Vascular computed tomography angiography technique and indications
What is CTA?
Computed tomography angiography (CTA) is a quick, non-invasive method to visualize the vasculature; in most cases with the help of iodinated contrast material (ICM). Modern CT scanners can provide sub-millimeter isotropic three-dimensional (3D) datasets within a single breath-hold during the first pass of intravenous ICM with excellent spatial and temporal resolution. There are varied applications of CTA including various vascular conditions in different vascular territories. However, the basic principles of CTA remain the same i.e., maintaining good contrast opacification in the anatomy of interest during the length of image acquisition. Hence the timing of image acquisition with respect to the contrast injection and the amount of ICM are critical.
Indications
Visualization of anatomy
CTA is often used for visualization of the vascular tree as a part of pre-procedural planning of surgical procedures. CTA is a standard procedure before performing endovascular procedures such as transcatheter aortic valve replacement (TAVR) (Figure 1) and endovascular repair (EVAR) (1). It may also be used prior to high risk-percutaneous coronary interventions (PCI) with potential use of assist device such as Impella and extracorporeal membrane oxygenation (ECMO) also requiring adequate assessment of vascular anatomy (2,3). CTA is also often utilized prior to surgical procedures which require harvesting of vascular stumps such as organ donors, pedicled soft tissue flaps (4). CTA may also be utilized as a scouting procedure to define the anatomy in high-risk surgeries such as coronary artery bypass grafting (CABG) or redo sternotomy (5,6).
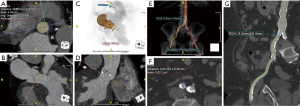
Obstructive atherosclerotic lesions
Atherosclerotic disease is a leading cause of morbidity and mortality in the western world (7). Atherosclerosis starts as a local inflammatory process within the vascular intima and can result in wide range of clinical outcomes such as fixed luminal stenosis, plaque rupture, aneurysm formation and plaque ulceration/penetration/dissection (8) ultimately leading to major cardiovascular events. Fixed luminal stenosis, which is the most common outcome, can limit blood flow in a given territory and can result in symptoms such as claudication, angina, renovascular hypertension and abdominal angina (8-10). Patients with atherosclerosis often require surgical/endovascular procedures and CTA is essential to localize and confirm a hemodynamically significant stenosis (11). CTA also provides a road map to the vascular lesion and helps in choosing a procedure and route of access. Relevant applications include coronary CTA, carotid CTA, renal artery CTA, aortic CTA, peripheral vascular run-off CTA.
Vessel enlargement
Aneurysms are excessive localized enlargement of an artery caused by a weakening or a breach of the artery wall. Aneurysm rupture can lead to catastrophic internal bleeding which can be fatal. Typically, aneurysms often have a size threshold/hinge point, after which the odds of rupture increase and become unacceptable for conservative management and may require surgical or endovascular therapy (12). High spatial resolution of CTA allows accurate measurement of aneurysm size and extent to determine risk of rupture and guide management (surgery vs. EVAR vs. conservative; Figure 2) (13-16). CTA is also used for surveillance if a conservative approach is adopted or an endovascular repair is performed (Figure 2).
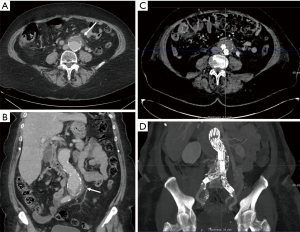
Vascular emergencies
CTA is crucial for management guidance and treatment planning in patients with vascular emergencies that include acute aortic syndromes, vascular trauma, internal bleeding, thrombosis and embolism. The aorta is the most common site of dissection and rapid diagnosis is critical due to high mortality rates (17,18). Aortic dissection results from splitting of the arterial wall layers by the blood entering through an entrance tear (17). Dissection may cause flow limitation in branch vessels and can lead to cardiac, neurological or visceral ischemia. Thus, appropriate detection and surveillance to guide management decisions with high quality vascular imaging is essential (17).
CTA is a diagnostic modality of choice for patients with mesenteric and critical limb ischemia. In suspected acute mesenteric ischemia, CTA provides for rapid and non-invasive assessment of ischemic and alternative diagnoses with high diagnostic accuracy (19). CTA is an excellent modality for detection of internal hemorrhage due various causes such as trauma, diverticulosis, angiodysplasia and spontaneous bleed (Figure 3). For rates of bleeding above 0.25 mL/min GI bleed can be detected by MDCT with a sensitivity of 97% and specificity of 100% (20). CTA of the head and neck, chest, pelvis, and extremities are commonly performed in busy trauma centers. Digital subtraction angiography has been largely replaced by CTA for arterial trauma due to its speed, noninvasive nature, accuracy, and widespread availability. Rapid acquisition of submillimeter isotropic data sets allows for accurate assessment of vascular injury extending from the head and neck to the torso and extremities. CTA is also helpful for the evaluation of deep venous thrombosis, particularly to assess the intracranial veins and the central venous vasculature of chest and abdomen which is not accessible with ultrasound. Pulmonary CTA is a commonly performed test for acute pulmonary arterial embolism. It is highly sensitive for embolism up to the subsegmental level and can also simultaneously assess the lung parenchymal changes and the heart strain (21).
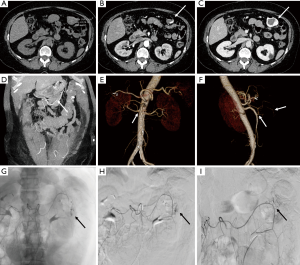
Vessels wall imaging
Plaque imaging/vasculitis/vasculopathy
Plaques at risk of rupture and precipitating myocardial infarction and stroke have certain characteristics that extend beyond simple stenosis severity. These include inflammation, microcalcification, angiogenesis, positive remodeling, a thin fibrous cap, a large necrotic core and plaque hemorrhage. CT plaque characterization has been an area of extensive research especially in coronary and carotid arteries and several CT features have been found to have adverse prognosis (22-24). CTA is not routinely performed primarily for plaque imaging, but plaque features are a useful secondary information which has potential to modify medical therapy (25). CTA is also performed for the assessment of vasculitis and vasculopathy such as fibromuscular dysplasia (15).
Extrinsic pathologies affecting vasculature
Certain vascular structures (most often veins) may be compressed by adjacent anatomic structures or can compress adjacent hollow viscera (arteries). Such compressions are often asymptomatic; however, they can lead to a variety of uncommon syndromes in the abdomen and pelvis such as May-Thurner syndrome, nutcracker syndrome, median arcuate ligament syndrome, superior mesenteric artery syndrome and various forms of ureteral compression (26). Similar syndromes may be encountered in the extremities, examples of which include thoracic outlet syndrome, Paget-Schroetter syndrome and popliteal entrapment. Direct venography or duplex ultrasonography can provide hemodynamic information in cases of vascular compression. However, multidetector CTA is particularly useful for comprehensive evaluation of the anatomy and resultant morphologic changes. Anatomic findings of these syndromes are also often encountered incidentally in patients who are undergoing imaging for unrelated clinical reasons (26).
Miscellaneous
CTA is a commonly performed test for non-coronary cardiac ailments such as cardiac masses, valvular heart disease, infective endocarditis and congenital heart disease. CTA is also performed for the evaluation of vascular device such as, IVC filters, vascular stents and cardiac assist devices.
Technical challenges in performing CTA and solutions
Bolus requirements
CTA mandates high contrast to noise ratio to visualize vessels. This is achieved by rapid injection of contrast material (CM) with high iodine concentration (preferably 350–400 mg/mL) via wide gauge IV access (20 or 18 G) using mechanical injectors and standardized multiphase injection protocols (4). The resultant attenuation in the vessels is a product of the iodine injection rate, injection duration, kVp and the venous return (4). Increasing the injection rate leads to a faster accumulation of contrast in the aorta and higher peak aortic enhancement. With modern scanners, an injection rate of 4–5 mL/s is usually enough for achieving excellent arterial opacification for most vascular studies. Traditional approach to determine injection duration was to match it to scan duration time; however, with modern faster scanners, this may result in inadequate opacification due to a lower total volume of contrast material being delivered. A usual approach for estimating injection duration is to set a minimum of 10 seconds and adding on the estimated scan duration. Power injectors are typically used to ensure a uniform high injection rate of bolus delivery. Use of a saline flush should be routine to help push the tail of the bolus into the central blood volume (27). The saline chaser also helps reduce streak artifact from dense contrast in the central veins, superior vena cava and right atrium which is especially important in coronary and thoracic CTA (28). The injection rate must also be tailored according to body size to match the increasing venous return which is important to achieve diagnostic image quality across different body size.
Time constraints and variance in cardiac output/flow velocity
The physiologic limits of contrast dosage and a high injection rate allow a small bolus length and narrow time window to perform the imaging (4). The time window also varies significantly with physiologic states which further complicates the task of achieving diagnostic images. To maximize the chances of appropriately timing the image acquisition with respect to contrast peak, a fast scanner and a bolus triggering or a timing bolus approach can be used to provide a close prediction of contrast peak in the anatomy of interest (Figure 4). Bolus triggering is usually a preferred method for most applications. While ICM is being injected, serial single slice scans are performed to monitor the contrast arrival in a region of interest (ROI) which is drawn on a vessel. The ROI can be drawn at different locations depending on the vascular territory being imaged: For example, right cardiac chambers for pulmonary CTA, ascending aorta for coronary CTA, aortic arch for neurovascular CTA, abdominal aorta for abdominal and run-off CTA. Acquisition of a delayed phase scan covers up for additional/extreme variations of flow dynamics such as slow filling endoleaks, contrast extravasation and slow filling atrial appendages or cardiac aneurysms. Also, as vasculature is a dynamic system a single acquisition (one time-point snapshot) is often not enough for complete understanding. Hence, a multiphase acquisition (including non-contrast, arterial and delayed phases) is a standard way of performing a comprehensive CTA. As previously mentioned, a short-lasting contrast bolus also mandates fast imaging. A 64-channel CT is a minimum requirement for more advanced CTA applications such as coronary CTA. A 16-channel CT is usually adequate for other less challenging vascular applications such as abdominal aorta or visceral aneurysm assessment. Wide-area detector CT scanners, with up to 320-detector rows, can provide up to 16-cm z-axis coverage in a single gantry rotation which allows for large volume coverage in both helical and axial sequential (step and shoot) acquisition modes. Coverage of large anatomy can also be achieved with ultra-low contrast dose using high-pitch helical mode on dual source scanners (4).
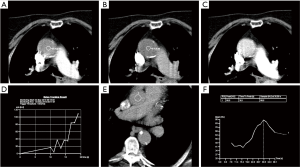
Motion
Image artifacts due to motion are highly detrimental for CT image quality. Hence, the patient must lie still, and imaging should be performed in absence of breathing motion for all body applications. ECG-gating is also essential for coronary/cardiac applications to help time scan acquisition in relatively motion free cardiac phases. Most centers also use beta-blockers to slow down the heart during coronary artery imaging. Dual-source CT scanners provide the maximum temporal resolution available, as the temporal resolution is equal to a quarter of the gantry rotation time; this is as low as 66 milliseconds (ms) in the third-generation scanners (29). Maximizing temporal resolution is advantageous when imaging structures are prone to cardiac motion artifact. Moreover, systolic imaging with fast dual source scanners can obviate the need of beta blockers (30). ECG-gating is also mandatory for aortic root imaging for most scanners (15) (Figure 5), except for dual source scanners operating in high-pitch helical/FLASH mode (15,31) (Figure 6). Due to the motion freezing fast scanning, high pitch helical acquisition can also be used when imaging trauma patients or poor breath-holders who are prone to motion artifacts (15,31).
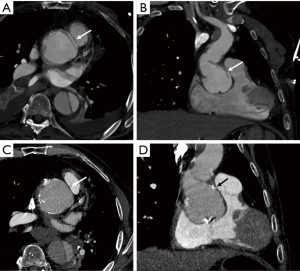
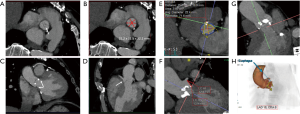
CTA image acquisition and post-processing
CTA protocol
A typical CTA protocol includes 3 phases including non-contrast, arterial phase and a delay. A non-contrast phase is particularly useful in patients with suspected acute aortic syndrome to evaluate for the presence of aortic intramural hematoma and patients with suspected bleed (15,17). Non-contrast images are also useful in differentiating calcifications and surgical graft material from peri-prosthetic contrast enhancement or graft leak. The radiation dose of non-contrast phase should be kept low by using wider collimation and low tube potential with concomitant reduction in tube current (15). The arterial phase imaging is most crucial, and the goal is to achieve a very high contrast-to-noise ratio (CNR) and maximum spatial resolution for visualization of small arterial branches. As previously mentioned, the timing of the arterial phase is very strictly tailored to bolus arrival in the anatomy of interest. The delayed phase imaging allows assessment of slow filling structures and venous circulation. The timing of a delayed scan varies according to the anatomy of interested. In neurovascular applications, delayed images are immediately performed after the completion of arterial phase. In body imaging, a 2 min delay is the standard except for the cardiac imaging (60 sec) and mesenteric vascular imaging (portal venous 90 sec) (4,15). For lower extremities, the delays are generally performed to have a second chance of getting optimum arterial images of infra popliteal vasculature. The timing of contrast arrival in this region can be variable and arterial phase scan can outrun the bolus, so an immediate delay provides an additional opportunity to visualize the vascular anatomy (32). The direction of the delayed scan is not crucial for body applications however the delays in run-off CTA and neurovascular imaging, where to avoid any pause between arterial and delayed phases, the direction of delayed scan is caudocranial and craniocaudal, respectively (which is reverse relative to the arterial scan).
Post processing
CTA is an established method to render high quality multiplanar reconstruction (MPR) and three-dimensional (3D) images from acquired axial CT scans using dedicated computer algorithms (33). MPR and 3D images are often visualized through maximum intensity projection (MIP) reformats which allow for superb visualization of vascular anatomy in any desired plane (Figure 7) (33). MIP images selectively display pixels with the highest attenuation number along the z-axis highlighting hyperdense structures to allow for adequate assessment of vascular pathology (33). For tortuous vascular anatomy, curved planar reformats (CPR) along the course of the target vessel can be generated (34). Bone removal is sometimes necessary to depict 3D anatomy which can be performed by manual segmentation of bone or by performing subtraction of a true non-enhanced image (35). Recently, several automated bone removal methods have been developed with newer generation dual energy CT (DECT) scanners (35). DECT can differentiate between iodine and bone because of varying effective atomic numbers (13.8 and 56 zeff, respectively) at different energies (35).
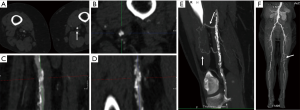
New advances
Most of the new CT technological advances allow fast imaging while minimizing radiation dose and contrast bolus requirements. These advances include low kV imaging, iterative reconstruction technique, high pitch helical imaging and DECT. Fractional flow reserve by CT is a technique to determine hemodynamic significance of luminal narrowing in the vessels. This post-processing technique has recently generated a lot of interest in the field of coronary artery imaging.
Low kV technique
When other scan factors are held constant, low tube potential results in radiation dose reduction (dose α V2). Low kV also results in attenuation gain (proximity with the k edge of iodine) and decreased requirement of ICM. However, low kV also results in excessive image noise which can be countered by using high tube current (mAs upto 1500 with 3rd generation dual source scanners), attenuation gain and simultaneous use of novel iterative reconstruction algorithms. The use of low-kV technique (as low as 70 kVp) has resulted in large radiation dose savings especially in coronary artery imaging (36). Similar applications in pulmonary artery CTA and aortic CTA have also resulted in similar radiation dose reductions (37,38). The application of lower tube potential requires careful adjustment of the tube output (mAs) to maintain the desired CNR, leading to higher mAs demands for larger patients. Hence, the use of low kV scanning should be limited to patients with smaller body size (36).
High-pitch helical (HPH/FLASH) mode
Single-source multidetector-row helical CT scanners are restricted to a pitch of up to 1.5:1. The two simultaneous overlapping helices on dual-source CT enable image reconstruction at a high pitch of over 3:1. The high pitch allows for rapid imaging at significantly lower radiation dose compared to conventional methods (31). High-pitch helical (HPH) acquisition mode with fast table speed and prospective ECG triggering enables coverage of the entire heart within one cardiac cycle. The HPH is particularly advantageous in children or young adults for assessment of coronary artery anomalies and anatomic assessment in patients with congenital heart disease (when information regarding cardiac function is not needed) (36). HPH can also allow motion freezing imaging of the aortic root without ECG gating (15,31,36). In most cases, HPH imaging time is drastically short and can cover the entire body in less than 2 seconds (15,31). This allows performance of CTA with ultra-low contrast volume as length of contrast bolus can be shortened. At our institution, we perform ultra-low dose imaging for pre-TAVR assessment with 20 cc contrast volume (15,39,40). This property can also allow extended anatomical coverage and performance of combined studies with single contrast bolus. At our institute we routinely perform imaging of coronary arteries and the entire aorta with a single extended bolus (6 sec/30 cc longer than standard injection). The coronary artery imaging is performed first and the HPH imaging of the aorta is acquired after a short pause (4–6 sec). HPH can obviate need for sedation or anesthesia in young children due to its rapidity. Several studies on HPH mode for CCTA in pediatric and adult populations have reported good diagnostic quality and consistently sub-millisievert radiation dose (36). Important limitations of HPH mode include inconsistent cardiac gating function in patients with increased or irregular heart rates resulting in motion artifacts and non-applicability of retrospective reconstruction and functional assessment of the heart. This technique is typically avoided in morbidly obese patients with suspected or known extensive atherosclerotic disease, metallic stents/hardware due to higher likelihood of artifacts and excessive image noise (36).
Iterative reconstruction (IR)
The IR techniques have made a major impact on reducing radiation dose in applications in every domain of CT imaging (36,41-43). All modern scanners from different CT vendors offer IR techniques which are replacing conventional filtered back projection (FBP) techniques as they are less prone to increased image noise at lower radiation doses. IR techniques remove image noise through a process of modeling of the imaging acquisition processes including the optics system, photon statistics fluctuations and other aspects of X-ray interactions that are ignored by the conventional FBP techniques. Different IR techniques include a simple algebraic reconstruction technique, statistical iterative reconstruction, and a more recent model-based IR (MBIR) (36). Most advanced IR algorithms combine MBIR with statistical modeling. Multiple studies in different body parts have evaluated the impact of IR techniques on image quality and radiation dose for different clinical indications in both pediatric and adult patients. These studies have found that IR techniques can lower radiation dose by up to 50% in comparison with the traditional FBP methods (36,41-43). For large patients, studies have shown that IR equipped CT scanners enable higher quality diagnostic images compared to FBP scanners without increase in radiation dose (44,45).
DECT
DECT uses data from different tube potentials to generate material specific information (46). Advanced postprocessing with DECT occurs after image acquisition to create virtual monoenergetic (VM) and material density (MD) images. Low-energy VM images (40–55 keV) increase contrast enhancement, intermediate-energy (60–75 keV) images are equivalent to SECT images (120 kVp), and high-energy (95–200 keV) images suppress metal related artifacts (47). MD iodine (MD-I) images, which are independent of inherent tissue attenuation, help differentiate contrast from other high attenuation materials allowing for a more accurate and objective measurement of enhancement compared to HU (48). MD-I images can also suppress artifacts from metals with high atomic number (e.g., platinum embolization coils) (48). Virtual unenhanced (VUE) images can be generated from contrast-enhanced DECT scans which can replace a non-contrast acquisition (Figure 8). Furthermore, DECT automated bone segmentation and calcification removal is superior to SECT in assessment of complex vascular anatomy on neuroimaging (49,50). DECT scanners have greater generator power, better detectors, and allow for more kVp options enabling low-dose scanning and acquisition of diagnostic images. DECT scanners also offer larger gantry size and table weight capacity to permit scanning of patients with large body habitus who are challenging to image on SECT (44,45). All these capabilities of DECT can aid CTA in number of ways.
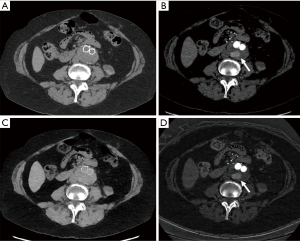
DECT imaging can be used to reduce the number of acquisitions in CTA protocols to reduce radiation exposure (51). As discussed earlier, a triple phase CTA protocol with true unenhanced (TUE), arterial and delayed acquisition are the standard of practice when evaluating for vascular pathologies. With DECT, dual phase CTA protocols (arterial and venous phase) with generation of virtual unenhanced (VUE) images from contrast-enhanced scans have shown comparable diagnostic performance to tri-phasic CTA (15,52-55). VUE serve the same purpose as TUE by depicting high-attenuation hematoma to differentiate extravasation from hemorrhage. In addition to simplifying the CTA protocol, eliminating the need for TUE acquisition allows for an estimated radiation dose reduction between 19–50% (56,57).
DECT material specific iodine and VUE images can also aid in problem solving by providing material specific information. With single energy/polychromatic CT (SECT), measuring attenuation values is necessary to distinguish between hemorrhage, contrast, and low-attenuation fluid (58). However, dilute contrast agent and blood can have similar attenuation values which may hinder accurate diagnosis. Additionally, suboptimal contrast enhancement and presence of image artifacts can decrease diagnostic confidence and lead to repeat exams with unnecessary exposure to additional ICM and radiation (59,60). Thus, DECT scanners overcome limitations of SECT with advanced post-processing capabilities for problem-solving vascular pathology in a more functional and quantitative method (47).
Patients with vascular disease often have several comorbidities (diabetes, hypertension) which can impair kidney function putting them at high risk for developing adverse effects from contrast agents (61-63). Since ICM use is integral for vascular imaging, there is continued incentive to decrease iodinated contrast dose for patient safety (62). Obtaining adequate image quality with lower ICM dose is challenging on SECT; particularly in larger patients. However, DECT low keV images allow for optimal contrast visualization while maintaining diagnostic image quality and enabling ICM dose reduction (62,64,65). Mean iodine dose as low as 15 g has been shown to be diagnostic for CTA protocols with DECT when compared to standard iodine dose for SECT (30–37 g) (62). Low-energy VM images demonstrate up to higher attenuation and contrast-to-noise ratio (185% and 25%, respectively) compared to standard iodine dose (33.3 g I) on SECT (64). Therefore, DECT applications will be able to compensate for inadequate contrast-enhancement in instances of improper bolus timing or due to extravasation and other patient related factors (62).
With all the advantages detailed above, DECT suffers from few limitations including higher scanner cost, and higher number of post processed images which can impact work-flow. Meticulous technologist training and involvement is critical to efficiently incorporate DECT into the clinical workflow on a regular basis (66). Recent versions of DECT platforms and software upgrades have the capability to automate post-processing such that DECT image reconstructions do not hinder workflow (67). Recent studies have shown that single source rapid switching rsDECT suffers from increased beam hardening/streak artifacts on material specific images and patient anatomy can extend outside dual energy field of view (FOV) on dual source scanners (68-70). However, newer detector-based spectral CT scanners have no limitations on FOV, gantry rotation time or cross-scatter (67).
CT fractional flow reserve (CT-FFR or FFR-CT)
Determining degree of vessel stenosis is important to determine risk of end organ ischemia. Thus, hemodynamic significance of a stenosis in a vessel guides management especially in coronary artery disease given the risk associated with invasive treatments. FFR-CT algorithms focus on the noninvasive derivation of flow dynamics through a diseased artery (typically coronaries) by employing mathematical modelling and computational fluid dynamics (71). The preliminary data suggest that the use of FFR-CT for rationalizing therapy decisions improves patient outcomes and quality of life with reduced health care cost (71). Conceptually, FFR CT can be applied to vessels other than coronaries but such applications are unknown.
Summary and conclusion
CTA is a robust tool to assess anatomy and pathology of the vascular tree with a submillimeter spatial resolution and high temporal resolution. Robust imaging protocols with rapid image acquisition and accurate bolus timing techniques are of utmost importance for high image quality. Low kV technique, iterative reconstructions, HPH imaging and dual energy CT are novel methods for radiation dose reduction. Low kV technique, DECT and HPH imaging can also allow contrast bolus requirement reduction in appropriate clinical setting. As HPH imaging is very less susceptible to motion, it can mitigate the requirements of ECG gating for aortic root imaging and requirement of anesthesia in poorly cooperative adult and pediatric patients.
Acknowledgments
None.
Footnote
Conflicts of Interest: The authors have no conflicts of interest to declare.
Ethical Statement: The authors are accountable for all aspects of the work in ensuring that questions related to the accuracy or integrity of any part of the work are appropriately investigated and resolved.
References
- Schoenhagen P, Hausleiter J, Achenbach S, et al. Computed tomography in the evaluation for transcatheter aortic valve implantation (TAVI). Cardiovasc Diagn Ther 2011;1:44-56. [PubMed]
- Nalluri N, Patel N, Saouma S, et al. Utilization of the Impella for hemodynamic support during percutaneous intervention and cardiogenic shock: an insight. Expert Rev Med Devices 2017;14:789-804. [Crossref] [PubMed]
- Sandhu A, McCoy LA, Negi SI, et al. Use of Mechanical Circulatory Support in Patients Undergoing Percutaneous Coronary Intervention. Circulation 2015;132:1243-51. [Crossref] [PubMed]
- Murphy DJ, Aghayev A, Steigner ML. Vascular CT and MRI: a practical guide to imaging protocols. Insights Imaging 2018;9:215-36. [Crossref] [PubMed]
- Khan NU, Yonan N. Does preoperative computed tomography reduce the risks associated with re-do cardiac surgery? Interact Cardiovasc Thorac Surg 2009;9:119-23. [Crossref] [PubMed]
- Lapar DJ, Ailawadi G, Irvine JN, et al. Preoperative computed tomography is associated with lower risk of perioperative stroke in reoperative cardiac surgery. Interact Cardiovasc Thorac Surg 2011;12:919-23. [Crossref] [PubMed]
- Roth GA, Johnson C, Abajobir A, et al. Global, Regional, and National Burden of Cardiovascular Diseases for 10 Causes, 1990 to 2015. J Am Coll Cardiol 2017;70:1-25. [Crossref] [PubMed]
- Rafieian-Kopaei M, Setorki M, Doudi M, et al. Atherosclerosis: Process, Indicators, Risk Factors and New Hopes. Int J Prev Med 2014;5:927-46. [PubMed]
- Valizadeh GA, Zareie S, Manafi A, et al. Stenosis level, plaque morphology and intima-media thickness of internal carotid artery in chronic stable angina and acute coronary syndrome; a comparative study. Iran Red Crescent Med J 2015;17:e10162. [Crossref] [PubMed]
- Lusis AJ. Atherosclerosis. Nature 2000;407:233-41. [Crossref] [PubMed]
- Kolodgie FD, Nakazawa G, Sangiorgi G, et al. Pathology of Atherosclerosis and Stenting. Neuroimaging Clin N Am 2007;17:285-301. vii. [Crossref] [PubMed]
- Elefteriades JA. Natural history of thoracic aortic aneurysms: indications for surgery, and surgical versus nonsurgical risks. Ann Thorac Surg 2002;74:S1877-80; discussion S1892-8.
- Johnson PT, Fishman EK. CT Angiography of Coronary Artery Aneurysms: Detection, Definition, Causes, and Treatment. AJR Am J Roentgenol 2010;195:928-34. [Crossref] [PubMed]
- Agarwal PP, Chughtai A, Matzinger FRK, et al. Multidetector CT of Thoracic Aortic Aneurysms. RadioGraphics 2009;29:537-52. [Crossref] [PubMed]
- Baliyan V, Verdini D, Meyersohn NM. Noninvasive aortic imaging. Cardiovasc Diagn Ther 2018;8:S3-18. [Crossref] [PubMed]
- Tomandl BF, Köstner NC, Schempershofe M, et al. CT Angiography of Intracranial Aneurysms: A Focus on Postprocessing. RadioGraphics 2004;24:637-55. [Crossref] [PubMed]
- Baliyan V, Parakh A, Prabhakar AM, et al. Acute aortic syndromes and aortic emergencies. Cardiovasc Diagn Ther 2018;8:S82-96. [Crossref] [PubMed]
- Howard DPJ, Sideso E, Handa A, et al. Incidence, risk factors, outcome and projected future burden of acute aortic dissection. Ann Cardiothorac Surg 2014;3:278-84. [PubMed]
- Henes FO, Pickhardt PJ, Herzyk A, et al. CT angiography in the setting of suspected acute mesenteric ischemia: prevalence of ischemic and alternative diagnoses. Abdom Radiol (NY) 2017;42:1152-61. [Crossref] [PubMed]
- Wortman JR, Landman W, Fulwadhva UP, et al. CT angiography for acute gastrointestinal bleeding: what the radiologist needs to know. Br J Radiol 2017;90:20170076. [Crossref] [PubMed]
- Moore AJE, Wachsmann J, Chamarthy MR, et al. Imaging of acute pulmonary embolism: an update. Cardiovasc Diagn Ther 2018;8:225-43. [Crossref] [PubMed]
- Puchner SB, Liu T, Mayrhofer T, et al. High-risk plaque detected on coronary CT angiography predicts acute coronary syndromes independent of significant stenosis in acute chest pain: results from the ROMICAT-II trial. J Am Coll Cardiol 2014;64:684-92. [Crossref] [PubMed]
- Motoyama S, Ito H, Sarai M, et al. Plaque Characterization by Coronary Computed Tomography Angiography and the Likelihood of Acute Coronary Events in Mid-Term Follow-Up. J Am Coll Cardiol 2015;66:337-46. [Crossref] [PubMed]
- Dweck MR, Fayad ZA. Multi-target Vulnerable Plaque Imaging. Circ Cardiovasc Imaging 2017;10:e006491. [Crossref] [PubMed]
- Cury RC, Abbara S, Achenbach S, et al. CAD-RADS(TM) Coronary Artery Disease - Reporting and Data System. An expert consensus document of the Society of Cardiovascular Computed Tomography (SCCT), the American College of Radiology (ACR) and the North American Society for Cardiovascular Imaging (NASCI). Endorsed by the American College of Cardiology. J Cardiovasc Comput Tomogr 2016;10:269-81. [Crossref] [PubMed]
- Lamba R, Tanner DT, Sekhon S, et al. Multidetector CT of Vascular Compression Syndromes in the Abdomen and Pelvis. RadioGraphics 2014;34:93-115. [Crossref] [PubMed]
- Schoellnast H, Tillich M, Deutschmann HA, et al. Improvement of parenchymal and vascular enhancement using saline flush and power injection for multiple-detector-row abdominal CT. Eur Radiol 2004;14:659-64. [Crossref] [PubMed]
- Haage P, Schmitz-Rode T, Hübner D, et al. Reduction of contrast material dose and artifacts by a saline flush using a double power injector in helical CT of the thorax. AJR Am J Roentgenol 2000;174:1049-53. [Crossref] [PubMed]
- Flohr TG, Schmidt B. CT technology for imaging the thorax: State of the art. In: Schoepf U, Meinel F, editors. Multidetector-Row CT of the Thorax. 2nd ed. Springer Nature, 2016:12-15.
- Meyersohn NM, Szilveszter B, Staziaki PV, et al. Coronary CT angiography in the emergency department utilizing second and third generation dual source CT. J Cardiovasc Comput Tomogr 2017;11:249-57. [Crossref] [PubMed]
- Beeres M, Wichmann JL, Frellesen C, et al. ECG-gated Versus Non-ECG-gated High-pitch Dual-source CT for Whole Body CT Angiography (CTA). Acad Radiol 2016;23:163-7. [Crossref] [PubMed]
- Fleischmann D, Hallett RL, Rubin GD. CT Angiography of Peripheral Arterial Disease. J Vasc Interv Radiol 2006;17:3-26. [Crossref] [PubMed]
- Fishman EK, Ney DR, Heath DG, et al. Volume rendering versus maximum intensity projection in CT angiography: what works best, when, and why. Radiographics 2006;26:905-22. [Crossref] [PubMed]
- Ochi T, Shimizu K, Yasuhara Y, et al. Curved planar reformatted CT angiography: usefulness for the evaluation of aneurysms at the carotid siphon. AJNR Am J Neuroradiol 1999;20:1025-30. [PubMed]
- van Straten M, Schaap M, Dijkshoorn ML, et al. Automated bone removal in CT angiography: comparison of methods based on single energy and dual energy scans. Med Phys 2011;38:6128-37. [Crossref] [PubMed]
- Hedgire SS, Baliyan V, Ghoshhajra BB, et al. Recent advances in cardiac computed tomography dose reduction strategies: a review of scientific evidence and technical developments. J Med Imaging (Bellingham) 2017;4:031211. [Crossref] [PubMed]
- Gill MK, Vijayananthan A, Kumar G, et al. Use of 100 kV versus 120 kV in computed tomography pulmonary angiography in the detection of pulmonary embolism: effect on radiation dose and image quality. Quant Imaging Med Surg 2015;5:524-33. [PubMed]
- Ippolito D, Talei Franzesi C, Fior D, et al. Low kV settings CT angiography (CTA) with low dose contrast medium volume protocol in the assessment of thoracic and abdominal aorta disease: a feasibility study. Br J Radiol 2015;88:20140140. [Crossref] [PubMed]
- Azzalini L, Abbara S, Ghoshhajra BB. Ultra-low contrast computed tomographic angiography (CTA) with 20-mL total dose for transcatheter aortic valve implantation (TAVI) planning. J Comput Assist Tomogr 2014;38:105-9. [Crossref] [PubMed]
- Pulerwitz TC, Khalique OK, Nazif TN, et al. Very Low Intravenous Contrast Volume Protocol for Computed Tomography Angiography Providing Comprehensive Cardiac and Vascular Assessment Prior to Transcatheter Aortic Valve Replacement in Patients with Chronic Kidney Disease. J Cardiovasc Comput Tomogr 2016;10:316-21. [Crossref] [PubMed]
- Padole A, Ali Khawaja RD, Kalra MK, et al. CT Radiation Dose and Iterative Reconstruction Techniques. AJR Am J Roentgenol 2015;204:W384-92. [Crossref] [PubMed]
- Singh S, Kalra MK, Shenoy-Bhangle AS, et al. Radiation Dose Reduction with Hybrid Iterative Reconstruction for Pediatric CT. Radiology 2012;263:537-46. [Crossref] [PubMed]
- Shah A, Rees M, Kar E, et al. Adaptive statistical iterative reconstruction use for radiation dose reduction in pediatric lower-extremity CT: impact on diagnostic image quality. Skeletal Radiol 2018;47:785-93. [Crossref] [PubMed]
- Desai GS, Uppot RN, Yu EW, et al. Impact of iterative reconstruction on image quality and radiation dose in multidetector CT of large body size adults. Eur Radiol 2012;22:1631-40. [Crossref] [PubMed]
- Shaqdan KW, Kambadakone AR, Hahn P, et al. Experience With Iterative Reconstruction Techniques for Abdominopelvic Computed Tomography in Morbidly and Super Obese Patients. J Comput Assist Tomogr 2018;42:124-32. [Crossref] [PubMed]
- Parakh A, Baliyan V, Sahani DV. Dual-Energy CT in Focal and Diffuse Liver Disease. Curr Radiol Rep 2017;5:35. [Crossref]
- Shaqdan KW, Parakh A, Kambadakone AR, et al. Role of dual energy CT to improve diagnosis of non-traumatic abdominal vascular emergencies. Abdom Radiol (NY) 2019;44:406-21. [Crossref] [PubMed]
- Patino M, Prochowski A, Agrawal MD, et al. Material Separation Using Dual-Energy CT: Current and Emerging Applications. Radiographics 2016;36:1087-105. [Crossref] [PubMed]
- Schulz B, Kuehling K, Kromen W, et al. Automatic bone removal technique in whole-body dual-energy CT angiography: performance and image quality. AJR Am J Roentgenol 2012;199:W646-50. [Crossref] [PubMed]
- Naruto N, Tannai H, Nishikawa K, et al. Dual-energy bone removal computed tomography (BRCT): preliminary report of efficacy of acute intracranial hemorrhage detection. Emerg Radiol 2018;25:29-33. [Crossref] [PubMed]
- Megibow AJ, Sahani D. Best practice: implementation and use of abdominal dual-energy CT in routine patient care. AJR Am J Roentgenol 2012;199:S71-7. [Crossref] [PubMed]
- Chandarana H, Godoy MCB, Vlahos I, et al. Abdominal aorta: evaluation with dual-source dual-energy multidetector CT after endovascular repair of aneurysms--initial observations. Radiology 2008;249:692-700. [Crossref] [PubMed]
- Buffa V, Solazzo A, D’Auria V, et al. Dual-source dual-energy CT: dose reduction after endovascular abdominal aortic aneurysm repair. Radiol Med (Torino) 2014;119:934-41. [Crossref] [PubMed]
- Flors L, Leiva-Salinas C, Norton PT, et al. Endoleak detection after endovascular repair of thoracic aortic aneurysm using dual-source dual-energy CT: suitable scanning protocols and potential radiation dose reduction. AJR Am J Roentgenol 2013;200:451-60. [Crossref] [PubMed]
- Stolzmann P, Frauenfelder T, Pfammatter T, et al. Endoleaks after endovascular abdominal aortic aneurysm repair: detection with dual-energy dual-source CT. Radiology 2008;249:682-91. [Crossref] [PubMed]
- Ho LM, Yoshizumi TT, Hurwitz LM, et al. Dual energy versus single energy MDCT: measurement of radiation dose using adult abdominal imaging protocols. Acad Radiol 2009;16:1400-7. [Crossref] [PubMed]
- De Cecco CN, Darnell A, Macías N, et al. Second-generation dual-energy computed tomography of the abdomen: radiation dose comparison with 64- and 128-row single-energy acquisition. J Comput Assist Tomogr 2013;37:543-6. [Crossref] [PubMed]
- Furlan A, Fakhran S, Federle MP. Spontaneous abdominal hemorrhage: causes, CT findings, and clinical implications. AJR Am J Roentgenol 2009;193:1077-87. [Crossref] [PubMed]
- Barrett JF, Keat N. Artifacts in CT: recognition and avoidance. Radiographics 2004;24:1679-91. [Crossref] [PubMed]
- Schindera ST, Tock I, Marin D, et al. Effect of beam hardening on arterial enhancement in thoracoabdominal CT angiography with increasing patient size: an in vitro and in vivo study. Radiology 2010;256:528-35. [Crossref] [PubMed]
- Andreucci M, Solomon R, Tasanarong A. Side effects of radiographic contrast media: pathogenesis, risk factors, and prevention. BioMed Res Int 2014;2014:741018. [PubMed]
- Shuman WP, O’Malley RB, Busey JM, et al. Prospective comparison of dual-energy CT aortography using 70% reduced iodine dose versus single-energy CT aortography using standard iodine dose in the same patient. Abdom Radiol (NY) 2017;42:759-65. [Crossref] [PubMed]
- Nicola R, Shaqdan KW, Aran K, et al. Contrast-Induced Nephropathy: Identifying the Risks, Choosing the Right Agent, and Reviewing Effective Prevention and Management Methods. Curr Probl Diagn Radiol 2015;44:501-4. [Crossref] [PubMed]
- Agrawal MD, Oliveira GR, Kalva SP, et al. Prospective Comparison of Reduced-Iodine-Dose Virtual Monochromatic Imaging Dataset From Dual-Energy CT Angiography With Standard-Iodine-Dose Single-Energy CT Angiography for Abdominal Aortic Aneurysm. AJR Am J Roentgenol 2016;207:W125-32. [Crossref] [PubMed]
- Xin L, Yang X, Huang N, et al. The initial experience of the upper abdominal CT angiography using low-concentration contrast medium on dual energy spectral CT. Abdom Imaging 2015;40:2894-9. [Crossref] [PubMed]
- Aran S, Shaqdan KW, Abujudeh HH. Dual-energy computed tomography (DECT) in emergency radiology: basic principles, techniques, and limitations. Emerg Radiol 2014;21:391-405. [Crossref] [PubMed]
- Rassouli N, Etesami M, Dhanantwari A, et al. Detector-based spectral CT with a novel dual-layer technology: principles and applications. Insights Imaging 2017;8:589-98. [Crossref] [PubMed]
- Baliyan V, Kordbacheh H, Serrao J, et al. Dual-Source Dual-Energy CT Portal Venous Phase Abdominal CT Scans in Large Body Habitus Patients: Preliminary Observations on Image Quality and Material Decomposition. J Comput Assist Tomogr 2018;42:932. [Crossref] [PubMed]
- Kordbacheh H, Baliyan V, Uppot RN, et al. Dual-Source Dual-Energy CT in Detection and Characterization of Urinary Stones in Patients With Large Body Habitus: Observations in a Large Cohort. AJR Am J Roentgenol 2019;212:796-801. [Crossref] [PubMed]
- Kordbacheh H, Baliyan V, Singh P, et al. Rapid kVp switching dual-energy CT in the assessment of urolithiasis in patients with large body habitus: preliminary observations on image quality and stone characterization. Abdom Radiol (NY) 2019;44:1019-26. [Crossref] [PubMed]
- Tesche C, De Cecco CN, Albrecht MH, et al. Coronary CT Angiography-derived Fractional Flow Reserve. Radiology 2017;285:17-33. [Crossref] [PubMed]