Comparison of diagnostic performance between quantitative flow ratio, non-hyperemic pressure indices and fractional flow reserve
Introduction
Treatment of physiologically significant coronary artery stenoses is associated with improvement in symptoms and cardiovascular outcomes (1-5). Methods to determine the physiological significance of stenoses have evolved over the past two decades. Fractional flow reserve (FFR) is currently the ‘gold standard’ to assess the significance of coronary stenoses (6). FFR-guided percutaneous coronary intervention (PCI) is superior to visual angiography-guided PCI and results in better patient outcomes (7,8). Although its use has increased in the developed world, FFR remains under-utilized due to the additional time taken and costs incurred to interrogate stenoses with a pressure wire, limitations associated with induction of hyperaemia, and ongoing operator confidence in their visual assessment of lesion severity (9-12).
Instantaneous wave-free ratio (iFR) is another physiological index to assess functional significance of coronary stenoses. In contrast to FFR, iFR is derived by calculating the mean ratio of the pressure distal to a lesion (Pd) over the aortic pressure (Pa) during the wave-free period in diastole, circumventing the need to pharmacologically induce hyperaemia (13). Like FFR, iFR requires the use of a pressure wire. Recent studies have shown comparable results between iFR and FFR, with an iFR threshold of 0.89 comparable to an FFR threshold of 0.80, to suggest physiological significance (14-17). Van’t Veer and colleagues recently demonstrated that all diastolic resting indices were identical to iFR, both numerically and with respect to their agreement with FFR (18). While the dPR (diastolic pressure ratio) calculates the mean Pd/Pa over the entire diastolic period, dPR25–75 only considers between 25% and 75% of the diastolic period (18).
As coronary angiography remains the cornerstone in clinical decision making, development of an angiography-based functional assessment of coronary artery stenosis would be ideal. This would overcome the growth limitations of FFR and iFR attributed to expenses associated with pressure wires. Two such methods are the DILEMMA score and quantitative flow ratio (QFR). The DILEMMA score evaluates coronary artery lesions by considering the degree of luminal stenosis, length of the lesion and area of myocardium at jeopardy subtended by that lesion (19). Our group had evaluated its diagnostic accuracy against FFR in recent studies (19,20). QFR is a novel method which utilises advancements in mathematical modelling techniques and scientific understanding of principles of computational fluid dynamics, to derive an angiography based assessment of functional significance (21). The diagnostic accuracy of QFR in identifying haemodynamically significant coronary artery stenoses had recently been demonstrated (21).
The aim of this study is to compare the diagnostic accuracy of these various novel contemporary techniques against the gold standard FFR, in stable coronary artery disease.
Methods
Patients
We retrospectively examined patients with stable coronary artery disease who underwent elective coronary angiography and FFR assessment at a major tertiary referral hospital (Monash Medical Centre) in Melbourne, Australia between January 2010 and December 2013. These dates were selected as angiography studies in our institution within these dates had a frame acquisition rate of 15 frames per second (required to perform QFR analyses). Data acquisition for this retrospective study was previously approved by our institutional human research ethics committee (Approval ID: 10326). All lesions had diameter stenosis between 30–90% and a reference vessel diameter ≥2 mm according to visual assessment. FFR was clinically indicated and performed at the discretion of their treating cardiologist. At our institution, and consistent with conventional methods, the clinically acceptable drift on FFR acquisition was <0.02. We identified patients who had retrievable pressure wire tracing data. Exclusion criteria included patients with bypass grafts, culprit vessels that collateralize other vessels, culprit vessels in the setting of myocardial infarction, lesions in diagonal vessels, vessels with myocardial bridging and those where angiographic acquisition image quality did not allow for QFR analysis.
Invasive coronary angiography, FFR and QCA
Invasive coronary angiography and FFR were performed as per standard practice via the femoral or radial approach. An FFR value ≤0.80 was taken to define functionally significant stenoses. The pressure wire (Pressure wire Certus 6, St Jude Medical, USA) was calibrated and electronically equalised with the aortic pressure before being placed in the distal third of the coronary artery being interrogated. Intracoronary glyceryl trinitrate (100 mcg) was injected to minimise vasospasm. Intravenous adenosine was administered (140 mcg/kg/min) through an intravenous line in the antecubital fossa. At steady-state hyperaemia, FFR was recorded on a RadiAnalyzer Xpress (St Jude Medical Systems, USA), calculated by dividing the coronary pressure measured with the sensor placed distal to the stenosis (Pd) by the aortic pressure measured through the guide catheter (Pa). Quantitative coronary angiographic analysis was performed to derive minimal luminal diameter (MLD) and lesion length.
iFR and dPR
The iFR calculated was similar, but not identical, to that described originally (13). Briefly, we analysed our diastolic period from the dicrotic notch up until 10 ms before the end of the diastolic period, rather than 5 ms. This was because pressure tracing data were available every 10 ms rather than 5 ms.
The dPRs we selected were dPR and dPR25–75. While dPR measured the average Pd/Pa ratio over the entire diastolic period, dPR25–75 measured the average Pd/Pa between 25% and 75% of the period into diastole (18). This period was identified by manually selecting values between the dicrotic notch and end of diastole.
All calculations were derived after averaging values over five cardiac cycles.
QFR
The QFR analysis was performed using a validated software (QAngio XA3D 3.1.1; Medis Medical Imaging System, Leiden, The Netherlands) by two independent investigators who were unaware of the FFR/iFR/dPR/dPR25–75/DILEMMA results. Two angiographic image projections acquired at ≥25º apart that presented the least foreshortening of the stenosis and minimum overlap of the main vessel and side branches were selected for analysis. All angiographic images were acquired at 15 frames per second. The lumen contour was automatically delineated and manual correction performed as appropriate. A 3D anatomical vessel model without side branches was derived from the software and QFR computation was performed using a specific flow model: contrast-flow QFR (cQFR) previously described by Tu et al. (21). In brief, cQFR was computed using a modelled hyperemic flow velocity on the basis of the thrombolysis in myocardial infarction (TIMI) frame count analysis without pharmacologically-induced hyperemia. The TIMI frame count analysis was carried out on either one of two angiographic projections that provided a more well-defined contrast flow. The QFR value was obtained at the distal coronary artery corresponding to the site of the FFR measurement. Diagonal lesions were excluded due to equivocal classification as there were disparate methods to compute QFR on LAD and non-LAD vessels.
DILEMMA score calculation
Methodology to calculate the DILEMMA Score is described in an earlier study by our institution, and summarised in Table 1 (19). Briefly, the DILEMMA score was a summation of points attributed to a target lesion based on three characteristics: minimum luminal diameter (maximum 4 points), lesion length (maximum 3 points) and myocardium at risk (maximum 5 points). The area of myocardium at risk was calculated from angiography using the BARI myocardial jeopardy index, which has been validated with cardiac MRI (22). Each lesion is assigned a DILEMMA score of between 0 and 12.
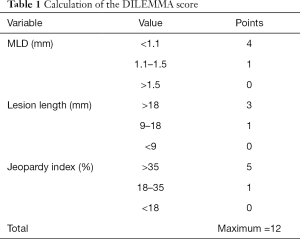
Full table
Statistical analysis
Statistical analyses were performed with IBM SPSS Statistics 25.0 (IBM SPSS Statistics for Windows, Version 25.0. Armonk, NY: IBM Corp) and R programming language version 3.6.2 (RStudio for Windows, Version 1.2.5033). Variables were tested for normality with visual assessment, the Shapiro-Wilk test and a test of skewness.
Wilcoxon paired signed-rank test was performed as a non-parametric substitute for paired t-test analysis. Correlation between non-parametric continuous variables were evaluated with Spearman’s rank correlation coefficient. Statistical comparison of coefficients was performed after Fisher-Z transformation. For all analyses, a two-tailed P value of less than 0.05 was considered statistically significant.
A receiver-operating characteristic curve was established with reference FFR ≤0.80 or iFR ≤0.89 to correspond to physiologically significant obstructive coronary artery stenosis and discriminatory function assessed for statistical significance.
Owing to the repeated-measures nature of the study, a general estimating equation approach was used assuming a binomial probability distribution.
Results
Clinical characteristics
We identified 115 lesions from 54 patients, for which FFR were performed between January 2010 and December 2013, with a radiographic frame rate of 15 fps, the minimum required for QFR analysis (Table 2). We excluded 15 cases due to poor image acquisition or suboptimal raw data capture of pressure tracings, 13 cases due to the lesion involving the diagonal artery and two cases due to presence of muscle bridging (Figure 1). There were five cases for which the pressure tracing did not have a sufficient time period of recording in the pre-hyperaemic phase and therefore an iFR, dPR or dPR25–75 were not able to be calculated accurately.
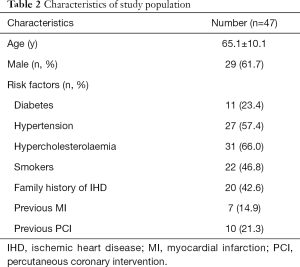
Full table
Among the 85 lesions included in the analysis, 38 were from the LAD (45%), 25 were from the LCX or branches (29%) and 22 were from the RCA or branches (26%). There were 21 vessels (25%) with FFR ≤0.80. The mean FFR was 0.86±0.09 and the median FFR was 0.88 (0.81–0.92) (Table 3).
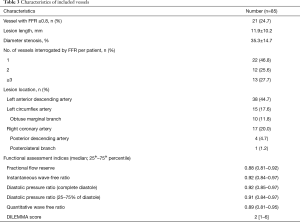
Full table
Correlation between FFR, QFR, iFR, dPR, dPR25–75 and DILEMMA score
Using the Spearman’s rank correlation coefficient (rs), FFR was significantly and positively correlated to QFR (rs=0.801; P<0.001), iFR (rs=0.710; P<0.001), dPR (rs=0.716; P<0.001) and dPR25–75 (rs=0.715; P<0.001). There was statistically significant negative correlation between FFR and the DILEMMA score (rs=−0.623; P<0.001). Direct comparison of correlations between FFR against QFR and FFR against iFR did not show statistical significance (P=0.17).
There was excellent correlation between the diastolic indices: iFR and dPR (rs=0.993; P<0.001); iFR and dPR25–75 (rs=0.995; P<0.001).
The correlation between FFR and QFR was greater than the correlation between iFR and QFR (rs=0.801 vs. rs=0.516, P<0.001). Scatter plots can be seen in Figure 2.
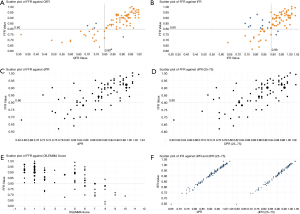
Diagnostic performance of iFR, dPR and dPR25–75 to predict FFR ≤0.8
There were 30 (38%) vessels with iFR ≤0.89. Mean iFR was 0.91±0.08 and median iFR was 0.92 (0.84–0.97). The specificity, sensitivity, positive predictive value (PPV) and negative predictive value (NPV) of iFR ≤0.89 to predict FFR ≤0.8 were 79%, 89%, 57% and 96% respectively. The overall diagnostic accuracy was 81%.
There were 30 (38%) vessels with dPR ≤0.89. Mean dPR was 0.90±0.08 and median dPR was 0.92 (0.85–0.97). The specificity, sensitivity, PPV and NPV of dPR ≤0.89 to predict FFR ≤0.8 were 80%, 89%, 59% and 96% respectively. The overall diagnostic accuracy was 83%.
There were 31 (39%) vessels with dPR25–75 ≤0.89. Mean dPR25–75 was 0.90±0.09 and median dPR25–75 was 0.91 (0.84–0.97). The specificity, sensitivity, PPV and NPV of dPR25–75 ≤0.89 to predict FFR ≤0.8 were 77%, 89%, 55% and 96% respectively. The overall diagnostic accuracy was 80%.
Diagnostic performance of QFR to predict FFR ≤0.8 and iFR ≤0.89
There were 21 (25%) vessels with QFR <0.8. Mean QFR was 0.86±0.13 and median QFR was 0.89 (0.81–0.95). A Wilcoxon paired signed-rank test had a P value of 0.357, suggesting no statistically significant difference between individual QFR and FFR values. The specificity, sensitivity, PPV and NPV of QFR <0.8 to predict FFR ≤0.8 was 95%, 86%, 86% and 95% respectively. The overall diagnostic accuracy was 93%.
When iFR
Diagnostic performance of DILEMMA score to predict FFR ≤0.8
A DILEMMA score of ≤2 (n=43) had an NPV of 95% for identifying FFR negative lesions while DILEMMA score of ≥9 (n=3) had a PPV of 100% to identify FFR positive lesions.
Comparison of receiver operating characteristic analyses
A receiver-operating characteristic (ROC) curve was created to assess the accuracy of QFR, iFR, dPR, dPR25–75 and DILEMMA score (Figure 3). The discriminatory function was numerically greatest for QFR (AUC =0.947; P<0.001), followed by DILEMMA (AUC =0.916; P<0.001), dPR (AUC =0.883; P<0.001), iFR (AUC =0.880; P<0.001) and dPR25–75 (AUC =0.880; P<0.001). Differences in AUC between the indices were not statistically significant (P>0.05).
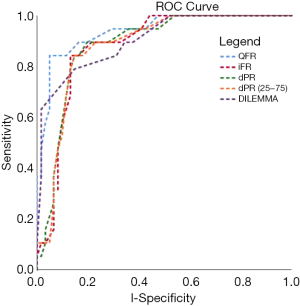
The discriminatory function of QFR was lower when iFR ≤0.89 was used as the reference standard instead of FFR ≤0.80 (AUC =0.770 versus 0.947; P<0.01).
Discussion
In this study, we compared the diagnostic accuracy of novel contemporary methods (iFR, dPR, dPR25–75, QFR and DILEMMA score) to determine functional significance of coronary artery stenosis against the reference standard, FFR. We showed that QFR compared favourably to iFR, dPR, dPR25–75 and DILEMMA score to predict significant FFR. We showed that QFR and DILEMMA scores can be potential angiography based “gatekeepers” to FFR.
iFR, dPR, dPR25–75 versus FFR
The overall diagnostic accuracy of iFR (81%), dPR (83%) and dPR25–75 (80%) to predict significant FFR in our study is comparable to previous studies. Prospective studies by Escaned et al. and Pisters et al. showed that iFR with a cut-off of 0.89 had an accuracy of 82.5% and 82% respectively (23,24). In our study, the Spearman’s Rho correlation between iFR and dPR was 0.993 and iFR and dPR25–75 was 0.995. This was similar to 0.993 and 0.997 respectively as obtained by Van’t Veer et al. (18).
iFR, dPR and dPR25–75 versus QFR
To the best of our knowledge, the present study is the first to compare QFR with iFR, dPR and dPR25–75. A recent study by Emori et al. found that QFR had superior diagnostic accuracy to iFR (94% vs. 74%) in predicting lesions with FFR less than or equal to 0.80 (25). Additionally, similar to our findings, Hwang et al. found QFR had better discriminatory function against a reference standard of FFR rather than iFR (0.953 vs. 0.880) (26).
DILEMMA score versus FFR
An alternative method requiring no additional software or expense to angiography, was the DILEMMA score. In our study, we showed that a DILEMMA score of ≤2 and ≥9 have high NPV and PPV respectively, consistent with previous findings which show that DILEMMA score of ≤2 had a NPV of 96.3% and a score ≥9 had a PPV of 88.9% (20).
QFR versus FFR
QFR is an emerging tool solely based on angiography. It relies on complex mathematical methods built upon principles of computational fluid dynamics. In contrast to FFR and iFR, QFR neither requires an intracoronary pressure wire, nor does it need adenosine administration to induce hyperaemia. It can be performed almost immediately with a result derived within minutes after angiography has completed. QFR has demonstrated substantial reproducibility with excellent inter-observer agreement (27). Our study is consistent with others that have investigated QFR. The international multicentre FAVOR Pilot study by Tu et al. compared QFR against the gold standard FFR and had similar results with a statistically significant Pearson’s correlation of 0.77, an AUC of 0.92 and an overall diagnostic accuracy of 86% (21). Given the advantages highlighted and speed with which QFR can be derived, QFR could play a potential role as a gatekeeper to FFR. Studies that included a hybrid approach with QFR and FFR suggest that in approximately two-thirds of cases, a pressure-wire could be avoided whilst maintaining a diagnostic accuracy of 95% (28,29).
Assessment of QFR as a diagnostic test
The prevalence of disease in a population affects the PPV and NPV of a diagnostic test in question. In our study, the proportion of lesions that were significant on FFR assessment were 25%. In the FAME study, the proportion of lesions that were angiographically of intermediate severity and later found to be FFR significant were 35% (7). Thus, given our lower prevalence rate of FFR significance, our results may overestimate the NPV and underestimate the PPV of QFR as a diagnostic test.
Clinical implications
It is commonly accepted that visual or 2D-QCA estimations of coronary stenoses are often inaccurate and not reflective of a stenotic lesion’s physiological significance. However, the process of deriving pressure indices is not without clinical risk, upfront financial cost for the institution, and added procedural time. Even though studies such as RIPCORD (30), R3F (31), and POST-IT (32) show that routine use of FFR changes revascularization strategy in 26–44% of cases, the adoption of FFR is not widespread internationally. Landmark studies demonstrate that FFR guidance typically lowers the number of lesions that require PCI, reduces the overall hospital care costs and improves patient outcomes (8,33). Additionally, FFR-guided treatment seems to lead to an overall economic benefit when compared to angiographically guided management (34-36). However, an overall economic benefit may not necessarily reflect a better financial position for the institute undertaking the procedure.
In today’s environment, where procedures need to pass the scrutiny of financial impact, clinical safety and clinical value, there may be a role for QFR. QFR may lower an institution’s financial burden by substantially reducing the number of lesions that proceed to FFR analysis, without compromising accuracy. As the input data necessary for QFR is the angiogram itself, the need to proceed to FFR can quickly be determined by the end of the initial image acquisition. This would provide meaningful information to guide the cardiologist and is important as the adoption of FFR, while increasing, is not universal. The cost of this would include training time of the technician involved in the analysis, as well as licensing for necessary software.
The DILEMMA score aims to provide guidance to the cardiologist without added cost to the process of angiography. While our study demonstrates that it can be clinically effective, the uptake of the DILEMMA score is also quite low, perhaps due to the tedious and arguably lengthy nature of the necessary calculations.
Limitations on the application of QFR are the circumstances and conditions within which QFR can be applied confidently. Situations where it may not be appropriate to use QFR to estimate FFR include extensive image foreshortening and overlap, ostial LCx lesions, left main stenosis and various others outlined by the software vendor. Other conditions where reliability and interpretation need further investigation are those that are also uncertain in FFR, for instance the presence of severe aortic stenosis.
QFR and the DILEMMA score are both angiographically-derived methods which, upon comparison, have their individual strengths. The DILEMMA score incorporates QCA derived parameters such as MLD and lesion length as well as an estimate of myocardial mass subtended by the stenosis using the BARI myocardial jeopardy index (19). Although the DILEMMA score has been shown to reduce the number of patients requiring invasive physiological assessment in a large multi-centre study of patients with intermediate coronary artery stenoses (20), the DILEMMA score does not directly estimate the FFR. QFR, on the other hand, provides an estimate of the FFR. However, it requires proprietary software and the added costs that come with that.
Study limitations
Our results represent a retrospective small, single-centre experience and hence needs confirmation with larger multi-centre studies. The major barrier to the number of patients included in our study was the availability of retrievable pressure tracing data between 2010 and 2013. Additionally, as our centre had transitioned to a lower frame rate as standard protocol to minimise radiation for angiography, we were unable to assess more recent angiographic cases in order to meet the frame rate requirement (>12.5 frames per second) to perform QFR analysis. Future studies to assess the utility of contrast-QFR on studies acquired at lower frame rate are therefore required. Side branches of bifurcation lesions with Medina type 1,1,1 or 1,0,1 were not assessed as generalizability of QFR to the side branches of bifurcation lesions have not been thoroughly assessed.
Conclusions
QFR had excellent correlation and diagnostic performance as measured against FFR when compared to iFR, dPR, dPR25–75 and DILEMMA score. QFR performed superiorly against a reference standard of FFR compared to iFR. QFR is a convenient tool to assess the physiological significance of coronary stenoses, providing a reliable alternative to other pressure wire based indices. Further studies are required to assess QFR performance in a prospective manner, particularly with outcome studies. Additionally, cost effectiveness studies are necessary for adoption into routine clinical practice.
Acknowledgments
Funding: None.
Footnote
Conflicts of Interest: All authors have completed the ICMJE uniform disclosure form (available at http://dx.doi.org/10.21037/cdt-20-179). DTLW currently serves on the editorial board for Cardiovascular Diagnosis and Therapy from Feb 2019–Jan 2021. The other authors have no conflicts of interest to declare.
Ethical Statement: The authors are accountable for all aspects of the work in ensuring that questions related to the accuracy or integrity of any part of the work are appropriately investigated and resolved. Data acquisition for this retrospective study was previously approved by our institutional human research ethics committee (Approval ID: 10326). Because of the retrospective nature of the research, the requirement for informed consent was waived.
Open Access Statement: This is an Open Access article distributed in accordance with the Creative Commons Attribution-NonCommercial-NoDerivs 4.0 International License (CC BY-NC-ND 4.0), which permits the non-commercial replication and distribution of the article with the strict proviso that no changes or edits are made and the original work is properly cited (including links to both the formal publication through the relevant DOI and the license). See: https://creativecommons.org/licenses/by-nc-nd/4.0/.
References
- Coronary angioplasty versus medical therapy for angina: the second Randomised Intervention Treatment of Angina (RITA-2) trial. RITA-2 trial participants. Lancet 1997;350:461-8. [Crossref] [PubMed]
- Erne P, Schoenenberger AW, Burckhardt D, et al. Effects of percutaneous coronary interventions in silent ischemia after myocardial infarction: the SWISSI II randomized controlled trial. JAMA 2007;297:1985-91. [Crossref] [PubMed]
- Fihn SD, Williams SV, Daley J, et al. Guidelines for the management of patients with chronic stable angina: treatment. Ann Intern Med 2001;135:616-32. [Crossref] [PubMed]
- Shaw LJ, Berman DS, Maron DJ, et al. Optimal medical therapy with or without percutaneous coronary intervention to reduce ischemic burden: results from the Clinical Outcomes Utilizing Revascularization and Aggressive Drug Evaluation (COURAGE) trial nuclear substudy. Circulation 2008;117:1283-91. [Crossref] [PubMed]
- Yusuf S, Zucker D, Peduzzi P, et al. Effect of coronary artery bypass graft surgery on survival: overview of 10-year results from randomised trials by the Coronary Artery Bypass Graft Surgery Trialists Collaboration. Lancet 1994;344:563-70. [Crossref] [PubMed]
- Pijls NH, De Bruyne B, Peels K, et al. Measurement of fractional flow reserve to assess the functional severity of coronary-artery stenoses. N Engl J Med 1996;334:1703-8. [Crossref] [PubMed]
- Tonino PA, De Bruyne B, Pijls NH, et al. Fractional flow reserve versus angiography for guiding percutaneous coronary intervention. N Engl J Med 2009;360:213-24. [Crossref] [PubMed]
- Tonino PA, Fearon WF, De Bruyne B, et al. Angiographic versus functional severity of coronary artery stenoses in the FAME study fractional flow reserve versus angiography in multivessel evaluation. J Am Coll Cardiol 2010;55:2816-21. [Crossref] [PubMed]
- Medicare Australia. MBS item statistics reports. Available online: https://medicarestatistics.humanservices.gov.au/statistics/mbs_item.jsp. Accessed June 2019.
- Harper RW, Ko BS. A new algorithm for the management of stable coronary artery disease incorporating CT coronary angiography and fractional flow reserve: how we can improve outcomes and reduce costs. Med J Aust 2011;194:186-9. [Crossref] [PubMed]
- Pothineni NV, Shah NN, Rochlani Y, et al. U.S. Trends in Inpatient Utilization of Fractional Flow Reserve and Percutaneous Coronary Intervention. J Am Coll Cardiol 2016;67:732-3. [Crossref] [PubMed]
- Tebaldi M, Biscaglia S, Fineschi M, et al. Evolving Routine Standards in Invasive Hemodynamic Assessment of Coronary Stenosis: The Nationwide Italian SICI-GISE Cross-Sectional ERIS Study. JACC Cardiovasc Interv 2018;11:1482-91. [Crossref] [PubMed]
- Sen S, Escaned J, Malik IS, et al. Development and validation of a new adenosine-independent index of stenosis severity from coronary wave-intensity analysis: results of the ADVISE (ADenosine Vasodilator Independent Stenosis Evaluation) study. J Am Coll Cardiol 2012;59:1392-402. [Crossref] [PubMed]
- Davies JE, Sen S, Dehbi HM, et al. Use of the Instantaneous Wave-free Ratio or Fractional Flow Reserve in PCI. N Engl J Med 2017;376:1824-34. [Crossref] [PubMed]
- Gotberg M, Christiansen EH, Gudmundsdottir IJ, et al. Instantaneous Wave-free Ratio versus Fractional Flow Reserve to Guide PCI. N Engl J Med 2017;376:1813-23. [Crossref] [PubMed]
- Harle T, Bojara W, Meyer S, et al. Comparison of instantaneous wave-free ratio (iFR) and fractional flow reserve (FFR)--first real world experience. Int J Cardiol 2015;199:1-7. [Crossref] [PubMed]
- Petraco R, Escaned J, Sen S, et al. Classification performance of instantaneous wave-free ratio (iFR) and fractional flow reserve in a clinical population of intermediate coronary stenoses: results of the ADVISE registry. EuroIntervention 2013;9:91-101. [Crossref] [PubMed]
- Van't Veer M, Pijls NHJ, Hennigan B, et al. Comparison of Different Diastolic Resting Indexes to iFR: Are They All Equal? J Am Coll Cardiol 2017;70:3088-96. [Crossref] [PubMed]
- Wong DT, Narayan O, Ko BS, et al. A novel coronary angiography index (DILEMMA score) for prediction of functionally significant coronary artery stenoses assessed by fractional flow reserve: A novel coronary angiography index. Am Heart J 2015;169:564-71.e4. [Crossref] [PubMed]
- Michail M, Dehbi HM, Nerlekar N, et al. Application of the DILEMMA score to improve lesion selection for invasive physiological assessment. Catheter Cardiovasc Interv 2019;94:E96-E103. [Crossref] [PubMed]
- Tu S, Westra J, Yang J, et al. Diagnostic Accuracy of Fast Computational Approaches to Derive Fractional Flow Reserve From Diagnostic Coronary Angiography: The International Multicenter FAVOR Pilot Study. JACC Cardiovasc Interv 2016;9:2024-35. [Crossref] [PubMed]
- Moral S, Rodriguez-Palomares JF, Descalzo M, et al. Quantification of myocardial area at risk: validation of coronary angiographic scores with cardiovascular magnetic resonance methods. Rev Esp Cardiol (Engl Ed) 2012;65:1010-7. [Crossref] [PubMed]
- Escaned J, Echavarria-Pinto M, Garcia-Garcia HM, et al. Prospective Assessment of the Diagnostic Accuracy of Instantaneous Wave-Free Ratio to Assess Coronary Stenosis Relevance: Results of ADVISE II International, Multicenter Study (ADenosine Vasodilator Independent Stenosis Evaluation II). JACC Cardiovasc Interv 2015;8:824-33. [Crossref] [PubMed]
- Pisters R, Ilhan M, Veenstra LF, et al. Instantaneous wave-free ratio and fractional flow reserve in clinical practice. Neth Heart J 2018;26:385-92. [Crossref] [PubMed]
- Emori H, Kubo T, Kameyama T, et al. Quantitative flow ratio and instantaneous wave-free ratio for the assessment of the functional severity of intermediate coronary artery stenosis. Coron Artery Dis 2018;29:611-7. [Crossref] [PubMed]
- Hwang D, Choi KH, Lee JM, et al. Diagnostic Agreement of Quantitative Flow Ratio With Fractional Flow Reserve and Instantaneous Wave-Free Ratio. J Am Heart Assoc 2019;8:e011605. [Crossref] [PubMed]
- van Rosendael AR, Koning G, Dimitriu-Leen AC, et al. Accuracy and reproducibility of fast fractional flow reserve computation from invasive coronary angiography. Int J Cardiovasc Imaging 2017;33:1305-12. [Crossref] [PubMed]
- Westra J, Andersen BK, Campo G, et al. Diagnostic Performance of In-Procedure Angiography-Derived Quantitative Flow Reserve Compared to Pressure-Derived Fractional Flow Reserve: The FAVOR II Europe-Japan Study. J Am Heart Assoc 2018;7:e009603. [Crossref] [PubMed]
- Koltowski L, Zaleska M, Maksym J, et al. Quantitative flow ratio derived from diagnostic coronary angiography in assessment of patients with intermediate coronary stenosis: a wire-free fractional flow reserve study. Clin Res Cardiol 2018;107:858-67. [Crossref] [PubMed]
- Curzen N, Rana O, Nicholas Z, et al. Does routine pressure wire assessment influence management strategy at coronary angiography for diagnosis of chest pain?: the RIPCORD study. Circ Cardiovasc Interv 2014;7:248-55. [Crossref] [PubMed]
- Van Belle E, Rioufol G, Pouillot C, et al. Outcome impact of coronary revascularization strategy reclassification with fractional flow reserve at time of diagnostic angiography: insights from a large French multicenter fractional flow reserve registry. Circulation 2014;129:173-85. [Crossref] [PubMed]
- Baptista SB, Raposo L, Santos L, et al. Impact of Routine Fractional Flow Reserve Evaluation During Coronary Angiography on Management Strategy and Clinical Outcome: One-Year Results of the POST-IT. Circ Cardiovasc Interv 2016;9:e003288. [Crossref] [PubMed]
- De Bruyne B, Pijls NH, Kalesan B, et al. Fractional flow reserve-guided PCI versus medical therapy in stable coronary disease. N Engl J Med 2012;367:991-1001. [Crossref] [PubMed]
- Fearon WF, Nishi T, De Bruyne B, et al. Clinical Outcomes and Cost-Effectiveness of Fractional Flow Reserve-Guided Percutaneous Coronary Intervention in Patients With Stable Coronary Artery Disease: Three-Year Follow-Up of the FAME 2 Trial (Fractional Flow Reserve Versus Angiography for Multivessel Evaluation). Circulation 2018;137:480-7. [Crossref] [PubMed]
- Siebert U, Arvandi M, Gothe RM, et al. Improving the quality of percutaneous revascularisation in patients with multivessel disease in Australia: cost-effectiveness, public health implications, and budget impact of FFR-guided PCI. Heart Lung Circ 2014;23:527-33. [Crossref] [PubMed]
- Murphy JC, Hansen PS, Bhindi R, et al. Cost benefit for assessment of intermediate coronary stenosis with fractional flow reserve in public and private sectors in australia. Heart Lung Circ 2014;23:807-10. [Crossref] [PubMed]