Effect of carvedilol on atrial remodeling in canine model of atrial fibrillation
Introduction
Atrial interstitial fibrosis plays an important role in the formation of the arrhythmogenic substrate for atrial fibrillation (AF). Because atrial interstitial fibrosis, fibroblastic degeneration, and extracellular matrix (ECM) synthesis destroy the electrophysiological connections between atrial myocytes, these processes can cause conduction disturbance. Interstitial changes, (i.e., ECM synthesis), can be caused by various stimuli such as inflammatory cytokines, angiotensin-II, oxidative stress, and sympathetic nerve activation.
Carvedilol is known as nonselective beta-blockade with anti-oxidative action, which is widely used in clinical practice especially for the patients with structural heart disease. Carvedilol’s antiarrhythmic properties in patients with heart failure have been demonstrated in the Carvedilol Post-Infarct Survival Control in Left Ventricular Dysfunction (CAPRICORN) (1) and Carvedilol Prospective Randomized Cumulative Survival (COPERNICUS) (2) trials. In a retrospective study in 115 patients who had undergone coronary artery bypass grafting surgery and/or valvular surgery, early postoperative administration of carvedilol resulted in a significantly lower incidence of postoperative AF than metoprolol or atenolol (3), suggesting a greater benefits of use of carvedilol than the other β blockers. However its precise mechanism and the effect of carvedilol against the primary AF remain unclear.
In the present study, we evaluated the effect of carvedilol against the AF inducibility, the development of atrial structural remodeling and the oxidative stress markers in a canine AF model.
Methods
Initial surgery
AF model was created as previously described (4). In brief, Fifteen adult female beagle dogs [12.6 (±1.2) kg] were anesthetized with pentobarbital (25 mg/kg IV). Mechanical ventilation was maintained through an endotracheal tube with a mechanical ventilator (Model SN-480-5; Shinano Manufacturing, Tokyo, Japan) with 100% oxygen. Two pairs of electrodes were sutured at the right atrial free wall for the monitoring of the atrial electrograms. The other ends of the electrode wires were tunneled subcutaneously and exposed at the back of the neck. For continuous rapid atrial pacing, a unipolar screw-in lead (CapSureFix 5568; Medtronic Inc. Minneapolis, MN, USA) was inserted through the right external jugular vein, and the distal end of the lead was screwed into the endocardial side of the right atrial appendage. The proximal end of the pacing lead was connected to a rapid pulse generator (Soletra, Medtronic Inc. Minneapolis, MN, USA), which was implanted into a subcutaneous pocket at the back of the neck. Atrioventricular block was not produced in this study to mimic the hemodynamic situation of clinical cases of AF (5-10).
All studies were performed in accordance with the guidelines specified by the Animal Experimentation and Ethics Committee of the Kitasato University School of Medicine.
Study protocol
To obtain stable baseline conditions, each dog was allowed to recover after the initial surgical procedure for at least one week without pacing. Atrial rapid pacing [400 beats per minute (bpm)] was performed in 10 of the 15 dogs, and the remaining five dogs without pacing were assigned into the non-pacing group. We divided the ten dogs with atrial rapid pacing into two groups: the pacing control group (n=5), i.e., dogs without any oral administration, and the pacing + carvedilol group (n=5), i.e., dogs with oral administration of carvedilol (50 mg/day) starting two weeks before the initiation of rapid pacing (Figure 1).
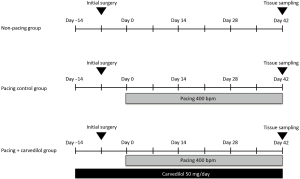
Electrophysiological studies
Electrophysiological studies were performed every week to evaluate the AF inducibility and atrial effective refractory period (AERP). To evaluate AF inducibility, the incidence of AF induction was evaluated with atrial burst pacing for 3 sec at the minimal pacing cycle length that achieved 1:1 atrial capture at right atrial pacing site. This pacing was delivered at 4-fold the diastolic threshold with a pulse width of 2 ms. When AF was induced, its duration was measured. We defined AF as a spontaneous irregular atrial rhythm lasting longer than 5 sec. The atrial burst pacing for AF induction was delivered five times at right atrial pacing site. At each evaluation time point, the AERP was measured with basic drive cycle lengths of 300 ms at the left atrial sites where the electrodes were sutured. The pacing energy output was set at twice the diastolic threshold during each evaluation at right atrial pacing site. The coupling interval of the premature stimulus was shortened by 2-ms steps. The longest coupling interval of the premature beat that failed to capture the atrium was determined as the local AERP.
Hemodynamic evaluation
To exclude the hemodynamic difference caused by carvedilol administration, the hemodynamic parameters, including systemic blood pressure, pulmonary arterial pressure, pulmonary arterial wedge pressure, and cardiac output, were evaluated using a thermo-dilution catheter in the pacing control and pacing + carvedilol groups at the end of the 6-week protocol.
Histology of the atrial tissue
At the end of the protocol, small portions of the right and left atrial free walls were excised for histological and biochemical analyses. The histology of the atrial tissue was evaluated by hematoxylin-eosin (HE) and Azan staining.
Immunostaining with 8-hydroxy-2'-deoxyguanosine
The cardiac tissues were fixed in Bouin’s solution for seven days. Paraffin sections, 3 μm in thickness, were mounted on silane-coated slides and deparaffinized with xylene followed by a stepwise change of ethyl alcohol. The tissue sections were then exposed to 1% hydrogen peroxide/methanol to block endogenous peroxidase activity, and treated in a pre-heated steamer with a staining dish containing Tris-EDTA buffer (10 mM Tris base, 1 mM EDTA solution, 0.05% Tween 20, pH 9.0) until the temperature reaches 121 °C. The processed sections were incubated with primary antibodies [anti-8-hydroxy-2'-deoxyguanosine (8-OHdG)-monoclonal mouse antibody; Japan Institute for the Control of Aging, NIKKEN SEIL CO., Ltd., Japan] and subsequently, with a secondary antibody (anti-mouse IgG antibody, EnVision + Labelled Polymer-HRP; DAKO). The sections were incubated liquid DAB (Liquid DAB + Substrate Chromogen System, DAKO). The stained sections were then observed with a microscope, (Olympus DP70; Olympus, Japan). The percentage of nuclei that stained positive for 8-OHdG is shown in the graph in Figure 2.
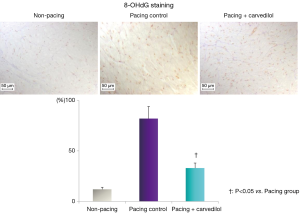
Histological analysis and in situ localization of reactive oxygen species
For in situ localization of reactive oxygen species (ROS), we incubated frozen sections (25 μm) of left atrial tissues with fluorophores sensitive to O2−, dihydroethidium (DHE, 10 µmol/L; Sigma), or hydrogen peroxide (H2O2), 5-(and 6)-chloromethyl-2,7-dichlorodihydrofluorescein diacetate, acetyl ester (DCF-DA, 10 µmol/L; Molecular Probes). DHE specifically reacts with intracellular O2− and is converted to the red fluorescent compound ethidium, which then binds irreversibly to double-stranded DNA and appears as punctuate nuclear staining. The specificity of DHE and DCF signals for O2− and H2O2 detection was confirmed by preincubation with polyethylene glycol-superoxide dismutase (PEG-SOD; 500 U/mL, Sigma) and PEG-catalase (350 U/mL, Sigma), respectively. The stained sections were then observed with a fluorescence microscope (LSM710; Carl Zeiss MicroImaging, USA).
Statistical analysis
All statistical analyses were performed using the JMP statistical software (SAS Institute Inc., Cary, NC, USA). The values are presented as mean (standard deviation). The basic comparative statistics were analyzed with a non-parametric test (Friedman test), or Mann-Whitney U test. A P-value of <0.05 was considered to indicate statistical significance.
Results
Electrophysiological studies
Figure 3 shows the time course of the results of the electrophysiologic studies. The AF inducibility was gradually increased along the time course in the pacing control group. In the pacing + carvedilol group, the AF inducibility tended to be increased along the time course in the earlier phase, but it was rather suppressed in the later phase compared to its inducibility in the pacing control group. The AERP shortening (negative ΔAERP) appeared from a relatively earlier phase in the pacing control group. The degree of the AERP shortening was relatively smaller in the pacing + carvedilol group than in the pacing control group, and the difference between the two groups was significant at day 35.
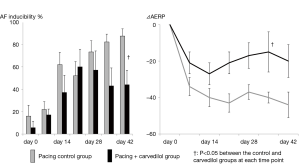
Hemodynamic parameters
Table 1 shows the hemodynamic parameters evaluated at the end of the 6-week protocol by using thermo-dilution catheter in the pacing control and pacing + carvedilol groups. There were no significant differences between the two groups in these parameters.
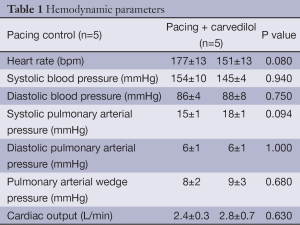
Full table
Histopathology
HE staining showed interstitial proliferation, disalignment, and size-irregularity of the muscle fibers in the pacing control group compared to its characteristics in the non-pacing group. Also on Azan staining, intercellular fibrosis increased to a greater extent in the pacing control group than the non-pacing group. In contrast, these histological changes were almost entirely suppressed in the pacing + carvedilol group (Figure 4).
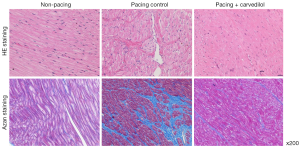
Expression of oxidative stress markers
In 8-OHdG staining, enhanced oxidative stress was observed in the atrial tissue in the pacing control, but not in the non-pacing and pacing + carvedilol groups. In DHE and DCF-DA staining, enhanced oxidative stress was observed in the atrial tissue in the pacing control, but not in the non-pacing and pacing + carvedilol groups (Figures 2,5).
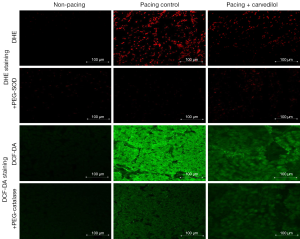
Discussion
In the present study, we have demonstrated several interesting findings. The 6-week rapid atrial pacing caused ECM synthesis in the atrial tissue as well as interstitial fibrosis in our canine AF model. And carvedilol, a beta-blockade with antioxidative action, suppressed this up-regulation of oxidative stress and atrial ECM remodeling and AF inducibility in our canine AF model.
Role of hyper-oxidative state in the atrial remodeling of AF
In previous publications, atrial interstitial fibrosis has been considered to be the mechanism for constructing the AF arrhythmogenic substrate because atrial fibrosis may destroy cell-to-cell electrophysiological coupling (11). As a result, the conduction velocity in the atrial tissue would be decreased and then the AF inducibility would be increased because of the shortening of the wavelength (12). In the present study, we have documented the enhanced expression of intercellular fibrosis in the pacing control group than the non-pacing group. And these histological changes were almost entirely suppressed in the pacing + carvedilol group.
Such ECM synthesis will be induced by various stimulations. In a condition of pressure and volume overload, progressive changes in myocardial structure and function may occur, which are referred to as myocardial remodeling. The oxidative stress is considered to play an important role in the process of construction of the myocardial remodeling. The mitochondrion has been recognized as a major source of ROS owing to electron leakage from the respiratory chain to oxygen, forming superoxide. In normal conditions, ROS plays a role as a second messenger for regulating cell proliferation, differentiation, and apoptosis. However in a condition with excessive ROS, a hyper-oxidative state will arise in which the balance between ROS production and oxidative stress removal collapses. ROS contributes to DNA damage by producing degenerative and deactivate changes in the lipid and protein. Mihm et al. reported the presence of oxidative injury in the right atrial appendage tissue of patients with AF (13). Kim et al. reported that a myocardial NAD(P)H oxidase and, to a lesser extent, dysfunctional NO synthases contribute to superoxide production in fibrillating human atrial myocardium, and suggested a possible role for atrial oxidative injury as an electrical remodeling process in patients with AF (14). These reports suggest a strong relation between ROS and the production of AF substrate. In the present study, carvedilol exhibited an obvious suppressive effect against atrial hyper-oxidative state.
Effect of carvedilol on ECM synthesis and the arrhythmogenic substrate for AF
In the present study, carvedilol suppressed the expression of hyper-oxidative state in the atrial tissue and partly suppressed the increase in AF inducibility in the canine AF model. To the best of our knowledge, this is the first report to show the effect of carvedilol on AF inducibility and oxidative stress in atrial remodeling.
In AF, various tissue-stimulating factors such as ROS, transforming growth factor beta, angiotensin II, and catecholamines are activated through the action of a stretch receptor, baroreceptor, or adrenergic receptor. It may produce various tissue responses, including intercellular fibrosis, which may result in atrial structural remodeling as the promotion of the arrhythmogenic substrate for AF. In the present study, the pacing control and the pacing + carvedilol groups did not show any differences in hemodynamic parameters. Although we have to pay attention to possible participation of relatively lower heart rate in pacing + carvedilol group because such “rate control” effect may result in lower atrial pressure and reduction in atrial wall stretch, the difference in the heart rate was not significant in this study, so its participation should be limited. In contrast, carvedilol exhibited an obvious suppressive effect against atrial hyper-oxidative state, so that the anti-oxidative effect of carvedilol was considered to play an important role against the arrhythmogenic substrate for AF.
Clinical implications
Because carvedilol was clearly effective for the prevention or suppression of the progression of atrial remodeling in the canine AF model induced by rapid right atrial stimulation, carvedilol could be expected to have a beneficial effect in the suppression of AF even in clinical cases. The sub-analysis of SCD-Heft (Sudden Cardiac Death in Heart Failure Trial) showed that beta-blockades suppressed the new onset AF in patients with congestive heart failure. In contrast, recent clinical trials, which set the AF suppression as the primary end-point, failed to document the beneficial effect on the suppression of lone AF (15,16). The study which evaluates limited for carvedilol will be needed to clarify this discrepancy.
Study limitations
This study has several limitations. Because His-bundle ablation was not performed in this model, progression to tachycardia-induced heart failure cannot be ruled out. We confirmed that the influence of this potential progression was small, however, as there were no significant changes in hemodynamic parameters, and we consider that this model is suitable for evaluating the possible clinical effects of drugs as it closely mimics clinical AF.
The hemodynamic parameters were evaluated using a thermo-dilution catheter only at the end of the 6-week protocol, not every week. The anesthesia with pentobarbital and mechanical ventilation are necessary for this protocol, and these procedures may occurs the oxidative stress, which could influence the evaluation of the AF inducibility and oxidative stress markers.
These limitations should be solved in future studies with different design protocols.
Conclusions
Carvedilol suppressed AF inducibility and oxidative stress in this canine AF model. The effect of anti-oxidative action of carvedilol could have played an important role against this phenomenon.
Acknowledgements
Disclosure: The authors declare no conflict of interest.
References
- McMurray J, Køber L, Robertson M, et al. Antiarrhythmic effect of carvedilol after acute myocardial infarction: results of the Carvedilol Post-Infarct Survival Control in Left Ventricular Dysfunction (CAPRICORN) trial. J Am Coll Cardiol 2005;45:525-30. [PubMed]
- Packer M, Fowler MB, Roecker EB, et al. Effect of carvedilol on the morbidity of patients with severe chronic heart failure: results of the carvedilol prospective randomized cumulative survival (COPERNICUS) study. Circulation 2002;106:2194-9. [PubMed]
- Merritt JC, Niebauer M, Tarakji K, et al. Comparison of effectiveness of carvedilol versus metoprolol or atenolol for atrial fibrillation appearing after coronary artery bypass grafting or cardiac valve operation. Am J Cardiol 2003;92:735-6. [PubMed]
- Kiryu M, Niwano S, Niwano H, et al. Angiotensin II-mediated up-regulation of connective tissue growth factor promotes atrial tissue fibrosis in the canine atrial fibrillation model. Europace 2012;14:1206-14. [PubMed]
- Niwano S, Kojima J, Fukaya H, et al. Arrhythmogenic difference between the left and right atria during rapid atrial activation in a canine model of atrial fibrillation. Circ J 2007;71:1629-35. [PubMed]
- Niwano S, Ortiz J, Abe H, et al. Characterization of the excitable gap in a functionally determined reentrant circuit. Studies in the sterile pericarditis model of atrial flutter. Circulation 1994;90:1997-2014. [PubMed]
- Moriguchi M, Niwano S, Yoshizawa N, et al. Inhomogeneity in the appearance of electrical remodeling during chronic rapid atrial pacing: evaluation of the dispersion of atrial effective refractoriness. Jpn Circ J 2001;65:335-40. [PubMed]
- Kojima J, Niwano S, Moriguchi M, et al. Effect of pilsicainide on atrial electrophysiologic properties in the canine rapid atrial stimulation model. Circ J 2003;67:340-6. [PubMed]
- Moriguchi M, Niwano S, Yoshizawa N, et al. Verapamil suppresses the inhomogeneity of electrical remodeling in a canine long-term rapid atrial stimulation model. Pacing Clin Electrophysiol 2003;26:2072-82. [PubMed]
- Fukaya H, Niwano S, Satoh D, et al. Inhomogenic effect of bepridil on atrial electrical remodeling in a canine rapid atrial stimulation model. Circ J 2008;72:318-26. [PubMed]
- Li D, Fareh S, Leung TK, et al. Promotion of atrial fibrillation by heart failure in dogs: atrial remodeling of a different sort. Circulation 1999;100:87-95. [PubMed]
- Wijffels MC, Kirchhof CJ, Dorland R, et al. Atrial fibrillation begets atrial fibrillation. A study in awake chronically instrumented goats. Circulation 1995;92:1954-68. [PubMed]
- Mihm MJ, Yu F, Carnes CA, et al. Impaired myofibrillar energetics and oxidative injury during human atrial fibrillation. Circulation 2001;104:174-80. [PubMed]
- Kim YM, Guzik TJ, Zhang YH, et al. A myocardial Nox2 containing NAD(P)H oxidase contributes to oxidative stress in human atrial fibrillation. Circ Res 2005;97:629-36. [PubMed]
- Goette A, Schön N, Kirchhof P, et al. Angiotensin II-antagonist in paroxysmal atrial fibrillation (ANTIPAF) trial. Circ Arrhythm Electrophysiol 2012;5:43-51. [PubMed]
- Yamashita T, Inoue H, Okumura K, et al. Randomized trial of angiotensin II-receptor blocker vs. dihydropiridine calcium channel blocker in the treatment of paroxysmal atrial fibrillation with hypertension (J-RHYTHM II study). Europace 2011;13:473-9. [PubMed]