Pulmonary vein remodeling following pulmonary vein isolation in patients with atrial fibrillation—do pulmonary veins represent only an epiphenomenon? A cardiac MRI study
Introduction
Atrial fibrillation (AF) is associated with adverse anatomical, physiological, and electrical changes at the level of the atria (1). These changes, if not interrupted, lead to a vicious circle of further deterioration affecting both atrial and ventricular levels, the so-called adverse remodeling (1).
In 1998, Haïssaguerre et al. noted that the pulmonary veins (PVs) were the initiating source of abnormal electrical activity in atrial fibrillation (2). Further, they showed that isolation of the PVs can abolish AF in many cases. The interventional approach to maintain normal sinus rhythm (NSR) has focused on pulmonary vein isolation (PVI) for drug resistant AF patients. Radiofrequency current or cryoballoon are usually used to create a transmural scar of the atrial tissue near the origin of each PV, thereby electrically isolating the PV from the atria (3).
It has been demonstrated that cardiac volumes remodel with successful PVI. We and others (4) demonstrated that in patients with successful PVI procedures, the left atrium undergoes favorable reverse remodeling and that the degree of remodeling is directly related to the long term PVI success rate (5).
A recent meta-analysis which included 63 studies addressing the topic of AF recurrence after PVI showed a single-procedure success rate of ablation with no antiarrhythmic drug therapy after 12 months follow-up of only 57% (6).
Thus, the search for predictors of outcome is ongoing, with a strong focus on the main body of the left atrium pre and post-PVI (1,7). Interestingly, despite intense investigation into this phenomenon, few investigations have directly addressed PV remodeling (8) and even fewer have focused on the PVs as a likely candidate marker for prediction of PVI success (9). Importantly, regarding the minority of investigations of the PVs there was no consensus concerning the site at which to measure the PV ostia. Most studies measured a distal segment remote from the area of PVI ablation focus. Thus, considerable heterogeneity exists in evaluation of the impact of PVI on the PVs’ anatomy, yet it retains biologic plausibility as it is the near focus for all ablative attempts (9,10).
Here we propose to define a reliable and reproducible method to measure the PV ostia directly at the exit site from the LA, i.e., not distal. We also seek to demonstrate if PV conditions in the pre-PVI state can serve as a marker for future long-term success defined as maintenance of NSR for >6 months.
Methods
This retrospective study on patients’ data was approved by the institutional review board (IRB) of the hospital (Allegheny General Hospital, Pittsburgh, PA).
Patient selection
Patients (n=100) were retrospectively and consecutively selected from those who remained symptomatic for AF despite medical therapy (medication use of: 1 drug, 67.7%; 2 drugs, 23.5%; and 3 or more, 8.8% of the patients, respectively) and who were scheduled to undergo standard PVI at our institution between the July 2007 and September 2011. All patients underwent a baseline cardiovascular magnetic resonance (CMR) imaging and magnetic resonance angiography examination of the left atria and PVs prior to PVI (typical time to PVI was 1–3 days). Following PVI, a follow-up CMR/MRA examination was performed at 6±2 months.
Patients with clinically significant PV stenosis were excluded. None of the patients studied had symptoms or signs of PV stenosis syndrome (11).
Study protocol
All patients underwent pre and post CMR scanning on a 1.5 T GE (GE CVI Excite Version, Milwaukee, WI, USA) cardiac MRI scanner. Cardiac structure and function was evaluated using a uniform CMR examination which included the vertical long axis (VLA), horizontal long axis (HLA), 3-chamber view, left ventricular outflow tract view (LVOT), right ventricular outflow tract view (RVOT), and MRA of the LA and PVs performed during administration of 0.15 mg/kg of gadolinium contrast agent followed by 3D volumetric manual reconstruction. All functional images were obtained using multiple breath-holds, ECG gated, steady state free-precession (TR/TE/flip 4.2°/2.1°/60°) cine MRI techniques.
Following the baseline CMR examination, patients underwent standard circumferential PVI radiofrequency ablation in the electrophysiology lab at the same institution. Patients were followed after the initial standard three-month blanking period to evaluate PVI success as described below. The follow-up CMR examination was identical to the baseline examination and allowed measurement of PV cross sectional areas (CSA) and LA volume.
CMR measures
Atrial and PV features were measured from the CMR image data using visualization/measurement software operating on a GE workstation ADW 4.2. The CSA of each PV at the ostium, adjacent to the antrum was systematically measured using a 3D angiographic view of the left atrium. In addition to the four expected PVs (right superior, right inferior, left superior, left inferior) occurrence of common and mid PVs were also noted. A common PV was defined as a single PV entering the left atrium on either side, as confirmed in all views (sagittal, coronal and axial). A middle PV was defined as any right or left sided PV entering the left atrium separately from the upper and lower veins on the same side as determined from all views.
A single investigator (SK) performed the measurements in a uniform manner blinded to the patient’s electrophysiological status. The anatomy and shape of each patient’s LA and the number and site of exit of their PVs were examined. This was accomplished using 3D multiplanar imaging with triangulation and detection of PV’s exit in multiple views, including axial, sagittal and coronal. Following this, the decision of where to measure the PV ostium was made depending on the transition between the LA-proper and the PV when the transition was well defined. However, in many cases, there was a gradual transition with no clear demarcation. In this case, we adopted the rule of measuring the PV ostium at the point 0.5 cm from the LA-proper and before any branching occurred. After this decision was made, combining information from multiple views (axial, coronal and sagittal), triangulation was performed using the LA 3D MRA to orient the target PV perpendicular to its long axis in order to measure the CSA (Figures 1-5). These rules were uniformly applied to each patient’s pre and follow-up studies to achieve close to identical if not superimposable measuring points. The time for analysis of each patient varied according to the number of veins and on the clarity of determining the location of the exit site, but after a training series of 20 patients, analysis per study was completed in approximately 20±10 minutes. To assess the degree of reproducibility in measuring the CSA, another investigator (GR), after receiving training on 20 cases, measured 20 more cases (20%) at baseline and follow-up. The same software package was used to measure left atrial volume, identifying the boundary by its high contrast and manually excluding the PVs and appendage (12). This protocol was established to ensure good reproducibility of the findings
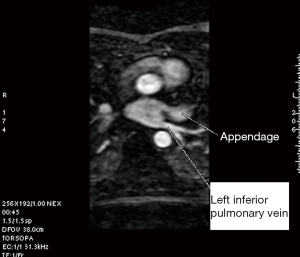
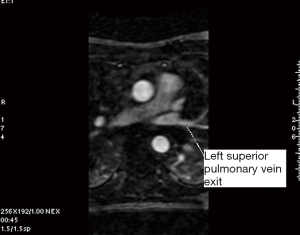
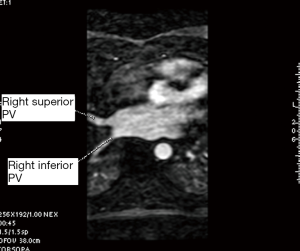
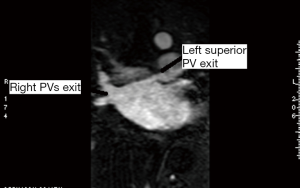
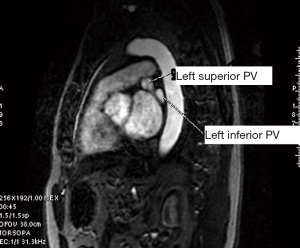
PVI procedure
With the patient under general anesthesia, the PVI procedure was performed by a single operator (WB). All cases were ablated using radiofrequency. The basic procedure was essentially similar for all cases. The heart was approached through the right femoral vein. The CMR generated 3D angiogram of the left atrium and the PVs was used as a template to start the electrophysiological/anatomical mapping. This was done using the EnSite mapping system. Each of the four (or occasionally more) PVs was isolated. The ablation was antral and circumferential. Extra lines of ablation were performed only if indicated by the presence of an active focus. Approximately 70% of the patients had extra lines performed, i.e., sites other than the 4 PVs including the roof and posterior wall. Absence of PV potentials was confirmed post-ablation. Patients were typically cardioverted partway through the procedure and remained in NSR, as a confirmation of complete electrical isolation. All patients tolerated the procedure well with no apparent complications.
Follow up for detection of recurrence
After the procedure, patients were admitted to the telemetry floor for overnight monitoring and observation. They were discharged next day on an antiarrhythmic drug for 3 months (blanking period) and the Coumadin dose was adjusted to reach a target INR of 2–3.
In the absence of patient-reported symptoms, patients were evaluated for symptoms during follow-up monthly hospital visits in the outpatient arrhythmia clinic of Allegheny General Hospital. After the 3-month blanking period, patients were assessed using a Holter monitor for 14 days to assess AF recurrence. A similar 14 days Holter assessment was performed at 6 months. In between the two Holter studies, patients had at least 3 electrocardiograms (ECGs) at routine clinic follow up (at least one/month). Patients were classified as responders (R) if they exhibited none or <30 seconds of AF during Holter monitoring and no AFib by ECG criteria while non-responders (NR) were defined by any documented atrial arrhythmia occurring after the blanking period and lasting >30 s (13) or any AFib detected by ECG.
Statistical analysis
The combined area of the PVs was calculated and compared between baseline and follow-up for the whole population and grouped by responders (R) and non-responders (NR). Paired t-test was used to determine the significance of changes between baseline and follow-up, a P value of ≤0.05 was considered significant. The LA volume was indexed to body surface area (BSA) and was compared in the pre and post conditions in both R and NR groups using a paired t-test. Correlation tests were performed to identify association between baseline, follow-up and changes of the measured variables. ANOVA test was performed to compare the 4 main PVs with each other in R and NR groups. For reproducibility, a Bland-Altman analysis was performed Analysis was performed using SPSS software (PASW v.18, Chicago, USA).
Results
Base line characteristics
All 100 patients (71% male) with AF successfully underwent PVI with 74 (74%) responders and 26 (26%) non-responders at follow-up (6±2 months). Demographic data including age, BSA and antiarrhythmic therapies were similar between the R and NR groups. Other baseline characteristics including medical conditions also did not show and difference between groups (Table 1).

Full table
A total of 394 PVs and 100 LAs were successfully measured in pre and post PVI, MRI studies, with average time needed for MRI assessment per patient of (20±10 min per exam). In equivocal cases, a decision was achieved by consensus. Non-standard PV variants (mid and common PVs) were seen 20 times in 19 patients; 14 responders (18.9%) and 5 non-responders (19%) with one patient having only a common right and a common left PV. In summary; a left common PV was found in 12 patients (12%), right common in 3 patients (3%) and right middle in 5 patients (5%). Fifty-two percent of the total population had persistent AF while 48% had paroxysmal AF.
LA volume
The LA volume index was not significantly different between R and NR groups at base line. While the LA volume index decreased in both R and NR groups, the reduction was more pronounced in responders (61±18 to 53±15 mL/m2; P<0.001 vs. 67±18 to 62±17 mL/m2; P=0.03) leading to a significant difference in the LA volume index at the post PVI conditions (P=0.02) (Table 2).
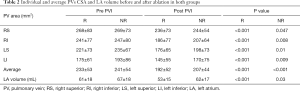
Full table
PV metrics pre-procedure
At baseline, the average PV CSA did not exhibit any significant difference between R and NR groups (233±53 vs. 241±54 mm2; P=0.5) indicating no predictive capability at baseline. However, there was a non-significant (P=0.5) trend for CSA to be smaller at baseline in R vs. NR in all 4 main PV CSAs (Figure 6).
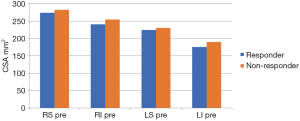
PV metrics post procedural
The average CSA for each PV considered alone or as a group showed a significant reduction in size pre to post ablation. Reduction in CSA occurred significantly in both groups; in R (233±53 to 192±52 mm2, P<0.001) and NR (241±54 to 207±44 mm2, P<0.001). However, there was no significant difference in the percent changes pre to post between groups (Figure 7). Unlike the relation in LA volume, the difference between R and NR in the post conditions was not significant (192±52 to 207±44 mm2, P=0.19) (Table 2).
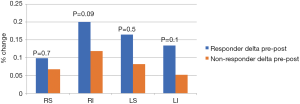
Correlation to LA reduction in size
Interestingly, the reduction in area of each vein only weakly correlated with the reduction of the LA volume when considered individually (r=0.4, 0.3, 0.2, 0.3 for right superior (RS), right inferior (RI), left superior (LS), left inferior (LI), respectively, P<0.01 for all except LS (P=0.07). However, when considering the average reduction of CSA for all four PVs there was a moderate correlation with reduction of the left atria (r=0.48, P<0.001).
Difference between the 4 PVs
Prior to PVI, the 4 main PVs demonstrated a relative difference between each other with respect to CSA, with the right superior being the largest, followed by the right inferior, left superior and then the left inferior, P<0.001 (Figure 6). Following PVI, the magnitude of PV reduction was also not uniform, with the right inferior pulmonary being the most affected (18%±20%) while the right superior changed the least (9%±20%) with the left superior (14%±30%) and left inferior (11%±27%) being intermediate (ANOVA test P value 0.07 for R vs. NR and 0.2 for individual veins).
Prediction of outcome for maintenance of NSR following PVI
A systematic attempt was made to relate post-PVI outcomes to pre-PVI baseline conditions to determine a mode or anatomic metric to predict PVI success or failure. With regard to PV conditions pre-PVI, no parameter demonstrated a significant difference between R and NR groups, including: (I) individual and average CSA of the 4 main PVs (right superior and inferior, left superior and inferior); (II) standard deviation; (III) the coefficient of variation; (IV) maximum vein CSA; (V) minimum vein CSA; (VI) absolute and (VII) the percentage of change before vs. after the procedure (Table 3).
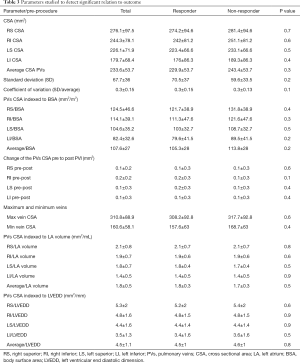
Full table
Reproducibility
The Bland-Altman analysis for reproducibility of measuring CSA showed a low bias (0.2 mm2) with 2 SD limits of agreement of 67 to −67 and 60 to −62 for the pre and post readings, respectively. The kappa correlation between the 2 readers was 0.7 and 0.8 (‘moderate’ to ‘near perfect’ agreement, respectively) for pre- and post-readings, respectively. The difference between the pre and post readings for the repeated group were almost identical (17% for reader 1 and 16% for reader 2 with P<0.005).
Discussion
In the search for a predictive model of long-term success of PVI, one of the elements that has not been extensively considered was the very focus of the PVI procedure, namely the PV’s ostia. Accordingly, determining where and how to measure the PVs ostia in a uniform manner is mandatory if one is to effectively incorporate this metric in any prediction model or assessment. Here, we described a new and highly reproducible method to define how to measure the ostium, given its expected closer relation to LA changes and its proximity to the site of ablation.
In this study, we confirmed the previous finding of significant LA reverse remodeling following PVI, which was greater for responders, but present for both groups. This likely reflects the beneficial anatomic and physiologic effects of attaining NSR. Herein we advanced the veracity of this finding for the LA volume measured from 3D MRA, while in our previous paper (4) as well as in the work of others (14), LA volume was calculated by the ellipse geometric 2D method (15).
The second important finding was characterization of the PVs conditions in response to the PVI procedure. PVs demonstrated a significant reduction in their CSA both in R and NR groups. PV CSA reduction not meeting the degree to cause PV stenosis syndrome is defined as remodeling (16). Those were the target population in our study, as we excluded any patient with clinical manifestations of PV stenosis syndrome. This finding was previously shown by Jayam et al. (8) but in fewer patients (n=51) and over a shorter follow-up interval (6–8 weeks). However, they did not relate these findings to success or failure. Further, in their study, the PV diameters (1D) were measured while we used the CSAs (2D). The longer follow-up interval between studies in our data allows for stabilization of the LA and PV condition after ablation and an appropriate window for AF recurrence to manifest. Further, the longer follow-up interval allows any expected reverse remodeling changes in LA and PVs to manifest.
We expected that reduction in CSA of the PVs at the site investigated here would strongly parallel the LA reverse remodeling. Pressure and volume changes affect both the LA and PVs as a unit, as seen in cases such as mitral regurgitation and AF, where both LA and PVs dilate and undergo adverse remodeling changes (17). Here, we found that the average reduction of the PV size moderately correlated to the reduction of LA size after ablation. This moderate correlation taken together with the findings of Hauser et al. (18) that the degree of reduction of the size of PV is linearly related to the amount of scar resulting from the process of ablation, indicates that part of the mechanism of PVs reverse remodeling includes but is not restricted to, the reduction of the LA volume. Further studies are needed to determine the proportion attributable to the components of fibrosis and functional remodeling, affecting both LA and PVs.
To address the main focus of our study, we anticipated that there would be a direct relation to predict procedural outcome dependent on the pre-PVI PVs’ conditions. However, despite intense efforts, we did not show a relationship. We note that Hauser et al. (9) also tested this hypothesis. Their study identifies the PVs as “predictors of PVI success”. Our results are in distinct disagreement. They reported 71 patients with pre and post PVI MRI studies. Their results demonstrated that those who had one or more “large pulmonary veins” related more to late recurrence of AF than others. They defined the “large PV as being in the top 10th percentile of their PVI population”. Importantly, the site where the PV ostia were measured was one of three main and crucial differences with our study. While our study was larger, they measured at the site of “separation of the PV from LA and each other as judged in the sagittal view”. They argue their attraction for that site was based on a previous small study of 24 patients in which measurements of the CSA at that site was more reproducible than measuring at the junction with better correlation with the diameters at the same site (19). We, however, measured at the exact junction between the PV and the LA as determined in multiple views, including axial, sagittal and coronal, within 0.5 cm of the LA body and before any bifurcation. Indeed, we argue that this is in the proximate location of the os/antrum where the ablation site occurred, representing the opportunity for closer interrogation of the effects of PVI. Further, our reproducibility tested in this way demonstrated very good results. In summary, our argument for choosing to put substantial effort in measuring at this site, as close to the LA as possible, and accordingly define a road map for achieving good reproducibility were for the following reasons:
- This is the site closest to the LA and most related to the anatomical and physiological changes directly affected via PVI.
- Anatomically, we know that myocardial muscle fibers extend from the LA into all the PVs with a length of 1–3 cm; the muscular sleeves are thickest at the proximal end of the veins (1–1.5 mm) and gradually taper distally (20).
- The electrophysiological significance of the proximal PV has long been understood (21) having the greater innervated muscle sleeve acting as the catalyst for the trigger firing of AF (22), i.e., it is the primary site of AF triggering, the site closest to ablation and the site that naturally relates mostly to the LA changes.
Another drawback with measuring at a more distal site is that this can often miss common PVs, a frequent occurrence, which would create a significant difference in the measured diameters, resulting in even less reliable results. Also, ablation line distance and intensity would be expected to be different for a common vein compared to separate veins.
Finally, regarding the quest to identify a correlation between PV size and ablation outcome, we sought a direct and clinically applicable parameter that would act as a clinical tool in which to predict outcome. With reference to Hauser’s study where a ‘positive finding’ was noted, they relied exclusively on the definition of ‘the largest PV as being in the highest 10th percentile’ to retrospectively define success. This is somewhat confusing and difficult to apply generally on a single patient basis. Taken together, we believe that the results obtained by the approach we utilized reliably raise several questions as to the ‘apparent’ use of PVs metrics as a clinical predictor of PVI success. So, as we started trying to confirm the positive relation between PV remodeling and PVI outcome, we ended to the conclusion that further studies are still needed to confirm or deny either or both theories.
A recent paper (23) showed similar negative results regarding a different aspect involving the PVs, namely the electrophysiological aspect. They studied the pre-ablation electrical activity of the PVs in relation to prediction of ablation outcome and concluded that the rapidity of PV firing or presence of fibrillation within the PV was not predictive of outcome. This added more emphasis to the concept described here; that despite the well documented role of the PVs in AF generation, their anatomical and electrophysiological role in prediction of ablation outcome is, as yet, not clear and likely not predictive of long-term success following PVI.
Acknowledgements
None.
Footnote
Conflicts of Interest: The authors have no conflicts of interest to declare.
Ethical Statement: This retrospective study on patients’ data was approved by the institutional review board (IRB) and the ethical committee of Allegheny General Hospital, Pittsburgh, PA (IRB Registration #00007364).
References
- Nattel S, Harada M. Atrial remodeling and atrial fibrillation: recent advances and translational perspectives. J Am Coll Cardiol 2014;63:2335-45. [Crossref] [PubMed]
- Haïssaguerre M, Jaïs P, Shah DC, et al. Spontaneous initiation of atrial fibrillation by ectopic beats originating in the pulmonary veins. N Engl J Med 1998;339:659-66. [Crossref] [PubMed]
- Xu J, Huang Y, Cai H, et al. Is cryoballoon ablation preferable to radiofrequency ablation for treatment of atrial fibrillation by pulmonary vein isolation? A meta-analysis. PLoS One 2014;9:e90323. [Crossref] [PubMed]
- Reddy ST, Belden W, Doyle M, et al. Mitral regurgitation recovery and atrial reverse remodeling following pulmonary vein isolation procedure in patients with atrial fibrillation: a clinical observation proof-of-concept cardiac MRI study. J Interv Card Electrophysiol 2013;37:307-15. [Crossref] [PubMed]
- Zalkind D, Joshi S, Richardson A, et al. 2042 Reduction in pulmonary vein size after atrial fibrillation ablation in the absence of pulmonary vein stenosis. J Cardiovasc Magn Reson 2008;10:A311. [Crossref]
- Epicoco Md G. Sorgente Md PhD A. Predictors of Atrial Fibrillation Recurrence after Catheter Ablation. J Atr Fibrillation 2014;6:1016. [PubMed]
- Tan ES, Mulder BA, Rienstra M, et al. Pulmonary vein isolation of symptomatic refractory paroxysmal and persistent atrial fibrillation: A single centre and single operator experience in the Netherlands. Neth Heart J 2009;17:366-72. [Crossref] [PubMed]
- Jayam VK, Dong J, Vasamreddy CR, et al. Atrial volume reduction following catheter ablation of atrial fibrillation and relation to reduction in pulmonary vein size: an evaluation using magnetic resonance angiography. J Interv Card Electrophysiol 2005;13:107-14. [Crossref] [PubMed]
- Hauser TH, Essebag V, Baldessin F, et al. Prognostic value of pulmonary vein size in prediction of atrial fibrillation recurrence after pulmonary vein isolation: a cardiovascular magnetic resonance study. J Cardiovasc Magn Reson 2015;17:49. [Crossref] [PubMed]
- Wu JH, Li HK, Couri DM, et al. Reversal of pulmonary vein remodeling after catheter ablation of atrial fibrillation. J Geriatr Cardiol 2016;13:163-8. [PubMed]
- Almendral J, Barrio-López MT. Pulmonary Vein Stenosis After Ablation: The Difference Between Clinical Symptoms and Imaging Findings, and the Importance of Definitions in This Context. Rev Esp Cardiol (Engl Ed) 2015;68:1056-8. [Crossref] [PubMed]
- Hauser TH, McClennen S, Katsimaglis G, et al. Assessment of left atrial volume by contrast enhanced magnetic resonance angiography. J Cardiovasc Magn Reson 2004;6:491-7. [Crossref] [PubMed]
- Fichtner S, Sparn K, Reents T, et al. Recurrence of paroxysmal atrial fibrillation after pulmonary vein isolation: is repeat pulmonary vein isolation enough? A prospective, randomized trial. Europace 2015;17:1371-5. [Crossref] [PubMed]
- Maceira AM, Cosín-Sales J, Roughton M, et al. Reference left atrial dimensions and volumes by steady state free precession cardiovascular magnetic resonance. J Cardiovasc Magn Reson 2010;12:65. [Crossref] [PubMed]
- Nacif MS, Barranhas AD, Türkbey E, et al. Left atrial volume quantification using cardiac MRI in atrial fibrillation: comparison of the Simpson's method with biplane area-length, ellipse, and three-dimensional methods. Diagn Interv Radiol 2013;19:213-20. [PubMed]
- Lee G, Sparks PB, Morton JB, et al. Low risk of major complications associated with pulmonary vein antral isolation for atrial fibrillation: results of 500 consecutive ablation procedures in patients with low prevalence of structural heart disease from a single center. J Cardiovasc Electrophysiol 2011;22:163-8. [PubMed]
- Tsao HM, Yu WC, Cheng HC, et al. Pulmonary vein dilation in patients with atrial fibrillation: detection by magnetic resonance imaging. J Cardiovasc Electrophysiol 2001;12:809-13. [Crossref] [PubMed]
- Hauser TH, Peters DC, Wylie MV, et al. Relationship of Ostial Pulmonary Vein Scar with Reduction in Pulmonary Vein Size after Radiofrequency Ablation for the Treatment of Atrial Fibrillation: An Observational Cohort Study. J Atr Fibrillation 2013;5:788. [PubMed]
- Hauser TH, Yeon SB, McClennen S, et al. A method for the determination of proximal pulmonary vein size using contrast-enhanced magnetic resonance angiography. J Cardiovasc Magn Reson 2004;6:927-36. [Crossref] [PubMed]
- Calkins H, Ho SY, Angel Cabrera J, et al. Anatomy of the left atrium and pulmonary veins. In: Atrial Fibrillation Ablation: The State of the Art Based on the Venicechart International Consensus. Blackwell Publishing Ltd, 2018: 1-10.
- Mahida S, Sacher F, Derval N, et al. Science Linking Pulmonary Veins and Atrial Fibrillation. Arrhythm Electrophysiol Rev 2015;4:40-3. [Crossref] [PubMed]
- Khan R. Identifying and understanding the role of pulmonary vein activity in atrial fibrillation. Cardiovasc Res 2004;64:387-94. [Crossref] [PubMed]
- Prabhu S, Kalla M, Peck KY, et al. Pulmonary vein activity does not predict the outcome of catheter ablation for persistent atrial fibrillation: A long-term multicenter prospective study. Heart Rhythm 2018;15:980-6. [Crossref] [PubMed]