Quantification of myocardial deformation in patients with Fabry disease by cardiovascular magnetic resonance feature tracking imaging
Introduction
Fabry disease (FD) is a rare X-linked lysosomal storage disorder caused by mutations in the gene encoding for α-galactosidase A (1). FD affects multiple organs including the heart. Cardiac involvement is a major contributor of morbidity and mortality in FD (2,3). Detection of early involvement, assessment of cardiac response to treatment and evaluation of disease severity are important in FD management (4,5). Cardiovascular magnetic resonance (CMR) is a non-invasive and multi-parametric imaging modality that has the potential to detect early cardiac involvement in FD and has quickly become the reference-standard imaging method. CMR allows for a detailed assessment of regional myocardial wall thickness, myocardial mass, myocardial fat (using native T1 mapping technique to reflect sphingolipid storage), fibrosis [using the late gadolinium enhancement (LGE) technique]. In the early stage of FD, even in the absence of left ventricular hypertrophy (LVH), low native T1 values were found to sensitively indicate sphingolipid accumulation (6). When patients progress to myocardial fibrosis or triggered LVH in men, native T1 values increase and may result in “pseudonormalization” (7,8). CMR derived feature fracking (FT) is a validated quantitative method of assessing myocardial deformation which may reflect early changes of myocardial structure and function in many diseases (9). Therefore, we sought to explore the utility of CMR-FT as a measure of myocardial dysfunction in FD patients with or without LVH and/or fibrosis. We present the following article in accordance with the Materials Design Analysis Reporting (MDAR) checklist. Available at: http://dx.doi.org/10.21037/cdt-20-897.
Methods
The study was conducted in accordance with the Declaration of Helsinki (as revised in 2013). The study was approved by Beijing Anzhen Hospital ethics committee (NO.: 2019065X) and informed consent was taken from all the patients.
Study populations
Twenty patients with genetically confirmed FD were prospectively enrolled. None of the patients with FD had received enzyme replacement therapy prior to the study entry. Exclusion criteria included prior myocardial infarction or known coronary artery disease and contraindications to CMR. Twenty healthy volunteers without history of cardiovascular disease were also enrolled as a control group.
CMR image acquisition
CMR was performed using a 3T MR system (MAGNETOM Verio, Siemens Healthcare, Erlangen, Germany) with a 32-channel cardiac coil. Steady-state free-precession cine images were obtained during repeated breath-holds in three long axes (2-chamber, 3-chamber, and 4-chamber view) and in a stack of short axes covering the LV. Imaging parameters were: repetition time (TR) 3.1 ms, echo time (TE) 1.3 ms, asymmetric echo with factor 0.29, flip angle (FA) 45°, field of view (FOV) 276×340 mm2, matrix 156×192, slice thickness 6 mm, receiver bandwidth (BW) 704 Hz/px, parallel imaging using GRAPPA reconstruction (R=2), 30 cardiac phases. LV native T1 maps and 20 min post-contrast T1 maps were acquired using modified Look-Locker inversion recovery sequence [MOLLI, sampling pattern: 5(3)3] at basal, mid-ventricular and apical short axis slices. Imaging parameters were: TR =2.6–2.7 ms, TE =1.0–1.1 ms, FA =35°, FOV =270×360 mm2, matrix =256×256, slice thickness =6 mm, BW=1,045–1,028 Hz/px, GRAPPA acceleration factor 2, linear phase-encoding ordering. Quality control was performed during scanning by reviewing the “goodness of fit” map and source images to allow an immediate repetition of suboptimal measurements to minimize the respiratory motion and off-resonance effects. Extracellular volume fraction (ECV) was derived from the pre-/post-contrast T1 maps and hematocrit. The blood sample was taken just before the CMR exam to measure hematocrit for ECV calculation. A commercially available gadolinium-based contrast agent (gadopentetate dimeglumine, Magnevist, Bayer Healthcare) was administered intravenously at a dose of 0.1 mmol/kg body weight and contrast-enhanced images were acquired after a 10-min delay with the use of an inversion-recovery segmented gradient echo sequence. Contrast-enhanced images were acquired in identical long- and short-axis planes to the cine images except for the most apical short-axis slice, which was excluded because it can be affected by partial-volume effects. The inversion time was meticulously adjusted to obtain maximal nulling of remote normal LV myocardium with voxel sizes of 1.9 mm × 1.4 mm × 7.0 mm.
CMR analysis
The LV functional indices and maximum wall thickness (MWT) of all subjects were analyzed using dedicated software (Argus, Siemens Healthcare, Erlangen, Germany). The following indices were measured: LV ejection fraction (LVEF), LV end-diastolic volume index (LVEDVi), LV end-systolic volume index (LVESVi), LV cardiac output (LVCO) and LV mass index (LVMi). LV wall thickness was measured at end-diastolic phase on the short-axis images with American Heart Association (AHA) 16-segmental mode, a MWT >12 mm or LVMi >85 g/m2 in males and >81 g/m2 in females were considered as being LVH positive (10,11). All LGE images were visually assessed for the presence of LGE. Quantitative LGE analysis was performed using a signal intensity threshold of 4 standard deviations (SD) above visually normal remote myocardium, expressed as absolute myocardial mass gram (12). Analysis of 3-dimensional (3D) LV strain and strain rate were obtained using commercially available software as previously described (13) (CVi42 version 5.9.1, Circle, Canada). Epicardial and endocardial borders of LV were manually drawn on the end-diastolic frame of short axis and long axis images respectively. Then the contours were automatically propagated in all frames throughout the entire cardiac cycle. CMR derived FT was visually reviewed to ensure accurate tracking. In cases of inadequate tracking, the myocardial borders were manually adjusted. LV global peak systolic longitudinal (GLS), circumferential (GCS) strain, LV global peak systolic longitudinal (GLSR), circumferential (GCSR) strain rates, LV global peak early diastolic longitudinal (GDLSR) and circumferential (GDCSR) strain rates were all derived. LV native T1 values and post-contrast T1 values were measured with AHA 16-segmental mode, global native T1 values and post-contrast T1 values were obtained by averaging segmental values respectively. Accordingly, segmental and global ECV were obtained (8).
The intra- and inter-observer variability for the LV strain indices were assessed by the intraclass correlation coefficient (ICC) in all patients. Intra-observer reproducibility was established by the same observer who re-analyzed all patients’ images after one month. Inter-observer reproducibility was assessed by a second-independent observer blinded to the first observer’s results.
Subgroup analysis
Patients were divided into three groups based on their clinical assessment of heart failure, blood biomarkers (troponin, brain natriuretic peptide), cardiac functional indices (by echocardiography or CMR), electrocardiography and CMR findings (14-16): group 1: patients without LVH, LGE negative, and without heart failure; group 2: patients with LVH positive, LGE either positive or negative, and without heart failure; group 3: patients with LGE positive, LV wall thinning compared to normal controls, presence of conduction abnormalities and heart failure (Figure 1).
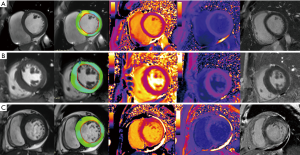
Statistical analysis
Statistical analyses were carried out using SPSS (version 22, IBM). Continuous variables are expressed as mean ± SD and categorical as frequencies or percentages. Normality was checked using the Shapiro-Wilk test. Differences between FD patients and healthy controls were compared using the paired t-test or U test or χ2 testing as indicated. Differences across three FD groups were compared with using ANOVA or Kruskal-Wallis for parametric and nonparametric variables, respectively, and χ2 testing for categorical variables. Person’s or Spearman’s correlation was performed to investigate the potential relationship between strain indices and other CMR indices (including LV functional indices, MWT, native T1, ECV) in all patients. The correlation was considered weak if correlation coefficient was <0.5, moderate if correlation coefficient was between 0.5–0.7, and strong if correlation coefficient was >0.7. A P<0.05 was considered significant.
Results
Baseline clinical characteristics of FD patients
Baseline demographic data and CMR findings of all subjects are summarized in Table 1. There were no statistical differences of age, sex, height and weight between FD patients and healthy controls (all P>0.05). Age and sex between FD patients and healthy controls also showed no statistical differences in every subgroup (Table 2). Among FD patients, 7 (35%) patients had a history of hypertension and 1 (5%) patient had a history of diabetes mellitus, 14 (70%) had LVH (mean MWT of these 14 patients was 15.2±2.0 mm) and 4 (20%) had LGE. Phenotypes and the enzymatic activity of FD patients are summarized in Table 3.
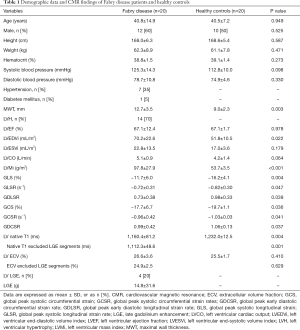
Full table
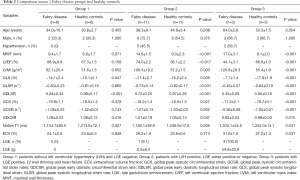
Full table
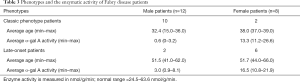
Full table
LVH and LV global function findings of FD patients according to group
There were statistical differences of age among FD patients (P=0.006), group 3 of FD patients had significant older mean age than other FD groups (all P<0.05). The MWT, LVEF and LVMi were significantly different among three FD groups (all P<0.01) and between each two groups (all P<0.01) except MWT between group 2 and group 3 (P=0.071), LVEF between group 1 and group 2 (P=0.274). The MWT, LVEF and LVMi were significantly different between FD patients and sex and age matched healthy controls in group 2 and group 3 respectively (all P<0.01, Table 2).
Myocardial deformation imaging findings of FD patients according to group
Compared with healthy controls, all the strain indices of FD with LVH (group 2 and group 3) were reduced (all P<0.05) except GCSR in group 2 (P=0.932), but in FD without LVH (group 1), only GLS and GDLSR were significantly lower than that of controls (all P<0.05, Table 2). Among FD groups, the strain indices impairment degree from mild to severe were group 1 < group 2 < group 3. When compared between different FD groups, there were significant differences of GLS and GCS among three groups and between each two groups (Figure 2). There were weak correlations between the LV functional parameters (LVEF, LVMi), MWT, T1 mapping indices (native T1, ECV) and LV strain indices (GLS, GLSR, GDLSR, GCS, GCSR, GDCSR) (Table 4). The strongest relation was between GDLSR and native T1 value (r=0.783, P<0.01).
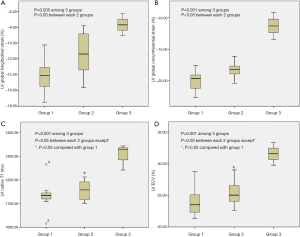
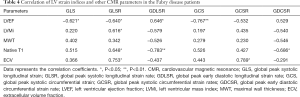
Full table
The strain indices were reproducible on an intra- and inter-observer level. For intra-observer reproducibility, ICC of GCS was 0.854, GCSR was 0.806, GDCSR was 0.807, ICC of GLS was 0.799, GLSR was 0.756, GDLSR was 0.749. For inter-observer reproducibility, ICC of GCS was 0.818, GCSR was 0.781, GDCSR was 0.763, ICC of GLS was 0.725, GLSR was 0.698, GDLSR was 0.598.
Tissue characterization findings of FD patients according to group
Overall mean LV native T1 value of FD (1,160.4±81.2 ms) was lower than that of controls (1,232.0±12.5 ms, P<0.01), after excluded LGE positive segments, mean LV native T1 value of 1,112.3±48.6 ms was further lower compared to healthy controls (Table 1). There were statistical differences of LV native T1 values between FD patients and age and sex matched healthy controls (Table 2). When compared between different FD groups, FD without LVH (group 1) had lower native T1 values and ECV than FD with LVH (group 2 and group 3), but the differences were not significant when compared to group 2 (all P>0.05) (Figure 2).
Discussion
In this study we examined the value of CMR-FT in the assessment of myocardial deformation in patients with FD. The principal findings of our study are: (I) impaired LV strain indices occur in FD patients at all stages of the disease. The impairment severity in myocardial deformation parallels along with disease severity. (II) In the earlier stage of the disease (without LVH), both LV systolic (global peak systolic longitudinal strain) and diastolic (early diastolic longitudinal strain rate) myocardial contractility were impaired compared with the normal controls. LV longitudinal strain indices are more sensitive than circumferential strain indices in detection of the early contractile abnormality in FD. (III) Also, in the earlier stages of the disease, although LV native T1 values overlap between patients with LVH and without LVH, LV strain indices in FD patients with LVH were significantly more impaired when compared to FD patients without LVH. Therefore, the distinctive T1 reduction makes native T1 mapping a valuable screening tool for FD, however, CMR-FT strain appears to be appropriate for tracking disease severity in FD.
CMR myocardial feature tracking is a new technique that allows for precise measurement of both global and regional myocardial strain. CMR-FT has been validated against traditional tagging methods of measuring strain and has also been able to distinguish between myocardial deformations in cardiac diseases including hypertrophic cardiomyopathy (HCM), cardiac amyloidosis and myocarditis (17-20). Abnormal strain values have previously been reported in FD patients with LVH in few studies using both echocardiographic strain and CMR feature tracking (10,12,21,22). The results in our study extends these prior findings and suggest that the degree of strain abnormalities varied significantly in all three FD groups and correlated with disease severity. Myocardial inflammation, hypertrophy and fibrosis follow sphingolipid deposition in FD. Myocardial inflammation, hypertrophy and fibrosis all have an adverse impact on myocardial contractility and lead to reduced myocardial strain indices in many cardiovascular diseases (23-25). In our study, group 1 only had mild LV native T1 reduction and mild myocardial strain indices impairment; group 2, in addition to LV native T1 reduction, this group also had LVH and myocardial fibrosis (assessed by LGE), correspondingly worse myocardial strain indices; and as a late stage, group 3 had LVH, heavy myocardial fibrosis burden, heart failure, and the worst myocardial strain indices. Therefore, CMR-FT may hold promise in the monitoring of FD cardiomyopathy progression in patients with FD.
Early detection of cardiac involvement even in late-onset FD allows for appropriate treatment decisions (26). Although myocardial strain abnormalities have previously been demonstrated in FD patients with LVH and without LVH, there has been contradictory findings in prior studies. Mathur et al. found that FD patients without LVH compared with controls did not differ significantly in GLS or GCS (12). Another group assessed GLS by CMR and echocardiography in serial studies and showed that FD patients without LVH had impaired GLS (10,27,28). In this present study, we found systolic and diastolic longitudinal strain indices, global peak systolic longitudinal strain and early diastolic longitudinal strain rate respectively were lower than normal in FD patients without LVH. However, circumferential strain indices were not different compared with healthy controls. These results suggested that the myocardial mechanical impairment assessed by GLS is sensitive in detecting the early contractile abnormality. Knott et al. assessed myocardial blood flow in FD patients and found impaired myocardial perfusion even before the onset of LVH. Their results also demonstrated that the FD perfusion impairment was more pronounced in the subendocardium (8). The subendocardium of LV is longitudinal oriented fibres and the abnormality of perfusion in the subendocardium may partially explain the GLS reduction in the early stage of the disease.
Low native T1 can detect cardiac involvement in FD as it represents sphingolipid accumulation (27,29). Our results showed that average LV native T1 values even in patients without LVH (early stage) were lower than normal controls, which is in line with previous studies (7,8,10). Notably, LV native T1 values in the early stage without LVH was even lower than that in patients in the intermediate stage with LVH. Furthermore, in the late stage, average native T1 was significantly higher than normal values. Reduction of native T1 values did not track disease severity. Native T1 reflects the overall myocardial changes including the myocyte and interstitium changes. Multiple pathology of FD, such as edema, fibrosis, and LVH lead to native T1 pseudonormalization by increasing the T1 values (7). Therefore, native T1 is sensitive for screening cardiac involvement (14), but when using native T1 for follow-up treatment, the changes of T1 (either progression or regression) may need cautious interpretation due to the impact of above factors. Previous studies have shown that the myocardial ECV in FD is similar to healthy controls which our results agree with for the early and intermediate stages. Furthermore, like other cardiomyopathies in the late stage, LV ECV in FD was elevated significantly in the final stage. In FD, ECV provided similar clinical significance like most cardiovascular diseases.
Limitations
There are several limitations to our study. FD is a rare disease, thus limiting the number of patients included in the study. We did not undertake myocardial biopsies in the patients to determine the lysosomal storage and myocardial fibrosis. Given the small number of patients, we were not able to compare the CMR indices between male and female in different stages. The values of myocardial strain assessed by 3D FT-CMR were lower than 2D FT-CMR (30). We used a 3D strain method, so the strain values of this study have to be interpreted within the same assessment method. Hypertension is one of the main adverse events occurring in the late stage of FD (31). We have seven patients with hypertension (five patients in group 2, two patients in group 3), LV hypertrophy, myocardial deformation and fibrosis in these patients maybe partially explained by hypertension. Future investigations are needed to investigate the effects of hypertension on the LV morphology, function and tissue characterization in FD.
Conclusions
Myocardial strain impairment is found in FD in all stages after diagnosis, even in the early stage, without LVH. CMR derived strain imaging may aid in the early diagnosis and appropriate management of patients with cardiac FD.
Acknowledgments
Funding: This work was supported by the National Natural Science Foundation of China (grant number 81671647, 81771787).
Footnote
Reporting Checklist: The authors have completed the MDAR reporting checklist. Available at http://dx.doi.org/10.21037/cdt-20-897
Data Sharing Statement: Available at http://dx.doi.org/10.21037/cdt-20-897
Peer Review File: Available at http://dx.doi.org/10.21037/cdt-20-897
Conflicts of Interest: All authors have completed the ICMJE uniform disclosure form (available at http://dx.doi.org/10.21037/cdt-20-897). The authors have no conflicts of interest to declare.
Ethical Statement: The authors are accountable for all aspects of the work in ensuring that questions related to the accuracy or integrity of any part of the work are appropriately investigated and resolved. The study was conducted in accordance with the Declaration of Helsinki (as revised in 2013). The study was approved by Beijing Anzhen Hospital ethics committee (NO.: 2019065X) and informed consent was taken from all the patients.
Open Access Statement: This is an Open Access article distributed in accordance with the Creative Commons Attribution-NonCommercial-NoDerivs 4.0 International License (CC BY-NC-ND 4.0), which permits the non-commercial replication and distribution of the article with the strict proviso that no changes or edits are made and the original work is properly cited (including links to both the formal publication through the relevant DOI and the license). See: https://creativecommons.org/licenses/by-nc-nd/4.0/.
References
- Zarate YA, Hopkin RJ. Fabry’s disease. Lancet 2008;372:1427-35. [Crossref] [PubMed]
- Waldek S, Patel MR, Banikazemi M, et al. Life expectancy and cause of death in males and females with Fabry disease: findings from the Fabry Registry. Genet Med 2009;11:790-6. [Crossref] [PubMed]
- Patel MR, Cecchi F, Cizmarik M, et al. Cardiovascular events in patients with fabry disease natural history data from the fabry registry. J Am Coll Cardiol 2011;57:1093-9. [Crossref] [PubMed]
- Arends M, Biegstraaten M, Hughes DA, et al. Retrospective study of long-term outcomes of enzyme replacement therapy in Fabry disease: analysis of prognostic factors. PLoS One 2017;12:e0182379. [Crossref] [PubMed]
- Hanneman K, Karur GR, Wasim S, et al. Prognostic significance of cardiac magnetic resonance imaging late gadolinium enhancement in Fabry disease. Circulation 2018;138:2579-81. [Crossref] [PubMed]
- Nordin S, Kozor R, Baig S, et al. Cardiac phenotype of prehypertrophic fabry disease. Circ Cardiovasc Imaging 2018;11:e007168. [Crossref] [PubMed]
- Nordin S, Kozor R, Medina-Menacho K, et al. Proposed Stages of Myocardial Phenotype Development in Fabry Disease. JACC Cardiovasc Imaging 2019;12:1673-83. [Crossref] [PubMed]
- Knott KD, Augusto JB, Nordin S, et al. Quantitative Myocardial Perfusion in Fabry Disease. Circ Cardiovasc Imaging 2019;12:e008872. [Crossref] [PubMed]
- Scatteia A, Baritussio A, Bucciarelli-Ducci C. Strain imaging using cardiac magnetic resonance. Heart Fail Rev 2017;22:465-76. [Crossref] [PubMed]
- Vijapurapu R, Nordin S, Baig S, et al. Global longitudinal strain, myocardial storage and hypertrophy in Fabry disease. Heart 2019;105:470-6. [Crossref] [PubMed]
- Kawel-Boehm N, Maceira A, Valsangiacomo Buechel ER, et al. Normal values for cardiovascular magnetic resonance in adults and children. J Cardiovasc Magn Reson 2015;17:29. [Crossref] [PubMed]
- Mathur S, Dreisbach JG, Karur GR, et al. Loss of base-to-apex circumferential strain gradient assessed by cardiovascular magnetic resonance in Fabry disease: relationship to T1 mapping, late gadolinium enhancement and hypertrophy. J Cardiovasc Magn Reson 2019;21:45. [Crossref] [PubMed]
- Nucifora G, Muser D, Tioni C, et al. Prognostic value of myocardial deformation imaging by cardiac magnetic resonance feature-tracking in patients with a first ST-segment elevation myocardial infarction. Int J Cardiol 2018;271:387-91. [Crossref] [PubMed]
- Militaru S, Ginghina C, Popescu BA, et al. Multimodality imaging in Fabry cardiomyopathy: from early diagnosis to therapeutic targets. Eur Heart J Cardiovasc Imaging 2018;19:1313-22. [Crossref] [PubMed]
- Biegstraaten M, Arngrímsson R, Barbey F, et al. Recommendations for initiation and cessation of enzyme replacement therapy in patients with Fabry disease: the European Fabry Working Group consensus document. Orphanet J Rare Dis 2015;10:36. [Crossref] [PubMed]
- Takenaka T, Teraguchi H, Yoshida A, et al. Terminal stage cardiac findings in patients with cardiac Fabry disease: an electrocardiographic, echocardiographic, and autopsy study. J Cardiol 2008;51:50-9. [Crossref] [PubMed]
- Bogarapu S, Puchalski MD, Everitt MD, et al. Novel Cardiac Magnetic Resonance Feature Tracking (CMR-FT) Analysis for Detection of Myocardial Fibrosis in Pediatric Hypertrophic Cardiomyopathy. Pediatr Cardiol 2016;37:663-73. [Crossref] [PubMed]
- Hor KN, Gottliebson WM, Carson C, et al. Comparison of magnetic resonance feature tracking for strain calculation with harmonic phase imaging analysis. JACC Cardiovasc Imaging 2010;3:144-51. [Crossref] [PubMed]
- Baeßler B, Schaarschmidt F, Dick A, et al. Diagnostic implications of magnetic resonance feature tracking derived myocardial strain parameters in acute myocarditis. Eur J Radiol 2016;85:218-27. [Crossref] [PubMed]
- Bhatti S, Vallurupalli S, Ambach S, et al. Myocardial strain pattern in patients with cardiac amyloidosis secondary to multiple myeloma: a cardiac MRI feature tracking study. Int J Cardiovasc Imaging 2018;34:27-33. [Crossref] [PubMed]
- Gruner C, Verocai F, Carasso S, et al. Systolic myocardial mechanics in patients with Anderson-Fabry disease with and without left ventricular hypertrophy and in comparison to nonobstructive hypertrophic cardiomyopathy. Echocardiography 2012;29:810-7. [Crossref] [PubMed]
- Labombarda F, Saloux E, Milesi G, et al. Loss of base-to-apex circumferential strain gradient: A specific pattern of Fabry cardiomyopathy? Echocardiography 2017;34:504-10. [Crossref] [PubMed]
- Luetkens JA, Schlesinger-Irsch U, Kuetting DL, et al. Feature-tracking myocardial strain analysis in acute myocarditis: diagnostic value and association with myocardial oedema. Eur Radiol 2017;27:4661-71. [Crossref] [PubMed]
- Vigneault DM, Yang E, Jensen PJ, et al. Left Ventricular Strain Is Abnormal in Preclinical and Overt Hypertrophic Cardiomyopathy: Cardiac MR Feature Tracking. Radiology 2019;290:640-8. [Crossref] [PubMed]
- Tahir E, Starekova J, Muellerleile K, et al. Impact of Myocardial Fibrosis on Left Ventricular Function Evaluated by Feature-Tracking Myocardial Strain Cardiac Magnetic Resonance in Competitive Male Triathletes with Normal Ejection Fraction. Circ J 2019;83:1553-62. [Crossref] [PubMed]
- Perry R, Shah R, Saiedi M, et al. The Role of Cardiac Imaging in the Diagnosis and Management of Anderson-Fabry Disease. JACC Cardiovasc Imaging 2019;12:1230-42. Erratum in: JACC Cardiovasc Imaging. 2019 Sep;12(9):1903. doi: 10.1016/j.jcmg.2019.07.004. [Crossref] [PubMed]
- Sado DM, White SK, Piechnik SK, et al. Identification and assessment of Anderson-Fabry disease by cardiovascular magnetic resonance noncontrast myocardial T1 mapping. Circ Cardiovasc Imaging 2013;6:392-8. [Crossref] [PubMed]
- Pica S, Sado DM, Maestrini V, et al. Reproducibility of native myocardial T1 mapping in the assessment of Fabry disease and its role in early detection of cardiac involvement by cardiovascular magnetic resonance. J Cardiovasc Magn Reson 2014;16:99. [Crossref] [PubMed]
- Thompson RB, Chow K, Khan A, et al. T1 mapping with cardiovascular MRI is highly sensitive for Fabry disease independent of hypertrophy and sex. Circ Cardiovasc Imaging 2013;6:637-45. [Crossref] [PubMed]
- Liu B, Dardeer AM, Moody WE, et al. Reference ranges for three-dimensional feature tracking cardiac magnetic resonance: comparison with two-dimensional methodology and relevance of age and gender. Int J Cardiovasc Imaging 2018;34:761-75. [PubMed]
- Marazzi MG, Galliera E, Vianello E, et al. Hypertension in adult Fabry's disease: is cardiotrophin-1 a diagnostic biomarker? Immun Ageing 2014;11:27. [Crossref] [PubMed]