Hyperglycemia: a bad signature on the vascular system
Hyperglycemia and cardiovascular disease (CVD)
Type 2 diabetes (T2D) is characterized by long-standing hyperglycemia which results from defective insulin sensitivity and secretion (1). Environmental factors as well as genetic background significantly concur to determine the diabetic phenotype and its detrimental effect on the vascular system. Nowadays, 382 million people are affected by T2D, with a global age-adjusted prevalence of 10% (2). Alarmingly, a substantial proportion of affected people are unaware of their condition and do not receive treatment. The incidence of CVD is two- to eight-fold higher in subjects with T2D than in those without and accounts for the majority of deaths (3). Seminal epidemiological work indicates that hyperglycemia is still considered the major risk factor implicated in the development and progression of diabetic vascular complications. In people with established diabetes, pooled analyses from a number of prospective studies provide strong evidence that glycated haemoglobin (HbA1c) predicts CVD risk, with an increase of about 18% in risk for each 1% absolute increase in HbA1c concentration, regardless of classical risk factors (4). Interestingly, a very recent study conducted on individuals without known CVD or diabetes, additional assessment of HbA1c values provided incremental benefit for prediction of CVD risk (5). Although the importance of hyperglycemic burden on cardiovascular phenotype, normalization of blood glucose levels in patients with long-standing hyperglycemia does not seem to reduce macrovascular complications. By contrast, intensive glycemic control is associated with clear cardiovascular benefits in patients with newly-diagnosed diabetes (6).
Early intervention studies
The landmark diabetes complications and control trial (DCCT) (7) demonstrated that early intensive glycemic control (goal of HbA1c 6.5%, mean achieved HbA1c 7%) in subjects with type 1 diabetes (T1D) reduced the risk and progression of microvascular complications as compared to conventional therapy. A clinically relevant observation extrapolated from this study was that the relationship between glucose control (as reflected by the mean on-study HbA1c value) and risk of complications was almost linear and extended to the normal HbA1c range (<6%), with no threshold noted (6). The observational follow-up of DCCT, known as the Epidemiology of Diabetes Interventions and Complications (EDIC) study (8), was the first large-scale clinical trial to demonstrate the persistent benefit among patients who were initially randomized to intensive control compared to conventional treatment, despite converging HbA1c values between the two groups. Emerging data from the EDIC also suggest that the influence of early glycemic control on eventual progression of macrovascular complications may become more evident with longer follow-up. Indeed 9 years after DCCT termination, participants previously randomized to the intensive arm had a 42% reduction (P=0.02) in CVD outcomes and a 57% reduction (P=0.02) in the risk of nonfatal myocardial infarction (MI), stroke, or CVD death compared with those previously in the standard arm. Data from the United Kingdom prospective diabates study (UKPDS) appear to be consistent with this evidence. UKPDS, a randomized, prospective, multicenter trial, showed that intensive glucose therapy (median HbA1c 7.0%) in patients with newly diagnosed T2D was associated with a reduced risk of microvascular complications (9). Taken together, the studies examined so far suggest that intensive glycemic control reduces the risk of microvascular complications whereas its impact on macrovascular disease remains not clearly defined.
Late intervention studies
The Action to Control Cardiovascular Risk in Diabetes study group (ACCORD) (10), Action in Diabetes and Vascular Disease-Preterax and Diamicron modified release Controlled Evaluation (ADVANCE) (11), and the Veterans Affairs Diabetes Trial (VADT) (12), sought to determine the effect of glucose lowering to near-normal levels on CV risk. These studies included a large number of participants with complete follow-up for a median of 3.5-5.6 years. The baseline characteristics were typical for adults with T2D: mean age of 60-66 years and duration of diabetes mellitus (DM) ranging from 8 to 11 years. Approximately one-third of patients in each study had a history of CVD, so these trials assessed the effect of intensive glycemic control in patients with and in those without pre-existing atherosclerotic vascular disease. In ADVANCE (11), the microvascular, but not macrovascular complications were improved during 5 years follow-up. Indeed, intensive glycemic control significantly reduced the primary end point [HR 0.90 (95% CI, 0.82-0.98), P=0.01], but this result was mostly driven by a significant reduction in the microvascular outcome [occurrence of macroalbuminuria, 0.86 (0.77-0.97), P=0.01], with no effects on macrovascular endpoints [0.94 (0.84-1.06), P=0.32]. In line with these negative results, the ACCORD trial (10) was even prematurely stopped after 3.4 years due to increased mortality in the intensive treatment arm. Despite many authors have attributed these findings to the aggressive control of hyperglycemia, sub-analyses of the mortality findings in ACCORD (considering variables such as weight gain, use of drug combination, and hypoglycemia) were unable to explain the excess mortality observed in the intensive arm (10). Yet, glycemic control achieved in the VADT trial (12) had no significant effect on the rates of major CV events, death, or microvascular complications. During a median 5.6-year follow-up period, the cumulative incidence of the primary outcome was not significantly lower in the intensive arm [HR 0.88 (95% CI, 0.74-1.05), P=0.12]. There were more CVD deaths in the intensive group than in the standard arm (38 vs. 29), but such difference was not statistically significant.
Albeit in all these studies patients were aggressively treated for all other CV risk factors, DM subjects remained exposed to a substantial risk of CV events and mortality. Indeed, a tight control of glycemia together with a multifactorial approach was not able to prevent the development of coronary artery disease in these patients. Possible explanations for these negative results—as previously shown by the DCCT and UKPDS investigators—may be a too short follow-up to demonstrate an effect on macrovascular complications and/or long-standing duration of DM beyond the stage where tight glycemic control could exert any protective effect (6). Another possible explanation is that in T2D, where other CVD risk factors are highly prevalent, the additive benefits of intensive glycemic control might be difficult to demonstrate. It is likely that a real benefit of glucose lowering on CVD in T2D, even if it could be proven, is modest compared with and incremental to treatment of other CVD risk factors.
The metabolic memory
Collectively, clinical data suggest that hyperglycemia may exert long-lasting detrimental effects the cardiovascular system (13,14). This phenomenon is defined “metabolic” or “hyperglycemic memory” to indicate a long-term persistence of hyperglycemic stress even after blood glucose normalization (Figure 1) (15). Molecular studies have recently demonstrated that oxidative stress is strongly implicated in this phenomenon (1,16). Accumulation of oxygen-derived free radicals in mitochondria is one of the main pathophysiological mechanisms underpinning DM-related vascular and cardiac complications (17). The mitochondrial adaptor p66Shc is a key redox enzyme implicated in ROS generation, mitochondrial disruption, and cellular death (18). Genetic deletion of p66Shc protects against hyperglycemia-induced endothelial dysfunction and oxidative stress in mice (18). The potential impact of this gene is supported by the clinical observation that p66Shc expression is increased in peripheral blood mononuclear cells obtained from patients with T2D and correlates with oxidative stress levels (19). We have recently posited that p66Shc might contribute to vascular hyperglycemic memory, in a ROS-dependent manner. Our experimental work demonstrated that hyperglycemia-induced p66Shc upregulation is not reverted by intensive glycemic control in diabetic mice and contributes to persistent oxidative damage and vascular dysfunction (20). These detrimental effects were explained by an intricate vicious cycle involving p66Shc, ROS, epigenetic changes and activation of protein kinase C (20). Interestingly enough, in vivo gene silencing of p66Shc at the time of normoglycemia restoration suppressed persistent endothelial dysfunction, indicating that p66Shc is an important source of free radicals involved in the “metabolic memory” phenomenon (13). Mechanistically, we have demonstrated that epigenetic changes occurring on p66Shc promoter are critically involved in persistent protein expression and, hence, vascular damage (20). In conditions of hyperglycemia, epigenetic modifications are emerging as a key player altering the expression of oxidant and inflammatory genes (21). The major mechanisms of epigenetic regulation are represented by DNA methylation of cytosine-paired-with-guanine (CpG) dinucleotide sequences as well as methylation or acetylation of histone proteins (22). Mapping the human epigenome in diabetic subjects may provide important insights on gene-activating events which are not erased by intensive glycemic control. A better molecular definition of the epigenetic cues is invaluable for the development of future therapeutic approaches to restore vascular homeostasis and reduce CVD complications in people with diabetes (23).
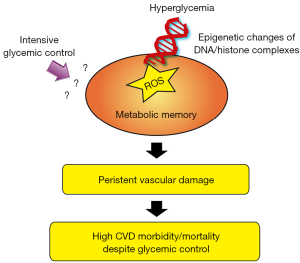
Conclusions
Despite clear advances in developing effective glucose-lowering drugs, clinical trials have recently shown that intensive glycemic control failed to reduce CVD burden in the diabetic population. These findings support the concept that the hyperglycemic environment may be remembered in the vasculature. This phenomenon has been recently defined “metabolic” or “hyperglycemic memory” and may contribute to explain the progression of diabetic vascular complications despite normoglycemia restoration. Recent experimental studies have unravelled putative molecular mechanisms involved in the persistence of CV damage despite glycemic control. Specifically, epigenetic changes of DNA/histone complexes are emerging as important modulators of oxidant and inflammatory genes, thus leading to persistent oxidative stress and vascular dysfunction. Future studies will be instrumental to understand the impact of epigenetic variations of vascular risk in patients with diabetes.
Acknowledgements
None.
Footnote
Conflicts of Interest: The authors have no conflicts of interest to declare.
References
- Paneni F, Beckman JA, Creager MA, et al. Diabetes and vascular disease: pathophysiology, clinical consequences, and medical therapy: part I. Eur Heart J 2013;34:2436-43. [PubMed]
- International Diabetes Federation. Diabetes Atlas, 6th eds. Brussels: International Diabetes Federation, 2013.
- Beckman JA, Paneni F, Cosentino F, et al. Diabetes and vascular disease: pathophysiology, clinical consequences, and medical therapy: part II. Eur Heart J 2013;34:2444-52. [PubMed]
- Selvin E, Steffes MW, Zhu H, et al. Glycated hemoglobin, diabetes, and cardiovascular risk in nondiabetic adults. N Engl J Med 2010;362:800-11. [PubMed]
- Emerging Risk Factors Collaboration, Di Angelantonio E, Gao P, et al. Glycated hemoglobin measurement and prediction of cardiovascular disease. JAMA 2014;311:1225-33. [PubMed]
- Skyler JS, Bergenstal R, Bonow RO, et al. Intensive glycemic control and the prevention of cardiovascular events: implications of the ACCORD, ADVANCE, and VA Diabetes Trials: a position statement of the American Diabetes Association and a Scientific Statement of the American College of Cardiology Foundation and the American Heart Association. J Am Coll Cardiol 2009;53:298-304. [PubMed]
- The effect of intensive treatment of diabetes on the development and progression of long-term complications in insulin-dependent diabetes mellitus. The Diabetes Control and Complications Trial Research Group. N Engl J Med 1993;329:977-86. [PubMed]
- Writing Team for the Diabetes Control and Complications Trial/Epidemiology of Diabetes Interventions and Complications Research Group. Sustained effect of intensive treatment of type 1 diabetes mellitus on development and progression of diabetic nephropathy: the Epidemiology of Diabetes Interventions and Complications (EDIC) study. JAMA 2003;290:2159-67. [PubMed]
- Intensive blood-glucose control with sulphonylureas or insulin compared with conventional treatment and risk of complications in patients with type 2 diabetes (UKPDS 33). UK Prospective Diabetes Study (UKPDS) Group. Lancet 1998;352:837-53. [PubMed]
- Action to Control Cardiovascular Risk in Diabetes Study Group, Gerstein HC, Miller ME, et al. Effects of intensive glucose lowering in type 2 diabetes. N Engl J Med 2008;358:2545-59. [PubMed]
- Advance Collaborative Group, Patel A, MacMahon S, et al. Intensive blood glucose control and vascular outcomes in patients with type 2 diabetes. N Engl J Med 2008;358:2560-72. [PubMed]
- Duckworth W, Abraira C, Moritz T, et al. Glucose control and vascular complications in veterans with type 2 diabetes. N Engl J Med 2009;360:129-39. [PubMed]
- Paneni F, Volpe M, Lüscher TF, et al. SIRT1, p66(Shc), and Set7/9 in vascular hyperglycemic memory: bringing all the strands together. Diabetes 2013;62:1800-7. [PubMed]
- Volpe M, Cosentino F, Tocci G, et al. Antihypertensive therapy in diabetes: the legacy effect and RAAS blockade. Curr Hypertens Rep 2011;13:318-24. [PubMed]
- Ceriello A. The emerging challenge in diabetes: the "metabolic memory". Vascul Pharmacol 2012;57:133-8. [PubMed]
- Ceriello A, Ihnat MA, Thorpe JE. Clinical review 2: The "metabolic memory": is more than just tight glucose control necessary to prevent diabetic complications? J Clin Endocrinol Metab 2009;94:410-5. [PubMed]
- Giacco F, Brownlee M. Oxidative stress and diabetic complications. Circ Res 2010;107:1058-70. [PubMed]
- Cosentino F, Francia P, Camici GG, et al. Final common molecular pathways of aging and cardiovascular disease: role of the p66Shc protein. Arterioscler Thromb Vasc Biol 2008;28:622-8. [PubMed]
- Pagnin E, Fadini G, de Toni R, et al. Diabetes induces p66shc gene expression in human peripheral blood mononuclear cells: relationship to oxidative stress. J Clin Endocrinol Metab 2005;90:1130-6. [PubMed]
- Paneni F, Mocharla P, Akhmedov A, et al. Gene silencing of the mitochondrial adaptor p66(Shc) suppresses vascular hyperglycemic memory in diabetes. Circ Res 2012;111:278-89. [PubMed]
- Cooper ME, El-Osta A. Epigenetics: mechanisms and implications for diabetic complications. Circ Res 2010;107:1403-13. [PubMed]
- Costantino S, Paneni F, Cosentino F. Targeting chromatin remodeling to prevent cardiovascular disease in diabetes. Curr Pharm Biotechnol 2015;16:531-43. [PubMed]
- Paneni F, Costantino S, Cosentino F. Molecular mechanisms of vascular dysfunction and cardiovascular biomarkers in type 2 diabetes. Cardiovasc Diagn Ther 2014;4:324-32. [PubMed]