Repair of abdominal aortic aneurysms: preoperative imaging and evaluation
Introduction
Endovascular aneurysm repair (EVAR) has become an increasingly prevalent treatment option for patients with abdominal aortic aneurysms (AAAs) (1). Relative to open surgical repair, EVAR relies heavily on imaging for proper assessment of procedural candidacy, relevant vascular anatomy, device selection, and surgical approach (2). EVAR success and outcomes are dependent on both clinical factors such as medical comorbidities and technical factors such as anatomy and morphology (3). Careful patient selection, based in part on pre-procedural imaging, is required. In this review, we highlight key anatomic, morphologic factors evaluated as part of the pre-procedural imaging of EVAR and summarize the extant imaging tools to facilitate procedure planning.
Clinical indications and contraindications
The goal of EVAR is to provide a less invasive alternative to open surgical repair of AAAs, as it carries significantly lower periprocedural morbidity and mortality up to 2 years after repair (4,5). If strict criteria are applied, roughly only 60% of patients are candidates for conventional EVAR, mainly due to anatomic limitations; thus proper patient selection is critical to the success of EVAR (6). Clinical indications for repair are similar to those of open surgical repair and include aneurysm diameter ≥5.5 cm or 2.5 times the normal aortic diameter, rapid growth over 10 mm per year and any size symptomatic AAA, variables which incur increased risk for aneurysm rupture (4,7,8). The role of EVAR in high-risk patients with other comorbidities such as advanced age, cardiac disease, pulmonary disease, and renal insufficiency that preclude them from open surgical repair is expanding (9,10).
Contraindications to EVAR overlap with those of open surgical repair and include high-risk comorbidities that increase perioperative morbidity and mortality, such as uncorrectable coagulopathy or high risk cardiac disease (9). Other major limitations specific to EVAR include severe contrast allergy and anatomic factors that provide unfavorable morphology for endograft delivery and placement. Severe renal insufficiency has also been considered a relative contraindication to EVAR, although alternative methods using minimal amount of iodinated contrast or contrast alternatives such as carbon dioxide (CO2) angiography and use of intravascular ultrasound (IVUS) have been successfully applied in order to reduce contrast load (11,12). Likewise, the complications of renal dysfunction following EVAR are important to consider prior to an EVAR procedure. Renal function outcomes have been attributed to several risk factors, both modifiable and non-modifiable, which include contrast usage, length of procedure, age, microemboli, baseline renal dysfunction and ace-inhibitor use (13,14). However the effects of proximal fixation and seal, and suprarenal fixation stents versus infrarenal fixations stents, on renal function are unclear (14,15). Understanding, preventing, and reducing the individual patient’s modifiable and non-modifiable risk factors allow for the best renal outcomes following EVAR. Unsuitable vascular anatomy with compromised landing zones may preclude EVAR as discussed in detail below. In brief, such limitations would include unfavorably short, wide, and excessively angulated proximal AAA neck, extensive circumferential thrombus, and calcifications that directly inhibit an adequate endograft seal. In addition, inadequate iliac-femoral artery access site that would preclude device delivery can be considered a contraindication. The introduction of complex EVAR repairs with fenestrated or branched devices has decreased the landing zone limitations, while at the same time has increased the complexity of repair (16). Pre-procedural imaging has been instrumental in successful planning of endovascular aortic aneurysm repairs, in both conventional and complex EVAR (16-18).
Clinical indications and contraindications
Factors to consider for patient selection based on pre-procedural imaging include aneurysm morphology, access vessel size, patency, and relevant visceral vessels. These properties in turn translate to appropriate and successful stent delivery, deployment, and fixation. AAAs can be classified according to their relation to the renal arteries (infrarenal, juxtarenal, or suprarenal), and whether there is iliac aneurysmal involvement; these characteristics are critical to determine the presence of safe proximal and distal endograft attachment sites. The endograft must attach to healthy arterial tissue proximally and distally to ensure adequate seal. The proximal and distal landing zones for the endograft are arguably the most important morphologic aspects of a successful EVAR. Evaluation of the proximal AAA neck is crucial to determine adequate fixation and seal to exclude the aneurysm from blood flow and prevent device migration and failure. For that purpose, all the aortic stent grafts have passive fixation with adequate radial force from stent oversizing and the majority have active fixation with spikes, without or without suprarenal stents. The proximal AAA neck is the distance between the most inferior renal artery and beginning of the aneurysm and is considered the preferred proximal seal zone for the endograft. Many features of the proximal aortic neck affect the appropriateness of EVAR treatment such as length ≥1.5 cm, diameter ≤32 mm, angulation ≤60 degrees, and amount of thrombus and/or calcification. Circumferential thrombus greater than 2 mm in thickness or >50% calcification increases the risk of inadequate seal formation, unwanted pressure transmission to the aneurysmal sac, and thrombus embolization (19,20). Recent studies, however, have questioned this risk by demonstrating that calcification, thrombus, or angulation were not associated with adverse outcomes, and that conical neck morphology was the single strongest predictor (21).
The distal landing zone of the endograft is dependent on the degree of aneurysmal involvement, whether one or both common iliac arteries are involved, and if the aneurysm extends beyond the iliac bifurcation. Likewise, length, diameter, angulation, and calcification are evaluated to determine adequate technical success and endograft seal (Figure 1).
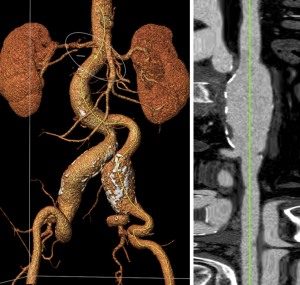
The quality and size of the vascular access site also contributes to the appropriateness of EVAR as these vessels are the sites for device delivery. The preferred access site for EVAR is the common femoral artery. Significant calcifications, tortuosity, and diminutive vessel diameter may be contraindications to EVAR, as these characteristics may complicate device delivery and withdrawal. Morphologic grading systems have been developed to aggregate these anatomic variables to determine procedure candidacy and to assist with pre-procedure planning (3,22).
Relevant vascular anatomy
The AAA treatment zone potentially spans several major aortic branches including the renal artery, inferior mesenteric artery (IMA), and iliac arteries. Each aortic branch is a potential pitfall to the success of EVAR and should be individually evaluated.
Celiac artery and superior mesenteric artery (SMA)
The celiac artery is the first major branch of the abdominal aorta, and the SMA arises just below. The celiac artery and SMA are infrequently involved in the repair of AAAs but adequate patency must be ensured. Occlusion of the IMA from the endograft may result in bowel ischemia in patients without adequate collateral blood from the celiac artery or SMA (Figure 2). The same holds true when endograft placement extends across the bilateral internal iliac arteries resulting in occlusion.
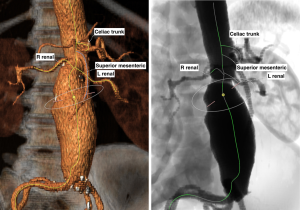
Renal artery
The renal arteries are used to not only classify AAAs but also determine the proximal neck in EVAR evaluation. Abdominal aortic aneurysm classification can be determined by the relation of the aneurysm border to the renal artery. Infrarenal AAAs are defined as having ≥10 mm between the superior aneurysmal border and the inferior margin of the more caudal renal artery. The majority of AAAs fall into this classification, and specifically >15 mm, which would make them potential candidates for EVAR. Juxtarenal AAAs have their superior aneurysmal border extending near the level of the renal arteries, which would preclude those patient from conventional EVAR, but could potentially be candidates for a more complex fenestrated-EVAR (FEVAR) (Figure 3). Suprarenal AAAs extend proximal to the most caudal renal artery and require even more complex reconstructions if endovascular repair is pursued, with fenestrated or branched-EVAR custom-designed modular endografts.
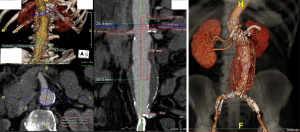
Renal dysfunction is an uncommon but feared complication related to EVAR that may arise from periprocedural contrast use or renal artery compromise from endograft fixation location. There is concern that the suprarenal stent proximal fixation across the renal artery ostia could result in renal dysfunction. However, there are controversial reports in literature and in a recent systematic review regarding suprarenal versus infrarenal stent graft fixation location did not show a significant difference in postoperative renal complications (23). Similarly, endograft coverage and sacrifice of accessory renal arteries may occur, but this has not been shown to correlate with increased renal dysfunction despite correlation with increased renal infarction volume (20,24,25). However, more recent studies report a mild decline in postoperative renal function of patients with covered accessory renal artery after EVAR (26). In addition, coverage of larger (>3 mm) accessory renal artery has been associated with an increased risk of type II endoleak (27).
IMA
The IMA is routinely excluded in endovascular repair of AAAs. Consequently, the IMA is often considered a potential cause of type II endoleaks. Endoleaks are complications that result in persistent blood flow within the aneurysm sac after EVAR. Type II endoleaks are the most common form of endoleak and are the result of retrograde flow from collateral artery. Pre-procedural imaging can be used to determine whether prophylactic embolization of the IMA may be warranted. Ward et al. demonstrated that ancillary embolization of the IMA reduced the incidence of aneurysm sac enlargement, secondary procedures, and endoleak at 24 months post-EVAR (28). Likewise, a recent systematic review demonstrated the technical success of IMA embolization is high and may be warranted in patients with an IMA >3 mm in the prevention of type II endoleaks (29). On the other hand, a patent IMA with a severely stenotic SMA or occluded SMA may place the patient at increased risk for colonic ischemia and may be considered an anatomic contraindication to EVAR (19,20) (Figure 4). In these cases, the pre-operative decision algorithm includes feasibility of endovascular SMA angioplasty, the patency of internal iliac arteries and history of prior colon resection.
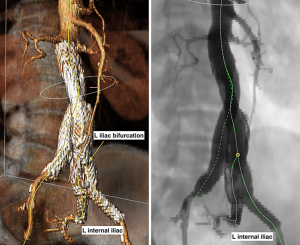
Lumbar arteries
The lumbar arteries are paired aortic branches that arise from the dorsal aspect of the aorta that roughly arise from each vertebral level. The number of patent lumbar arteries determined from preoperative imaging can be used to categorize patients that are at risk for endoleaks (30). Large lumbar arteries arising from the aorta and potentially the IMA should be identified on preoperative imaging. Although this is a topic of debate, some studies have shown a positive correlation between the number of patent lumbar arteries and probability of endoleak (20,31,32). Preoperative embolization of the lumbar arteries and their effects on significant endoleak is still uncertain, and further studies investigating confounding factors that contribute to persistent type II endoleak are needed.
Common iliac artery
The common iliac arteries originate from the aortic bifurcation and subsequently bifurcate into the external and internal iliac arteries. AAAs occasionally extend to involve the common iliac arteries, and their anatomy must be determined prior to EVAR for an assessment of the distal endograft landing zone target. The common iliac arteries are the preferred landing zone and therefore most EVAR endografts are bifurcated. Not only are the common iliac arteries critical in determining the proper distal fixation site, they are also used to dictate proper delivery of the endograft devices. Common iliac artery measurements and characteristics such as diameter, length, thrombus or circumferential calcification, and excessive tortuosity may preclude proper endograft seal formation, delivery, and proper positioning (19,20,33). Less demanding but similar to the proximal landing zone for endograft seal, an adequate distal landing zone length of 10 to 15 mm is required for appropriate endograft seal. Vessel tortuosity complicates device delivery due to the relative stiffness of the devices and is made more complicated by the presence of calcification, though newer devices are more flexible. Additionally, non-favorable common iliac anatomy has been shown to correlate with increased fluoroscopy time and contrast usage (34).
External iliac artery
The external iliac artery originates from the common iliac artery bifurcation and extends through the inguinal ligament. Again, similar attention to vessel tortuosity, calcification, and diameter should be evaluated for potential procedural pitfalls and complications, such as vessel dissection or rupture. Depending on the stent graft, vessels may need to be large enough to accept delivery sheaths up to 18 Fr for EVAR or 22 Fr for FEVAR. If aneurysmal dilation extends into the common iliac arteries, the external iliac artery can become the distal landing zone for endograft seal formation, with prior embolization of the internal iliac artery (19). However, there can be complications associated with unilateral or bilateral internal iliac artery embolization and, lately, preservation of the internal iliac artery can be achieved in select cases with iliac-branch modular devices that require similar preoperative planning and technical expertise to the more complex FEVAR (35).
Internal iliac artery
The internal iliac arteries are divided into two divisions that supply the pelvis. In cases of external iliac artery landing zone placement, the internal iliac artery is often coil embolized proximally to prevent complications of endoleak. Occlusion of the internal iliac artery, whether through embolization or exclusion from endograft placement, has been reported to cause ischemic complications including buttock claudication and erectile dysfunction (36-39). The feared, although rare, major complication of pelvic and bowel ischemia after bilateral internal iliac occlusion may be considered a contraindication to EVAR (36). Although recent systematic reviews performed by Bosanquet et al. and Kouvelos et al. have concluded that bilateral internal iliac artery occlusion rather than unilateral internal iliac artery occlusion results in more overall complications, vascular plugs rather than coils for embolization result in more favorable outcomes including decreased fluoroscopy and contrast usage, and even primary stent coverage rather embolization of the internal iliac arteries is preferred due to improved outcomes without definitive worse complications secondary to endoleak (36-38). Additionally, new endovascular and open developments involving internal iliac artery preservation have been shown to have high technical success with favorable patient outcomes (38,40).
Common femoral artery
The common femoral artery is the anatomic continuation of the external iliac artery distal to the inguinal ligament and is often the site for EVAR vascular access. Adequacy for device delivery should be evaluated on pre and post contrast pre-procedural imaging. General considerations for percutaneous access site should avoid areas of heavy calcification and attention to bony landmarks should also be noted. In adequately screened patients, technical success of percutaneous EVAR access closure is expected to be >80% (41).
Imaging tools for surgical planning
Computed tomography angiography (CTA) has long been considered the preferred imaging modality for surgical planning of EVAR. CTA is readily available, fast, and non-invasive. Despite near-universal use of CTA, there are variations from institution to institution on imaging protocols. In general, pre and post contrast images are obtained. Pre-contrast images are obtained to assess the vessel walls such as presence and degree of calcification. After contrast injection and bolus-tracking software, images are obtained in the arterial phase to optimize evaluation of vascular pathology. Rapid acquisition reduces motion artifact and optimizes contrast evaluation, while thin slice thickness potentiates maximum resolution and enhances subsequent post-processing (42).
Other imaging modalities are available for preoperative imaging in the evaluation of AAA repair, each with their respective advantages and disadvantages. Digital subtraction angiography (DSA) has largely been replaced by CTA as a pre-procedure assessment tool but was once considered the standard for preoperative evaluation. Angiography allows for accurate measurement and detailed real-time evaluation of aortic branches. Angiography is however invasive and is unable to accurately evaluate the qualitative morphologic factors of vessel thrombus and calcification.
Magnetic resonance imaging (MRI) is also used in the preoperative imaging evaluation for AAA repair. MRI is non-invasive and avoids the use of ionizing radiation and iodinated contrast agents; however, it is more susceptible to motion artifact. Goshima et al., compared the feasibility of non-enhanced MRI and CTA in preoperative imaging evaluation of AAA and demonstrated that measurements obtained using non-enhanced MRI were equally accurate to CTA (43). Ultrasound and IVUS have also been utilized in preoperative imaging (Figure 5). Ultrasound is operator dependent and less reliable for required accurate measurements. IVUS is more often used intraoperatively prior to deployment of endograft or throughout the procedure for diameter and length measurements to decrease the intraoperative contrast volume (12).
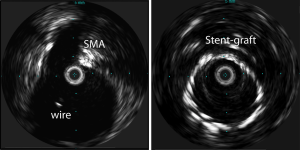
Quantitative measurements based on pre-procedure imaging are critical in determining the technical success of proper stent choice and deployment. Such measurements include but are not limited to the diameter, length and angulation of the proximal aortic neck, aneurysm sac, aortic bifurcation, common and external iliac arteries, however perhaps the most critical measurements relate to the proximal neck. An adequate, >15 mm non-aneurysmal proximal attachment length of infrarenal aorta is arguably the most important and decisive measurement for conventional EVAR placement. Adequate neck length translates to proper seal formation with the end result of improved device fixation, decreased risk of type I endoleaks and reintervention (20). New fenestrated devices have been approved for short, <15 mm, proximal neck length use with good results in high-risk patients for open repair (44). The aortic neck angulation is the angle between the proximal aortic neck and the longitudinal axis of the aneurysm. Unfavorable neck angulation greater than 60 degrees has been considered to be a contraindication to EVAR, although recent studies using more modern, conformable endografts have demonstrated the efficacy of EVAR treatment in patients with unfavorable neck angulation without significant differences in primary or secondary outcomes of EVAR 4 years out (21,45-47). Further long-term results with these modern endograft devices are still ongoing (47). Proximal neck diameter must also be quantified in order to provide proper stent oversizing. Typical manufacturer recommended proximal neck diameters generally require diameters to not be ≥32 mm. Endograft oversizing by 10–20% of the proximal neck allows for adequate radial force against the vessel wall for optimal approximation and therefore decreased long-term risk of aneurysm sac enlargement from device migration and type I endoleaks.
Image processing tools
Three-dimensional (3D) reconstruction after CTA image acquisition is a vital post-processing step in the evaluation prior to EVAR through accurate, true luminal measurements that are otherwise difficult with standard axial CT images (48). 3D CTA images allow for a more comprehensive evaluation of the AAA from all points of view, which is particularly indispensable for morphologically complex aneurysms. Ultimately, a comprehensive and accurate evaluation translates into a more exact fit and surgical plan for EVAR. 2D evaluation when compared to 3D CTA pre-procedure evaluation results in greater inter and intra-observer variability from reproducibility and objectivity which is heavily influenced by vessel tortuosity on axial 2D images (49,50). Sobocinski et al. demonstrated that the proper utilization of a 3D workstation resulted in less type I endoleaks and secondary interventions when compared to pre-procedure imaging evaluation solely by axial 2D CTA (48). CTA 3D reconstruction can also utilize a centerline flow rendering of the aorta and aneurysm, which produces orthogonal images that can be used for pre-procedure imaging evaluation for EVAR, providing an accurate method for aortic measurements (33,51,52). Production of orthogonal images minimizes the inaccurate measurements of a tortuous aorta by allowing for perpendicular measurements. Post-processing reconstruction technologies have advanced and thus have resulted in a wide range of available options. Corriere et al. compared three different reconstruction programs and could demonstrate reproducibility between the three programs that similar results of anatomic measurements and endograft selection could be obtained (53). Lastly, 3D printing of complex aortic aneurysms has evolved as a new 3D tool to assist with preoperative planning in fenestrated endograft design and prediction of endograft deployment in challenging cases.
Complex EVAR options
Lastly, pre-operative imaging for current fenestrated and branched EVAR options is particularly important and where it is needed most. Fenestrated and branched EVAR options have reduced the limitations of the proximal landing-zone, which has long been considered a major limitation for conventional EVAR. Traditionally, proximal landing zones would require aneurysms that do not extend above or near the renal or mesenteric arteries as the endograft would have to extend across and above these arteries, thus compromising the perfusion of the viscera. However, with the advent of branched and fenestrated endograft technology, exclusion of the aneurysm while also maintain visceral artery perfusion became possible. Fenestrated and branched endografts, are endografts with openings and side branches arising directly from the graft and include the visceral artery with the endograft. Recent data suggest that fenestrated and branched endograft placement results in relatively safe, effective, and durable outcomes in high-surgical risk patients treated for complex aortic aneurysms (17,18,44,54,55). As complex EVAR experience and use with branched and fenestrated endografts continue to grow preoperative imaging for endograft customization becomes increasingly important. Accurate measurements that determine size and location of the fenestrations or branches of the endograft are evaluated and incorporated into the device design based on each manufacture and patient specifications (17). Deviation between true aneurysm anatomy and endograft design may result in device failure. Again measurements should be obtained through high resolution contrast-enhanced CTA with centerline flow reconstruction for optimal accuracy. Complex EVAR endograft technology will continue to evolve but pre-operative imaging for device design will continue to remain a mainstay of success.
Conclusions
Appropriate candidacy for EVAR derives from clinical, anatomic, and morphologic factors that are unique to each patient. Morphologic and anatomic factors that can be accurately and effectively evaluated on pre-procedure imaging, such as CTA, help in assessment and preparation for the procedure. In addition to the evaluation of the aneurysm sac, proximal landing zone and distal landing zone, the aortic branch vessels must be thoroughly evaluated and quantified for the technical success of EVAR and prevention of reintervention. Further developments and advancements in endograft and imaging technology will indisputably continue to transpire and will continue evolve the dynamic field of EVAR. A thorough pre-operative planning on a 3D-rendering workstation and a skilled endovascular team in a dedicated hybrid suite set the current standards for a successful outcome in the endovascular treatment of both uncomplicated and complex AAAs.
Acknowledgements
None.
Footnote
Conflicts of Interest: The authors have no conflicts of interest to declare.
References
- Dua A, Kuy S, Lee CJ, et al. Epidemiology of aortic aneurysm repair in the United States from 2000 to 2010. J Vasc Surg 2014;59:1512-7. [Crossref] [PubMed]
- Chaikof EL, Brewster DC, Dalman RL, et al. SVS practice guidelines for the care of patients with an abdominal aortic aneurysm: executive summary. J Vasc Surg 2009;50:880-96. [Crossref] [PubMed]
- Walker TG, Kalva SP, Yeddula K, et al. Clinical practice guidelines for endovascular abdominal aortic aneurysm repair: written by the Standards of Practice Committee for the Society of Interventional Radiology and endorsed by the Cardiovascular and Interventional Radiological Society of Europe and the Canadian Interventional Radiology Association. J Vasc Interv Radiol 2010;21:1632-55. [Crossref] [PubMed]
- EVAR trial participants. Endovascular aneurysm repair versus open repair in patients with abdominal aortic aneurysm (EVAR trial 1): randomised controlled trial. Lancet 2005;365:2179-86. [Crossref] [PubMed]
- Lederle FA, Freischlag JA, Kyriakides TC, et al. Outcomes following endovascular vs open repair of abdominal aortic aneurysm: a randomized trial. JAMA 2009;302:1535-42. [Crossref] [PubMed]
- Picel AC, Kansal N. Essentials of endovascular abdominal aortic aneurysm repair imaging: preprocedural assessment. AJR Am J Roentgenol 2014;203:W347-57. [PubMed]
- Prinssen M, Verhoeven EL, Buth J, et al. A randomized trial comparing conventional and endovascular repair of abdominal aortic aneurysms. N Engl J Med 2004;351:1607-18. [Crossref] [PubMed]
- Lederle FA, Wilson SE, Johnson GR, et al. Immediate repair compared with surveillance of small abdominal aortic aneurysms. N Engl J Med 2002;346:1437-44. [Crossref] [PubMed]
- EVAR trial participants. Endovascular aneurysm repair and outcome in patients unfit for open repair of abdominal aortic aneurysm (EVAR trial 2): randomised controlled trial. Lancet 2005;365:2187-92. [Crossref] [PubMed]
- Sicard GA, Zwolak RM, Sidawy AN, et al. Endovascular abdominal aortic aneurysm repair: long-term outcome measures in patients at high-risk for open surgery. J Vasc Surg 2006;44:229-36. [Crossref] [PubMed]
- Gallitto E, Faggioli G, Gargiulo M, et al. Planning, execution, and follow-up for endovascular aortic aneurysm repair using a highly restrictive iodinated contrast protocol in patients with severe renal disease. Ann Vasc Surg 2018;47:205-211. [PubMed]
- Pisimisis GT, Khoynezhad A, Bashir K, et al. Incidence and risk factors of renal dysfunction after thoracic endovascular aortic repair. J Thorac Cardiovasc Surg 2010;140:S161-7. [Crossref] [PubMed]
- Mohammed S, Kougias P, Mandviwala TM, et al. Progression to stage 3 and 4 chronic kidney disease and risk factor stratification following endovascular aortic aneurysm repair. Vascular 2016;24:598-603. [Crossref] [PubMed]
- Pisimisis GT, Bechara CF, Barshes NR, et al. Risk factors and impact of proximal fixation on acute and chronic renal dysfunction after endovascular aortic aneurysm repair using glomerular filtration rate criteria. Ann Vasc Surg 2013;27:16-22. [Crossref] [PubMed]
- Walsh SR, Boyle JR, Lynch AG, et al. Suprarenal endograft fixation and medium-term renal function: systematic review and meta-analysis. J Vasc Surg 2008;47:1364-70. [Crossref] [PubMed]
- Oderich GS, Ribeiro M, Reis de Souza L, et al. Endovascular repair of thoracoabdominal aortic aneurysms using fenestrated and branched endografts. J Thorac Cardiovasc Surg 2017;153:S32-41.e7. [Crossref] [PubMed]
- Greenberg RK, Sternbergh WC 3rd, Makaroun M, et al. Intermediate results of a United States multicenter trial of fenestrated endograft repair for juxtarenal abdominal aortic aneurysms. J Vasc Surg 2009;50:730-7.e1. [Crossref] [PubMed]
- Mastracci TM, Greenberg RK, Eagleton MJ, et al. Durability of branches in branched and fenestrated endografts. J Vasc Surg 2013;57:926-33; discussion 933. [Crossref] [PubMed]
- Bromley PJ, Kaufman JA. Abdominal aortic aneurysms before and after endograft implantation: Evaluation by computed tomography. Tech Vasc Interv Radiol 2001;4:15-26. [Crossref] [PubMed]
- Iezzi R, Cotroneo AR. Endovascular repair of abdominal aortic aneurysms: CTA evaluation of contraindications. Abdom Imaging 2006;31:722-31. [Crossref] [PubMed]
- Pitoulias GA, Valdivia AR, Hahtapornsawan S, et al. Conical neck is strongly associated with proximal failure in standard endovascular aneurysm repair. J Vasc Surg 2017;66:1686-95. [Crossref] [PubMed]
- Chaikof EL, Fillinger MF, Matsumura JS, et al. Identifying and grading factors that modify the outcome of endovascular aortic aneurysm repair. J Vasc Surg 2002;35:1061-6. [Crossref] [PubMed]
- Miller LE, Razavi MK, Lal BK. Suprarenal versus infrarenal stent graft fixation on renal complications after endovascular aneurysm repair. J Vasc Surg 2015;61:1340-9.e1. [Crossref] [PubMed]
- Greenberg JI, Dorsey C, Dalman RL, et al. Long-term results after accessory renal artery coverage during endovascular aortic aneurysm repair. J Vasc Surg 2012;56:291-6; discussion 296-7. [Crossref] [PubMed]
- Antoniou GA, Karkos CD, Antoniou SA, et al. Can an accessory renal artery be safely covered during endovascular aortic aneurysm repair? Interact Cardiovasc Thorac Surg 2013;17:1025-7. [Crossref] [PubMed]
- Sadeghi-Azandaryani M, Zimmermann H, Korten I, et al. Altered renal functions in patients with occlusion of an accessory renal artery after endovascular stenting of an infrarenal aneurysm. J Vasc Surg 2017;65:635-42. [Crossref] [PubMed]
- Malgor RD, Oderich GS, Vrtiska TJ, et al. A case-control study of intentional occlusion of accessory renal arteries during endovascular aortic aneurysm repair. J Vasc Surg 2013;58:1467-75. [Crossref] [PubMed]
- Ward TJ, Cohen S, Fischman AM, et al. Preoperative inferior mesenteric artery embolization before endovascular aneurysm repair: decreased incidence of type II endoleak and aneurysm sac enlargement with 24-month follow-up. J Vasc Interv Radiol 2013;24:49-55. [Crossref] [PubMed]
- Manunga JM, Cragg A, Garberich R, et al. Preoperative inferior mesenteric artery embolization: a valid method to reduce the rate of type II endoleak after EVAR? Ann Vasc Surg 2017;39:40-7. [Crossref] [PubMed]
- Piazza M, Squizzato F, Miccoli T, et al. Definition of Type II endoleak risk based on preoperative anatomical characteristics. J Endovasc Ther 2017;24:566-72. [Crossref] [PubMed]
- Fan CM, Rafferty EA, Geller SC, et al. Endovascular stent-graft in abdominal aortic aneurysms: the relationship between patent vessels that arise from the aneurysmal Sac and early endoleak. Radiology 2001;218:176-82. [Crossref] [PubMed]
- Lalys F, Durrmann V, Dumenil A, et al. Systematic review and meta-analysis of preoperative risk factors of type II endoleaks after endovascular aneurysm repair. Ann Vasc Surg 2017;41:284-93. [Crossref] [PubMed]
- Lau C, Feldman DN, Girardi LN, et al. Imaging for surveillance and operative management for endovascular aortic aneurysm repairs. J Thorac Dis 2017;9:S309-16. [Crossref] [PubMed]
- Rockman C. Reducing complications by better case selection: anatomic considerations. Semin Vasc Surg 2004;17:298-306. [Crossref] [PubMed]
- Schneider DB, Matsumura JS, Lee JT, et al. Prospective, multicenter study of endovascular repair of aortoiliac and iliac aneurysms using the Gore Iliac Branch Endoprosthesis. J Vasc Surg 2017;66:775-85. [Crossref] [PubMed]
- Bosanquet DC, Wilcox C, Whitehurst L, et al. Systematic review and meta-analysis of the effect of internal iliac artery exclusion for patients undergoing EVAR. Eur J Vasc Endovasc Surg 2017;53:534-48. [Crossref] [PubMed]
- Kouvelos GN, Koutsoumpelis A, Peroulis M, et al. In endovascular aneurysm repair cases, when should you consider internal iliac artery embolization when extending a stent into the external iliac artery? Interact Cardiovasc Thorac Surg 2014;18:821-4. [Crossref] [PubMed]
- Kouvelos GN, Katsargyris A, Antoniou GA, et al. Outcome after interruption or preservation of internal iliac artery flow during endovascular repair of abdominal aorto-iliac aneurysms. Eur J Vasc Endovasc Surg 2016;52:621-34. [Crossref] [PubMed]
- Rayt HS, Bown MJ, Lambert KV, et al. Buttock claudication and erectile dysfunction after internal iliac artery embolization in patients prior to endovascular aortic aneurysm repair. Cardiovasc Intervent Radiol 2008;31:728-34. [Crossref] [PubMed]
- Fernández-Alonso L, Fernández-Alonso S, Grijalba FU, et al. Endovascular treatment of abdominal aortic aneurysms involving iliac bifurcation: role of iliac branch graft device in prevention of buttock claudication. Ann Vasc Surg 2013;27:851-5. [Crossref] [PubMed]
- Bechara CF, Barshes NR, Pisimisis G, et al. Short- and midterm results of iliac artery stenting for flush occlusion with the assistance of an occlusive contralateral iliac artery balloon. Ann Vasc Surg 2014;28:59-64. [Crossref] [PubMed]
- Beebe HG, Kritpracha B. Computed tomography scanning for endograft planning: evolving toward three-dimensional, single source imaging. Semin Vasc Surg 2004;17:126-34. [Crossref] [PubMed]
- Goshima S, Kanematsu M, Kondo H, et al. Preoperative planning for endovascular aortic repair of abdominal aortic aneurysms: feasibility of nonenhanced MR angiography versus contrast-enhanced CT angiography. Radiology 2013;267:948-55. [Crossref] [PubMed]
- Oderich GS, Greenberg RK, Farber M, et al. Results of the United States multicenter prospective study evaluating the Zenith fenestrated endovascular graft for treatment of juxtarenal abdominal aortic aneurysms. J Vasc Surg 2014;60:1420-8.e1-5.
- Sternbergh WC, Carter G, York JW, et al. Aortic neck angulation predicts adverse outcome with endovascular abdominal aortic aneurysm repair. J Vasc Surg 2002;35:482-6. [Crossref] [PubMed]
- Zeng Q, Huang L, Huang X, et al. Endovascular repair of abdominal aortic aneurysm with severely angulated neck and tortuous artery access: case report and literature review. BMC Surg 2015;15:20. [Crossref] [PubMed]
- Oliveira NF, Bastos Goncalves FM, de Vries JP, et al. Mid-term results of EVAR in severe proximal aneurysm neck angulation. Eur J Vasc Endovasc Surg 2015;49:19-27. [Crossref] [PubMed]
- Sobocinski J, Chenorhokian H, Maurel B, et al. The benefits of EVAR planning using a 3D workstation. Eur J Vasc Endovasc Surg 2013;46:418-23. [Crossref] [PubMed]
- Pitoulias GA, Donas KP, Schulte S, et al. Two-dimensional versus three-dimensional CT angiography in analysis of anatomical suitability for stentgraft repair of abdominal aortic aneurysms. Acta Radiol 2011;52:317-23. [Crossref] [PubMed]
- Ihara T, Komori K, Yamamoto K, et al. Three-dimensional workstation is useful for measuring the correct size of abdominal aortic aneurysm diameters. Ann Vasc Surg 2013;27:154-61. [Crossref] [PubMed]
- Higashiura W, Kichikawa K, Sakaguchi S, et al. Accuracy of centerline of flow measurement for sizing of the Zenith AAA endovascular graft and predictive factor for risk of inadequate sizing. Cardiovasc Intervent Radiol 2009;32:441-8. [Crossref] [PubMed]
- Velazquez OC, Woo EY, Carpenter JP, et al. Decreased use of iliac extensions and reduced graft junctions with software-assisted centerline measurements in selection of endograft components for endovascular aneurysm repair. J Vasc Surg 2004;40:222-7. [Crossref] [PubMed]
- Corriere MA, Islam A, Craven TE, et al. Influence of computed tomography angiography reconstruction software on anatomic measurements and endograft component selection for endovascular abdominal aortic aneurysm repair. J Vasc Surg 2014;59:1224-31.e1-3.
- Ou J, Chan YC, Cheng SW. A systematic review of fenestrated endovascular repair for juxtarenal and short-neck aortic aneurysm: evidence so far. Ann Vasc Surg 2015;29:1680-8. [Crossref] [PubMed]
- Yaoguo Y, Zhong C, Lei K, et al. Treatment of complex aortic aneurysms with fenestrated endografts and chimney stent repair: Systematic review and meta-analysis. Vascular 2017;25:92-100. [Crossref] [PubMed]