Molecular mechanisms of vascular dysfunction and cardiovascular biomarkers in type 2 diabetes
Cardiovascular disease burden in patients with type 2 diabetes (T2DM)
A pandemic of metabolic diseases
Prevalence of obesity and T2DM is alarmingly increasing worldwide due to sedentary lifestyles, high fat diet regimens and genetic predisposition (1,2). One third of the global population is overweight and one fifth is clearly obese, with a very high risk of developing diabetes over the upcoming years. The International Diabetes Federation currently estimates that 382 million people are affected by T2DM, with an age-adjusted prevalence of the disease ranging from 6% to 37.5% in the adult population (3). Noteworthy, recent projections forecast a 32% increase of T2DM prevalence in Europe, 72% in the United States and 160% in developing countries, with about 439 million of diabetic individuals by the year 2030 (7% of the global population) (4). Beside these strong epidemiological data, thoughtful genetic and epigenetic analyses are revealing that gene-activating events occurring in obese and T2DM subjects are transmitted to the offspring (5,6). Inheritance of these modifications may anticipate disease phenotypes already in young, normoweight individuals. Therefore, transmissions of metabolic signatures over the next generations implicate an exponential increase of obesity-related disorders, with a further rise of morbidity and mortality (7).
Cardiovascular prevention in T2DM patients
Seminal epidemiological observations have shown a strong association between T2DM and risk of cardiovascular events (8). Indeed, T2DM is associated with an increased risk of micro- and macrovascular complications and an approximate two-fold greater risk of mortality as compared with the general population (9). Albeit advances in therapy have reduced morbidity and mortality in T2DM, cardiovascular risk is far to be eradicated. Recent randomized studies have shown that intensive control of cardiovascular risk factors is not always associated with reduced cardiovascular endpoints in diabetic subjects (10). Moreover when benefits are observed, these may not last over the next years. In the ADVANCE trial (Action in Diabetes and Vascular Disease: Preterax and Diamicron MR Controlled Evaluation) intensive glycemic control led to a 21% reduction in the risk of nephropathy, as assessed by microalbuminuria (11). However, restoration of normoglycemia did not affect the risk of retinopathy and, most importantly, did not reduce the occurrence of macrovascular events. Along the same line, tight blood pressure control with the combination perindopril/indapamide reduced renal events without affecting other microvascular and macrovascular complications (11). The ACCORD study (Action to Control Cardiovascular Risk in Diabetes) significantly contributed to raise confusion and scepticism about benefits of intensive treatment strategies (12). This randomized trial was indeed prematurely stopped because of an excess of cardiovascular events in the intensive blood glucose control arm. In contrast with these recent studies, previous evidence from the STENO-2 trial showed that an intensive, multifactorial intervention with glucose-lowering drugs, anti-hypertensive agents and statins was beneficial (13). Unfortunately, such benefit is negligible when compared with the residual risk of microvascular complications observed during long-term follow-up (10,14). Indeed, over the 7.8 years treatment period, 51% of intensively treated patients developed or showed progression of diabetic retinophathy, nephropathy (25%) and peripheral neuropathy (55%) (10). Unexpectedly, new microvascular complications developed and progressed during the extended follow-up period, despite an optimal control of blood pressure values (131±13 and 73±11 vs. 146±18 vs. 78±10 mmHg) and Hb1Ac (7.9%±1.2% vs. 9.0%±1.8%) in the intensive as compared with conventional treatment group, respectively (14). Residual vascular risk remains elevated also after consistent low density lipoprotein (LDL) reduction by statins, likely due to the large prevalence of atherogenic dyslipidemia in T2DM (15). Indeed, high triglycerides and low high density lipoprotein (HDL) cholesterol are important determinants of cardiovascular risk in these patients. However, pharmacological approaches modulating other lipid components failed to reduce cardiovascular outcomes, as observed in the FIELD study (16) and, more recently, in the ACCORD (17) and AIM-HIGH (18) trials. Taken together, these results suggest that cardiovascular complications progress even after intensive control of risk factor clustering in the diabetic patients. In this perspective, deciphering novel molecular networks will be instrumental to develop novel diagnostic and therapeutic strategies in this setting.
Mechanisms of diabetic vascular dysfunction
Over the last 20 years, basic and translational studies have unravelled a strong biological relation between high glucose levels, impaired insulin signalling and vascular disease in the setting of T2DM (19). However, despite these investigations provided key mechanistic insights, it remains hard to distinguish the detrimental effects of hyperglycemia and insulin resistance on the diabetic endothelium and, more in general, in the vessel wall (2). The peculiarity of T2DM consists in the fact that the disease simultaneously manifests with a cluster of conditions including low-grade inflammation, impaired insulin pathway and hyperglycemia (20,21). This scenario makes the approach much more complex than type 1 diabetes (T1DM) when high glucose levels represent the major driver of vascular dysfunction.
Redox-sensitive pathways in the diabetic endothelium
Recent mechanistic studies performed in endothelial cells isolated from T2DM subjects have shown the activation of detrimental biochemical pathways favouring mitochondrial disruption and apoptosis (22,23). A well-established theory on the pathophysiology of vascular diabetic complications claims that reactive oxygen species (ROS) are upstream regulators of complex molecular networks ensuing in endothelial dysfunction (24). Seminal studies have indeed demonstrated that in patients with diabetes, hyperglycemia leads to accumulation of mitochondrial ROS and subsequent activation of advanced glycation end products (AGEs), protein kinase C (PKC), nuclear factor-kB (NF-κB), polyol and hexosamine flux (25) (Figure 1). The hyperglycemic environment induces a chronic elevation of diacyglycerol levels in endothelial cells with subsequent membrane translocation of conventional (α, β1, β2) and non-conventional (δ) PKC isoforms. We and others have shown that PKCβ2 isoform is highly activated in the diabetic endothelium and correlates with oxidative stress, impaired insulin signalling and, most importantly, endothelial dysfunction assessed by flow-mediated vasodilation (23,26). Once triggered, PKC β2 perpetuates its maladaptive signalling through the mitochondrial adaptor p66Shc and NADPH oxidase subunit p47(phox), two major enzymatic sources of free radicals (19) (Figure 1). Indeed, glucose-induced activation of PKC β2 isoform phosphorylates p66Shc at serine 36 leading to its translocation to the mitochondria, cytochrome c oxidation and accumulation of ROS into the organelle (27,28). Furthermore, hyperglycemia-induced activation of PKC increases superoxide production via NADPH oxidase. This latter mechanism is supported by the notion that treatment with a PKCβ inhibitor suppresses NADPH-dependent ROS generation in the human endothelium (29). A previous study from our group has shown that PKCβ is also a master regulator of NF-κB signalling in hyperglycemic conditions. Indeed, PKCβ2 activation reduces protein level of the inhibitory subunit of nuclear factor-kappaB (IkBα) thus enabling NF-κB-driven transcription of VCAM-1 (30). Indeed, selective PKCβ2 inhibition abolished IkBα degradation thus preventing hyperglycemia-induced endothelial inflammation. Taken together, evidence reported so far regards PKC as the upstream regulator of hyperglycemic damage as well as of impaired insulin signalling (27).
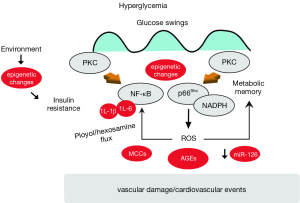
Mitochondrial adaptor p66Shc in T2DM: can we switch it off?
The adaptor p66Shc is a redox enzyme implicated in mitochondrial ROS generation and translation of oxidative signals into apoptosis (31,32). We have previously reported that genetic deletion of p66Shc protects against hyperglycemia-induced endothelial dysfunction and oxidative stress in mice (33). The relevance of p66Shc in the clinical setting of diabetes is supported by the notion that p66Shc gene expression is increased in peripheral blood mononuclear cells obtained from patients with T2DM and correlates with plasma isoprostane levels, a reliable in vivo marker of oxidative stress (34). In addition, our recent work has demonstrated that hyperglycemia-induced p66Shc upregulation is not reverted by intensive glycemic control in diabetic mice and contributes to persistent oxidative damage and vascular dysfunction via an intricate vicious cycle involving ROS, epigenetic changes and PKC activation (26) (Figure 1). Interestingly enough, in vivo gene silencing of p66Shc, performed at the time of normoglycemia restoration with insulin, was able to blunt persistent endothelial dysfunction, indicating that p66Shc is an important source of free radicals involved in the “metabolic memory” phenomenon (35,36) (Figure 1). Therefore, our work suggested that switching off p66Shc gene may be a promising option to rescue ongoing endothelial damage in diabetes. These mechanistic insights might indeed contribute to understand why glycemic control failed to improve cardiovascular events in diabetic patients.
Glucose swings: drivers of maladaptive endothelial signalling
An important determinant of vascular damage in T2DM is represented by glycemic fluctuations (37). Indeed, experimental evidence suggests that intermittent rather than constant high glucose is able to maintain the activation of ROS-generating machineries such as PKC and NADPH oxidase (29) (Figure 1). Consistently, transient hyperglycemic spikes activate epigenetic changes responsible for persistent upregulation of the transcription factor NF-κB p65 and subsequent increase of inflammatory adhesion molecules (38). Understanding molecular cues regulating NF-κB signalling may provide important therapeutic implications since the transcription factor is emerging as an important molecular link between endothelial dysfunction, insulin resistance and premature vascular aging (39). The novel concept that glycemic variability may trigger inflammatory pathways deserves attention since blood glucose levels in T2DM patients always fluctuate from hyperglycemic peaks to glucose nadirs (40). A seminal study by Monnier et al. showed that glucose fluctuations during postprandial periods and, more generally, during glucose swings exhibited a more specific triggering effect on oxidative stress than chronic sustained hyperglycemia in T2DM subjects (41). On the whole, persistent vascular damage in T2DM might be the result of continuous glucose oscillations which are difficult to grasp with standard glycemic markers used in the clinical practice. Indeed, epidemiological studies have shown that HbA1c explains <25% of the variation in the risk of developing diabetic complications (42,43).
Prediction of cardiovascular risk in T2DM: seeking for the right biomarker
Our understanding of the mechanisms involved in diabetic vascular complications may be instrumental to identify potential biochemical precursors of cardiovascular damage in T2DM. The issue of risk stratification deserves attention because not every obese/diabetic subject carries the same degree of inflammation and oxidative stress. The diversity of metabolic phenotypes with different outcomes underscores the need for novel biomarkers to be used within such heterogeneous population. The atherosclerotic risk in communities (ARIC) study prospectively evaluated whether adding C-reactive protein or 18 other novel risk factors individually to a basic risk model would improve prediction of incident coronary artery disease in middle-aged men and women (44). Unfortunately, none of these risk markers predicted CVD beyond established risk calculators. Beside these disappointing results, current ESC/EASD guidelines confirm that albuminuria remains the most powerful predictor of incident CV events and heart failure in T2DM patients and recommend to estimate urinary albumin excretion rate when performing risk stratification in DM subjects (class I, level B) (45). Moreover, commonly used vascular risk calculators are flawed and clinicians are aware of their important variability and limitations (46).
Epigenetic signatures
Despite the initial enthusiasm, the clinical utility of genetic biomarkers for prediction and prevention of coronary heart disease has proved to be limited (47). More recently, cardiovascular scientists started to claim that epigenetics may better satisfy the unmet needs in cardiovascular disease prevention (48). Epigenetics refers to heritable changes in gene expression that do not affect DNA sequence while altering its activity (49). Epigenetic variations falls into three main categories: (I) DNA methylation; (II) RNA-based mechanisms including microRNAs and non-coding RNAs; and (III) post-translational histone modifications. However, most of available studies with translational value focus on DNA methylation pattern and microRNA signatures and their link with cardiovascular phenotype.
DNA methylation
The first studies of epigenetic biomarkers in the context of cardiovascular disease focused on global DNA methylation. Reduced DNA methylation is a well-established mechanism favouring gene transcription in mammals (49). With regard to the diabetic condition, promoter hypomethylation triggers upregulation of genes involved in inflammation, adiposity, beta cell dysfunction and oxidative vascular damage (6). A Dutch study showed that individuals with the metabolic syndrome had relative DNA hypomethylation compared to participants without the syndrome (50). This finding was mainly attributable to linear associations of two metabolic syndrome components with DNA methylation: fasting plasma glucose and high-density lipoprotein cholesterol. Furthermore, in the same study people with T2DM or impaired glucose metabolism had DNA hypomethylation compared to normoglycemic individuals. Mechanistically, we have recently reported that hypomethyaltion of the oxidant gene p66Shc is a key mechanism underlying the metabolic memory in experimental diabetes (26). Together with these results, many other investigators are currently exploring the impact of DNA methylation on cardiovascular damage and mortality. However, current enthusiasm about its clinical applications is likely exceeding available evidence. The general impression is that global changes in DNA methylation may only provide a rough estimate of the biological processes occurring in different tissues and organs. The possibility that modification of the epigenome may help to predict vascular risk will be strongly supported by large-scale initiatives such as the International Human Epigenome Consortium, aimed at mapping 1,000 reference epigenomes within a decade (51). Such wide epigenomic analysis will be instrumental for the identification of epigenetic variations specifically associated with major pathological states including T2DM and cardiovascular events. Together with epigenomics, the predictive value of other high-throughput ‘omics’ technologies such as metabolomics, transcriptomics and proteomics are being intensively studied in patients with T2DM with the aim to obtain large-scale snapshots of the etiological processes linking diabetes and vascular disease. The application of such postgenomics approaches as diagnostic/preventive tools has been extensively described elsewhere and is beyond the scope of the present review (52,53).
MicroRNAs
MicroRNAs (miRs), a newly identified class of small non-coding RNAs, are emerging as key players in the pathogenesis of vascular damage in diabetes (54). These small non-coding RNAs orchestrate complex molecular networks by regulating gene expression at the post-transcriptional level. Microarray profiling has shown an altered profile of miRs expression in subjects with T2DM (55). In this study, diabetic patients had a significant deregulation of miRs involved in angiogenesis, vascular repair, and endothelial homeostasis. Among other miRs, miR-126, an important pro-angiogenic effector (56), was significantly downregulated in plasma samples of 822 patients from the Brunick cohort (55). Similarly, expression analysis of miR-126 in circulating microparticles from 176 patients with stable coronary artery disease with and without diabetes mellitus revealed a significantly reduced miR-126 expression in circulating microparticles from diabetic patients (57).
Inflammatory cytokines
Activation of inflammatory pathways represents a key feature in the etiologic pathway linking hyperglycemia and insulin resistance with endothelial dysfunction. Several investigations have provided important breakthroughs on the regulation of inflammatory cytokines and chemokines and their relation with vascular damage in T2DM. A case-control study, within the prospective population-based EPIC (European Prospective Investigation into Cancer and Nutrition) study, has demonstrated that a combined elevation of IL-1β and IL-6 was independently associated with an increased risk of T2DM, suggesting the importance of low-grade inflammation in the pathogenesis of diabetes. A cross-sectional analysis performed in patients with and without T1DM showed that IL-6 and fibrinogen levels were significantly elevated in T1DM patients, regardless of adiposity and glycemic control (58). Another study showed that IL-6 is significantly increased in diabetics undergoing PCI with peri-interventional hyperglycemic state and inversely correlates with responsiveness to clopidogrel and aspirin (59). By contrast, other indices of systemic inflammation such as C-reactive protein failed to predict incident cardiovascular disease in diabetic subjects (44).
Vascular calcification markers
Vascular calcification is a pathological hallmark of atherosclerosis in diabetic subjects (60). Recent work has suggested that excess concentration of procalcific factors as well as reduction of osteogenic inhibitors may be involved in this process (61). Circulating osteoblastic cells isolated from human peripheral blood are able to calcify in vitro and in vivo (62). These cells, which express the bone protein osteocalcin (OC) and bone alkaline phosphatase (BAP), have been considered circulating osteoprogenitor cells and might participate to vascular calcification and atherosclerosis. Indeed, preliminary clinical studies found that coronary atherosclerosis and arterial stiffening are associated with activation of an osteogenic program in bone marrow-derived cells (63). A recent study has identified a subtype of circulating inflammatory monocytes, called myeloid calcifying cells (MCCs), which are involved in vascular calcification and are over-represented in patients with T2DM (64). MCCs have also been reported to exert anti-angiogenic activity, further contributing to the diabetic vascular disease phenotype. Hence, this cell subpopulation may represent an important tool to stratify cardiovascular risk in diabetes (61).
Advanced glycation end products (AGEs)
An increasing body of evidence indicates that AGEs maybe considered potential cardiovascular biomarkers in diabetes. AGEs are a large family of extensively sugar-modified proteins which can be formed in atherosclerotic plaques as a consequence of increased metabolic activity (65). It is well-established that AGEs are present in atherosclerotic lesions (66). Of note, inhibition of AGEs synthesis prevents or attenuates atherosclerosis in experimental models (67). Measuring AGEs in the skin using auto-fluorescence has provided important information on risk stratification in diabetic patients. A study involving 972 diabetic patients demonstrated that the addition of skin AGEs to the UKPDS risk engine resulted in re-classification of 27% of the patients from the low- to the high-risk group (68). Indeed, the 10-year cardiovascular event rate was higher in patients with a UKPDS score >10% when skin AGEs were above the median (56% vs. 39%) (68). A recent work found that two major AGEs, the methylglyoxal-derived 5-hydro-5-methylimidazolone (MG-H1) and Nε (carboxylmethyl) lysine (CML), measured with tandem mass spectrometry, were significantly higher in symptomatic as compared with asymptomatic carotid plaques (69). MG-H1 and CML were associated with increased levels of inflammatory cytokines IL-8 and MCP-1 as well as with higher activity of MMP-9, suggesting that AGEs may also provide information on plaque composition and stability. The relevance of AGEs is outlined by the notion that blocking their synthesis may rescue pathological features of diabetes-related vascular dysfunction. Pharmacological AGEs degradation by the cross-link breaker ALT-711 reduced arterial pulse pressure and improved the compliance of large arteries (70). Another study with benfotiamine prevented both macro- and microvascular endothelial dysfunction and oxidative stress induced by an AGE-rich meal (71). Based on these studies, AGEs formation may represent an upstream event triggering vascular inflammation, oxidative stress and eventually plaque instability (67).
Taken together, available evidence suggests that an array of upcoming biomarkers may contribute to improve prediction of cardiovascular disease in the setting of diabetes. Among the different biomarkers studied, AGEs may represent a solid predictor able to perform beyond traditional risk calculators (68). Inflammatory cytokines (IL-6, TNF-α) as well as C-reactive protein represent an important part in the pathophysiology of T2DM but studies conducted so far do not confirm an independent predictive value of such inflammatory signatures (44). On the other hand, large prospective studies are needed to confirm the potential of emerging biomarkers such as microRNAs, DNA methylation and myeloid calcifying cells.
Conclusions
Despite great progress in prevention strategies, pharmacotherapy and interventional treatment, cardiovascular events still constitute the first cause of mortality in T2DM subjects. Traditional risk factors, including hypertension, obesity and dyslipidemia significantly contribute to the strong link between diabetes and vascular disease. However, other mechanisms are being considered in the etiological pathway linking hyperglycemia, insulin resistance and vascular dysfunction. In the present review, we have shed light on novel mechanisms of diabetic vascular disease and their potential implication in the clinical setting. Future translational studies and large randomized trials will provide knowledge on the applicability of novel circulating biomarkers as well as mechanism-based approach for the diagnosis and treatment of T2DM subjects.
Acknowledgements
Disclosure: The authors declare no conflict of interest.
References
- Després JP. Body fat distribution and risk of cardiovascular disease: an update. Circulation 2012;126:1301-13. [PubMed]
- Eckel RH, Kahn SE, Ferrannini E, et al. Obesity and type 2 diabetes: what can be unified and what needs to be individualized? J Clin Endocrinol Metab 2011;96:1654-63. [PubMed]
- Inzucchi SE, Bergenstal RM, Buse JB, et al. Management of hyperglycemia in type 2 diabetes: a patient-centered approach: position statement of the American Diabetes Association (ADA) and the European Association for the Study of Diabetes (EASD). Diabetes Care 2012;35:1364-79. [PubMed]
- Beckman JA, Paneni F, Cosentino F, et al. Diabetes and vascular disease: pathophysiology, clinical consequences, and medical therapy: part II. Eur Heart J 2013;34:2444-52. [PubMed]
- Napoli C, Crudele V, Soricelli A, et al. Primary prevention of atherosclerosis: a clinical challenge for the reversal of epigenetic mechanisms? Circulation 2012;125:2363-73. [PubMed]
- Liu L, Li Y, Tollefsbol TO. Gene-environment interactions and epigenetic basis of human diseases. Curr Issues Mol Biol 2008;10:25-36. [PubMed]
- Paneni F, Costantino S, Volpe M, et al. Epigenetic signatures and vascular risk in type 2 diabetes: a clinical perspective. Atherosclerosis 2013;230:191-7. [PubMed]
- Haffner SM, Lehto S, Rönnemaa T, et al. Mortality from coronary heart disease in subjects with type 2 diabetes and in nondiabetic subjects with and without prior myocardial infarction. N Engl J Med 1998;339:229-34. [PubMed]
- Wei M, Gaskill SP, Haffner SM, et al. Effects of diabetes and level of glycemia on all-cause and cardiovascular mortality. The San Antonio Heart Study. Diabetes Care 1998;21:1167-72. [PubMed]
- Fioretto P, Dodson PM, Ziegler D, et al. Residual microvascular risk in diabetes: unmet needs and future directions. Nat Rev Endocrinol 2010;6:19-25. [PubMed]
- ADVANCE Collaborative Group, Patel A, MacMahon S, et al. Intensive blood glucose control and vascular outcomes in patients with type 2 diabetes. N Engl J Med 2008;358:2560-72. [PubMed]
- ACCORD Study Group, Gerstein HC, Miller ME, et al. Long-term effects of intensive glucose lowering on cardiovascular outcomes. N Engl J Med 2011;364:818-28.. [PubMed]
- Gaede P, Vedel P, Larsen N, et al. Multifactorial intervention and cardiovascular disease in patients with type 2 diabetes. N Engl J Med 2003;348:383-93. [PubMed]
- Gaede P, Lund-Andersen H, Parving HH, et al. Effect of a multifactorial intervention on mortality in type 2 diabetes. N Engl J Med 2008;358:580-91. [PubMed]
- Arca M, Pigna G, Favoccia C. Mechanisms of diabetic dyslipidemia: relevance for atherogenesis. Curr Vasc Pharmacol 2012;10:684-6. [PubMed]
- Keech A, Simes RJ, Barter P, et al. Effects of long-term fenofibrate therapy on cardiovascular events in 9795 people with type 2 diabetes mellitus (the FIELD study): randomised controlled trial. Lancet 2005;366:1849-61. [PubMed]
- ACCORD Study Group, Ginsberg HN, Elam MB, et al. Effects of combination lipid therapy in type 2 diabetes mellitus. N Engl J Med 2010;362:1563-74. [PubMed]
- AIM-HIGH Investigators, Boden WE, Probstfield JL, et al. Niacin in patients with low HDL cholesterol levels receiving intensive statin therapy. N Engl J Med 2011;365:2255-67. [PubMed]
- Paneni F, Beckman JA, Creager MA, et al. Diabetes and vascular disease: pathophysiology, clinical consequences, and medical therapy: part I. Eur Heart J 2013;34:2436-43. [PubMed]
- Cornier MA, Marshall JA, Hill JO, et al. Prevention of overweight/obesity as a strategy to optimize cardiovascular health. Circulation 2011;124:840-50. [PubMed]
- Paneni F, Costantino S, Cosentino F. Insulin resistance, diabetes, and cardiovascular risk. Curr Atheroscler Rep 2014;16:419. [PubMed]
- Shenouda SM, Widlansky ME, Chen K, et al. Altered mitochondrial dynamics contributes to endothelial dysfunction in diabetes mellitus. Circulation 2011;124:444-53. [PubMed]
- Tabit CE, Shenouda SM, Holbrook M, et al. Protein kinase C-β contributes to impaired endothelial insulin signaling in humans with diabetes mellitus. Circulation 2013;127:86-95. [PubMed]
- Giacco F, Brownlee M. Oxidative stress and diabetic complications. Circ Res 2010;107:1058-70. [PubMed]
- Nishikawa T, Edelstein D, Du XL, et al. Normalizing mitochondrial superoxide production blocks three pathways of hyperglycaemic damage. Nature 2000;404:787-90. [PubMed]
- Paneni F, Mocharla P, Akhmedov A, et al. Gene silencing of the mitochondrial adaptor p66(Shc) suppresses vascular hyperglycemic memory in diabetes. Circ Res 2012;111:278-89. [PubMed]
- Geraldes P, King GL. Activation of protein kinase C isoforms and its impact on diabetic complications. Circ Res 2010;106:1319-31. [PubMed]
- Sun L, Xiao L, Nie J, et al. p66Shc mediates high-glucose and angiotensin II-induced oxidative stress renal tubular injury via mitochondrial-dependent apoptotic pathway. Am J Physiol Renal Physiol 2010;299:F1014-25. [PubMed]
- Quagliaro L, Piconi L, Assaloni R, et al. Intermittent high glucose enhances apoptosis related to oxidative stress in human umbilical vein endothelial cells: the role of protein kinase C and NAD(P)H-oxidase activation. Diabetes 2003;52:2795-804. [PubMed]
- Kouroedov A, Eto M, Joch H, et al. Selective inhibition of protein kinase Cbeta2 prevents acute effects of high glucose on vascular cell adhesion molecule-1 expression in human endothelial cells. Circulation 2004;110:91-6. [PubMed]
- Giorgio M, Migliaccio E, Orsini F, et al. Electron transfer between cytochrome c and p66Shc generates reactive oxygen species that trigger mitochondrial apoptosis. Cell 2005;122:221-33. [PubMed]
- Migliaccio E, Giorgio M, Mele S, et al. The p66shc adaptor protein controls oxidative stress response and life span in mammals. Nature 1999;402:309-13. [PubMed]
- Camici GG, Schiavoni M, Francia P, et al. Genetic deletion of p66(Shc) adaptor protein prevents hyperglycemia-induced endothelial dysfunction and oxidative stress. Proc Natl Acad Sci U S A 2007;104:5217-22. [PubMed]
- Pagnin E, Fadini G, de Toni R, et al. Diabetes induces p66shc gene expression in human peripheral blood mononuclear cells: relationship to oxidative stress. J Clin Endocrinol Metab 2005;90:1130-6. [PubMed]
- Paneni F, Volpe M, Lüscher TF, et al. SIRT1, p66(Shc), and Set7/9 in vascular hyperglycemic memory: bringing all the strands together. Diabetes 2013;62:1800-7. [PubMed]
- Ceriello A, Ihnat MA, Thorpe JE. Clinical review 2: The “metabolic memory”: is more than just tight glucose control necessary to prevent diabetic complications? J Clin Endocrinol Metab 2009;94:410-5. [PubMed]
- Frontoni S, Di Bartolo P, Avogaro A, et al. Glucose variability: An emerging target for the treatment of diabetes mellitus. Diabetes Res Clin Pract 2013;102:86-95. [PubMed]
- El-Osta A, Brasacchio D, Yao D, et al. Transient high glucose causes persistent epigenetic changes and altered gene expression during subsequent normoglycemia. J Exp Med 2008;205:2409-17. [PubMed]
- Baker RG, Hayden MS, Ghosh S. NF. -κB, inflammation, and metabolic disease. Cell Metab 2011;13:11-22. [PubMed]
- Johnson EL. Glycemic variability in type 2 diabetes mellitus: oxidative stress and macrovascular complications. Adv Exp Med Biol 2012;771:139-54. [PubMed]
- Monnier L, Mas E, Ginet C, et al. Activation of oxidative stress by acute glucose fluctuations compared with sustained chronic hyperglycemia in patients with type 2 diabetes. JAMA 2006;295:1681-7. [PubMed]
- Brownlee M, Hirsch IB. Glycemic variability: a hemoglobin A1c-independent risk factor for diabetic complications. JAMA 2006;295:1707-8. [PubMed]
- Paneni F. 2013 ESC/EASD guidelines on the management of diabetes and cardiovascular disease: Established knowledge and evidence gaps. Diab Vasc Dis Res 2014;11:5-10. [PubMed]
- Folsom AR, Chambless LE, Ballantyne CM, et al. An assessment of incremental coronary risk prediction using C-reactive protein and other novel risk markers: the atherosclerosis risk in communities study. Arch Intern Med 2006;166:1368-73. [PubMed]
- Authors/Task Force Members, Rydén L, Grant PJ, et al. ESC Guidelines on diabetes, pre-diabetes, and cardiovascular diseases developed in collaboration with the EASD: the Task Force on diabetes, pre-diabetes, and cardiovascular diseases of the European Society of Cardiology (ESC) and developed in collaboration with the European Association for the Study of Diabetes (EASD). Eur Heart J 2013;34:3035-87. [PubMed]
- Allan GM, Nouri F, Korownyk C, et al. Agreement among cardiovascular disease risk calculators. Circulation 2013;127:1948-56. [PubMed]
- Di Angelantonio E, Butterworth AS. Clinical utility of genetic variants for cardiovascular risk prediction: a futile exercise or insufficient data? Circ Cardiovasc Genet 2012;5:387-90. [PubMed]
- Cooper ME, El-Osta A. Epigenetics: mechanisms and implications for diabetic complications. Circ Res 2010;107:1403-13. [PubMed]
- Handy DE, Castro R, Loscalzo J. Epigenetic modifications: basic mechanisms and role in cardiovascular disease. Circulation 2011;123:2145-56. [PubMed]
- Luttmer R, Spijkerman AM, Kok RM, et al. Metabolic syndrome components are associated with DNA hypomethylation. Obes Res Clin Pract 2013;7:e106-e115. [PubMed]
- Abbott A. Project set to map marks on genome. Nature 2010;463:596-7. [PubMed]
- Gjesing AP, Pedersen O. ‘Omics’-driven discoveries in prevention and treatment of type 2 diabetes. Eur J Clin Invest 2012;42:579-88. [PubMed]
- Herder C, Karakas M, Koenig W. Biomarkers for the prediction of type 2 diabetes and cardiovascular disease. Clin Pharmacol Ther 2011;90:52-66. [PubMed]
- Quiat D, Olson EN. MicroRNAs in cardiovascular disease: from pathogenesis to prevention and treatment. J Clin Invest 2013;123:11-8. [PubMed]
- Zampetaki A, Kiechl S, Drozdov I, et al. Plasma microRNA profiling reveals loss of endothelial miR-126 and other microRNAs in type 2 diabetes. Circ Res 2010;107:810-7. [PubMed]
- Mocharla P, Briand S, Giannotti G, et al. AngiomiR-126 expression and secretion from circulating CD34(+) and CD14(+) PBMCs: role for proangiogenic effects and alterations in type 2 diabetics. Blood 2013;121:226-36. [PubMed]
- Jansen F, Yang X, Hoelscher M, et al. Endothelial microparticle-mediated transfer of MicroRNA-126 promotes vascular endothelial cell repair via SPRED1 and is abrogated in glucose-damaged endothelial microparticles. Circulation 2013;128:2026-38. [PubMed]
- Spranger J, Kroke A, Möhlig M, et al. Inflammatory cytokines and the risk to develop type 2 diabetes: results of the prospective population-based European Prospective Investigation into Cancer and Nutrition (EPIC)-Potsdam Study. Diabetes 2003;52:812-7. [PubMed]
- Geisler T, Mueller K, Aichele S, et al. Impact of inflammatory state and metabolic control on responsiveness to dual antiplatelet therapy in type 2 diabetics after PCI: prognostic relevance of residual platelet aggregability in diabetics undergoing coronary interventions. Clin Res Cardiol 2010;99:743-52. [PubMed]
- Avogaro A, Rattazzi M, Fadini GP. Ectopic calcification in diabetic vascular disease. Expert Opin Ther Targets 2014;18:595-609. [PubMed]
- Albiero M, Avogaro A, Fadini GP. Circulating Cellular Players in Vascular Calcification. Curr Pharm Des 2014. [Epub ahead of print]. [PubMed]
- Eghbali-Fatourechi GZ, Lamsam J, Fraser D, et al. Circulating osteoblast-lineage cells in humans. N Engl J Med 2005;352:1959-66. [PubMed]
- Flammer AJ, Gössl M, Widmer RJ, et al. Osteocalcin positive CD133+/CD34-/KDR+ progenitor cells as an independent marker for unstable atherosclerosis. Eur Heart J 2012;33:2963-9. [PubMed]
- Fadini GP, Albiero M, Menegazzo L, et al. Widespread increase in myeloid calcifying cells contributes to ectopic vascular calcification in type 2 diabetes. Circ Res 2011;108:1112-21. [PubMed]
- Jandeleit-Dahm K, Cooper ME. The role of AGEs in cardiovascular disease. Curr Pharm Des 2008;14:979-86. [PubMed]
- Basta G, Schmidt AM, De Caterina R. Advanced glycation end products and vascular inflammation: implications for accelerated atherosclerosis in diabetes. Cardiovasc Res 2004;63:582-92. [PubMed]
- Paneni F, Cosentino F. Advanced glycation endproducts and plaque instability: a link beyond diabetes. Eur Heart J 2014;35:1095-7. [PubMed]
- Meerwaldt R, Graaff R, Oomen PH, et al. Simple non-invasive assessment of advanced glycation endproduct accumulation. Diabetologia 2004;47:1324-30. [PubMed]
- Hanssen NM, Wouters K, Huijberts MS, et al. Higher levels of advanced glycation endproducts in human carotid atherosclerotic plaques are associated with a rupture-prone phenotype. Eur Heart J 2014;35:1137-46. [PubMed]
- Kass DA, Shapiro EP, Kawaguchi M, et al. Improved arterial compliance by a novel advanced glycation end-product crosslink breaker. Circulation 2001;104:1464-70. [PubMed]
- Stirban A, Negrean M, Stratmann B, et al. Benfotiamine prevents macro- and microvascular endothelial dysfunction and oxidative stress following a meal rich in advanced glycation end products in individuals with type 2 diabetes. Diabetes Care 2006;29:2064-71. [PubMed]