas M, Fayad P, Rosenblatt M, et al. Pulmonary arteriovenous malformations: cerebral ischemia and neurologic manifestations. Neurology 2000;55:959-64. [Crossref] [PubMed]
- Shovlin CL, Chamali B, Santhirapala V, et al. Ischaemic strokes in patients with pulmonary arteriovenous malformations and hereditary hemorrhagic telangiectasia: associations with iron deficiency and platelets. PLoS One 2014;9. [Crossref] [PubMed]
- Yipp BG, Kim JH, Lima R, et al. The Lung is a Host Defense Niche for Immediate Neutrophil-Mediated Vascular Protection. Sci Immunol 2017;2. [Crossref] [PubMed]
- Dupuis-Girod S, Giraud S, Decullier E, et al. Hemorrhagic hereditary telangiectasia (Rendu-Osler disease) and infectious diseases: an underestimated association. Clin Infect Dis 2007;44:841-5. [Crossref] [PubMed]
- Lee DW, White RI Jr, Egglin TK, et al. Embolotherapy of large pulmonary arteriovenous malformations: long-term results. Ann Thorac Surg 1997;64:930-9; discussion 939-40. [Crossref] [PubMed]
- Santhirapala V, Williams LC, Tighe HC, et al. Arterial oxygen content is precisely maintained by graded erythrocytotic responses in settings of high/normal serum iron levels, and predicts exercise capacity: an observational study of hypoxaemic patients with pulmonary arteriovenous malformations. PLoS One 2014;9. [Crossref] [PubMed]
- Santhirapala V, Chamali B, McKernan H, et al. Orthodeoxia and postural orthostatic tachycardia in patients with pulmonary arteriovenous malformations: a prospective 8-year series. Thorax 2014;69:1046-7. [Crossref] [PubMed]
- Kjeldsen AD, Torring PM, Nissen H, et al. Cerebral abscesses among Danish patients with hereditary haemorrhagic telangiectasia. Acta Neurol Scand 2014;129:192-7. [Crossref] [PubMed]
- Shovlin CL, Jackson JE, Bamford KB, et al. Primary determinants of ischaemic stroke/brain abscess risks are independent of severity of pulmonary arteriovenous malformations in hereditary haemorrhagic telangiectasia. Thorax 2008;63:259-66. [Crossref] [PubMed]
- Takeshita M, Kagawa M, Yonetani H, et al. Risk factors for brain abscess in patients with congenital cyanotic heart disease. Neurol Med Chir (Tokyo) 1992;32:667-70. [Crossref] [PubMed]
- White RI Jr, Lynch-Nyhan A, Terry P, et al. Pulmonary arteriovenous malformations: techniques and long-term outcome of embolotherapy. Radiology 1988;169:663-9. [Crossref] [PubMed]
- Velthuis S, Buscarini E, van Gent MWF, et al. Grade of pulmonary right-to-left shunt on contrast echocardiography and cerebral complications: a striking association. Chest 2013;144:542-8. [Crossref] [PubMed]
- Livesey JA, Manning RA, Meek JH, et al. Low serum iron levels are associated with elevated plasma levels of coagulation factor VIII and pulmonary emboli/deep venous thromboses in replicate cohorts of patients with hereditary haemorrhagic telangiectasia. Thorax 2012;67:328-33. [Crossref] [PubMed]
- Ference BA, Shannon TM, White RI Jr, et al. Life-threatening pulmonary hemorrhage with pulmonary arteriovenous malformations and hereditary hemorrhagic telangiectasia. Chest 1994;106:1387-90. [Crossref] [PubMed]
- Berg AM, Amirbekian S, Mojibian H, et al. Hemothorax due to rupture of pulmonary arteriovenous malformation: an interventional emergency. Chest 2010;137:705-7. [Crossref] [PubMed]
- El Hajjam M, Lacout A, Marcy PY, et al. Rupture of a pulmonary arteriovenous malformation: a diagnosis not to be missed. Postgrad Med J 2017;93:570-1. [Crossref] [PubMed]
- Shovlin CL, Sodhi V, McCarthy A, et al. Estimates of maternal risks of pregnancy for women with hereditary haemorrhagic telangiectasia (Osler-Weber-Rendu syndrome): suggested approach for obstetric services. BJOG 2008;115:1108-15. [Crossref] [PubMed]
- de Gussem EM, Lausman AY, Beder AJ, et al. Outcomes of pregnancy in women with hereditary hemorrhagic telangiectasia. Obstet Gynecol 2014;123:514-20. [Crossref] [PubMed]
- Lincoln MJ, Shigeoka JW. Pulmonary telangiectasia without hypoxemia. Chest 1988;93:1097-8. [Crossref] [PubMed]
- Nanthakumar K, Graham AT, Robinson TI, et al. Contrast echocardiography for detection of pulmonary arteriovenous malformations. Am Heart J 2001;141:243-6. [Crossref] [PubMed]
- Oxhøj H, Kjeldsen AD, Nielsen G. Screening for pulmonary arteriovenous malformations: contrast echocardiography versus pulse oximetry. Scand Cardiovasc J 2000;34:281-5. [Crossref] [PubMed]
- Gazzaniga P, Buscarini E, Leandro G, et al. Contrast echocardiography for pulmonary arteriovenous malformations screening: does any bubble matter? Eur J Echocardiogr 2009;10:513-8. [Crossref] [PubMed]
- Barzilai B, Waggoner AD, Spessert C, et al. Two-dimensional contrast echocardiography in the detection and follow-up of congenital pulmonary arteriovenous malformations. Am J Cardiol 1991;68:1507-10. [Crossref] [PubMed]
- Zukotynski K, Chan RP, Chow CM, et al. Contrast echocardiography grading predicts pulmonary arteriovenous malformations on CT. Chest 2007;132:18-23. [Crossref] [PubMed]
- Parra JA, Bueno J, Zarauza J, et al. Graded contrast echocardiography in pulmonary arteriovenous malformations. Eur Respir J 2010;35:1279-85. [Crossref] [PubMed]
- van Gent MW, Post MC, Snijder RJ, et al. Grading of pulmonary right-to-left shunt with transthoracic contrast echocardiography: does it predict the indication for embolotherapy? Chest 2009;135:1288-92. [Crossref] [PubMed]
- Velthuis S, Buscarini E, Mager JJ, et al. Predicting the size of pulmonary arteriovenous malformations on chest computed tomography: a role for transthoracic contrast echocardiography. Eur Respir J 2014;44:150-9. [Crossref] [PubMed]
- Bhatia VK, Senior R. Contrast echocardiography: evidence for clinical use. J Am Soc Echocardiogr 2008;21:409-16. [Crossref] [PubMed]
- Romero JR, Frey JL, Schwamm LH, et al. Cerebral ischemic events associated with 'bubble study' for identification of right to left shunts. Stroke 2009;40:2343-8. [Crossref] [PubMed]
- Gossage JR, Kanj G. Pulmonary arteriovenous malformations. A state of the art review. Am J Respir Crit Care Med 1998;158:643-61. [Crossref] [PubMed]
- Mager JJ, Zanen P, Verzijlbergen F, et al. Quantification of right-to-left shunt with (99m)Tc-labelled albumin macroaggregates and 100% oxygen in patients with hereditary haemorrhagic telangiectasia. Clin Sci (Lond) 2002;102:127-34. [Crossref] [PubMed]
- Whyte MK, Peters AM, Hughes JM, et al. Quantification of right to left shunt at rest and during exercise in patients with pulmonary arteriovenous malformations. Thorax 1992;47:790-6. [Crossref] [PubMed]
- Remy J, Remy-Jardin M, Giraud F, et al. Angioarchitecture of pulmonary arteriovenous malformations: clinical utility of three-dimensional helical CT. Radiology 1994;191:657-64. [Crossref] [PubMed]
- Hanneman K, Faughnan ME, Prabhudesai V. Cumulative radiation dose in patients with hereditary hemorrhagic telangiectasia and pulmonary arteriovenous malformations. Can Assoc Radiol J 2014;65:135-40. [Crossref] [PubMed]
- Lee WL, Graham AF, Pugash RA, et al. Contrast echocardiography remains positive after treatment of pulmonary arteriovenous malformations. Chest 2003;123:351-8. [Crossref] [PubMed]
- Boother EJ, Brownlow S, Tighe HC, et al. Cerebral abscess associated with odontogenic bacteremias, hypoxemia, and iron loading in immunocompetent patients with right-to-left shunting through pulmonary arteriovenous malformations. Clin Infect Dis 2017;65:595-603. [Crossref] [PubMed]
- Dutton JA, Jackson JE, Hughes JM, et al. Pulmonary arteriovenous malformations: results of treatment with coil embolization in 53 patients. AJR Am J Roentgenol 1995;165:1119-25. [Crossref] [PubMed]
- Remy-Jardin M, Dumont P, Brillet PY, et al. Pulmonary arteriovenous malformations treated with embolotherapy: helical CT evaluation of long-term effectiveness after 2-21-year follow-up. Radiology 2006;239:576-85. [Crossref] [PubMed]
- Woodward CS, Pyeritz RE, Chittams JL, et al. Treated pulmonary arteriovenous malformations: patterns of persistence and associated retreatment success. Radiology 2013;269:919-26. [Crossref] [PubMed]
- Milic A, Chan RP, Cohen JH, et al. Reperfusion of pulmonary arteriovenous malformations after embolotherapy. J Vasc Interv Radiol 2005;16:1675-83. [Crossref] [PubMed]
- Pollak JS, Saluja S, Thabet A, et al. Clinical and anatomic outcomes after embolotherapy of pulmonary arteriovenous malformations. J Vasc Interv Radiol 2006;17:35-44. [Crossref] [PubMed]
- Shovlin C, Bamford K, Wray D. Post-NICE 2008: Antibiotic prophylaxis prior to dental procedures for patients with pulmonary arteriovenous malformations (PAVMs) and hereditary haemorrhagic telangiectasia. Br Dent J 2008;205:531-3. [Crossref] [PubMed]
- Wilson W, Taubert KA, Gewitz M, et al. Prevention of infective endocarditis: guidelines from the American Heart Association: a guideline from the American Heart Association Rheumatic Fever, Endocarditis, and Kawasaki Disease Committee, Council on Cardiovascular Disease in the Young, and the Council on Clinical Cardiology, Council on Cardiovascular Surgery and Anesthesia, and the Quality of Care and Outcomes Research Interdisciplinary Working Group. Circulation 2007;116:1736-54. [Crossref] [PubMed]