Prognostic biomarkers in pediatric pulmonary arterial hypertension
Introduction
Pediatric pulmonary arterial hypertension (PAH) is a progressive life-threatening disease of the pulmonary vasculature (1). In the past decades, major advances in treatment options for adults with PAH have been achieved, with currently 12 approved PAH targeted drugs (2). Nevertheless, it remains a devastating disease without a cure, with unsatisfactory high mortality rates. This is especially the case for children (3). Although randomized controlled trials (RCT’s) demonstrating efficacy in childhood PAH are lacking, these PAH targeted drugs are generally used in infants and children with PAH (4).
In the current era with an increased number of available PAH targeted drugs, one of the major challenges for clinicians is to tailor optimal treatment regimens for the individual child with PAH (5). Children with PAH present at different stages of disease and at different levels of risk for disease progression and mortality. Prognostic biomarkers are needed to inform clinicians on disease progression and risk of mortality and to guide decisions on how and when to use the available treatments, when to escalate therapy, and when to consider surgical options such as Pott’s shunt and listing for lung-transplantation.
According to the recently harmonized definition by regulatory authorities, a biomarker is “a defined characteristic that is measured as an indicator of normal biological processes, pathogenic processes, or responses to an exposure or intervention” (6). These include biochemical biomarkers in serum or plasma, but also functional, clinical, hemodynamic and imaging markers. The updated definition adds that a biomarker is not a direct assessment of how a patient feels, functions, or survives and therefore not of direct clinical meaning for a patient (6).
Prognostic biomarkers are a subtype of biomarkers that can be used to identify patients with greater likelihood of having a disease-related outcome event such as lung-transplantation or death in PAH (6). For this type of biomarkers, clinical or epidemiological studies are required to show a strong association with such endpoint events (7). Prognostic biomarkers are essential for risk stratification, informing treatment decisions in the management of chronic progressive diseases such as PAH and will therefore be reviewed in this report.
A common misconception regarding prognostic biomarkers is that their demonstrated association with mortality justifies their use for the evaluation of treatment efficacy, either in clinical management or in randomized clinical trials (7,8). However, only few prognostic biomarkers are useful for this purpose. Treatment efficacy is ideally measured by directly clinically meaningful outcomes (i.e., direct assessments of how patients feel, function or survive). Alternatively, surrogates that substitute for these directly clinically meaningful outcomes might be used (7). Surrogates are intrinsically part of the causal pathway of the disease, which can be validated by testing whether influences on the final clinically meaningful outcomes are causally explained by influences on the surrogate (7). A treatment goal represents a special use of validated surrogates, for which achieving predefined target levels of the measurement during treatment has been demonstrated to translate into improved disease outcome (9-11).
As pediatric PAH is a progressive disease with poor outcomes despite the available treatments, prognostic biomarkers are essential for risk stratification informing treatment decisions. This report reviews prognostic biomarkers in pediatric PAH, that are currently considered useful for the assessment of disease progression and outcome and may thereby aid in treatment decisions for individual children with PAH. Since prognostic biomarkers provide a pool of candidate surrogates that might play a role in evaluating treatment efficacy and a goal-oriented treatment strategy, we also comment on their potential as treatment goals when this is applicable.
Conventional physiological biomarkers
Heart rate
In the general population, resting heart rate carries prognostic value (12). Increased resting heart rate reflects neurohormonal activation and is an independent modifiable predictor of all-cause mortality in heart disease (13,14). In PAH, increased right ventricular afterload leads to impairment of ventricular stroke volume, via sympathetic activation leading to an increased heart rate to maintain cardiac output (15,16). Although heart rate obviously is not a disease specific parameter, the degree of heart rate increase has been shown to predict outcome in adults with PAH (17,18). Also in children, heart rate has been reported to correlate with outcome, although heart rate was not the primary marker under study and patient age might have confounded the results in these cohorts (19,20).
A more reliable non-invasive way to evaluate autonomic dysfunction based on heart rate, is assessment of heart rate variability (HRV) (21,22). Impaired HRV is a predictor of mortality in the general population, and impairments can be due to chronic increased sympathetic nervous system activity or due to stretching of the sinoatrial node in situations of atrial overload (21). Lammers et al. have evaluated HRV in children with PAH, by analyzing holter registrations for parameters reflecting the degree of fluctuations of heart rate around its mean value (22). The authors found all HRV parameters to be predictive of death or lung-transplantation. A similar study conducted by Latus et al. confirmed associations of HRV with disease severity and hemodynamic parameters in a different cohort (21). These studies in pediatric PAH underscore the potential of HRV as a prognostic biomarker, and their results might find clinical applications in the upcoming digital biomarker era when wearable technology and telemonitoring are expected to be rolled out on a larger scale.
Height and weight
A disease aspect that exclusively applies to the pediatric age, is the impact of the disease on growth. In childhood, growth is a marker of disease severity that may be predictive for outcome (23). Z-scores for weight and height are decreased in children with PAH and the degree of decrease has been shown to be associated with mortality (24). A study that pooled data from four prospective international registries of children with PAH, has found pediatric PAH to be associated with impaired growth, especially in younger patients and in those with concomitant congenital heart disease (CHD) (25). In addition, the degree of growth impairment was found to be independently associated with disease severity and disease duration. Moreover, catch-up growth over time was associated with a favorable clinical disease course, suggesting standardized height to be a simple and useful biomarker to monitor children over time. Increased caloric expenditure and altered metabolic demands may play an important role in growth impairment in PAH. Unraveling the mechanisms of growth failure, potential effects of treatment on growth and evaluating whether nutritional interventions may modify outcomes are important areas of future research.
Other conventional physiological measurements
In addition to heart rate and growth measurements, other conventional physiological measurements have anecdotally been shown to correlate with outcome in PAH, including transcutaneous oxygen saturation and blood pressure (3,26,27). Limited disease specificity and inconsistency regarding reported prognostic value in pediatric PAH currently hamper their usefulness as prognostic biomarkers for monitoring patients over time.
Biomarkers of functional status
World Health Organization functional class (WHO-FC)
WHO-FC is routinely applied in many expert centers for pediatric PAH and has been shown to be a strong predictor of mortality (28). Despite the disadvantages of being a potentially subjective measurement due to dependency on the observation and impression of caregivers, it has been demonstrated to be a robust and useful prognostic biomarker. Its applicability has been questioned in the youngest children, as it is a subjective measurement, especially in young children due to dependency on the observation and impression of caregivers. However, WHO-FC has been repeatedly demonstrated to be a powerful prognostic biomarker in pediatric PAH, also in the youngest patients (28).
WHO-FC was not originally designed for children and infants and characterization of “ordinary physical activity” has to be adjusted to the child’s age, similar as in the elderly. To address pediatric-specific aspects of PAH including growth and development, in 2011 the Pediatric Task Force of the Pulmonary Vascular Research Institute (PVRI) proposed the Panama “Pediatric Functional Class” with modifications according to developmental abilities and skills in different age ranges (29). In a single center retrospective chart review study, Balkin et al. demonstrated a high inter-observer agreement when scoring the Panama Functional Class (30). Although the authors were not able to demonstrate the prognostic value of Panama Functional Class at time of diagnosis, serial associations with morbidity and mortality increased during follow-up. Prospective longitudinal multicenter validation of the Panama Functional Class will be required before it can be recommended as prognostic biomarker.
6-minute walk distance (6MWD)
The 6-minute walk test is a standardized submaximal exercise test measuring the distance a patient is able to walk over a time frame of 6 minutes (31). 6MWD has been reported to correlate with mortality in adults with PAH and has long been used as an endpoint in treatment efficacy trials in adults (32). In young children, 6MWD is limited due to developmental restrictions: infants are not able to walk and developmental capabilities and motivational issues may affect the test results. 6MWD was not identified as a predictor of mortality in cohorts that included many children below the age of 7 (28). However, in pediatric cohorts consisting of patient that are sufficiently able and old enough to perform this test reliably (e.g., >7 years), 6MWD was demonstrated to be a strong predictor of outcome (22,33). Improvement of 6MWD following treatment initiation or escalation likely reflects improved exercise capacity and quality of life and thus efficacy of treatment (34). It is unclear, however, whether such treatment-induced improvements reflect decreased mortality risks in children with PAH (10). The 6MWD is regarded to reflect patients exercise tolerance, and thus a clinically meaningful endpoint, and this has lead the pediatric task force of the 6th World Symposium on Pulmonary Hypertension to recommend achieving to improve 6MWD as a treatment goal (4).
Cardiopulmonary exercise testing (CPET)
CPET is a noninvasive method to comprehensively assess exercise capacity, and in expert hands it is a reliable method to assess the degree of exercise intolerance (34). Three measurements shown to be prognostic biomarkers in both adults and children are (I) peak oxygen consumption and (II) the relationship between minute ventilation and carbon dioxide output (VE/VCO2) also called ventilatory efficiency slope and (III) peak systolic blood pressure (34-37). Even more than is the case with 6MWT, the feasibility of CPET in children depends on developmental and motivational factors. Moreover, CPET requires considerable experience and expertise hampering standardization. The first RCT in pediatric PAH used peak oxygen consumption measured by CPET as the primary endpoint (38). In this pediatric trial, only half of the included children appeared physically and developmentally able to perform CPET, illustrating the limitation of CPET biomarkers in a pediatric population.
Daily physical activity level
Portable electronic accelerometers can accurately and continuously monitor physical activity in the home setting, providing extensive information on exercise capacity that might go far beyond a 6MWT or CPET in a hospital setting (39). A pilot study from Zijlstra et al. demonstrated the feasibility of objective physical activity level assessment by accelerometry in children with PAH of various ages (40). Moreover, this pilot study showed that physical activity measured by accelerometry was significantly lower in children with PAH when compared to age-matched controls and correlated with disease severity and time to a clinical event. These findings suggest that continuously measured physical activity level can serve as a prognostic biomarker. Daily physical activity level, as measured by wearable accelerometers, is now under investigation as a potential end point in both adult and pediatric PAH trials (clinicaltrials.gov Identifier NCT03078907). If confirmed, this technique holds promise as a cost-effective monitoring tool for both clinical practice and future treatment efficacy trials (41).
Electrocardiographic biomarkers
Routine electrocardiography (ECG) is non-invasive and can be performed repeatedly during the disease course of patients with PAH. The ECG of patients with PAH can show signs of right atrial enlargement, RV hypertrophy and right axis deviation, although this is not the case in all children with PAH (42-44). In adults with PAH, increased P wave amplitude in lead II, the presence of a qR-pattern or increased R wave amplitude in lead V1 are associated with worse hemodynamics and decreased survival (45,46). Treatment-induced changes of ECG parameters reflecting RV hypertrophy are associated with concurrent changes in hemodynamics and survival (47-49), providing clues that ECG biomarkers can serve as adjunct prognosticators for risk stratification and for monitoring the disease over time.
Although age-dependent references have to be carefully taken into account in children, recent data suggests that serial ECG’s could also have a supporting role in the follow-up of pediatric PAH. In a retrospective study of children with idiopathic PAH, RV hypertrophy ECG parameters were shown to correlate with hemodynamics and to differ between survivors and non-survivors (survival free of Potts shunt or transplantation) (50). Larger scale longitudinal outcome studies are indicated to determine the clinical value of ECG parameters as potential prognostic biomarkers in pediatric PAH.
Circulating serum biomarkers
Circulating serum biomarkers have the advantage of being investigator-independent and easy to obtain through peripheral blood samples (4). Several circulating biomarkers have been investigated in children with PAH.
Natriuretic peptides
Natriuretic peptides are synthesized in cardiomyocytes in response to increased muscular wall stress (51). After cleavage of Pre Pro B-type Natriuretic Peptide (BNP), Pro BNP is split in active BNP and inactive, more stable N-terminal BNP (NT-proBNP). Serum levels of BNP and NT-proBNP have been shown to be closely linked to RV function, the main determinant of clinical outcome in PAH (52).
In pediatric PAH, both biomarkers are associated with disease severity, as associations have been demonstrated with WHO functional class and 6MWD (53-55). It has been demonstrated that changes in NT-proBNP during clinical management also correlate with serial changes in disease severity (53). Both BNP and NT-proBNP are also associated with invasive hemodynamics measurements, and their value as robust prognostic biomarkers has been demonstrated in multiple studies (28). In clinical practice, NT-proBNP is preferred over BNP, as it is more stable with a longer half-life and carries a stronger predictive value for right heart failure and mortality. NT-proBNP was found not only to be associated with mortality at time of diagnosis, but also at different time points during the course of the disease (10,56). Moreover, serial changes in NT-proBNP over time have been demonstrated to reflect changes in long-term outcome, strongly suggesting that NT-proBNP can function as a surrogate for mortality and can be used to evaluate treatment efficacy (56).
Uric acid
Hyperuricemia is a common finding in cardiovascular disease, including pulmonary hypertension (57-60). Increased levels of uric acid can result from several mechanisms, with increased activation of xanthine oxidase caused by oxidative stress or tissue hypoxia being the most likely in the setting of vascular and cardiac dysfunction (61,62). It has been speculated that elevated uric acid levels are not only a consequence of pulmonary hypertension, but that it may also have a secondary role in the pathogenesis, contributing to progression of disease (63). Its role in the pathophysiology (irrespective of whether or not being pathogenic), makes uric acid a suitable candidate in the quest for prognostic biomarkers in PAH.
In both adults and children, increased uric acid levels have been shown to be associated with increased mortality (36,54,60,64). In the pediatric age group, uric acid has been comprehensively studied, and it has been demonstrated that uric acid levels carry prognostic value, not only at time of diagnosis, but also during the full course of the disease (65). Moreover, a gradual incline of uric acid over time was found to be associated with increased mortality, a finding that indicates that uric acid is useful as prognostic biomarker but can also be valuable in the evaluation of treatment efficacy. Closely monitoring uric acid levels and their profile over time provides important information on the course of the disease and may aid in clinical decision-making in the management of pediatric PAH. In adults with PAH, the use of uric acid serum levels is limited due to the frequent presence of comorbidities that affect purine metabolism such as gout, renal dysfunction and insulin resistance, decreasing its specificity (61,66). Since these comorbidities are significantly less frequent in children, uric acid serum level is an appropriate prognostic biomarker specific in the pediatric population.
Biochemical markers of neurohormonal activation
Sympathetic nervous system activity is increased in PAH (67). Ciarka et al. showed that the degree of sympathetic nervous system activation, directly measured by microneurography, is an independent predictor of mortality in adults with PAH (68). Serum levels of catecholamines such as norepinephrine and epinephrine are believed to reflect the degree of sympathetic nervous system activation, and have been shown to be increased in a subset of patients with PAH. Norepinephrine levels have been suggested to be associated with outcome in both children and adults with PAH (28,69), but for epinephrine the prognostic value is less clear (54). The small sample size in which the catecholamines are studied so far (hampering extensive multivariable or longitudinal modeling), currently limits conclusions regarding its usefulness as potential prognostic biomarkers.
Because of the central involvement of endothelin-1 (ET-1) in vascular remodeling and hence in the pathophysiology of PAH, ET-1 has long been considered a potential candidate prognostic biomarker in several cardiovascular diseases including PAH (70). Despite promising associations with disease severity in adults with PAH, ET-1 has not been adopted as a biomarker into clinical PAH practice (71). The main reasons are a lack of disease specificity, contradictory study findings and unpredictable treatment effects. A study on ET-1 in pediatric PAH did not show associations with disease severity or mortality, arguing against a potential role for ET-1 as a prognostic biomarker in PAH (72).
Inflammatory markers
In view of the important role of inflammation in the pathobiology of PAH, there is growing interest in inflammatory markers as potential prognostic biomarkers. For instance, circulating cytokines including interleukin-6 have been suggested to correlate with hemodynamic disease severity measurements in children with idiopathic and associated PAH and were demonstrated to identify patients at risk for various adverse outcome events (73). In a small experimental study using a proteomics approach, serum amyloid-A4 (an acute phase marker released in response to inflammatory stimuli), was higher in children with idiopathic PAH with poor outcome profile (defined as death, initiation of intravenous prostacyclin) compared to those with a more favorable outcome profile (74). Using a similar approach, serum levels of apolipoprotein-A1 have also been suggested to correlate with disease severity and outcome in 83 children with idiopathic and CHD-associated PAH (36). Plasma growth differentiation factor 15 is a stress responsive cytokine that has been shown to be elevated in children with CHD-associated PAH compared to controls, and to correlate with disease severity and hemodynamic measurements (75). Galectin-3 is secreted by immune cells that regulate inflammation, fibrogenesis, cell proliferation and tissue repair and high levels have been suggested to predict PH and correlate with echocardiographic disease severity measurements in children with ventricular septal defects (76). Although promising from a pathobiological perspective, all these inflammatory markers are not yet in routine clinical use and further investigations including prospective validations are warranted.
Other circulating serum biomarkers with potential prognostic value
Of the serum biomarkers, natriuretic peptides, uric acid, neurohormones and inflammatory markers have been studied most extensively in pediatric PAH and have therefore been discussed. There is a large set of other potential biomarkers under investigation of which some have also been suggested to carry prognostic value in pediatric PAH. Among others these include: markers of myocyte stress or injury (e.g., cardiac troponins, soluble ST2, midregional pro-adrenomedullin), markers of oxidative stress (other than uric acid), markers of RV extracellular matrix remodeling (e.g., matrix metalloproteinases and its tissue inhibitors), and several markers derived from high-througput “omics” technologies (e.g., genomics, transcriptomics, proteomics and metabolomics) (36,75,77,78). As some of these hold much promise as prognostic biomarkers in pediatric PAH, their usefulness requires further confirmation in well-defined and phenotyped cohorts of children with PAH.
Hemodynamic biomarkers
Cardiac catheterization is required to define PAH at time of diagnosis, and yields several hemodynamic biomarkers with prognostic value (4). The conventional hemodynamic biomarkers that have most frequently been shown to be associated with outcome in pediatric PAH include mean right atrial pressure, cardiac index and pulmonary vascular resistance index (28). Also, pulmonary-to-systemic arterial pressure ratio (mPAP/mSAP) is associated with mortality (19).
In addition to this, acute response to vasodilator testing has consistently been shown to be associated with improved survival (79,80). A comparison of several clinically used responder criteria in the TOPP registry has shown that transplant-free survival of responders according the so-called Sitbon criteria was superior, whereas children identified by alternative criteria had no better outcome compared to non-responders (80). Apitz et al. suggested a relationship between pulmonary endothelial function (by vasodilator response to acetylcholine administered in segmental arteries) and clinical outcome, indicating its potential as prognostic biomarker (81).
Cardiac catheterization in childhood requires sedation or general anesthesia and is accompanied by a, compared to adults with PAH, relatively high mortality risk of 0.6% and a risk for major complications of 1–3%, especially in young infants and those children in high WHO-functional class (82-84). Cardiac catheterization is the gold standard diagnostic modality in PAH, but the associated risks makes it less suitable for regular serial follow-up that is needed to monitor the disease over time.
Imaging biomarkers
Echocardiography
Echocardiography is a generally accessible non-invasive tool without the need for sedation or anesthesia and is well tolerated by children. This imaging modality not only allows for an assessment of the cardiac anatomy and morphology, but also allows for functional assessment of both ventricles and the pulmonary vasculature in pediatric PAH (85). However, accuracy and reproducibility of the measurements is limited due to operator-dependent acquisition and interpretation variability.
A wide range of echocardiographic measurements have been evaluated in adults and children with PAH, and a number of these qualify as prognostic biomarkers.
The tricuspid annular plane systolic excursion (TAPSE) measured in M-Mode in 4-chamber view reflects the degree of excursion of the tricuspid annulus towards the apex (86). This measurement of longitudinal RV function has been demonstrated one of the most robust predictors of disease severity and outcome (independent from age), and is one of the few prognostic biomarkers for which it was demonstrated that treatment-induced improvements are associated with improvements in survival (10,87).
The right-to-left ventricular dimension ratio, which is measured in the parasternal short-axis view at the level of the papillary muscles and combines information on the pressure-load induced RV enlargement with the degree of septal shift compressing the LV, has also been demonstrated to carry prognostic value (87,88). The reported prognostic value of echocardiographic variables measuring functional properties of both the RV and the LV, supports the emerging recognition that ventricular interdependency is an important determinant of outcome in PAH. Pulmonary artery acceleration time has been shown to be associated with disease severity and invasive hemodynamics, but has not been shown to carry prognostic value in children with PAH (89). An increased systolic to diastolic duration ratio as measured by echocardiography reflects the decrease in diastolic and increase in systolic duration due to increased RV afterload and has been linked to mortality in a child with PAH, but could not be consistently reproduced in other pediatric cohorts (87,90).
More advanced echocardiographic techniques have also been suggested to be valuable in the assessment of children with PAH. Right ventricular free wall longitudinal strain assessed by speckle tracking echocardiography has been shown to be decreased in children with PAH, and to be associated with clinical outcomes (91-94). This assessment is suggested to be better reproducible with lower inter-/intra-observer variability compared to other echocardiographic variables and better suited for serial follow-up (92,95,96). Other emerging echocardiographic techniques that are explored in pediatric PAH include 3D-echocardiography and 4D flow profiles (94,97). Follow-up studies in larger well-defined cohorts of children with PAH are expected to yield essential information on the usefulness of these new echocardiographic measurements as prognostic biomarkers.
Cardiac magnetic resonance (CMR)
CMR imaging is a safe and non-invasive imaging modality and yields reliable and reproducible measurements of right ventricular and pulmonary arterial structure and function. Compared to echocardiography, images are more reproducible, and 3D measurements within the complex anatomical right ventricular configuration allow for better assessments of volumes and tissue masses.
Conventional CMR measurements that have been shown to be associated with disease severity and mortality in both children and adults with PAH include the following: RV volumes, RA and RV dimensions, RV mass, RV ejection fraction, LV stroke volume and tricuspid regurgitation fraction (20,98,99). These measurements may be used as prognostic biomarkers. In adults with PAH, changes in RV functional CMR measurements have been shown to correlate with outcome, suggesting that such measurements might serve as survival surrogates and can be used in defining treatment goals. This remains to be demonstrated for children with PAH.
In addition to conventional measurements, CMR allows for the assessment of a wide range of cardiovascular functional properties, including systemic and pulmonary blood flow profiles, global and regional myocardial mechanics, interventricular (dys-)synchrony, pulmonary arterial wall dynamics and RV to pulmonary arterial interaction assessments (99,100). All of these may hold promise for the evaluation of the severity of PAH and the adaptive capacity of the heart, both in adults and children. CMR feature tracking techniques are emerging as accurate and reproducible ways for the comprehensive evaluation of myocardial fiber deformation, yielding parameters such as strain, strain rate and mechanical dispersion (101). These CMR biomarkers are under current investigation in cohorts of children with PAH.
It has been argued that CMR is not feasible in small children without sedation or anesthesia as they cannot hold their breath, however, acquisition of real time sequences during free breathing has recently been shown to be feasible even in the youngest awake children (20,102). Disadvantages of CMR are that it requires specific expertise and that this modality is not yet generally available on a global scale. However, in view of the extensive and expanding possibilities, CMR is progressively establishing its role in the evaluation of children with PAH.
Clinical implications
The reviewed prognostic biomarkers reflect different aspects of the disease process and functional implications of the disease. In day-to-day clinical practice, assessment of disease severity and evaluation of treatment efficacy is not based on the result of one of the reviewed prognostic biomarkers, but on the interpretation of the composite result of multiple clinical measurements that also include parameters reflecting how patients feel and function (that might not carry direct prognostic value in terms of mortality risk).
During serial editions of the World Symposia on PH (WSPH), the pediatric task force has proposed (and updated) a WSPH pediatric risk assessment tool in which a set of “risk criteria” are defined on the basis of expert consensus supported by the emerging evidence from observational studies (4). The prognostic value of this risk assessment tool has recently been evaluated in a national cohort of pediatric patients with PAH (103). The results demonstrated that the number of low-risk criteria, as defined in the WSPH pediatric risk stratification tool, present at diagnosis indeed accurately predicted the risk of mortality in children with idiopathic or heritable PAH. To illustrate, the recommended low risk criteria are listed in Figure 1. Reaching or preserving a low-risk profile during follow-up was associated with improved survival, suggesting that this low-risk state could be strived for as a multidimensional treatment goal in pediatric PAH.
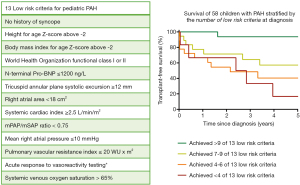
Concluding remarks
Assessment of prognosis is key in designing an optimal and personalized treatment strategy for the individual child with PAH. In recent years, multiple potential prognostic biomarkers have become available for the management of PAH in children. As the available prognostic biomarkers reflect different aspects of the disease process and functional implications, a multi-marker approach appears the most useful for guiding therapy decisions and improve outcome in pediatric PAH.
Acknowledgments
Funding: None.
Footnote
Provenance and Peer Review: This article was commissioned by the Guest Editors (Christian Apitz and Astrid Lammers) for the series “Pediatric Pulmonary Hypertension” published in Cardiovascular Diagnosis and Therapy. The article has undergone external peer review.
Conflicts of Interest: Both authors have completed the ICMJE uniform disclosure form (available at http://dx.doi.org/10.21037/cdt-20-374). The series “Pediatric Pulmonary Hypertension” was commissioned by the editorial office without any funding or sponsorship. RMFB reports other from Actelion, other from Lilly, other from GSK, other from Pfizer, outside the submitted work. MJP has no conflicts of interest to declare.
Ethical Statement: The authors are accountable for all aspects of the work in ensuring that questions related to the accuracy or integrity of any part of the work are appropriately investigated and resolved.
Open Access Statement: This is an Open Access article distributed in accordance with the Creative Commons Attribution-NonCommercial-NoDerivs 4.0 International License (CC BY-NC-ND 4.0), which permits the non-commercial replication and distribution of the article with the strict proviso that no changes or edits are made and the original work is properly cited (including links to both the formal publication through the relevant DOI and the license). See: https://creativecommons.org/licenses/by-nc-nd/4.0/.
References
- Schermuly RT, Ghofrani HA, Wilkins MR, et al. Mechanisms of disease: pulmonary arterial hypertension. Nat Rev Cardiol 2011;8:443-55. [Crossref] [PubMed]
- Galiè N, Channick RN, Frantz RP, et al. Risk stratification and medical therapy of pulmonary arterial hypertension. Eur Respir J 2019;53:1801889 [Crossref] [PubMed]
- Zijlstra WMH, Douwes JM, Rosenzweig EB, et al. Survival differences in pediatric pulmonary arterial hypertension: clues to a better understanding of outcome and optimal treatment strategies. J Am Coll Cardiol 2014;63:2159-69. [Crossref] [PubMed]
- Rosenzweig EB, Abman SH, Adatia I, et al. Paediatric pulmonary arterial hypertension: updates on definition, classification, diagnostics and management. Eur Respir J 2019;53:1801916 [Crossref] [PubMed]
- Zijlstra WMH, Ploegstra MJ, Berger RMF. Current and advancing treatments for pulmonary arterial hypertension in childhood. Expert Rev Respir Med 2014;8:615-28. [Crossref] [PubMed]
- Robb MA, McInnes PM, Califf RM. Biomarkers and Surrogate Endpoints: Developing Common Terminology and Definitions. JAMA 2016;315:1107-8. [Crossref] [PubMed]
- Fleming TR, Powers JH. Biomarkers and surrogate endpoints in clinical trials. Stat Med 2012;31:2973-84. [Crossref] [PubMed]
- Fleming TR, DeMets DL. Surrogate end points in clinical trials: are we being misled? Ann Intern Med 1996;125:605-13. [Crossref] [PubMed]
- Ploegstra MJ, Berger RMF. What’s the (end) point? Eur Respir J 2015;45:854-5. [Crossref] [PubMed]
- Ploegstra MJ, Douwes JM, Roofthooft MTR, et al. Identification of treatment goals in paediatric pulmonary arterial hypertension. Eur Respir J 2014;44:1616-26. [Crossref] [PubMed]
- Hoeper MM, Markevych I, Spiekerkoetter E, et al. Goal-oriented treatment and combination therapy for pulmonary arterial hypertension. Eur Respir J 2005;26:858-63. [Crossref] [PubMed]
- Nauman J, Janszky I, Vatten LJ, et al. Temporal changes in resting heart rate and deaths from ischemic heart disease. JAMA 2011;306:2579-87. [Crossref] [PubMed]
- Eckberg DL, Drabinsky M, Braunwald E. Defective cardiac parasympathetic control in patients with heart disease. N Engl J Med 1971;285:877-83. [Crossref] [PubMed]
- Arnold JM, Fitchett DH, Howlett JG, et al. Resting heart rate: a modifiable prognostic indicator of cardiovascular risk and outcomes? Can J Cardiol 2008;24 Suppl A:3A-8A.
- Andersen S, Andersen A, de Man FS, et al. Sympathetic nervous system activation and β-adrenoceptor blockade in right heart failure. Eur J Heart Fail 2015;17:358-66. [Crossref] [PubMed]
- Ren X, Johns RA, Gao WD. EXPRESS: Right Heart in Pulmonary Hypertension: From Adaptation to Failure. Pulm Circ 2019;9:2045894019845611 [Crossref] [PubMed]
- Hildenbrand FF, Fauchère I, Huber LC, et al. A low resting heart rate at diagnosis predicts favourable long-term outcome in pulmonary arterial and chronic thromboembolic pulmonary hypertension. A prospective observational study. Respir Res 2012;13:76. [Crossref] [PubMed]
- Henkens IR, Van Wolferen SA, Gan CTJ, et al. Relation of Resting Heart Rate to Prognosis in Patients With Idiopathic Pulmonary Arterial Hypertension. Am J Cardiol 2009;103:1451-6. [Crossref] [PubMed]
- Douwes JM, Roofthooft MTR, Bartelds B, et al. Pulsatile haemodynamic parameters are predictors of survival in paediatric pulmonary arterial hypertension. Int J Cardiol 2013;168:1370-7. [Crossref] [PubMed]
- Moledina S, Pandya B, Bartsota M, et al. Prognostic significance of cardiac magnetic resonance imaging in children with pulmonary hypertension. Circ Cardiovasc Imaging 2013;6:407-14. [Crossref] [PubMed]
- Latus H, Bandorski D, Rink F, et al. Heart Rate Variability is Related to Disease Severity in Children and Young Adults with Pulmonary Hypertension. Front Pediatr 2015;3:63. [Crossref] [PubMed]
- Lammers AE, Munnery E, Hislop A, et al. Heart rate variability predicts outcome in children with pulmonary arterial hypertension. Int J Cardiol 2010;142:159-65. [Crossref] [PubMed]
- Daymont C, Neal A, Prosnitz A, et al. Growth in children with congenital heart disease. Pediatrics 2013;131:e236-42. [Crossref] [PubMed]
- Moledina S, Hislop AA, Foster H, et al. Childhood idiopathic pulmonary arterial hypertension: a national cohort study. Heart 2010;96:1401-6. [Crossref] [PubMed]
- Ploegstra MJ, Ivy DD, Wheeler JG, et al. Growth in children with pulmonary arterial hypertension: a longitudinal retrospective multiregistry study. Lancet Respir Med 2016;4:281-90. [Crossref] [PubMed]
- van Loon RLE, Roofthooft MTR, Delhaas T, et al. Outcome of pediatric patients with pulmonary arterial hypertension in the era of new medical therapies. Am J Cardiol 2010;106:117-24. [Crossref] [PubMed]
- Khirfan G, Naal T, Abuhalimeh B, et al. Hypoxemia in patients with idiopathic or heritable pulmonary arterial hypertension. PLoS One 2018;13:e0191869 [Crossref] [PubMed]
- Ploegstra MJ, Zijlstra WMH, Douwes JM, et al. Prognostic factors in pediatric pulmonary arterial hypertension: A systematic review and meta-analysis. Int J Cardiol 2015;184:198-207. [Crossref] [PubMed]
- Lammers AE, Adatia I, Cerro MJ, Del , et al. Functional classification of pulmonary hypertension in children: Report from the PVRI pediatric taskforce, Panama 2011. Pulm Circ 2011;1:280-5. [Crossref] [PubMed]
- Balkin EM, Olson ED, Robertson L, et al. Change in Pediatric Functional Classification During Treatment and Morbidity and Mortality in Children with Pulmonary Hypertension. Pediatr Cardiol 2016;37:756-64. [Crossref] [PubMed]
- ATS Committee on Proficiency Standards for Clinical Pulmonary Function Laboratories. ATS statement: guidelines for the six-minute walk test. Am J Respir Crit Care Med 2002;166:111-7. [Crossref] [PubMed]
- Parikh KS, Rajagopal S, Arges K, et al. Use of outcome measures in pulmonary hypertension clinical trials. Am Heart J 2015;170:419-29.e3. [Crossref] [PubMed]
- Douwes JM, Hegeman AK, van der Krieke-van der Horst M, et al. Six-minute walk-test in childhood pulmonary arterial hypertension: Walking distance and decrease in oxygen saturation provide additional prognostic information. Eur Heart J 2014;35:174-5.
- Lammers AE, Diller GP, Odendaal D, et al. Comparison of 6-min walk test distance and cardiopulmonary exercise test performance in children with pulmonary hypertension. Arch Dis Child 2011;96:141-7. [Crossref] [PubMed]
- Rausch CM, Taylor AL, Ross H, et al. Ventilatory efficiency slope correlates with functional capacity, outcomes, and disease severity in pediatric patients with pulmonary hypertension. Int J Cardiol 2013;169:445-8. [Crossref] [PubMed]
- Wagner BD, Takatsuki S, Accurso FJ, et al. Evaluation of circulating proteins and hemodynamics towards predicting mortality in children with pulmonary arterial hypertension. PLoS One 2013;8:e80235 [Crossref] [PubMed]
- Farina S, Correale M, Bruno N, et al. The role of cardiopulmonary exercise tests in pulmonary arterial hypertension. Eur Respir Rev 2018;27:170134 [Crossref] [PubMed]
- Barst RJ, Ivy DD, Gaitan G, et al. A randomized, double-blind, placebo-controlled, dose-ranging study of oral sildenafil citrate in treatment-naive children with pulmonary arterial hypertension. Circulation 2012;125:324-34. [Crossref] [PubMed]
- Pugh ME, Buchowski MS, Robbins IM, et al. Physical activity limitation as measured by accelerometry in pulmonary arterial hypertension. Chest 2012;142:1391-8. [Crossref] [PubMed]
- Zijlstra WMH, Ploegstra MJ, Vissia-Kazemier T, et al. Physical Activity in Pediatric Pulmonary Arterial Hypertension Measured by Accelerometry. A Candidate Clinical Endpoint. Am J Respir Crit Care Med 2017;196:220-7. [Crossref] [PubMed]
- Ollivier C, Sun H, Amchin W, et al. New Strategies for the Conduct of Clinical Trials in Pediatric Pulmonary Arterial Hypertension: Outcome of a Multistakeholder Meeting With Patients, Academia, Industry, and Regulators, Held at the European Medicines Agency on Monday, June 12, 2017. J Am Heart Assoc 2019;8:e011306 [Crossref] [PubMed]
- Lau KC, Frank DB, Hanna BD, et al. Utility of electrocardiogram in the assessment and monitoring of pulmonary hypertension (idiopathic or secondary to pulmonary developmental abnormalities) in patients≤18 years of age. Am J Cardiol 2014;114:294-9. [Crossref] [PubMed]
- Sawada H, Mitani Y, Nakayama T, et al. Detection of Pediatric Pulmonary Arterial Hypertension by School Electrocardiography Mass Screening. Am J Respir Crit Care Med 2019;199:1397-406. [Crossref] [PubMed]
- Berger RMF, Beghetti M. Early Diagnosis in Pulmonary Arterial Hypertension: The Search for the Holy Grail. Am J Respir Crit Care Med 2019;199:1306-7. [Crossref] [PubMed]
- Bossone E, Paciocco G, Iarussi D, et al. The prognostic role of the ECG in primary pulmonary hypertension. Chest 2002;121:513-8. [Crossref] [PubMed]
- Cheng XL, He JG, Liu ZH, et al. The Value of the Electrocardiogram for Evaluating Prognosis in Patients with Idiopathic Pulmonary Arterial Hypertension. Lung 2017;195:139-46. [Crossref] [PubMed]
- Waligóra M, Tyrka A, Podolec P, et al. ECG Markers of Hemodynamic Improvement in Patients with Pulmonary Hypertension. Biomed Res Int 2018;2018:4606053 [Crossref] [PubMed]
- Henkens IR, Gan CT-J, van Wolferen SA, et al. ECG monitoring of treatment response in pulmonary arterial hypertension patients. Chest 2008;134:1250-7. [Crossref] [PubMed]
- Sato S, Ogawa A, Matsubara H. Change in R wave in lead V1 predicts survival of patients with pulmonary arterial hypertension. Pulm Circ 2018;8:2045894018776496 [Crossref] [PubMed]
- Krämer J, Kreuzer F, Kaestner M, et al. Impact of the Right Ventricular Sokolow-Lyon Index in Children with Idiopathic Pulmonary Arterial Hypertension. Pediatr Cardiol 2018;39:1115-22. [Crossref] [PubMed]
- King L, Wilkins MR. Natriuretic peptide receptors and the heart. Heart 2002;87:314-5. [Crossref] [PubMed]
- Gan CT, McCann GP, Marcus JT, et al. NT-proBNP reflects right ventricular structure and function in pulmonary hypertension. Eur Respir J 2006;28:1190-4. [Crossref] [PubMed]
- Bernus A, Wagner BD, Accurso F, et al. Brain natriuretic peptide levels in managing pediatric patients with pulmonary arterial hypertension. Chest 2009;135:745-51. [Crossref] [PubMed]
- Van Albada ME, Loot FG, Fokkema R, et al. Biological serum markers in the management of pediatric pulmonary arterial hypertension. Pediatr Res 2008;63:321-7. [Crossref] [PubMed]
- Takatsuki S, Wagner BD, Ivy DD. B-type Natriuretic Peptide and Amino-terminal Pro-B-type Natriuretic Peptide in Pediatric Patients with Pulmonary Arterial Hypertension. Congenit Heart Dis 2012;7:259-67. [Crossref] [PubMed]
- Said F, Haarman MG, Roofthooft MTR, et al. Serial Measurements of N-Terminal Pro-B-Type Natriuretic Peptide Serum Level for Monitoring Pulmonary Arterial Hypertension in Children. J Pediatr 2020;220:139-45. [Crossref] [PubMed]
- Hoeper MM, Hohlfeld H, Fabel H. Hyperuricaemia in patients with right or left heart failure. Eur Respir J 1999;13:682-5. [Crossref] [PubMed]
- Oya H, Nagaya N, Satoh T, et al. Haemodynamic correlates and prognostic significance of serum uric acid in adult patients with Eisenmenger syndrome. Heart 2000;84:53-8. [Crossref] [PubMed]
- Voelkel MA, Wynne KM, Badesch DB, et al. Hyperuricemia in severe pulmonary hypertension. Chest 2000;117:19-24. [Crossref] [PubMed]
- Nagaya N, Uematsu M, Satoh T, et al. Serum uric acid levels correlate with the severity and the mortality of primary pulmonary hypertension. Am J Respir Crit Care Med 1999;160:487-92. [Crossref] [PubMed]
- Leyva F, Anker S, Swan JW, et al. Serum uric acid as an index of impaired oxidative metabolism in chronic heart failure. Eur Heart J 1997;18:858-65. [Crossref] [PubMed]
- McCord JM. Oxygen-derived free radicals in postischemic tissue injury. N Engl J Med 1985;312:159-63. [Crossref] [PubMed]
- Zharikov SI, Swenson ER, Lanaspa M, et al. Could uric acid be a modifiable risk factor in subjects with pulmonary hypertension? Med Hypotheses 2010;74:1069-74. [Crossref] [PubMed]
- Bendayan D, Shitrit D, Ygla M, et al. Hyperuricemia as a prognostic factor in pulmonary arterial hypertension. Respir Med 2003;97:130-3. [Crossref] [PubMed]
- Leberkühne LJ, Ploegstra MJ, Douwes JM, et al. Serially Measured Uric Acid Levels Predict Disease Severity and Outcome in Pediatric Pulmonary Arterial Hypertension. Am J Respir Crit Care Med 2017;195:401-4. [PubMed]
- Anker SD, Doehner W, Rauchhaus M, et al. Uric acid and survival in chronic heart failure: validation and application in metabolic, functional, and hemodynamic staging. Circulation 2003;107:1991-7. [Crossref] [PubMed]
- de Man FS, Handoko ML, Guignabert C, et al. Neurohormonal axis in patients with pulmonary arterial hypertension: friend or foe? Am J Respir Crit Care Med 2013;187:14-9. [Crossref] [PubMed]
- Ciarka A, Doan V, Velez-Roa S, et al. Prognostic significance of sympathetic nervous system activation in pulmonary arterial hypertension. Am J Respir Crit Care Med 2010;181:1269-75. [Crossref] [PubMed]
- Maron BA, Leopold JA. Emerging Concepts in the Molecular Basis of Pulmonary Arterial Hypertension: Part II: Neurohormonal Signaling Contributes to the Pulmonary Vascular and Right Ventricular Pathophenotype of Pulmonary Arterial Hypertension. Circulation 2015;131:2079-91. [Crossref] [PubMed]
- Giaid A, Yanagisawa M, Langleben D, et al. Expression of endothelin-1 in the lungs of patients with pulmonary hypertension. N Engl J Med 1993;328:1732-9. [Crossref] [PubMed]
- Rubens C, Ewert R, Halank M, et al. Big endothelin-1 and endothelin-1 plasma levels are correlated with the severity of primary pulmonary hypertension. Chest 2001;120:1562-9. [Crossref] [PubMed]
- Latus H, Karanatsios G, Basan U, et al. Clinical and prognostic value of endothelin-1 and big endothelin-1 expression in children with pulmonary hypertension. Heart 2016;102:1052-8. [Crossref] [PubMed]
- Duncan M, Wagner BD, Murray K, et al. Circulating cytokines and growth factors in pediatric pulmonary hypertension. Mediators Inflamm 2012;2012:143428 [Crossref] [PubMed]
- Yeager ME, Colvin KL, Everett AD, et al. Plasma proteomics of differential outcome to long-term therapy in children with idiopathic pulmonary arterial hypertension. Proteomics Clin Appl 2012;6:257-67. [Crossref] [PubMed]
- Li G, Li Y, Tan XQ, et al. Plasma Growth Differentiation Factor-15 is a Potential Biomarker for Pediatric Pulmonary Arterial Hypertension Associated with Congenital Heart Disease. Pediatr Cardiol 2017;38:1620-6. [Crossref] [PubMed]
- Salem Zayed KM, Abdelhakeem AM, Elhady M, et al. Novel biomarkers for pulmonary hypertension in children with ventricular septal defect. Egypt Pediatr Assoc Gaz 2016;64:61-8. [Crossref]
- Chida A, Sato H, Shintani M, et al. Soluble ST2 and N-terminal pro-Brain Natriuretic Peptide Combination. Circ J 2014;78:436-42. [Crossref] [PubMed]
- Welch CL, Chung WK. Genetics and Other Omics in Pediatric Pulmonary Arterial Hypertension. Chest 2020;157:1287-95. [Crossref] [PubMed]
- Barst RJ, Maislin G, Fishman AP. Vasodilator Therapy for Primary Pulmonary Hypertension in Children. Circulation 1999;99:1197-208. [Crossref] [PubMed]
- Douwes JM, Humpl T, Bonnet D, et al. Acute Vasodilator Response in Pediatric Pulmonary Arterial Hypertension: Current Clinical Practice From the TOPP Registry. J Am Coll Cardiol 2016;67:1312-23. [Crossref] [PubMed]
- Apitz C, Zimmermann R, Kreuder J, et al. Assessment of pulmonary endothelial function during invasive testing in children and adolescents with idiopathic pulmonary arterial hypertension. J Am Coll Cardiol 2012;60:157-64. [Crossref] [PubMed]
- Zuckerman WA, Turner ME, Kerstein J, et al. Safety of cardiac catheterization at a center specializing in the care of patients with pulmonary arterial hypertension. Pulm Circ 2013;3:831-9. [Crossref] [PubMed]
- O’Byrne ML, Kennedy KF, Kanter JP, et al. Risk Factors for Major Early Adverse Events Related to Cardiac Catheterization in Children and Young Adults With Pulmonary Hypertension: An Analysis of Data From the IMPACT (Improving Adult and Congenital Treatment) Registry. J Am Heart Assoc 2018;7:e008142 [Crossref] [PubMed]
- Beghetti M, Schulze-Neick I, Berger RMF, et al. Haemodynamic characterisation and heart catheterisation complications in children with pulmonary hypertension: Insights from the Global TOPP Registry (tracking outcomes and practice in paediatric pulmonary hypertension). Int J Cardiol 2016;203:325-30. [Crossref] [PubMed]
- Koestenberger M, Friedberg MK, Nestaas E, et al. Transthoracic echocardiography in the evaluation of pediatric pulmonary hypertension and ventricular dysfunction. Pulm Circ 2016;6:15-29. [Crossref] [PubMed]
- Forfia PR, Fisher MR, Mathai SC, et al. Tricuspid Annular Displacement Predicts Survival in Pulmonary Hypertension. Am J Respir Crit Care Med 2006;174:1034-41. [Crossref] [PubMed]
- Ploegstra MJ, Roofthooft MTR, Douwes JM, et al. Echocardiography in pediatric pulmonary arterial hypertension: early study on assessing disease severity and predicting outcome. Circ Cardiovasc Imaging 2014;8:e000878 [PubMed]
- Jone P-N, Hinzman J, Wagner BD, et al. Right ventricular to left ventricular diameter ratio at end-systole in evaluating outcomes in children with pulmonary hypertension. J Am Soc Echocardiogr 2014;27:172-8. [Crossref] [PubMed]
- Levy PT, Patel MD, Groh G, et al. Pulmonary Artery Acceleration Time Provides a Reliable Estimate of Invasive Pulmonary Hemodynamics in Children. J Am Soc Echocardiogr 2016;29:1056-65. [Crossref] [PubMed]
- Alkon J, Humpl T, Manlhiot C, et al. Usefulness of the right ventricular systolic to diastolic duration ratio to predict functional capacity and survival in children with pulmonary arterial hypertension. Am J Cardiol 2010;106:430-6. [Crossref] [PubMed]
- Haeck MLA, Scherptong RWC, Marsan NA, et al. Prognostic value of right ventricular longitudinal peak systolic strain in patients with pulmonary hypertension. Circ Cardiovasc Imaging 2012;5:628-36. [Crossref] [PubMed]
- Okumura K, Humpl T, Dragulescu A, et al. Longitudinal assessment of right ventricular myocardial strain in relation to transplant-free survival in children with idiopathic pulmonary hypertension. J Am Soc Echocardiogr 2014;27:1344-51. [Crossref] [PubMed]
- Muntean I, Benedek T, Melinte M, et al. Deformation pattern and predictive value of right ventricular longitudinal strain in children with pulmonary arterial hypertension. Cardiovasc Ultrasound 2016;14:27. [Crossref] [PubMed]
- Jone PN, Schäfer M, Pan Z, et al. 3D echocardiographic evaluation of right ventricular function and strain: a prognostic study in paediatric pulmonary hypertension. Eur Heart J Cardiovasc Imaging 2018;19:1026-33. [Crossref] [PubMed]
- Karsenty C, Hadeed K, Dulac Y, et al. Two-dimensional right ventricular strain by speckle tracking for assessment of longitudinal right ventricular function after paediatric congenital heart disease surgery. Arch Cardiovasc Dis 2017;110:157-66. [Crossref] [PubMed]
- Levy PT, Holland MR, Sekarski TJ, et al. Feasibility and reproducibility of systolic right ventricular strain measurement by speckle-tracking echocardiography in premature infants. J Am Soc Echocardiogr 2013;26:1201-13. [Crossref] [PubMed]
- Jone P-N, Schäfer M, Pan Z, et al. Right Ventricular-Arterial Coupling Ratio Derived From 3-Dimensional Echocardiography Predicts Outcomes in Pediatric Pulmonary Hypertension. Circ Cardiovasc Imaging 2019;12:e008176 [Crossref] [PubMed]
- Baggen VJM, Leiner T, Post MC, et al. Cardiac magnetic resonance findings predicting mortality in patients with pulmonary arterial hypertension: a systematic review and meta-analysis. Eur Radiol 2016;26:3771-80. [Crossref] [PubMed]
- Frank BS, Schäfer M, Douwes JM, et al. Novel measures of left ventricular electromechanical discoordination predict clinical outcomes in children with pulmonary arterial hypertension. Am J Physiol Heart Circ Physiol 2020;318:H401-12. [Crossref] [PubMed]
- Latus H, Kuehne T, Beerbaum P, et al. Cardiac MR and CT imaging in children with suspected or confirmed pulmonary hypertension/pulmonary hypertensive vascular disease. Expert consensus statement on the diagnosis and treatment of paediatric pulmonary hypertension. The European Paediatric Pulmo. Heart 2016;102:ii30-5. [Crossref] [PubMed]
- Muser D, Castro SA, Santangeli P, et al. Clinical applications of feature-tracking cardiac magnetic resonance imaging. World J Cardiol 2018;10:210-21. [Crossref] [PubMed]
- Pandya B, Quail M, Steeden J, et al. Real-time magnetic resonance assessment of septal curvature accurately tracks acute hemodynamic changes in pediatric pulmonary hypertension. Circ Cardiovasc Imaging 2014;7:706-13. [Crossref] [PubMed]
- Haarman MG, Douwes JM, Ploegstra MJ, et al. The Clinical Value of Proposed Risk Stratification Tools in Pediatric Pulmonary Arterial Hypertension. Am J Respir Crit Care Med 2019;200:1312-5. [Crossref] [PubMed]