Validation of black blood late gadolinium enhancement (LGE) for evaluation of myocardial infarction in patients with or without pathological Q-wave on electrocardiogram (ECG)
Introduction
Identification of myocardial infarction (MI) is critical for determination of prognosis and treatment. The electrocardiogram (ECG) is the most commonly used method for clinical diagnosis of MI because of its speed, convenience, and practicality. MR is capable of detecting small areas of infarction that may not be detected by ECG (1,2). The presence of pathological Q-wave (QW) on ECG is considered an important indicator of previous MI, and MIs with QW portend worse prognosis compared to non-Q-wave (NQW) infarctions (3). The traditional view that infarction transmurality correlates with the presence of QW has been challenged (1). Previous work demonstrated an inconsistent relationship between QWs and infarction transmurality (4,5). Clinical studies suggest that pathological QW on ECG is highly related to infarct size and extent of subendocardial infarction (6-8).
The pathological basis of QWs has mainly been studied through autopsy and animal experiments (9-11). However, in vivo studies more accurately inform clinical practice. In vivo assessment with late gadolinium enhancement (LGE) CMR has evolved as an excellent method for identification of infarcted myocardium and has been widely adopted for the qualitative and quantitative assessment of MI (12). LGE studies have demonstrated an inconsistent relationship between QW and infarction transmurality. Moon et al. showed that 29% of transmural MIs didn’t demonstrate pathological QWs and 28% of the NQW MIs showed transmurality (6). Additionally, Engblom et al. reported that the extent of subendocardial MI had greater predictive value for QW than infarct transmurality (8). Therefore, QW/NQW MIs are not respectively synonymous to transmural/non-transmural MIs.
However, the bright blood pool of Conv-LGE poses a practical challenge reducing the conspicuity of subendocardial MI (13). Given that the extent of subendocardial MI has greater predictive value for the presence of QWs, more accurate quantitation of subendocardial MI may improve the determination of the pathological basis of QW. Various methods have been proposed to suppress the blood pool signal and improve delineation of infarcted myocardium from the bright blood pool. These methods are collectively referred to as dark- (suppressed) or black- (nulled) blood LGE techniques, and most of these require special preparation and parameter adjustment (14-18). The method described by Holtackers does not require magnetization preparation, a feature facilitating availability on all scanners (19). For the purposes of this work, we will use the term black blood LGE or (BB-LGE) going forward. Our aim was to test a novel black blood (BB) sequence for LGE in patients with known previous MI, compare this sequence to the standard Conv LGE sequence in these patients, and to correlate these findings with the ECG findings in terms of presence or absence of QW. We adopted a recently proposed BB-LGE sequence: T(Rho) And Magnetization Transfer and INvErsion Recovery (TRAMINER) for dark blood LGE (referred as BB-LGE here forward). We hypothesized that improved detection of subendocardial enhancement with BB-LGE would result in improved correlation of ECG and extent of infarction.
Methods
Patient population
The local institutional review board approved this study. Written informed consent was obtained from all participants. Consecutive inpatients in our hospital with confirmed MI were enrolled from February 2017 to September 2017. The interval since MI was expressed in days. When available, ECG, troponin levels, and coronary artery angiography (CAG) were used to confirm MI. All the participants were affirmed for MR compatibility. Demographics and medical history were obtained from the patient’s medical record. Exclusion criteria included severe arrhythmia, myocarditis, a history indicating infiltrative cardiomyopathy, and severe impairment of renal function (glomerular filtration rate <30 mL/min/1.73 m2).
ECG analysis
The ECG was analyzed by one experienced cardiologist blinded to the results of the CMR. The patients were divided into QW and NQW groups. The definition of QW MI is based on the work of Moon et al. who compared the Thrombolysis In Myocardial Infarction (TIMI) definition with the European Society of Cardiology/American College of Cardiology (ESC/ACC) (6).
MRI technique
Cardiac MR scans were performed using a 3.0T whole body scanner (Discovery MR 750W, GE, WI) equipped with a 32-channel phase array cardiac coil. Patients were scanned in the head-first and supine position. The whole imaging protocol mainly included Localizers (Axial, Sagittal, Coronal), Cine SSFP plane localizing scans, Cine SSFP, T1WI TSE without fat sat, T1WI TSE with fat sat, T2WI TSE with fat sat (some patients with acute MI), first-pass perfusion, LGE images and BB-LGE images. Cine, LGE images and BB-LGE images were acquired in two chamber, four chamber and short-axis (from basal to apical) views, T1WI and T2WI images were acquired in short-axis (basal, mid-ventricular, and apical) views. The TRAMINER technique used three B1-insensitive rotation-4 (BIR-4) pulses prior to a non-selective IR pulse combing with single-shot balanced steady-state free-precession (bSSFP) readout (18). In the present study, the TRAMINER preparation was combined with a breath-hold segmented spoiled gradient recall (SPGR) acquisition.
Conv-LGE acquisition was performed in the mid-diastolic phase 10–20 min following the intravenous administration of gadopentetate dimeglumine (Bayer Schering, Germany) contrast agent administration (2.0 mL/s, 0.2 mmol/kg). Images were obtained in the long axis and short axis planes from base to apex. The scan parameters of Conv-LGE are as follows: ECG triggered acquisition, long axis slice thickness 5 mm, short axis slice thickness 8 mm, slice gap 0 mm, TE/TR 1.0 ms/5.4 ms, FOV 38×28 cm2, matrix 220×192, FA 25°, TI 280–380 ms. Selection of the inversion time (TI) was performed empirically, or aided by a IR-cine scout based on the nulling of the healthy myocardial signal (20,21). The duration of Conv-LGE acquisition was 6–8 min.
The order of Conv-LGE and BB-LGE was randomized. Identical parameters were used except the TI, which was also obtained based on a TI-scout sequence to null the signal of the blood pool. TI determined by the spatially nonselective inversion recovery (NSIR) pulse to reading out the contrast-relevant data portion. Selection of the inversion time (TI) of BB-LGE was aided by the TI-scout sequence, TI varied from 200–280 ms with time post contrast. The duration of BB-LGE acquisition was also 6–8 min. Hence the overall acquisition duration of LGE and BB-LGE was about 22–25 min after contrast administration.
Image analysis
Subjective image quality assessment of the long and short axis images was rated on a 3-point Likert scale (1, poor; 2, acceptable; 3 excellent) describing the ease of differentiation of MI from normal myocardium remote from the infarct, MI from blood pool, and blood pool from normal myocardium remote from the infarct.
Objective image quality assessment utilized the short axis images of Conv-LGE and BB-LGE that were processed using vendor supplied workstation (AW volume share 5, GE, WI). For every patient, ROIs of normal myocardium, MI and blood pool were selected. The averages of the ROI signal were recorded and normalized into 0–100 SI scale (18). Noise measurements are not reliable in BB-LGE images as a result of signal normalization done by phase-sensitive inversion recovery (PSIR) (22). Consequently, signal-to-noise ratio (SNR) and contrast-to-noise ratio (CNR) were not calculated, but signal intensity ratio (SIR) and contrast-to-noise ratio (CNRA–B) of different tissues were calculated using the normalized signal intensities according to the following formula (18,23):
| [1] |
| [2] |
In above equation, SImax,new and SImin,new are the normalized SI according to new scale (0 and 100, respectively), SIv,new is the normalized SI value of any voxel, SIv,orig is the actual SI of the same voxel, where SA and SB are defined as the signal of two tissues (myocardial wall and blood pool) and SD(SA) and SD(SB) are defined as the standard deviation of two tissues signal, respectively.
Two experienced radiologists assessed the MI based on the American Heart Association 17-segment model including the number of infarcted segments and transmural degree (24). If there was discrepancy between two reviewers, a third senior reviewer was invited for the final judgement. To false-positive characterization of blood pool as hyperintense myocardium due to partial volume effects or incomplete nulling of the blood pool on the TRAMINER sequence correlation of hyperintense regions to left ventricular wall on the other structural images assisted our determination.
In assessing transmural grading of MI with or without QW, the patients were divided into QW and NQW groups according to ECG by an experienced cardiologist. Transmural grading was rated as: Grade 0 (0%), Grade 1 (0–25%), Grade 2 (26–50%), Grade 3 (51–75%), Grade 4 (76–100%) (25).
In assessing the size of MI, the short axis images of Conv-LGE and BB-LGE were imported in commercial software (cvi42, Circle Cardiovascular Imaging, Canada). The endocardium and epicardium were drawn in the short axis images covering from base to apex, and ROIs were manually drawn on healthy myocardium and MI. Finally, the ratio of MI volume to whole myocardial volume (MI%) was automatically calculated based on the full width half max.
Statistical analysis
All the statistical analyses were performed using SPSS 20.0. Data distributions were tested using Shapiro-Wilk test, enumeration data were reported as mean ± SD and categorical data were reported using frequencies and grading.
The differences of SI, SIR and CNR between healthy myocardium, MI and blood pool obtained by Conv-LGE and BB-LGE were tested using paired Student’s t-test, and P<0.05 was considered statistically significant. Differences of MI detection rate between Conv-LGE and BB-LGE were assessed using McNemar test. Differences of MI transmural grading using Conv-LGE and BB-LGE in patients with or without QW were tested using Wilcoxon signed rank text and Mann-Whitney U-test. Paired t-test and Bland-Altman analysis were used to test the differences of MI% in two techniques. The level of agreements between reviewers was assessed using intraclass correlation coefficients (ICCs): ICC <0.21, poor; ICC =0.21–0.40, fair; ICC =0.41–0.60, moderate; ICC =0.61–0.80, good; ICC >0.80, excellent (18). ICCs were reported with 95% confidence interval (CI).
Results
Clinical characteristics
Although 64 patients were enrolled, 8 were excluded due to incomplete CMR or poor image quality due to arrhythmias or breathing artifacts. Fifty-six subjects were analyzed for the final analysis. Fifty-two were inpatients and 4 were outpatients. All subjects had ECG evidence of MI and had CAG confirmation of vessel occlusion concordant with MI. Thirty-six percent of patients (n=20) had elevated troponins. Patient characteristics are shown in Table 1. It needs to be emphasized that the time of infarction had a broad distribution with the interval from the time of infarction to imaging from 2 to 7,200 days and the median was 225 days, left ventricular ejection fraction (LVEF) of patients were from 8% to 82% and the mean standard deviation was 38%±18%. QW were found in 23/56 cases (41%) among which 2/23 cases (9%) showed subendocardial MI (Figure 1A,B,C,D,E) and 21/23 cases (91%) showed transmural MI (Figure 1F,G,H,I,J). NQW were found in 33/56 cases (59%), among which 14/33 (42.4%) demonstrated transmural MI (Figure 1K,L,M,N,O).
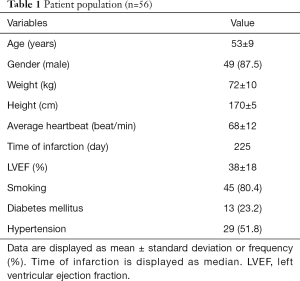
Full table
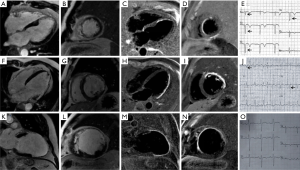
Quantitative assessment of image quality and MI% between Conv-LGE and BB-LGE
BB-LGE provided a higher subjective rating discriminating infarcted myocardium from blood pool compared to Conv-LGE (P<0.001). There were no significant differences in other subjective image quality parameters (P=0.068, P=0.407) (Table 2). On objective image quality assessment, BB-LGE provided higher CNR (P<0.001) and SIR (P<0.001) between infarcted myocardium and blood pool compared to Conv-LGE (Table 3).
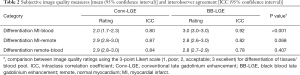
Full table
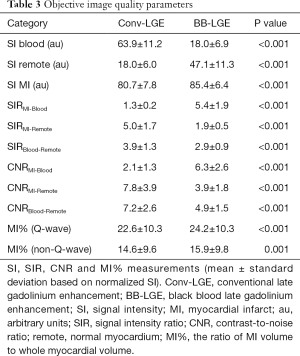
Full table
A larger MI% was revealed in BB-LGE than with Conv-LGE in both QW (P<0.001) and NQW (P<0.001) groups (Table 3, Figure 2). The MI% was larger in the QW group than the NQW group (P<0.001). The interobserver agreement assessed using ICC (intraclass correlation coefficient) showed excellent consistency of the two techniques (0.99, 0.99). The Bland-Altman analysis showed low measurement bias in Conv-LGE and BB-LGE techniques (Figure 3).
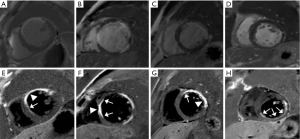
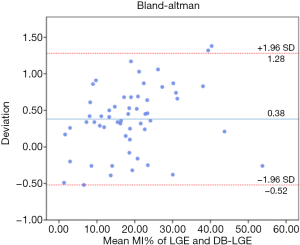
Transmural grading with Conv-LGE and BB-LGE in QW and NQW groups
Based on American Heart Association 17-segment model, a total of 401 MI segments (approx. 42.1%) were found using Conv-LGE, and a total of 450 MI segments (approx. 47.3%) were found using BB-LGE. The 49 additional MI segments that were only found on BB-LGE and not present on Conv-LGE were all subendocardial MI. All of these MI segments were indistinct on Conv-LGE and missed by radiologists. The difference in two techniques was statistically significant (P<0.001) (Table 4).
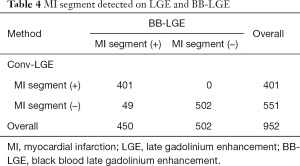
Full table
The number of MI segments in different transmural grades by both techniques in the QW group (n=23) are displayed in Figure 4A. A significant difference in transmural grading was found between the two techniques. Compared to Conv-LGE, BB-LGE detected more subendocardial infarcted segments in QW group (Z=−4.24, P<0.001).
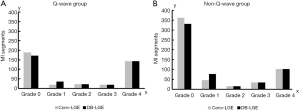
The number of MI segments in different transmural grades by both techniques in NQW group (n=33) are displayed in Figure 4B. There was a significant difference in transmural grading between the two techniques. In the NQW group, BB-LGE detected more subendocardial infarcted segments compared to Conv-LGE (Z=−5.57, P<0.001).
Discussion
In this single-center cohort study, BB-LGE provided greater contrast between infarcted myocardium and blood pool, which improved detection and sizing of subendocardial MI. Our study demonstrated that (I) QW MIs were more frequently present in transmural MIs and in larger subendocardial infarctions, (II) NQW MIs were more frequently present in subendocardial MIs, (III) subendocardial infarcts were more frequently found in NQW MIs rather than QW MIs, (IV) transmural infarcts were more frequently found in QW MIs, and (V) the MI% of QW MIs was larger than that of MI% of NQW MIs.
It is generally believed that the pathological QW is an important indicator of infarcted myocardium, and the classification by QW or NQW plays a significant role in determining prognosis of patient with MIs (3). Our work further informs the pathological basis of QW vs. NQW MIs and supports challenges to the classical respective correlation to transmural vs. non-transmural MIs (4,5). Our results are concordant with previous Conv-LGE studies showing pathological QWs are more highly related to infarcted myocardium size rather than transmurality (6,7). Therefore, presence of QW on ECG is an indicator for larger infarcted myocardial volume.
One of the important factors in determining if a patient will benefit from vascular intervention is having scar tissue less than 50% of the myocardial thickness (26). We showed that the BB-LGE method detected more subendocardial scars than Conv-LGE. In particular, more subendocardial infarcted segments were found in the NQW group. Therefore, BB-LGE can provide more accurate assessment of NQW patients and better determine those who could benefit from intervention better predict their prognosis.
Conv-LGE has been recognized as the gold standard in identifying infarcted myocardium producing good contrast between infarcted and healthy myocardium (21,27-30). However, the Conv-LGE hyperintensity of blood pool and subendocardial MI results in lower contrast and conspicuity between the blood pool and subendocardial MI (13). This is particularly problematic in NQW MIs that are frequently smaller.
Many efforts have been made to mitigate this limitation (31-35). Obviously, increasing the contrast between the blood pool and infarcted myocardium will help improve the detection of subendocardial MI, as shown in Figure 2. Typically, LGE with blood suppression may be achieved by combining an inversion recovery (IR) pulse and a T2 preparation module (14,16). In this study, we adopted a modification of the recently proposed T(Rho) And Magnetization Transfer and INvErsion Recovery (TRAMINER) technique for black blood LGE (18). This technique uses three BIR-4 pulses prior to a non-selective IR pulse combined with single-shot bSSFP readout to provide high image contrast between subendocardial infarction and adjacent blood pool. However, single-shot readout may lead to relatively poor spatial resolution and low SNR. In the present study, the TRAMINER preparation combining with segmented SPGR acquisition was used to obtain relative high-resolution black-blood LGE. BB-blood LGE and traditional PSIR images were acquired with the same resolution and position, leading to one-by-one comparison. Recently, Holtackers et al. used a novel dark-blood LGE approach without using additional magnetization preparation (36), although our BB-LGE needed magnetic preparation, and used a modified magnetic preparation with lower power and energy requirements. The applicability in routine clinical practice is still further strengthened and readily available without the need for scanner adjustments, extensive optimizations, or additional training. Furthermore, our BB-LGE images had excellent blood suppression and high observer confidence.
The subjective and objective assessments used to evaluate the image quality are reported in Table 2 and Table 3. Compared to Conv-LGE images, BB-LGE provided better contrast between MI and blood pool because of nulling of the high signal of the blood pool. This provided more accurate diagnostic information for identification of subendocardial MI. We found 49 more subendocardial MI segments on BB-LGE than Conv-LGE. All of these MI segments were indistinct on Conv-LGE and missed by radiologists. In addition, although in objective image quality assessment there were significant differences between MI from normal myocardium and blood from normal myocardium between two techniques, there were no visual differences for readers in subjective image quality assessment. While the possibility of false-positive characterization myocardium in a region of LGE due to partial volume effects or incomplete nulling of the blood pool was considered, the overall excellent blood pool nulling of the TRAMINER sequence and correlation of LGE regions with left ventricular wall on the other structural images lend confidence to our determination.
The greater conspicuity of subendocardial MIs found not only in NQW MIs but also in QW MIs led to larger MI% with BB-LGE compared to Conv-LGE. Therefore, BB-LGE can provide better insight into the pathologic basis of QW and NQW. While our results did support part of our hypothesis in that BB-LGE findings correlated with ECG findings, the key insight related to MI%, with QW associating with larger MI% not solely to transmurality.
Our study has several limitations. First, the study population is small, and from a single study center. Second, we used the Conv-LGE and BB-LGE as the reference of infarcted myocardium, but this more accurately reflects myocardial fibrosis. Our inclusion and exclusion criteria largely mitigated this potential confounder. Patients with known infiltrative cardiomyopathy or myocarditis were excluded. Although Conv-LGE is a generally accepted criterion for infarcted myocardium and BB-LGE has higher SIR and CNR in the identification of subendocardial MIs, we did not have pathological proof for infarcted myocardium. However, the patients who showed an ischemic scar pattern on BB-LGE but not on Conv-LGE, had known coronary artery disease that was related to the infarct territory, which was confirmed by ECG, troponin levels, and CAG. Third, ideally for accurate signal-to-noise and contrast-to-noise assessment, noise measurements could have been done by running dedicated noise scans without using RF excitations, also for PSIR sequences. Fourth, the excellent work of Dall’Armellina et al. provided a clarification of dynamic changes of LGE in acute MI (37), and there was a diverse population of acute and chronic MI patients ranging from 2–7,200 days post infarction thus potentially confounding the analysis by including both infarcted myocardium and salvage tissue in the LGE volumes. This issue, while important for analysis of a primary endpoint of MI% accuracy determination, is less important given that this is an intraindividual comparison of two sequences at essentially a single time point, and the impact of inclusion of salvage tissue is the same across the two techniques. That being said, the inclusion of both myocardial salvage tissue and infarction should be acknowledged in review of our data Fifth, our version of TRAMINER used an SPGR rather than bSSFP sequence and this has not been independently validated. Finally, there has been no follow-up for medium and long-term prognosis in patients with QW or NQW MIs in our study, and we have no data focusing on correlative imaging, clinical outcomes and prognosis.
Conclusions
In conclusion, compared to Conv-LGE, BB-LGE provided more accurate detection and characterization of infarction in terms of transmurality and subendocardial extent with volume of LGE contributing to the presence or absence of QWs. Due to nulling the high signal of blood pool, BB-LGE can effectively improve the identification of subendocardial MI which may be missed on Conv-LGE. In both QW and NQW MIs, BB-LGE detects more subendocardial MIs and a larger MI% is found. This may be helpful in more accurate quantitative assessment in the study of both QW and NQW MIs and may offer greater insights in the management of NQW patients who might have prognostic benefit from intervention.
Acknowledgments
Funding: This work was supported by the National Natural Science Foundation of China (81771787, 81671647), Beijing Municipal Natural Science Foundation (7172069, 7161003), Capital Health Research and Development of Special (2016-4-2063, 2016-2-2062), Talent Project of Beijing Municipal Human Resource and Social Security Bureau (2016-117). Foundation for Major Research Program of Yunnan Province (2017FE468-178), Talent Project of Yunnan Province (2015HB068; D-201646).
Footnote
Conflicts of Interest: All authors have completed the ICMJE uniform disclosure form (available at http://dx.doi.org/10.21037/cdt.2019.12.11). LZ serves as an unpaid editorial board member of Cardiovascular Diagnosis and Therapy from Jul 2019 to Jun 2021. The other authors have no conflicts of interest to declare.
Ethical Statement: The authors are accountable for all aspects of the work in ensuring that questions related to the accuracy or integrity of any part of the work are appropriately investigated and resolved. The local institutional review board approved this study. Written informed consent was obtained from all participants.
Open Access Statement: This is an Open Access article distributed in accordance with the Creative Commons Attribution-NonCommercial-NoDerivs 4.0 International License (CC BY-NC-ND 4.0), which permits the non-commercial replication and distribution of the article with the strict proviso that no changes or edits are made and the original work is properly cited (including links to both the formal publication through the relevant DOI and the license). See: https://creativecommons.org/licenses/by-nc-nd/4.0/.
References
- Nadour W, Doyle M, Williams RB, et al. Does the presence of Q waves on the EKG accurately predict prior myocardial infarction when compared to cardiac magnetic resonance using late gadolinium enhancement? A cross-population study of noninfarct vs infarct patients. Heart Rhythm 2014;11:2018-26. [Crossref] [PubMed]
- Kwong RY, Chan AK, Brown KA, et al. Impact of unrecognized myocardial scar detected by cardiac magnetic resonance imaging on event-free survival in patients presenting with signs or symptoms of coronary artery disease. Circulation 2006;113:2733-43. [Crossref] [PubMed]
- Lorgis L, Jourda F, Hachet O, et al. Prognostic value of fragmented QRS on a 12-lead ECG in patients with acute myocardial infarction. Heart Lung 2013;42:326-31. [Crossref] [PubMed]
- Pipberger H, Schwartz DJ, Massumi RA, et al. Studies on the mechanism of ventricular activity. XXI. The origin of the depolarization complex, with clinical applications. American Heart Journal 1957;54:511-30. [Crossref] [PubMed]
- Cook RW, Edwards J, Pruitt R. Electrocardiographic changes in acute subendocardial infarction. I. Large subendocardial and large nontransmural infarcts. Circulation 1958;18:603-12. [Crossref] [PubMed]
- Moon JCC, De Arenaza DP, Elkington AG, et al. The pathologic basis of Q-wave and non-Q-wave myocardial infarction: a cardiovascular magnetic resonance study. J Am Coll Cardiol 2004;44:554-60. [Crossref] [PubMed]
- Kaandorp TAM, Bax JJ, Lamb HJ, et al. Which parameters on magnetic resonance imaging determine Q waves on the electrocardiogram? Am J Cardiol 2005;95:925-9. [Crossref] [PubMed]
- Engblom H, Carlsson MB, Hedström E, et al. The endocardial extent of reperfused first-time myocardial infarction is more predictive of pathologic Q waves than is infarct transmurality: a magnetic resonance imaging study. Clin Physiol Funct Imaging 2007;27:101-8. [Crossref] [PubMed]
- Prinzmetal M, Shaw CM Jr, Maxwall MH, et al. Studies on the mechanism of ventricular activity. VI. The depolarization complex in pure subendocardial infarction; role of the subendocardial region in the normal electrocardiogram. Am J Med 1954;16:469-89. [Crossref] [PubMed]
- Stone PH, Raabe DS, Jaffe AS, et al. Prognostic significance of location and type of myocardial infarction: independent adverse outcome associated with anterior location. J Am Coll Cardiol 1988;11:453-63. [Crossref] [PubMed]
- Aguirre FV, Younis LT, Chaitman BR, et al. Early and 1-year clinical outcome of patients' evolving non-Q-wave versus Q-wave myocardial infarction after thrombolysis. Results from The TIMI II Study. Circulation 1995;91:2541-8. [Crossref] [PubMed]
- Kwon DH, Asamoto L, Popovic ZB, et al. Infarct characterization and quantification by delayed enhancement cardiac magnetic resonance imaging is a powerful independent and incremental predictor of mortality in patients with advanced ischemic cardiomyopathy. Circ Cardiovasc Imaging 2014;7:796-804. [Crossref] [PubMed]
- Kellman P, Chung YC, Simonetti OP, et al. Multi-contrast delayed enhancement provides improved contrast between myocardial infarction and blood pool. J Magn Reson Imaging 2005;22:605-13. [Crossref] [PubMed]
- Liu CY, Wieben O, Brittain JH, et al. Improved delayed enhanced myocardial imaging with T2-Prep inversion recovery magnetization preparation. J Magn Reson Imaging 2008;28:1280-6. [Crossref] [PubMed]
- Peel SA, Morton G, Chiribiri A, et al. Dual inversion-recovery mr imaging sequence for reduced blood signal on late gadolinium-enhanced images of myocardial scar. Radiology 2012;264:242-9. [Crossref] [PubMed]
- Kellman P, Xue H, Olivieri LJ, et al. Dark blood late enhancement imaging. J Cardiovasc Magn Reson 2016;18:77. [Crossref] [PubMed]
- Kim HW, Rehwald WG, Jenista ER, et al. Dark-Blood Delayed Enhancement Cardiac Magnetic Resonance of Myocardial Infarction. JACC Cardiovasc Imaging 2018;11:1758-69. [Crossref] [PubMed]
- Muscogiuri G, Rehwald WG, Schoepf UJ, et al. T(Rho) and magnetization transfer and INvErsion recovery (TRAMINER)-prepared imaging: A novel contrast-enhanced flow-independent dark-blood technique for the evaluation of myocardial late gadolinium enhancement in patients with myocardial infarction. J Magn Reson Imaging 2017;45:1429-37. [Crossref] [PubMed]
- Holtackers RJ, Chiribiri A, Schneider T, et al. Dark-blood late gadolinium enhancement without additional magnetization preparation. J Cardiovasc Magn Reson 2017;19:64-10. [Crossref] [PubMed]
- Kim RJ, Shah DJ, Judd RM. How we perform delayed enhancement imaging. J Cardiovasc Magn Reson 2003;5:505-14. [Crossref] [PubMed]
- Gupta A, Lee VS, Chung YC, et al. Myocardial infarction: optimization of inversion times at delayed contrast-enhanced MR imaging. Radiology 2004;233:921-6. [Crossref] [PubMed]
- Kellman P, Arai AE, McVeigh ER, et al. Phase-sensitive inversion recovery for detecting myocardial infarction using gadolinium-delayed hyperenhancement. Magn Reson Med 2002;47:372-83. [Crossref] [PubMed]
- Ratering D, Baltes C, Dörries C, et al. Accelerated cardiovascular magnetic resonance of the mouse heart using self-gated parallel imaging strategies does not compromise accuracy of structural and functional measures. J Cardiovasc Magn Reson 2010;12:43. [Crossref] [PubMed]
- Cerqueira MD, Weissman NJ, Dilsizian V, et al. Standardized myocardial segmentation and nomenclature for tomographic imaging of the heart. A statement for healthcare professionals from the Cardiac Imaging Committee of the Council on Clinical Cardiology of the American Heart Association. Circulation 2002;105:539-542. [Crossref] [PubMed]
- Kramer CM, Barkhausen J, Flamm SD, et al. Society for Cardiovascular Magnetic Resonance Board of Trustees Task Force on Standardized Protocols. Standardized cardiovascular magnetic resonance (CMR) protocols 2013 update. J Cardiovasc Magn Reson 2013;15:91. [Crossref] [PubMed]
- Ogawa M, Doi K, Fukumoto A, et al. Reverse-remodeling after coronary artery by pass grafting in ischemic cardiomyopathy: assessment of myocardial viability by delayed-enhanced magnetic resonance imaging can help cardiac surgeons. Interact Cardiovasc Thorac Surg 2007;6:673-5. [Crossref] [PubMed]
- Fieno DS, Kim RJ, Chen EL, et al. Contrast-enhanced magnetic resonance imaging of myocardium at risk: distinction between reversible and irreversible injury throughout infarct healing. J Am Coll Cardiol 2000;36:1985-91. [Crossref] [PubMed]
- Rayatzadeh H, Tan A, Chan RH, et al. Scar heterogeneity on cardiovascular magnetic resonance as a predictor of appropriate implantable cardioverter defibrillator therapy. J Cardiovasc Magn Reson 2013;15:31. [Crossref] [PubMed]
- Kim RJ, Fieno DS, Parrish TB, et al. Relationship of MRI delayed contrast enhancement to irreversible injury, infarct age, and contractile function. Circulation 1999;100:1992-2002. [Crossref] [PubMed]
- Kellman P, Arai AE. Cardiac imaging techniques for physicians: late enhancement. J Magn Reson Imaging 2012;36:529-42. [Crossref] [PubMed]
- Bandettini WP, Kellman P, Mancini C, et al. MultiContrast Delayed Enhancement (MCODE) improves detection of subendocardial myocardial infarction by late gadolinium enhancement cardiovascular magnetic resonance: a clinical validation study. J Cardiovasc Magn Reson 2012;14:83. [Crossref] [PubMed]
- Ibrahim SH, Weiss RG, Stuber M, et al. Stimulated-echo acquisition mode (STEAM) MRI for black-blood delayed hyperenhanced myocardial imaging. J Magn Reson Imaging 2008;27:229-38. [Crossref] [PubMed]
- Tao Q, Piers SRD, Lamb HJ, et al. Automated left ventricle segmentation in late gadolinium-enhanced MRI for objective myocardial scar assessment. J Magn Reson Imaging 2015;42:390-9. [Crossref] [PubMed]
- Basha TA, Tang MC, Tsao C, et al. Improved dark blood late gadolinium enhancement (DB-LGE) imaging using an optimized joint inversion preparation and T2 magnetization preparation. Magn Reson Med 2018;79:351-60. [Crossref] [PubMed]
- Francis R, Kellman P, Kotecha T, et al. Prospective comparison of novel dark blood late gadolinium enhancement with conventional bright blood imaging for the detection of scar. J Cardiovasc Magn Reson 2017;19:91. [Crossref] [PubMed]
- Holtackers RJ, Van De Heyning CM, Nazir MS, et al. Clinical value of dark-blood late gadolinium enhancement cardiovascular magnetic resonance without additional magnetization preparation. J Cardiovasc Magn Reson 2019;21:44. [Crossref] [PubMed]
- Dall'Armellina E, Karia N, Lindsay AC, et al. Dynamic Changes of Edema and Late Gadolinium Enhancement After Acute Myocardial Infarction and Their Relationship to Functional Recovery and Salvage Index. Circ Cardiovasc Imaging 2011;4:228-36. [Crossref] [PubMed]