Epicardial adipose tissue: far more than a fat depot
Introduction
Cardiovascular disease is the largest cause of death in the world, and coronary artery disease (CAD) makes up the greatest proportion of those deaths (1). Considerable time and effort has been invested identifying cardiovascular risk factors that may contribute to early stages of CAD, and the search continues for novel factors that may enable more sensitive measures for risk stratification, or offer novel therapeutic targets for the prevention and treatment of coronary vascular disease.
Excess adiposity has long been associated with increased cardiovascular disease (2). There are many plausible mechanisms that could account for this, including changes in blood pressure, blood glucose regulation, lipid metabolism and overall systemic inflammation (3). Furthermore, adipose tissue secretes multiple exocrine and paracrine factors that may directly influence vessel wall homeostasis by altering the function of endothelial cells, arterial smooth muscle cells and macrophages, leading to the development of atherosclerotic plaques in the vessel wall (4). There is substantial evidence that visceral adipose tissue (VAT), defined as fat in the trunk and abdomen, confers a much higher cardiovascular risk than peripheral adiposity [subcutaneous adipose tissue (SCAT)] that is present on hips and thighs (4-6). Thus measurement of waist-to-hip ratio is favoured as a better predictor of cardiovascular disease than the traditionally used BMI (6). A limitation of waist-to-hip measurements is that population studies indicate that general obesity is not necessarily associated with cardiovascular disease, and that not all obese patients present a similar risk profile (7). For example, a large waist circumference may not necessarily be indicative of a large amount of abdominal visceral fat but rather may be due to substantial accumulation of subcutaneous fat, which is often associated with a benign cardiometabolic profile (8). It is becoming increasingly evident that cardiovascular risk is not simply linked to the quantity of adipose tissue, but more importantly the location in which it accumulates. Regional fat distribution appears to play a significant role in cardiovascular disease and recent work has shown that regional fat deposits have a variety of functions other than simply a storage depot for fat.
Adipose tissue that directly surrounds the heart, which is in direct contact with the coronary vasculature, is of particular interest. This fat deposit is known as epicardial adipose tissue (EAT). Rapid advancement in non-invasive imaging techniques such as multidetector computed tomography (MDCT), echocardiography and cardiac magnetic resonance (CMR) imaging has allowed for the accurate measurement and quantification of this local fat depot. This review will focus on evidence suggesting a role for epicardial fat as a quantifiable independent risk marker for cardiovascular disease, as well as describing the role it may have as an active player in the development and vulnerability of coronary artery plaque in the arteries that it surrounds.
Discussion
Definition of cardiac fat deposits
A variety of terms including ‘epicardial’, ‘pericardial’, ‘paracardial’ and ‘intra-thoracic’ have been used to describe adipose tissue deposits in proximity to the heart or within the mediastinum. The use of these terms appears to be a point of confusion, as there is vast variation and inconsistency with their use within the literature. Of particular confusion is the term used to describe the adipose tissue that is found between the outer wall of the myocardium and the visceral layer of the pericardium, thus describing the fat that is fully enclosed within the pericardial sac. This has previously been described in the literature as “pericardial fat” (9-12), while other groups have referred to this same fat deposit as “epicardial fat” (13-18). These terms are not simply interchangeable, as others use the term “pericardial fat” to describe the fat that is contained within the pericardial sac combined with the fat found on the external surface of the parietal pericardium (19-22).
As illustrated in Figure 1, the most accurate term for the adipose tissue—between the epicardial surface of the heart and the visceral surface of the pericardium—that is fully enclosed by the pericardial sac and directly surrounds the coronary arteries is “EAT”. Fat that is present on the external surface of the parietal pericardium is known as paracardial fat or mediastinal fat, and the term pericardial describes the combination of the adipose tissue within the pericardium and the paracardial adipose tissue that surrounds the external surface of the parietal pericardium.
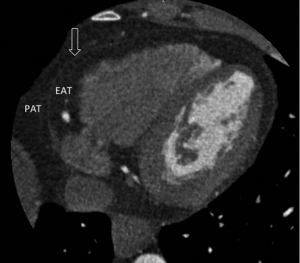
Difference between epicardial and paracardial adipose tissue
It is very important to differentiate adipose tissue within the pericardium as “epicardial” and adipose tissue outside the pericardium as “paracardial” as they are quite different, not only anatomically, but also embryologically and biochemically. Under physiological conditions EAT constitutes approximately 20% of cardiac weight (23). It is found to be most concentrated in the atrioventricular and interventricular grooves, and as a consequence the coronary arteries and their major branches are often embedded within this tissue. Smaller fat foci are also found along the free walls of the atria and around the two atrial appendages, and a small amount often extends into the myocardium following branches of the coronary arteries (24). As the amount of epicardial fat increases, it begins to cover the walls of the ventricles, and in extreme cases may even cover the entire epicardial surface of the heart.
EAT is derived from the splanchonopleuric mesoderm associated with the gut, and is of the same embryologic origin as mesenteric and omental fat cells, having evolved from brown adipose tissue during embryogenesis (24-26). EAT is composed of far more than simply adipocytes; also containing nervous and nodal tissue, as well as inflammatory, stromal and immune cells within it (27).
The coronary arteries perfuse the EAT and also supply the myocardium (28). They are in direct contact with one another given there is no fascia or similar structure that separates EAT from the adventitial surface of the coronary arteries or the myocardium. This facilitates an environment for a local interaction between EAT and the coronary arteries that it directly opposes.
Paracardial adipose tissue (PAT) on the other hand refers to the fat depot that is superficial to the pericardium. Under physiologic conditions it covers 80% of the heart and constitutes between 20-50% of the cardiac mass (23). In contrast to EAT, PAT originates from the primitive thoracic mesenchyme, which splits to form the parietal pericardium and the outer thoracic wall (20,24). PAT is not perfused by the coronary arteries but rather supplied by branches of the internal mammary artery such as the pericardiacophrenic artery (20,24). These are clearly two vastly different cardiac fat depots, and as Iacobellis et al. has rightly pointed out, it is imperative to differentiate between them (29).
Quantifying EAT
EAT can be relatively easily measured by a variety of different imaging techniques (Table 1). EAT fat thickness was first visualized by and measured with Transthoracic Two-dimensional (2D) Echocardiography. EAT is visible as an echo free space between the outer wall of the myocardium and the visceral layer of the pericardium. The thickness of this space is measured on the right ventricular free wall in the parasternal long and short axis views where EAT is thought to be thickest (35). Using this technique Iacobellis et al. reported excellent interobserver and intraobserver agreement with an intraclass coefficient of 0.90 and 0.98, respectively (30). Another study found the echocardiographic concordance of EAT to be poor with an intraobserver intraclass correlation of 0.625 and an interobserver intraclass correlation of 0.605 (36). Echocardiographic measurement of EAT thickness is a cheap and readily available non-invasive measurement. It does, however, have the limitation of not being truly volumetric, as it is a linear measure at a single location. Therefore it does not reflect variability in fat thickness or provide information about the total fat volume. Whether echocardiographic EAT thickness measured at a single point is an accurate surrogate marker for total EAT volume is doubtful considering the significant inter-individual differences in EAT distribution. Three-dimensional echocardiography may be able to provide more accurate volumetric assessment of EAT in the future.
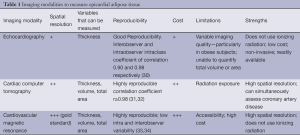
Full table
Computed tomography (CT) has also been used to measure EAT (Figure 2). Given the high spatial resolution of CT and that adipose tissue has distinct attenuation values, EAT is readily identified allowing for accurate volumetric measurement. Volumetric quantification of EAT involves identification of the limits of the heart and the pericardium, followed by tracing of the pericardium to exclude any extra-cardiac fat. This can be done manually or via a semi-automated process. Software is readily available that can be used to identify and quantify EAT on the basis of thresholds for CT attenuation.
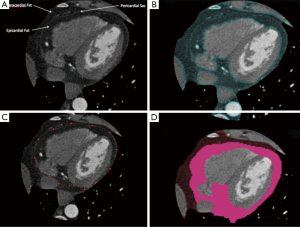
Nakazato et al. demonstrated excellent interscan reproducibility using a semi-automated method with a correlation coefficient of ≥0.98 (31). CT disadvantages include exposure to ionizing radiation and a relatively high cost, making it somewhat poorly accessible and impractical for daily practice.
CMR imaging can also be used to measure EAT, with excellent image resolution it is considered the ‘gold standard’ modality for imaging adipose tissue (37,38). Similar to CT, a tracing is obtained of the pericardial sac and the adipose tissue voxels in each slice are added to calculate the total volume of this tissue. The use of CMR has been validated as an accurate and reproducible modality for the assessment of EAT (33). While the image resolution and accuracy of CMR is greater than CT, it is also far more costly and less accessible at this stage, which significantly limits its utility.
As has been described, EAT can be quantified by measuring either the thickness at a single point or volumetric quantification of total EAT volume. Using cardiac dual source CT, Bastarrika et al. performed a comparison between the two methods to determine which was the most reproducible and which correlated best with the extent of CAD. They found using automated volumetry is more reproducible than a manual bidimensional approach which is based on thickness measurements. In addition, using automated volumetry, patients with significant coronary artery stenosis had significantly greater EAT volumes (154.58±58.91 mL) than those without significant coronary artery stenosis (120.94±81.85 mL) (P=0.016). Yet, epicardial distance measurements failed to show any significant difference between these two groups (39). Thus, volumetric quantification of total EAT is not only more reproducible but also correlates better with extent of CAD.
EAT—biologically active organ
It has become evident that adipose tissue is far more than simply a fat storage depot, but rather a biologically active organ that may play a central role in the association between obesity and cardiovascular disease (3). The metabolic profile of EAT is dependent on the metabolic context of the patient. EAT and PAT are normally present in humans, and under normal conditions EAT is thought to serve a number of physiologic functions, including acting as an energy source to the myocardium. EAT has a greater capacity for the uptake and release of free fatty acids (FFAs) and has a lower rate of glucose utilization than other visceral fat depots (40). Fatty acids are the major energy source for the contractile function in a healthy heart (41), and FFA oxidation is responsible for about 50-70% of the energy production of the heart. The myocardium sources these FFAs via the coronary arteries, which the EAT surrounds. Given that EAT has such high rates of FFA synthesis, incorporation, and breakdown, it is thought that EAT may actually act as a buffer between the myocardium and the local vasculature, scavenging excess FFAs and thus protecting the heart from lipotoxicity (25). In addition, the high lipolytic activity of EAT suggests that it may also play a role as a ready source of FFAs to meet increased myocardial energy demand through the β-oxidation of FFAs (42). EAT has also been shown to be a source of many putatively beneficial adipokines with anti-atherogenic and anti-inflammatory effects, of which adiponectin appears to be most important with its anti-atherosclerotic properties (43,44). Anatomically EAT provides mechanical protection of the coronary arteries, buffering them against the torsion induced by the arterial pulse wave and cardiac contraction (45).
EAT is, however, a visceral fat deposit and as such has the capacity to secrete a number of cytokines and chemokines, known collectively as adipokines. These are biologically active molecules that are secreted by mature adipocytes themselves, as well as stromal preadipocytes, macrophages, fibroblasts, mast cells and lymphocytes that are located within the adipose tissue (46). Interleukin (IL)-1β, -6, -8 and -10, tumor necrosis factor α (TNF-α), monocyte chemoattractive protein 1 (MCP-1), adiponectin, leptin and plasminogen activator inhibitor 1 (PAI-1) are all examples of adipokines (47). Adipokines can have both endocrine and paracrine functions that influence the development of cardiovascular disease, and in particular CAD (47). For example, elevated IL-6, TNF-α, MCP-1 and decreased adiponectin have been correlated with the development of atherosclerosis (48).
Adipokines produced by EAT are thought to gain access and thus influence underlying atherosclerotic plaque development via two main mechanisms: paracrine and vasocrine action (Figure 3). In paracrine signalling, it is hypothesised that adipokines produced by EAT surrounding the coronary vessels could diffuse directly through the layers of the vessel wall via the interstitial fluid to interact with the vasa vasorum, vascular smooth muscle cells, endothelium and the cellular components of the plaque leading to the initiation of inflammation, the development of atherosclerosis and changes in plaque phenotype. An alternative vasocrine signalling mechanism has been proposed in which it is hypothesized that adipokines secreted from the EAT directly enter the lumen of closely opposed adventitial vasa vasorum and thus are transported downstream into the arterial wall where they may act on cells in and around the atherosclerotic plaques (20,40).
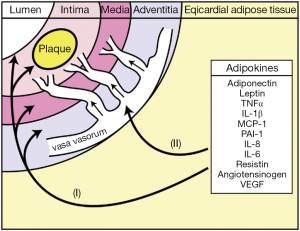
EAT—mediator of local inflammation
While at normal quantities, there are many putative physiologic functions of EAT. In obesity however, many changes occur as EAT volumes substantially increase. These changes are often characterized by hypertrophy, failure to store triglycerides, increased lipolysis and inflammation (43). As EAT expands it becomes hypoxic and dysfunctional (49) and is invaded by increased numbers of macrophages and T lymphocytes, resulting in a shift in its metabolic profile. The result is increased secretion of pro-inflammatory cytokines such as TNF-α, MCP-1, IL-1β, IL-6 and many others that contribute to the inflammatory environment characteristic of atherogenesis (27), as well as reduced secretion of anti-atherosclerotic adipokines such as adiponectin (50). In this setting, the beneficial paracrine effects of EAT are lost, and such dysfunctional changes may play a causative role in local inflammation, with the potential to influence the development and progression of coronary artery plaque.
EAT—development of CAD
Atherosclerosis has long been recognised as an inflammatory disease (51), involving an ongoing inflammatory response that has been shown to play a fundamental role in mediating all stages of atherosclerotic plaque, from initiation through to progression, and ultimately in the thrombotic complications of atherogenesis (48). Many studies have linked long-term cardiovascular risk in both healthy populations and those with established CAD with circulating levels of inflammatory cytokines such as IL-6 and TNF-α (52-54) and raised high-sensitivity C-reactive Protein (55,56). Systemic inflammation and the production of adipokines by adipose tissue are considered important mechanistic contributors to the atherogenic process (4,57). Inflammation from within the vessel wall and from remote extravascular structures such as adipose tissue contributes significantly to atherogenesis. EAT plays a significant role in the inflammatory process that contributes to the inflammatory burden within and around atherosclerotic plaque (40). Mazurek et al. showed that EAT harvested during cardiac surgery from patients with severe CAD contained a substantial inflammatory infiltrate when compared to subcutaneous fat. They demonstrated a significantly higher expression of the chemokine, MCP-1, and inflammatory cytokines, IL-6, IL-1β and TNF-α in EAT compared to leg subcutaneous fat in patients with established CAD. These proinflammatory properties of EAT were independent of plasma concentration of circulating biomarkers as well as patient clinical variables such as diabetes, BMI and chronic statin use (27). Adiponectin, which has been shown to have anti-atherosclerotic properties (44), has been shown to be significantly lower in EAT isolated from patients with severe CAD compared to subjects without CAD (58,59). Iwayama et al. demonstrated the role EAT may play in the early development of CAD via impairment of adiponectin secretion in non-obese individuals, finding that not only was EAT volume significantly greater in non-obese individuals with evidence of CAD compared to non-obese patients with no evidence of CAD, but also adiponectin concentration was significantly lower in the pericardial fluid of non-obese patients with evidence of CAD than non-obese patients with no evidence of CAD (18). In addition, lower adiponectin levels correlate with more complex coronary lesions in men with coronary vascular disease (60). Interestingly, it has long been noted that segments of coronary arteries that course through a myocardial bridge (thus are surrounded by myocardium and free of any immediately adjacent epicardial fat) tend to be free from atherosclerotic plaque and immune to atherosclerosis (61,62). Robicsek et al. reported a study of 250 patients undergoing coronary bypass surgery for severe CAD. In this cohort, 26 of these patients had a circumflex that gave off a major branch that ran entirely intramyocardially, but required bypass due to severe proximal disease. Of these 26 patients, 24 had no evidence of atherosclerosis in the myocardial segment (63). While this may lend support for a paracrine effect of EAT in the development of atherosclerosis, it must be noted that there is significantly altered flow dynamics within the myocardial segments that may account somewhat for these changes. Additionally, it has been shown that EAT is not required for the development of coronary plaque, as autopsies of patients with congenital generalized lipodystrophy that possess a total absence of EAT, VAT and SCAT, still demonstrated evidence of CAD (64). This does not, however, exclude a secondary role for EAT in the development of CAD, given its association with the development of CAD that will be addressed in the next section.
EAT—association with coronary atherosclerotic plaque
A significant amount of research has investigated the link between increased Epicardial fat volume (EFV) and CAD (Table 2). The presence of coronary artery calcification is intimately associated with vascular injury and atherosclerotic plaque and is considered diagnostic of the presence of coronary atherosclerosis (73). Rosito et al. investigated 1,155 patients free of cardiovascular disease from the Framingham Heart Study that underwent MSCT for quantification of paracardial fat volume, EFV and coronary artery calcium (CAC). They found that epicardial fat, but not paracardial fat was associated with coronary artery calcification even after adjustment for cardiovascular risk factors [odds ratio (OR): 1.21; 95% CI, 1.005-1.46; P=0.04] (11). In a study of 159 patients without known CAD, selected from the Multi-Ethnic Study of Atherosclerosis (MESA), Ding et al. measured CAC and the adipose tissue volume located around the proximal coronary arteries. They found that the volume of adipose tissue was greater in those with a calcified coronary artery plaque than those with no evidence of calcified coronary plaque, as assessed by MSCT (21). They showed that peri-coronary adipose tissue volume (defined as a region of fat surrounding the left main coronary artery) was positively associated with both the presence and amount of calcified coronary artery plaque, even after adjustments for other cardiovascular risk factors. In addition, they demonstrated that BMI and height-adjusted waist circumference in their cohort were not associated with the presence of coronary artery plaque. While it must be noted that this group did not measure total EFV, they demonstrated that their method of measuring peri-coronary fat correlated highly with total EFV quantification on CT (Pearsons correlation coefficient: 0.93; P<0.001). Many other studies have also demonstrated a link between EAT volume and CAD using coronary calcification as a marker for CAD (11,17,74,75). Additionally, in a cohort of 330 asymptomatic diabetic patients with no prior history of CAD, Yerramasu et al. investigated the association of EAT with progression of CAC defined as >2.5 mm3 increase in square root transformed volumetric CAC scores. They found that EAT showed significant association with CAC progression (OR: 1.12; 95% CI, 1.05-1.19; P<0.001), finding that for each 10 cm3 increase in the EAT volume, the probability of CAC progression went up by 12% (95% CI, 5-9%; P<0.001) (69). Overall, this demonstrated that local fat depots surrounding coronary arteries were more related to coronary artery calcification than total body fat, and may suggest that EAT plays a role in exerting a toxic effect on the adjacent vasculature.
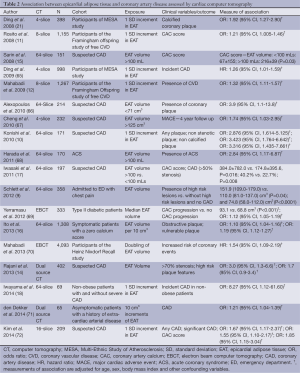
Full table
While the absence of calcified plaque in asymptomatic patients is reassuring and often a good prognosis, there is no doubting that CAD caused by non-calcified plaques do occur and can result in fatal cardiovascular events (76,77). Ito et al. showed that EFV was a significant predictor of obstructive coronary disease after multivariate adjustment in patients with a zero calcium score (16), demonstrating the utility of EFV as a useful marker of CAD in symptomatic patients with non-calcified plaques. Additionally, the aforementioned studies indicate that EAT is correlated with CAD independent of traditional risk factors and anthropometric measures of obesity (BMI and waist circumference), and that the accumulation of EAT, rather than abdominal obesity, could be implicated in the early development of CAD.
A study by Konishi et al. assessed plaque characteristics in relation to volumetric quantification of EAT in 171 patients. They characterised plaques as calcified, mixed and non-calcified and assessed data for a relationship between plaque characteristics and EAT volume, and found that EAT but not abdominal fat (measured as waist circumference) was independently and significantly associated with the presence of all types of coronary plaques, including both non-stenotic and non-calcified coronary plaques. They found no significant difference among patients with non-calcified, mixed or calcified coronary plaques and EAT volumes (10), concluding that EAT volumes are more associated with early development CAD than other simple anthropometric measures of abdominal obesity such as waist-to-hip ratio.
In contrast, Alexopoulos et al. were able to demonstrate that EAT volume differs significantly between different types of plaque. They were the first to demonstrate that EAT volume was not only greater in patients with coronary atherosclerosis, but was also larger in patients with mixed or non-calcified plaques compared to those with calcified plaques (66). This is of particular significance as non-calcified and mixed plaques are associated with a higher rate of cardiovascular events than purely calcified plaques (78). These results extended previous observations that EAT is associated with the presence of coronary atherosclerosis and began to demonstrate a link between EAT and particular plaque compositions.
The association of EAT volume with the presence of non-calcified plaques would suggest that EAT volume may not only be associated with the presence and extent of coronary atherosclerosis, but that EAT accumulation may precede plaque calcification and the development of mature atherosclerotic plaques in general. As such, EAT could provide a future avenue for risk stratification by enabling detection of those at risk of developing significant CAD while plaques are still immature, or potentially before there is detectable evidence of CAD.
Studies have also investigated the association of EAT volume and characteristics of plaque other than composition, such as size and the degree of stenosis. Numerous studies have examined the association between plaque stenosis and volume of EAT (9,14,17,66,79). The degree of coronary stenosis plays a critical role in the outcome of plaque rupture in coronary arteries. The degree of coronary luminal narrowing is decisive for whether plaque rupture causes occlusive thrombus or just internal hemorrhage: the higher the degree of stenosis the more likely the plaque is to cause an occlusive thrombus upon rupture (80). Several studies have linked increased EAT volume to degree of coronary stenosis, with results showing that greater EAT volumes were an independent risk factor for the development of significant (>50%) coronary artery stenosis (17,79). A more recent study by Rajani et al. also showed that EAT is associated with more severe coronary stenosis (>70%) independent of conventional cardiac risk factors (14). Studies that used a Gensini score, or a modified version of it, to assess severity of CAD also found EAT was an independent predictor for CAD severity (72,81).
Degree of coronary artery stenosis is only one of many characteristics describing plaques, and evidence suggests that lesions most at risk of developing complications of CAD are not necessarily those with the greatest degree of stenosis (82,83). High-risk plaque features that are often measured include positive vessel remodeling or low-attenuation plaques, both of which are associated with a significantly higher risk of the occurrence of an acute coronary artery syndrome (84). These pathological characteristics are considered to make plaques more “high-risk” or “vulnerable”, meaning they are more prone to disruption with subsequent superimposed thrombosis, which is the prime mechanism of most acute coronary syndromes (ACS) (85). A number of studies have focused more specifically on the association of EAT and high risk coronary lesions, and found that EFV is in fact positively associated with high risk plaque characteristics such as positive remodeling, spotty calcification and low-attenuation plaque (9,14). A study by Schlett and colleagues suggested that EFV was significantly associated with high-risk coronary artery lesion morphology. They found that EFV was nearly twice as high in patients with high-risk coronary lesions than in those without CAD. Furthermore, they also found that EFV was still significantly higher in patients with high-risk lesions compared to patients with CAD but with no evidence of high-risk plaque morphology. In summary, they concluded that low EAT volume effectively excluded the presence of high-risk coronary morphology (9).
EAT—cardiovascular outcome
EFV has been shown by many to be associated not only with the presence of CAD, but also with characteristics of coronary plaque that deem these lesions to be “high risk” of rupture and the development of ACS such as myocardial infarct, unstable angina or sudden cardiac death. Consequently, one would expect to see this translate into increased incidence of major adverse cardiac events (MACE) in the population. Increased EFV has been independently linked to adverse cardiovascular outcomes (67). It has been suggested to be an independent risk factor for ACS, with significantly increased EFV shown in patients with ACS when compared to a control group whose coronary CT results were normal (68), as well as being shown to be significantly associated with the presence of myocardial ischemia in patients without known CAD (86,87). Ding et al. conducted a case-cohort study in 998 patients who were randomly selected from 6814 MESA participants and found EAT was associated with CAD [relative risk for increase of one standard deviation (SD) in EAT =1.26, 95% CI, 1.01-1.59] even after adjustment for cardiovascular risk factors. A 1-standard deviation increment in EAT presented as significant a risk for CAD as an 18-pack-year increment in cigarette smoking (65). Mahabadi et al. quantified EAT volume and incidence of coronary events over a follow-up of 8±1.5 years in 4,093 participants in the Heinz Nixdorf Recall study. They found that incidence of coronary events increased with amount of EAT, with subjects in the highest quartile of EAT having a 5 fold higher risk when compared to subjects in the lowest quartile (0.9% vs. 4.7% for 1st and 4th quartiles respectively, P<0.001). In addition, they found that EFV remained independently associated with coronary events, with a doubling of EFV associated with a 1.5 fold risk of coronary events when adjusted for cardiovascular risk factors [hazard ratio (HR), 1.54; 95% CI, 1.09-2.19] (70).
EAT—future research interests
There is growing evidence to suggest that EAT, as a visceral fat depot, may contribute to the development of coronary artery atherosclerosis. Increased EAT correlates not only with increased development of atherosclerotic plaque, but is also associated with the development of high-risk lesions and adverse coronary events. Considering that EAT may precede the development of a mature atherosclerotic plaque, and may contribute to early stages of coronary atherogenesis, it may have a role as a risk stratification tool not only to identity those simply at risk of developing coronary vascular disease, but also to identify those at risk of MACE. Even if EAT is involved in the pathogenesis of coronary atherosclerosis, the question remains whether its quantification would improve risk stratification beyond already existing measures that are used in clinical practice, such as the measurement of coronary calcium score. A study by Forouzandeh et al. showed that while overall risk stratification was improved by combining EFV and CACS severity information, no specific cut off points were found that better defined patients with or without MACE than simply using CACS alone (88). Even though there is a substantial body of evidence from multiple studies linking increased EAT volume to CAD and MACE, the evidence to date would not support performing cardiac CT examination purely for the purpose of quantifying EAT, as the prognostic benefit beyond traditional measurements is not sufficient. However, given that EAT may be the factor triggering atherosclerotic development, its utility may be in screening for subclinical atherosclerosis before the development of coronary calcification. The next step is to investigate the role that EAT volume plays in the development and progression of atherosclerotic plaque, and how its presence and consequent changes to the local environment may alter not only plaque progression but also plaque phenotype. To our knowledge only one study has investigated progression (89), and this was only in regard to coronary artery calcification. It is still unknown, which effects EAT has in the context of coronary atherosclerotic plaque burden, in particular in the development of subclinical atherosclerosis or non-calcified plaque. In addition, there is ongoing research to determine if higher EAT volumes contribute to increased CAD or if progression of EAT alters the microenvironment and leads to inflammatory processes that contribute to atherosclerotic development. Furthermore, before the quantification of EAT may enter the clinician’s arsenal as a tool for risk stratification, more work must be completed to develop normal distribution curves of fat volumes in the population, as little data exists to determine cut off values for what should be considered low, moderate, and severe levels of cardiac fat and how they relate to relative risk of plaque development or MACE.
Conclusions
EAT is the true visceral fat deposit of the heart, as it exists directly on the epicardial surface of the myocardium and is contained entirely within the pericardial sac. As the coronary arteries and their major branches are embedded in this fat, this creates a perfect environment for a local interaction between a metabolically active EAT and the coronary vessels it surrounds. Coronary plaque development and phenotype are hypothesized to be influenced by the inflammatory burden created by altered expression and production of adipokines from EAT. As such, EAT is consistently associated not only with increased atherosclerotic plaque, but also high risk lesions and adverse coronary events. Despite the capacity of different methods to assess EAT there is currently no rationale for its primary use as a risk stratification device, as it is unclear if it provides any greater prognostic benefit than currently employed techniques. Given additional research, the future application of volumetric quantification of EAT may be found to add to the prognostic benefit of pre-existing risk stratification techniques, and screening for sub-clinical atherosclerosis and non-calcified plaque.
Acknowledgements
DW is supported by NHF (Australia) Post-Doctoral fellowships and Robertson Family Research Cardiologist Fund. PP is supported by National Health & Medical Research Institute (NHMRC) Postdoctoral fellowship.
Disclosure: The authors declare no conflict of interest.
References
- The 10 leading causes of death. World Health Organisation. Available online: http://www.who.int/mediacentre/factsheets/fs310/en/index.html. Accessed October 16, 2013.
- Calle EE, Thun MJ, Petrelli JM, et al. Body-mass index and mortality in a prospective cohort of U.S. adults. N Engl J Med 1999;341:1097-105. [PubMed]
- Van Gaal LF, Mertens IL, De Block CE. Mechanisms linking obesity with cardiovascular disease. Nature 2006;444:875-80. [PubMed]
- Fantuzzi G, Mazzone T. Adipose tissue and atherosclerosis: exploring the connection. Arterioscler Thromb Vasc Biol 2007;27:996-1003. [PubMed]
- Okura T, Nakata Y, Yamabuki K, et al. Regional body composition changes exhibit opposing effects on coronary heart disease risk factors. Arterioscler Thromb Vasc Biol 2004;24:923-9. [PubMed]
- Larsson B, Svardsudd K, Welin L, et al. Abdominal adipose tissue distribution, obesity, and risk of cardiovascular disease and death: 13 year follow up of participants in the study of men born in 1913. Br Med J (Clin Res Ed) 1984;288:1401-4. [PubMed]
- Wildman RP, Muntner P, Reynolds K, et al. The obese without cardiometabolic risk factor clustering and the normal weight with cardiometabolic risk factor clustering: prevalence and correlates of 2 phenotypes among the US population (NHANES 1999-2004). Arch Intern Med 2008;168:1617-24. [PubMed]
- Raggi P. Epicardial Adipose Tissue as a Marker of Coronary Artery Disease Risk. J Am Coll Cardiol 2013;61:1396-7. [PubMed]
- Schlett CL, Ferencik M, Kriegel MF, et al. Association of pericardial fat and coronary high-risk lesions as determined by cardiac CT. Atherosclerosis 2012;222:129-34. [PubMed]
- Konishi M, Sugiyama S, Sugamura K, et al. Association of pericardial fat accumulation rather than abdominal obesity with coronary atherosclerotic plaque formation in patients with suspected coronary artery disease. Atherosclerosis 2010;209:573-8. [PubMed]
- Rosito GA, Massaro JM, Hoffmann U, et al. Pericardial fat, visceral abdominal fat, cardiovascular disease risk factors, and vascular calcification in a community-based sample: the Framingham Heart Study. Circulation 2008;117:605-13. [PubMed]
- Mahabadi AA, Massaro JM, Rosito GA, et al. Association of pericardial fat, intrathoracic fat, and visceral abdominal fat with cardiovascular disease burden: the Framingham Heart Study. Eur Heart J 2009;30:850-6. [PubMed]
- Nabati M, Saffar N, Yazdani J, et al. Relationship between epicardial fat measured by echocardiography and coronary atherosclerosis: a single-blind historical cohort study. Echocardiography 2013;30:505-11. [PubMed]
- Rajani R, Shmilovich H, Nakazato R, et al. Relationship of epicardial fat volume to coronary plaque, severe coronary stenosis, and high-risk coronary plaque features assessed by coronary CT angiography. J Cardiovasc Comput Tomogr 2013;7:125-32. [PubMed]
- Sarin S, Wenger C, Marwaha A, et al. Clinical significance of epicardial fat measured using cardiac multislice computed tomography. Am J Cardiol 2008;102:767-71. [PubMed]
- Ito T, Suzuki Y, Ehara M, et al. Impact of epicardial fat volume on coronary artery disease in symptomatic patients with a zero calcium score. Int J Cardiol 2013;167:2852-8. [PubMed]
- Iwasaki K, Matsumoto T, Aono H, et al. Relationship between epicardial fat measured by 64-multidetector computed tomography and coronary artery disease. Clin Cardiol 2011;34:166-71. [PubMed]
- Iwayama T, Nitobe J, Watanabe T, et al. The role of epicardial adipose tissue in coronary artery disease in non-obese patients. J Cardiol 2014;63:344-9. [PubMed]
- Wheeler GL, Shi R, Beck SR, et al. Pericardial and visceral adipose tissues measured volumetrically with computed tomography are highly associated in type 2 diabetic families. Invest Radiol 2005;40:97-101. [PubMed]
- Sacks HS, Fain JN. Human epicardial adipose tissue: a review. Am Heart J 2007;153:907-17. [PubMed]
- Ding J, Kritchevsky SB, Harris TB, et al. The association of pericardial fat with calcified coronary plaque. Obesity (Silver Spring) 2008;16:1914-9. [PubMed]
- Greif M, Leber AW, Saam T, et al. Determination of Pericardial Adipose Tissue Increases the Prognostic Accuracy of Coronary Artery Calcification for Future Cardiovascular Events. Cardiology 2012;121:220-7. [PubMed]
- Corradi D, Maestri R, Callegari S, et al. The ventricular epicardial fat is related to the myocardial mass in normal, ischemic and hypertrophic hearts. Cardiovasc Pathol 2004;13:313-6. [PubMed]
- Iacobellis G, Willens HJ. Echocardiographic epicardial fat: a review of research and clinical applications. J Am Soc Echocardiogr 2009;22:1311-9. [PubMed]
- Marchington JM, Mattacks CA, Pond CM. Adipose tissue in the mammalian heart and pericardium: structure, foetal development and biochemical properties. Comp Biochem Physiol B 1989;94:225-32. [PubMed]
- Ho E, Shimada Y. Formation of the epicardium studied with the scanning electron microscope. Dev Biol 1978;66:579-85. [PubMed]
- Mazurek T, Zhang L, Zalewski A, et al. Human epicardial adipose tissue is a source of inflammatory mediators. Circulation 2003;108:2460-6. [PubMed]
- Iacobellis G, Corradi D, Sharma AM. Epicardial adipose tissue: anatomic, biomolecular and clinical relationships with the heart. Nat Clin Pract Cardiovasc Med 2005;2:536-43. [PubMed]
- Iacobellis G. Epicardial and pericardial fat: close, but very different. Obesity (Silver Spring) 2009;17:625; author reply 626-7. [PubMed]
- Iacobellis G, Willens HJ, Barbaro G, et al. Threshold values of high-risk echocardiographic epicardial fat thickness. Obesity (Silver Spring) 2008;16:887-92. [PubMed]
- Nakazato R, Shmilovich H, Tamarappoo BK, et al. Interscan reproducibility of computer-aided epicardial and thoracic fat measurement from noncontrast cardiac CT. J Cardiovasc Comput Tomogr 2011;5:172-9. [PubMed]
- Nichols JH, Samy B, Nasir K, et al. Volumetric measurement of pericardial adipose tissue from contrast-enhanced coronary computed tomography angiography: a reproducibility study. J Cardiovasc Comput Tomogr 2008;2:288-95. [PubMed]
- Nelson AJ, Worthley MI, Psaltis PJ, et al. Validation of cardiovascular magnetic resonance assessment of pericardial adipose tissue volume. J Cardiovasc Magn Reson 2009;11:15. [PubMed]
- Doesch C, Haghi D, Fluchter S, et al. Epicardial adipose tissue in patients with heart failure. J Cardiovasc Magn Reson 2010;12:40. [PubMed]
- Iacobellis G, Assael F, Ribaudo MC, et al. Epicardial fat from echocardiography: a new method for visceral adipose tissue prediction. Obes Res 2003;11:304-10. [PubMed]
- Saura D, Oliva MJ, Rodriguez D, et al. Reproducibility of echocardiographic measurements of epicardial fat thickness. Int J Cardiol 2010;141:311-3. [PubMed]
- Gronemeyer SA, Steen RG, Kauffman WM, et al. Fast adipose tissue (FAT) assessment by MRI. Magn Reson Imaging 2000;18:815-8. [PubMed]
- Machann J, Thamer C, Schnoedt B, et al. Standardized assessment of whole body adipose tissue topography by MRI. J Magn Reson Imaging 2005;21:455-62. [PubMed]
- Bastarrika G, Broncano J, Schoepf UJ, et al. Relationship between coronary artery disease and epicardial adipose tissue quantification at cardiac CT: comparison between automatic volumetric measurement and manual bidimensional estimation. Acad Radiol 2010;17:727-34. [PubMed]
- Iacobellis G, Bianco AC. Epicardial adipose tissue: emerging physiological, pathophysiological and clinical features. Trends Endocrinol Metab 2011;22:450-7. [PubMed]
- Lopaschuk GD. Metabolic abnormalities in the diabetic heart. Heart Fail Rev 2002;7:149-59. [PubMed]
- Lima-Martínez MM, Blandenier C, Iacobellis G. Epicardial adipose tissue: more than a simple fat deposit? Endocrinol Nutr 2013;60:320-8. [PubMed]
- Fitzgibbons TP, Czech MP. Epicardial and perivascular adipose tissues and their influence on cardiovascular disease: basic mechanisms and clinical associations. J Am Heart Assoc 2014;3:e000582. [PubMed]
- Han SH, Sakuma I, Shin EK, et al. Antiatherosclerotic and anti-insulin resistance effects of adiponectin: basic and clinical studies. Prog Cardiovasc Dis 2009;52:126-40. [PubMed]
- Rabkin SW. Epicardial fat: properties, function and relationship to obesity. Obes Rev 2007;8:253-61. [PubMed]
- Shioji K, Moriguchi A, Moriwaki S, et al. Hypoadiponectinemia implies the development of atherosclerosis in carotid and coronary arteries. J Cardiol 2005;46:105-12. [PubMed]
- McLean DS, Stillman AE. Epicardial adipose tissue as a cardiovascular risk marker. Clin Lipidol 2009;4:55-62.
- Libby P, Ridker PM, Maseri A. Inflammation and atherosclerosis. Circulation 2002;105:1135-43. [PubMed]
- Greenstein AS, Khavandi K, Withers SB, et al. Local inflammation and hypoxia abolish the protective anticontractile properties of perivascular fat in obese patients. Circulation 2009;119:1661-70. [PubMed]
- Greif M, Becker A, von Ziegler F, et al. Pericardial adipose tissue determined by dual source CT is a risk factor for coronary atherosclerosis. Arterioscler Thromb Vasc Biol 2009;29:781-6. [PubMed]
- Ross R. Atherosclerosis--an inflammatory disease. N Engl J Med 1999;340:115-26. [PubMed]
- Lindahl B, Toss H, Siegbahn A, et al. Markers of myocardial damage and inflammation in relation to long-term mortality in unstable coronary artery disease. FRISC Study Group. Fragmin during Instability in Coronary Artery Disease. N Engl J Med 2000;343:1139-47. [PubMed]
- Ridker PM, Rifai N, Pfeffer M, et al. Elevation of tumor necrosis factor-alpha and increased risk of recurrent coronary events after myocardial infarction. Circulation 2000;101:2149-53. [PubMed]
- Ridker PM, Rifai N, Stampfer MJ, et al. Plasma concentration of interleukin-6 and the risk of future myocardial infarction among apparently healthy men. Circulation 2000;101:1767-72. [PubMed]
- Kuller LH, Tracy RP, Shaten J, et al. Relation of C-reactive protein and coronary heart disease in the MRFIT nested case-control study. Multiple Risk Factor Intervention Trial. Am J Epidemiol 1996;144:537-47. [PubMed]
- Ridker PM, Hennekens CH, Buring JE, et al. C-reactive protein and other markers of inflammation in the prediction of cardiovascular disease in women. N Engl J Med 2000;342:836-43. [PubMed]
- Berg AH, Scherer PE. Adipose tissue, inflammation, and cardiovascular disease. Circ Res 2005;96:939-49. [PubMed]
- Iacobellis G, Pistilli D, Gucciardo M, et al. Adiponectin expression in human epicardial adipose tissue in vivo is lower in patients with coronary artery disease. Cytokine 2005;29:251-5. [PubMed]
- Baker AR, Silva NF, Quinn DW, et al. Human epicardial adipose tissue expresses a pathogenic profile of adipocytokines in patients with cardiovascular disease. Cardiovasc Diabetol 2006;5:1. [PubMed]
- Otsuka F, Sugiyama S, Kojima S, et al. Plasma adiponectin levels are associated with coronary lesion complexity in men with coronary artery disease. J Am Coll Cardiol 2006;48:1155-62. [PubMed]
- Ishii T, Asuwa N, Masuda S, et al. Atherosclerosis suppression in the left anterior descending coronary artery by the presence of a myocardial bridge: an ultrastructural study. Mod Pathol 1991;4:424-31. [PubMed]
- Ishii T, Asuwa N, Masuda S, et al. The effects of a myocardial bridge on coronary atherosclerosis and ischaemia. J Pathol 1998;185:4-9. [PubMed]
- Robicsek F, Thubrikar MJ. The freedom from atherosclerosis of intramyocardial coronary arteries: reduction of mural stress--a key factor. Eur J Cardiothorac Surg 1994;8:228-35. [PubMed]
- Chandalia M, Garg A, Vuitch F, et al. Postmortem findings in congenital generalized lipodystrophy. J Clin Endocrinol Metab 1995;80:3077-81. [PubMed]
- Ding J, Hsu FC, Harris TB, et al. The association of pericardial fat with incident coronary heart disease: the Multi-Ethnic Study of Atherosclerosis (MESA). Am J Clin Nutr 2009;90:499-504. [PubMed]
- Alexopoulos N, McLean DS, Janik M, et al. Epicardial adipose tissue and coronary artery plaque characteristics. Atherosclerosis 2010;210:150-4. [PubMed]
- Cheng VY, Dey D, Tamarappoo B, et al. Pericardial fat burden on ECG-gated noncontrast CT in asymptomatic patients who subsequently experience adverse cardiovascular events. JACC Cardiovasc Imaging 2010;3:352-60. [PubMed]
- Harada K, Amano T, Uetani T, et al. Cardiac 64-multislice computed tomography reveals increased epicardial fat volume in patients with acute coronary syndrome. Am J Cardiol 2011;108:1119-23. [PubMed]
- Yerramasu A, Dey D, Venuraju S, et al. Increased volume of epicardial fat is an independent risk factor for accelerated progression of sub-clinical coronary atherosclerosis. Atherosclerosis 2012;220:223-30. [PubMed]
- Mahabadi AA, Berg MH, Lehmann N, et al. Association of epicardial fat with cardiovascular risk factors and incident myocardial infarction in the general population: the Heinz Nixdorf Recall Study. J Am Coll Cardiol 2013;61:1388-95. [PubMed]
- den Dekker MA, Takashima R, van den Heuvel ER, et al. Relationship between epicardial adipose tissue and subclinical coronary artery disease in patients with extra-cardiac arterial disease. Obesity (Silver Spring) 2014;22:72-8. [PubMed]
- Kim SH, Chung JH, Kwon BJ, et al. The associations of epicardial adipose tissue with coronary artery disease and coronary atherosclerosis. Int Heart J 2014;55:197-203. [PubMed]
- Budoff MJ, Achenbach S, Blumenthal RS, et al. Assessment of coronary artery disease by cardiac computed tomography: a scientific statement from the American Heart Association Committee on Cardiovascular Imaging and Intervention, Council on Cardiovascular Radiology and Intervention, and Committee on Cardiac Imaging, Council on Clinical Cardiology. Circulation 2006;114:1761-91. [PubMed]
- Gorter PM, van Lindert AS, de Vos AM, et al. Quantification of epicardial and peri-coronary fat using cardiac computed tomography; reproducibility and relation with obesity and metabolic syndrome in patients suspected of coronary artery disease. Atherosclerosis 2008;197:896-903. [PubMed]
- Dey D, Wong ND, Tamarappoo B, et al. Computer-aided non-contrast CT-based quantification of pericardial and thoracic fat and their associations with coronary calcium and Metabolic Syndrome. Atherosclerosis 2010;209:136-41. [PubMed]
- Gottlieb I, Miller JM, Arbab-Zadeh A, et al. The absence of coronary calcification does not exclude obstructive coronary artery disease or the need for revascularization in patients referred for conventional coronary angiography. J Am Coll Cardiol 2010;55:627-34. [PubMed]
- Morita H, Fujimoto S, Kondo T, et al. Prevalence of computed tomographic angiography-verified high-risk plaques and significant luminal stenosis in patients with zero coronary calcium score. Int J Cardiol 2012;158:272-8. [PubMed]
- Blankstein R, Ferencik M. The vulnerable plaque: Can it be detected with Cardiac CT? Atherosclerosis 2010;211:386-9. [PubMed]
- Kim TH, Yu SH, Choi SH, et al. Pericardial fat amount is an independent risk factor of coronary artery stenosis assessed by multidetector-row computed tomography: the Korean Atherosclerosis Study 2. Obesity (Silver Spring) 2011;19:1028-34. [PubMed]
- Falk E. Plaque rupture with severe pre-existing stenosis precipitating coronary thrombosis. Characteristics of coronary atherosclerotic plaques underlying fatal occlusive thrombi. Br Heart J 1983;50:127-34. [PubMed]
- Okura K, Maeno K, Okura S, et al. Pericardial fat volume is an independent risk factor for the severity of coronary artery disease in patients with preserved ejection fraction. J Cardiol 2014. [Epub ahead of print]. [PubMed]
- Ambrose JA, Tannenbaum MA, Alexopoulos D, et al. Angiographic progression of coronary artery disease and the development of myocardial infarction. J Am Coll Cardiol 1988;12:56-62. [PubMed]
- Motoyama S, Kondo T, Sarai M, et al. Multislice computed tomographic characteristics of coronary lesions in acute coronary syndromes. J Am Coll Cardiol 2007;50:319-26. [PubMed]
- Motoyama S, Sarai M, Harigaya H, et al. Computed tomographic angiography characteristics of atherosclerotic plaques subsequently resulting in acute coronary syndrome. J Am Coll Cardiol 2009;54:49-57. [PubMed]
- Nemirovsky D. Imaging of high-risk plaque. Cardiology 2003;100:160-75. [PubMed]
- Tamarappoo B, Dey D, Shmilovich H, et al. Increased pericardial fat volume measured from noncontrast CT predicts myocardial ischemia by SPECT. JACC Cardiovasc Imaging 2010;3:1104-12. [PubMed]
- Nakazato R, Dey D, Cheng VY, et al. Epicardial fat volume and concurrent presence of both myocardial ischemia and obstructive coronary artery disease. Atherosclerosis 2012;221:422-6. [PubMed]
- Forouzandeh F, Chang SM, Muhyieddeen K, et al. Does quantifying epicardial and intrathoracic fat with noncontrast computed tomography improve risk stratification beyond calcium scoring alone? Circ Cardiovasc Imaging 2013;6:58-66. [PubMed]
- Nakanishi R, Rajani R, Cheng VY, et al. Increase in epicardial fat volume is associated with greater coronary artery calcification progression in subjects at intermediate risk by coronary calcium score: a serial study using non-contrast cardiac CT. Atherosclerosis 2011;218:363-8. [PubMed]