Delayed uptake and washout of contrast in non-viable infarcted myocardium shown with dynamic computed tomography
Introduction
Heart failure secondary to coronary artery disease is a major health issue in the western world affecting a substantial percentage of the population, and inducing major costs to the healthcare systems (1,2). The assessment of ischemic and potentially viable myocardium plays an important role in planning of revascularization of patients with critical coronary atherosclerosis (3-5) as myocardial revascularization in patients with viable myocardium has shown to improve ventricular dysfunction and long-term survival (6,7).
Assessment of myocardial perfusion and viability can be performed by non-invasive modalities including PET, SPECT, and with gadolinium-DTPA-enhanced MRI for detailed assessment and differentiation of viable versus non-viable myocardium (8-13). The nuclear techniques and MRI are advanced time-consuming and expensive techniques, which are not readily available everywhere. Furthermore, several patients groups cannot be investigated with e.g., MR due to claustrophobia, pacemaker or metal devices.
Coronary artery disease is increasingly diagnosed with cardiac computed tomography (CT) scanning and this has increased the interest in CT as an imaging modality to diagnose myocardial viability based on the kinetics of intravascular contrast agents (14). Contrary to MRI the advantages of cardiac CT include superior spatial resolution and the ability to assess myocardial lesions in relation to estimating viability in the same dataset (15-17). Furthermore, cardiac CT is widely available and has a considerably shorter acquisition time compared to MRI, lower cost and it is accessible for almost all patients including e.g., pacemaker patients who are not candidates for MRI.
A number of previous animal studies have shown the clinical potential of CT to diagnose viability and that the extent of myocardial damage and microvascular obstruction can be assessed by cardiac CT (18-20). However, these studies do not accurately evaluate on an individual basis the potential emerging clinical usage of cardiac CT to differentiate viable from non-viable tissue. In particular the regional wall motion abnormalities have not been considered. Thus, the aim of the present study was to use dynamic CT with optimal spatial and temporal resolution to investigate the pattern of contrast distribution over time in pigs with experimentally induced antero-septal myocardial infarction and evaluate the uptake/washout kinetics in the infarcted myocardium and remote healthy myocardium as an indicator of viable myocardium. Dynamic CT acquisition allows us to use the regional wall motion abnormalities for correlating impaired contraction with the infarct zone.
Methods
We used a porcine model since the human and the porcine hearts are anatomically and physiologically similar (21). The surgical procedures were conducted after approval from the Danish Inspectorate of Animal Experimentation.
Chronic porcine infarct model
Twelve 50 kg female mixed Danish landrace and Yorkshire pigs were used in this study. Each animal received an injection with dormicum (0.5 mg/kg) and stresnil (0.5 mg/kg) before transport from the farm facilities to the laboratory where the animals were further pre-anesthetized with midazolam (0.5 mg/kg) and ketaminol (2.5 mg/kg) to allow endotracheal intubation and coupling to a ventilator. Anesthesia was maintained with 1.5% inhalational sevoflurane (mean alveolar concentration: 1-2%) and analgesia with intravenous fentanyl infusion (1-2 µg/kg) at the beginning of surgery and before balloon-occlusion. The entire procedure was performed with ECG monitoring. To prevent both ventricular and supraventricular arrhythmias, amiodarone (50 mg) was injected intravenously before surgery.
The left common carotid artery was surgically exposed to insert a uni-directional 7F sheath. Balloon occlusion of the left anterior descending artery was performed guided by fluoroscopy. Through a launcher catheter a guide-wire was placed in the target coronary vessel and a 2.5-3.0 mm balloon was positioned and inflated immediately distal to the first diagonal branch (Figure 1). After 60 minutes of occlusion, the balloon was deflated, and the guide-wire and catheters were withdrawn and the sheath removed from the vessel which was ligated to secure hemostasis. All twelve pigs developed ventricular fibrillation during the balloon occlusion. Eight pigs were successfully defibrillated. Four pigs died from intractable VF. The neck incision was closed in three layers and the pig was aroused and transported back to the farm when its condition was considered acceptable.
Dynamic CT protocol
After 6 weeks all pigs (N=8) underwent dynamic CT scans using an intravascular contrasts agent. Pre-anesthesia and anesthesia followed the same procedure as during surgery and the pigs were ventilated mechanically during the CT scan. The CT scans were obtained using a Siemens Definition Flash, Siemens Healtcare, Erlangen, Germany. We performed helical, retrospective ECG-gated CT scans in the supine position at 20 s, 1, 3, 5, 8 and 12 min after contrast infusion. The scanner settings were 120 kV, 785 mAs, gantry rotation speed of 0.28 s, pitch 0.23 and a detector collimation of 66 mm × 0.6 mm with a flying focus spot. Slice thickness was 0.75 mm and slices were reconstructed with 20% overlap. The contrast media was optiray 350 mg/mL and 90 mL was used. Contrast was injected by a multiphase contrast administration regime (6 cc/s) immediately followed by a saline flush (5 cc/s). A test bolus of 10 mL was performed to obtain best timing for the arterial phase app. 20 s. Images were reconstructed using dedicated cardiac filters (B26f and I26f) and reconstruction was performed for every 5% of the cardiac cycle to get 4D image loop sets. All pigs received 400 microgram of nitroglycerine sublingually just prior to contrast injection in order to mimic the clinical setting.
CT image analysis
Images were analyzed on a dedicated workstation using Siemens a Multimodality Workplace, syngo 2009B. The dynamic 4D CT images enables identification of dysfunctional myocardium, and both dynamic short and long axial images were evaluated for infarct location (Figures 2,3). Slice thickness was 0.67 mm. The window centre and width were fixed at 200 and 1,000 Hounsfield units (HU), respectively.
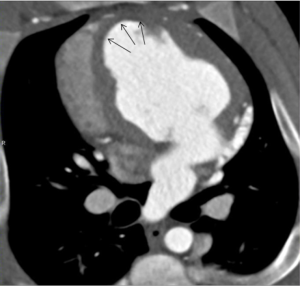
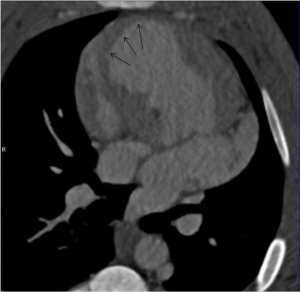
Measurements of HU in the infarct zone and the normal lateral wall were performed at 20 s, and at 1, 3, 5, 8 and 12 min after contrast injection. The zone of infarction was defined as the hypo-enhanced sub-endocardium in the antero-septal wall without wall-motion. It was identified using still-images (Figures 2,3) combined with dynamic images. The location was confirmed by post mortem macroscopic inspection after removal of the heart. Previous studies have documented and published histological data confirming the extent of myocardial infarction (20). The remote myocardium in the lateral wall was defined by normal contractility and clearly away from the infarct zone. Due to its thin myocardium, the posterior wall was not selected to reduce noise. At all time-points HU measurements were performed in three different adjacent levels of the heart in the infarct zone and the zone of remote myocardium. Each measurement region of interest constituted 100-104 pixels.
Statistical analysis
The data were analyzed using STATA software version 10.0. ANOVA was used to test overall difference between regions. Student’s t-test was used to detect statistically significant differences in attenuation values between the anterior wall with infarction and the lateral wall. Results are reported as means [standard deviation (SD)] for continuous variables and a P value of less than 0.05 indicated statistical significance. The ratio between the attenuation values of the two zones was calculated. We used a paired t-test to investigate significant differences comparing the mean ratio between early and late attenuation values measurements in the infarct to that in the lateral wall.
Results
Four pigs developed ventricular fibrillation and died during balloon occlusion. Consequently, eight pigs survived for cardiac CT follow-up at 6 weeks. Results from HU measurements in the infarcted and remote myocardium of the lateral wall are shown in Table 1 and depicted in Figure 4. At baseline (20 s) attenuation values in terms of HUs was significantly lower in the infarct than in the remote normal lateral wall {27 [12] vs. 84 [15], P<0.001}. Mean attenuation values for both zones reached its maximal intensity at 1 minute {95 [26] and 93 [16], P=0.846}. For the remaining time points the infarcted zone showed higher HU values (P<0.01). These results indicate that the remote myocardium showed a more rapid uptake and washout of contrast compared to the infarct zone.

Full table
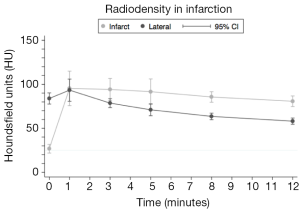
The ratio between attenuation values in the infarcted and the remote myocardium was calculated at all time-points. As shown in Figure 5, the ratio was initially low (0.31) but increased abruptly after 1 minute. This would indicate that accumulation of contrast is decreased in the infarcted myocardium and once trapped in the coronary microcirculation of the infarct clearance is decreased from this region.
The ratio between early (20 s) and late (12 min) attenuation values would depict the ability to accumulate and release contrast in the infarct and in the remote myocardium. On average the ratios are very different 0.33 vs. 1.44 (P<0.001). As depicted in Figure 6 there is no overlap between the ratios between the two areas in all the animals. All infarct zones have a ratio between early and late HU values above 1 and all normal areas has a ratio below 1.
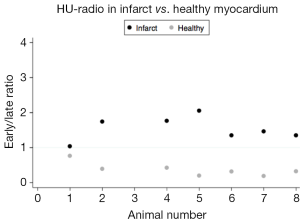
Discussion
The major finding in the present study is that viable myocardium is easily and accurately assessable with dynamic cardiac CT in a chronic infarct setting. Our results confirmed that infarcted myocardium was characterized by initial hypo-enhancement compared to the remote myocardium. Both zones exhibited an early peak in attenuation values at one minute after contrast injection. Conversely, at the following time-points values were lower in the remote myocardium. This likely reflects the formation of collagenous scars in the infarct zone which causes alteration of the characteristics of contrast distribution with slower wash-in and wash-out kinetics.
In the setting of chronic ischemic heart disease, assessment of residual myocardial viability is of major clinical importance for patients with reversible myocardial dysfunction since it provides the rationale for either aggressive revascularization or conservative medical therapy and also predicts the likelihood of functional improvement (5,22). The fact that cardiac CT will yield accurate anatomical and in particular coronary anatomical information in the same dataset is important clinically.
Other studies yield support to our findings, and quantitative assessment of infarcted myocardium mass by MRI and CT correlates (23,24). Lardo et al. found that CT was able to distinguish dysfunctional but viable myocardium from non-viable myocardium by comparing attenuation values in an occlusion/reperfusion animal study with MRI. While these studies have demonstrated a tendency towards a more rapid washout, our ratio (Figure 6) allows the distinction between viable and non-viable tissue on an individual basis. Lardo et al. and Brodoefel et al. found an attenuation value peak in the infarct zone after 5 min. However, the imaging protocol of these studies did not include time-points between 0 and 5 min, and the differences in early perfusion as elucidated by the present study was not identified.
In our study all eight myocardial infarcts were chronic, i.e., no ongoing ischemia since the affected myocardium was reperfused immediately following balloon occlusion. The sensitivity for diagnosing viability with CT has been suggested to be optimal for chronic infarctions (25). However, recent studies have also shown promising diagnostic accuracy for more acute ischemia (26). A major concern in adopting CT for assessment of viable myocardium is the additional radiation exposure. In our study a 120 kV protocol was used, but several studies shows promising results with as low as 80 or 90 kV protocols for the assessment of viable myocardium (27,28). This would lower the radiation considerably and with only one extra low dose scan after 10 min e.g., flash scan as suggested by our data, this will add very little in terms of radiation exposure. Furthermore, in a clinical setting using new edge detection methodology would make it possible to use as low as 4% radiation for left ventricular measurements. This will be adequate for measuring the ejection fraction and evaluate the contractility over time.
CT imaging was performed 6 weeks after reperfusion, to insure the infarction process was complete and no further remodeling was ongoing. As stated above, our infarcts are chronic infarcts, which is preferable since it reflects the relevant patient group we want to investigate. Viability imaging is not important in the case of acute myocardial infarction, where vascularization must be performed rapidly. However, it is relevant in chronic patients, for instance patients suffering from dilated cardiomyopathy. The occlusion time and our time intervals were based on experience from previous studies and by the dynamics of contrast distribution, since it shows the most rapid changes in attenuation values at the earlier time-points (20,24,29). These different time-points do not provide direct cut-off discrimination between infarcted vs. remote segments of myocardium. However, a cut-off (1) is provided by the calculated ratio between early and late attenuation values (Figure 6) also indicating that only two measurements are required.
Furthermore, in the clinic situation it can be difficult to correlate the dynamic images obtained by echocardiography with CT images. Using a dynamic CT imaging protocol such correlation problems can be avoided.
In addition to the assessment of the myocardial viability patterns, there are several other clinical implications of cardiac CT. Because fast rotation time and multi-slice acquisition with a high spatial resolution are combined, cardiac CT could, in an acute setting, provide rapid information about changes in dynamic parameters of myocardial infarction such as alteration of overall left ventricular geometry and—although not specifically addressed in the present study—simultaneous changes in coronary artery morphology. The results provided by our study confirms that dynamic CT viability imaging can provide the information for the planning of therapeutic strategy of myocardial infarction, whether to choose aggressive revascularization or conservative medical therapy. Since CT systems are standard equipment in almost every emergency department this method of assessment will likely be cost-effective.
Study limitations
The present study was conducted in pigs without coronary arthrosclerosis. Clinically, infarct characterization in patients might not be as homogeneous. Furthermore, all eight myocardial infarctions present in the study population were chronic (6 weeks). It is not well described how the applications will perform in sub-acute infarct or in an acute setting.
Using sevoflurane might have resulted in a smaller infarct than if using another anesthetic but the infarcts had a suitable size and if larger infarcts were induced the survival rate would have been even lower. Also, no gold standard modalities were used for comparison purposes and HU measurements was used as a surrogate marker for real contrast concentrations. Finally, image analysis is to some extent subjective although both motion and several planes were averaged. Further studies will need to address the issue of reproducibility and inter-observer uniformity, and possibly introduce a method of automated quantitative data analysis.
Conclusions
In conclusion cardiac CT seems to provide useful viability information with a slightly modified imaging protocol to the standard coronary anatomy image acquisition. This may hold promise to be a cost-effective way of obtaining clinical information in a precise manner in patients with severe coronary artery disease.
Acknowledgements
Research secretary Jette Breiner is warmly acknowledged for her highly valuable contribution. The study was made possible by a donation from the Danish Ministry of Science, Innovation and Higher Education.
Disclosure: The authors declare no conflict of interest.
References
- Roger VL, Go AS, Lloyd-Jones DM, et al. Heart disease and stroke statistics--2012 update: a report from the American Heart Association. Circulation 2012;125:e2-e220. [PubMed]
- Lewis EF. Beyond the P value: The quest for improving health status in patients with ischemic heart disease. Circulation 2010;122:1664-6. [PubMed]
- Reimer KA, Lowe JE, Rasmussen MM, et al. The wavefront phenomenon of ischemic cell death. 1. Myocardial infarct size vs duration of coronary occlusion in dogs. Circulation 1977;56:786-94. [PubMed]
- Nikolaou K, Sanz J, Poon M, et al. Assessment of myocardial perfusion and viability from routine contrast-enhanced 16-detector-row computed tomography of the heart: preliminary results. Eur Radiol 2005;15:864-71. [PubMed]
- Gutberlet M, Fröhlich M, Mehl S, et al. Myocardial viability assessment in patients with highly impaired left ventricular function: comparison of delayed enhancement, dobutamine stress MRI, end-diastolic wall thickness, and TI201-SPECT with functional recovery after revascularization. Eur Radiol 2005;15:872-80. [PubMed]
- Alderman EL, Fisher LD, Litwin P, et al. Results of coronary artery surgery in patients with poor left ventricular function (CASS). Circulation 1983;68:785-95. [PubMed]
- Pagley PR, Beller GA, Watson DD, et al. Improved outcome after coronary bypass surgery in patients with ischemic cardiomyopathy and residual myocardial viability. Circulation 1997;96:793-800. [PubMed]
- Kim RJ, Fieno DS, Parrish TB, et al. Relationship of MRI delayed contrast enhancement to irreversible injury, infarct age, and contractile function. Circulation 1999;100:1992-2002. [PubMed]
- Beek AM, Kühl HP, Bondarenko O, et al. Delayed contrast-enhanced magnetic resonance imaging for the prediction of regional functional improvement after acute myocardial infarction. J Am Coll Cardiol 2003;42:895-901. [PubMed]
- Knuuti MJ, Saraste M, Nuutila P, et al. Myocardial viability: fluorine-18-deoxyglucose positron emission tomography in prediction of wall motion recovery after revascularization. Am Heart J 1994;127:785-96. [PubMed]
- Nagueh SF, Mikati I, Weilbaecher D, et al. Relation of the contractile reserve of hibernating myocardium to myocardial structure in humans. Circulation 1999;100:490-6. [PubMed]
- Constantine G, Shan K, Flamm SD, et al. Role of MRI in clinical cardiology. Lancet 2004;363:2162-71. [PubMed]
- Wu KC, Lima JA. Noninvasive imaging of myocardial viability: current techniques and future developments. Circ Res 2003;93:1146-58. [PubMed]
- Koyama Y, Mochizuki T, Higaki J. Computed tomography assessment of myocardial perfusion, viability, and function. J Magn Reson Imaging 2004;19:800-15. [PubMed]
- Nikolaou K, Poon M, Sirol M, et al. Complementary results of computed tomography and magnetic resonance imaging of the heart and coronary arteries: a review and future outlook. Cardiol Clin 2003;21:639-55. [PubMed]
- Nieman K, Cademartiri F, Lemos PA, et al. Reliable noninvasive coronary angiography with fast submillimeter multislice spiral computed tomography. Circulation 2002;106:2051-4. [PubMed]
- Becker CR, Knez A, Leber A, et al. Detection of coronary artery stenoses with multislice helical CT angiography. J Comput Assist Tomogr 2002;26:750-5. [PubMed]
- Huber DJ, Lapray JF, Hessel SJ. In vivo evaluation of experimental myocardial infarcts by ungated computed tomography. AJR Am J Roentgenol 1981;136:469-73. [PubMed]
- Slutsky RA, Mattrey RF, Long SA, et al. In vivo estimation of myocardial infarct size and left ventricular function by prospectively gated computerized transmission tomography. Circulation 1983;67:759-65. [PubMed]
- Lardo AC, Cordeiro MA, Silva C, et al. Contrast-enhanced multidetector computed tomography viability imaging after myocardial infarction: characterization of myocyte death, microvascular obstruction, and chronic scar. Circulation 2006;113:394-404. [PubMed]
- Weaver ME, Pantely GA, Bristow JD, et al. A quantitative study of the anatomy and distribution of coronary arteries in swine in comparison with other animals and man. Cardiovasc Res 1986;20:907-17. [PubMed]
- Kim RJ, Wu E, Rafael A, et al. The use of contrast-enhanced magnetic resonance imaging to identify reversible myocardial dysfunction. N Engl J Med 2000;343:1445-53. [PubMed]
- Gerber BL, Belge B, Legros GJ, et al. Characterization of acute and chronic myocardial infarcts by multidetector computed tomography: comparison with contrast-enhanced magnetic resonance. Circulation 2006;113:823-33. [PubMed]
- Brodoefel H, Klumpp B, Reimann A, et al. Late myocardial enhancement assessed by 64-MSCT in reperfused porcine myocardial infarction: diagnostic accuracy of low-dose CT protocols in comparison with magnetic resonance imaging. Eur Radiol 2007;17:475-83. [PubMed]
- Nikolaou K, Knez A, Sagmeister S, et al. Assessment of myocardial infarctions using multidetector-row computed tomography. J Comput Assist Tomogr 2004;28:286-92. [PubMed]
- Hoffmann U, Millea R, Enzweiler C, et al. Acute myocardial infarction: contrast-enhanced multi-detector row CT in a porcine model. Radiology 2004;231:697-701. [PubMed]
- Gerber BL, Rochitte CE, Melin JA, et al. Microvascular obstruction and left ventricular remodeling early after acute myocardial infarction. Circulation 2000;101:2734-41. [PubMed]
- Paul JF, Wartski M, Caussin C, et al. Late defect on delayed contrast-enhanced multi-detector row CT scans in the prediction of SPECT infarct size after reperfused acute myocardial infarction: initial experience. Radiology 2005;236:485-9. [PubMed]
- Brødløs HK, Bramsen MB, Agger P, et al. A catheter based chronic porcine model of post-infarct dilated heart failure. Scand Cardiovasc J 2009;43:260-6. [PubMed]