Vascular repair strategies in type 2 diabetes: novel insights
Introduction
The maintenance of blood vessel function throughout life requires the continuous replacement of lost or dysfunctional cells as well as the adaptation to changing physiologic or pathologic stimuli, including changes in local oxygen demand, varying amounts of pro- or anti-inflammatory agents, or injury. Over decades, those mechanisms fulfill their function unnoticed. Repetitive challenge of vascular regenerative mechanisms by lifestyle-mediated cues, including the intake of noxious substances and lipids, as well as insufficient physical activity, evokes a gradual exhaustion and dysregulation of reparative pathways over the years (Figure 1). The impairment of cellular function—affecting resident vascular cells as well as “accessory” cells—is associated with the development of microvascular and macrovascular dysfunction. The resulting clinical manifestations include microvascular rarefaction in the limbs, myocardium, kidneys and bone marrow, causing the loss of the affected limb and/or dysfunction of the organ, impaired wound healing and altered hematopoiesis. Macrovascular dysfunction increases the risk for myocardial, cerebral or peripheral occlusive events through atherosclerotic vascular remodeling, endothelial erosion and, finally thrombotic events.
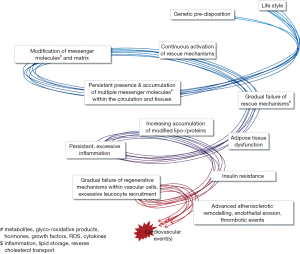
Circulating inflammatory mediators and cells play a crucial role in the regenerative processes guiding the dynamic adaptation to changing conditions (oxygen demand, injury). Recent years have seen a more varied perception of the role of inflammation in both, vascular regeneration and pathological remodeling. While inflammatory mechanisms are crucial in the mounting phase of angiogenesis and damage repair, its transformation into a chronic process underlies various pathologies. Likewise, inflammatory cell infiltration of adipose tissue critically determines the turning point at which adipose tissue becomes dysfunctional and in turn conveys systemic insulin insensitivity, a hallmark of type 2 diabetes (T2D) and a limitation of vascular regenerative capacity. In the recent years, our conceptions about endogenous repair mechanisms have widened to include a better appreciation of the high degree of integration between various tissues and organs, including adipose tissue, the hematopoietic system, skeletal muscles and the liver, all interconnected by the vascular system (Figure 2). Already the clinical routine is successfully using statins, angiotensin-receptor blockade or angiotensin-converting enzyme inhibition, which target multiple molecular pathways and cell types to support vascular repair mechanisms in patients with T2D (1). In this review, we will discuss further novel treatment strategies at various stages of clinical translation.
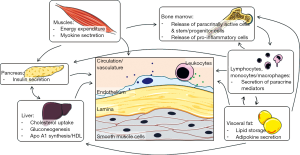
Clinical need
More than 50% of all diabetic patients die from cardiovascular-related causes and suffer from a diffuse and more aggressive course of atherosclerosis resulting in poorer outcomes (2,3). Despite advances in multifactorial management, the rate of coronary events remains disproportionally high in diabetic patients. Finding the appropriate revascularization strategy for these patients is therefore of outstanding importance (4). However, due to the impaired response to vascular injury, which is a major factor in the pathophysiology of diabetic vascular complications, percutaneous coronary intervention (PCI) poses a higher risk for diabetic than for non-diabetic patients. A recent network meta-analysis including trials comparing combinations of bare metal stenting (BMS), drug eluting stent (DES) placement and coronary artery bypass grafting (CABG) revealed an increased need for repeated revascularization as well as a higher mortality of diabetic patients after PCI (5). These observations confirm the findings of the BARI 2D and FREEDOM trial which both favor CABG over PCI as revascularization strategy in patients with T2D and coronary artery disease (6,7). Another meta-analysis demonstrated that diabetes is still one of the most important risk factors for restenosis after PCI (8). Recently, two research groups addressed the need for a comparison of PCI with second generation DES and CABG, the lack of which was a major limitation of previous studies (9,10). Park et al. performed a randomized noninferiority trial randomly assigning 1,776 patients with multivessel CAD to PCI with everolimus-eluting stents or to CABG. There was no statistically significant difference in the occurrence of the primary endpoint consisting of a composite of death, myocardial infarction or target-vessel revascularization at 2 years after randomization. However, at longer-term follow-up, the primary endpoint had been observed in 15.3% of the patients in the PCI group and in 10.6% of those in the CABG group. The incidence of stroke did not differ between both groups. Furthermore, the incidence of any repeat revascularization and spontaneous myocardial infarction were significantly higher in patients undergoing PCI than in those undergoing CABG (9). Bangalore et al. conducted an observational registry study comparing again the outcomes of patients undergoing PCI with the use of everolimus-eluting stents with the outcomes of patients undergoing CABG. They observed a similar risk of death associated with the respective procedure but a higher risk of repeat revascularization and a lower risk of stroke for PCI (10). Nevertheless, researchers and clinicians agree that the decision between PCI and CABG for diabetic patients remains a controversial one. CABG tends to yield better outcomes in terms of myocardial infarction and repeat revascularization but could also be associated with a higher risk for stroke. These results should be taken into account when entering the clinical process of shared decision making to enable the patient to make the best choice for both, disease and personal preferences. In addition, extensive efforts should be undertaken to address cardiovascular risk factors in diabetic patients and thus render primary and secondary prevention of cardiovascular events more effective (11).
Adipokine-related tissue inflammation and insulin resistance
Obesity—the enlargement of fat mass by increasing numbers and/or size of mature adipocytes—is traditionally considered a crucial component of metabolic syndrome, responsible for the development of insulin resistance and for the increase of cardiovascular risk. Newer experimental findings as well as observational studies paint a more differentiated picture in which a certain degree of adiposity is not immediately tied to adipose tissue inflammation and insulin desensitization (12). Instead, pro-inflammatory and insulin-desensitizing mechanisms are only triggered once adipocyte hypertrophy reaches a certain point at which their capacity to store free fatty acids (FFA) is exceeded (13-15). These “overfed” adipocytes alter their secretome, resulting in the enhanced recruitment of leukocytes, as well as a shift of macrophage functional subtypes present within the adipose tissue (16-18). Hypertrophic adipocytes will release, rather than store FFA, which then links to insulin resistance, as well as TLR4 activation and other pro-inflammatory mechanisms (19-25). Vice versa, insulin itself regulates fat uptake and inflammatory cytokines secreted by the activated macrophages perpetuate adipose tissue inflammation and dysfunction, and further insulin desensitization (26,27).
Several studies have therefore aimed to identify molecular players that delay the “trigger point” at which hypertrophic adipocytes become dysfunctional and upregulate pro-inflammatory mechanisms. Promising molecular strategies have targeted the transcription factor Rev-ErbA and phosphoenolpyruvate carboxykinase (PEPCK), both increasing adiposity, but without a concomitant increase in white adipose tissue (WAT) inflammation or loss of insulin sensitivity (28,29). Instead, serum adiponectin levels were increased in Rev-ErbA knockout mice and leptin levels kept low in PEPCK overexpressing mice (28,29). The Triggering Receptor Expressed on Myeloid Cells 2 (TREM2), instead, accelerates adipocyte hypertrophy and inflammatory functional dysregulation, together with causing decreased plasma adiponectin levels and elevated leptin levels (30). Adiponectin and leptin belong to a group of adipose tissue-derived cytokines termed “adipokines”, which harbour widespread implications for systemic metabolism and vascular biology (31). Adiponectin modulates macrophage polarization and function towards a less inflammatory profile (28,32-35). Given the important surveillance function of the M2-type macrophages within the vasculature, the adipokines-macrophage-axis might crucially contribute to vascular healing as well as promote angiogenesis (36).
Another knob to adjust might be DNA transcriptional regulation; regulated amongst many others by a family of histone deacetylases (HDAC) termed sirtuins. Sirtuins are involved in aging as well as a series of cardiovascular relevant processes (37). Sirtuin 1 levels are reduced in leukocytes of patients with insulin resistance/metabolic syndrome and are negatively associated with carotid intima media thickness (38). High glucose, palmitate-induced insulin resistance and FFAs all downregulated SIRT1 in vitro (38). Sirtuin 1 itself might be regulated by the product of the Deleted in Breast Cancer 1 gene (DBC1), which is upregulated under high fat diet (39). Mice lacking DBC1 became more obese but showed lower blood FFA levels, less atherosclerosis and longer life expectancy under high fat diet (39). Ablation of another histone deacetylase, HDAC9, led to reduced weight gain and improved glucose tolerance, associated with a shift of white adipose (WAT) tissue towards beige adipose tissue, which usually has higher energy expenditure rates than WAT (40).
Several depots of adipose tissue throughout our body differ in their phenotypic and functional characteristics (41). Macrophage infiltration rates differ between visceral and subcutaneous adipose tissue, potentially contributing to the different secretory spectra of subcutaneous, visceral and perivascular WAT depots (31,42). Especially visceral adipose tissue can increase cardiovascular risk significantly, likely through its more inflammatory secretome, as well as its direct connection with the portal circulation. Anatomical localization also characterizes the vascular relevance of perivascular adipose tissue (PVAT) (43-45). Similar to visceral adipose tissue, PVAT mass increases with obesity and features macrophage infiltrates, as well as paracrine dysfunction (43). Thus, dysfunctional PVAT can severely affect the vascular response to acute injury (46). One relevant mechanism might be through PVAT-derived ROS (47). On the other hand, vascular wall-released superoxide and adiponectin released by “healthy” PVAT are able to balance each other in a way that increased oxidative stress from the vascular wall can induce the counterregulatory increase of adiponectin in the PVAT (48). Interestingly, this mechanism was still functional in diabetic specimens, supporting the conclusion that in diabetic patients the reduction of adiponectin levels rather than compromised downstream signaling has a role in enhanced NAPDH oxidase-derived superoxide (48).
In contrast to WAT, brown adipose tissue (BAT)—featuring a higher mitochondrial density—is characterized by a high-energy expenditure. BAT is moreover a source of growth factors and chemokines regulating vascular layout and function, such as VEGF (49,50). Concomitantly, vascular density is higher in BAT than in WAT and reduced BAT VEGF-A levels in obesity were associated to vascular rarefaction and “whitening” of BAT with mitochondrial dysfunction and lipid droplet accumulation (49). Of interest, the diet-induced decline of VEGF-A and its receptor, KDR, preceded the development of adiposity and mitochondrial dysfunction, pointing to a causal role of VEGF-A shortage for the observed BAT whitening, a hypothesis backed by the fact that re-introduction of VEGF-A was able to rescue glucose uptake and insulin sensitivity (49). The findings are also supported by another study describing a crucial role of VEGF-A in BAT maintenance (51).
Thus, targeting of the individual adipose tissue depots might help support their intrinsic protective mechanisms while limiting the establishment of an inflammatory vicious cycle in adipose patients. As an example, VEGF-A manipulation or dietary intervention did affect brown, but not WAT vascularization, thus indicating a tissue-specific effect (49).
Lifestyle-based therapies
Despite a role of genetic predisposition, a larger impact on individual cardiovascular risk is accredited to lifestyle factors, such as sedentary behavior and excess caloric intake. A wealth of studies has linked sedentary time to metabolic disorders and more frequent occurrence of cardiovascular events, while physical activity is recommended for primary as well as secondary cardiovascular prevention (1,52-57). In fact, lifestyle modifications, consisting of diet changes combined with physical exercise, may be even more effective for the prevention of T2D than medical therapy such as metformin (58-60).
Both, dietary interventions and exercise training programs act at several leverages in addition to limiting caloric excess. Myokines are secreted by the active skeletal muscle and exert anti-inflammatory and pro-survival/pro-angiogenic effects in a systemic manner (61,62). Moreover, the increased blood flow during physical activity can directly affect endothelial cell signaling, supporting anti-oxidative and pro-survival signaling (63,64). Beyond the control of lipid, carbohydrate and protein intake, dietary interventions target the intake of a plethora of substances including minerals, anti-oxidants, unsaturated fatty acids, as well as hormonally active substances (65-69). Therefore, optimal parameters of both, dietary as well as physical activity interventions are still under intense investigation, including the choice of relevant endpoints as well as applicability for a wide part of the general population, considering motivational aspects and cost-effectiveness.
Several parameters influence the effects of exercise training, including the type (resistance or endurance), intensity, duration and frequency of exercise. Current guidelines recommend a weekly exercise duration of at least 150 min of moderate-intensity aerobic exercise or at least 90 min of vigorous aerobic exercise per week for the prevention/delay of diabetes onset (1). Both, endurance and resistance exercise can improve glucose disposal and hence lower HbA1c levels, albeit by differential mechanisms (54,70-72). Commonly, endurance training is favored for cardiovascular benefits due to more solid data (73). However, the addition of two to three sessions of resistance training per week is specifically recommended for T2D patients (74,75).
Despite all this evidence, adherence to an active life with a more healthy diet is a main obstacle with the majority of patients at risk, as well as the general population in the Western world. A recent widely discussed study failed to achieve a reduction of cardiovascular events in overweight or obese adults withT2D after a 10-year intense lifestyle intervention, despite improvements in body weight, physical fitness and metabolic markers (76). Of note, a delay in the onset of diabetes was observed in the exercise group, allowing for reduced insulin medication (76). Crucial information, however, can be gained from the time course of the measured parameters. The main difference between the intervention and the treatment group in HbA1C as well as weight loss was achieved at 1 year, with a gradual loss of the beneficial effects in the following years. This coincides with a gradual reduction in the frequency of counseling sessions, as well as physical fitness (76). One might therefore speculate that adherence to the exercise protocol declined after 1 year, very likely due to a lack of motivation. Indeed, the American Diabetes Association explicitly stresses the importance of follow-up counseling for the success of weight loss and physical exercise programs for the prevention and/or delay of T2D (1).
The precise control of dietary habits over long periods of time proves equally difficult than adherence to regular exercise regimens. Diets in most long-term studies are only roughly defined, but have been shown to improve endothelial function and inflammatory parameters in patients at high risk to develop coronary artery disease and with a family history of diabetes (66). In a food component analysis, lean fish and raw vegetable consumption were negatively associated with the overall endothelial dysfunction score, while a positive association was established between high-fat dairy products and endothelial dysfunction (66). Beneficial effects of a diet high in cereal-derived fibers on insulin resistance were associated with changes in plasma amino acid signatures, including branched-chain amino acids (77). Those findings are in line with earlier reports suggesting a diagnostic relevance of plasma amino acid levels, especially for insulin resistance and the risk to develop diabetes (77-79). Insulin resistance as well as various inflammatory parameters can also be improved by increasing uptake of mono- or poly-unsaturated as compared to saturated fatty acids (69,80,81).
Despite the adverse effects of extreme obesity, the indiscriminate indication for weight loss is currently under intense discussion and more patient-specific indications will have to be developed, taking into account the individual distribution of AT depots, as well as motivational aspects and obviously co-morbidities. Indeed, we might have to look towards other endpoints, such as a gain of certain fitness levels instead of weight loss (82,83).
Cell therapy
T2D is defined by peripheral tissue insulin resistance. Transplantation of β cells—in contrast to T1D—would therefore not address the peripheral component of the pathological process (84). Promising cell therapy approaches in T2D therefore need to account also for cardiovascular endpoints. Several large trials have successfully demonstrated the safety and efficacy of multipotent mesenchymal stem cells for the treatment of cardiovascular disease (85). Before clinical application, however, several limitations have to be overcome, especially the unsuitability of autologous cells due to their loss of functional capacity in chronic diseases such as CAD and T2D (86-90). Furthermore, the hyper- as well as hypoglycaemic conditions in the recipient can inhibit angiogenic repair capacity of cardiac stem cells (91,92). Another promising cell type, adipose tissue-derived stromal cells, also exert angiogenic effects (93-95), but exhibit a significantly impaired angiogenic capacity when obtained from patients with CAD and T2D (96). This is thought to be due to an altered secretome and delayed differentiation to an endothelial cell phenotype (96).
Beyond the provision of new cellular material, transplanted cells can also be employed for their paracrine actions, thus bypassing the obstacle of inefficient homing and retention at the injection site (97). CD34+ stem cells, ex vivo modified to overexpress the angiogenic factor sonic hedgehog (SHH) exert beneficial effects by secreting SHH laden exosomes, which in turn induce the canonical SHH pathway in the surrounding tissue ultimately leading to a better preservation of myocardial function after MI (98). Similarly, exosomes might be conveyors of cardioprotection in ischemic preconditioning (99). The better characterisation of relevant paracrine factors and the development of non-immunogenic delivery mechanisms, including cell-derived vesicles are therefore of considerable interest for targeting vascular complications in a chronic disease such as diabetes which would require repeated applications.
micro-RNAs (miRNAs)
miRNAs comprise a class of endogenous short non-coding RNAs that regulate gene expression by repressing protein translation or by inducing mRNA degradation (100). miRNAs are involved in virtually every physiological and pathological cellular process, usually targeting several proteins within the same pathway. They have been shown to influence differentiation, function and survival of immune cells, stem cells, vascular endothelial and smooth muscle cells as well as adipocytes and are highly regulated in cardiovascular diseases and diabetes. Two main approaches to miRNA modulation are commonly applied, depending on the desired effect on the target miRNA: miRNA antagonists (antagomirs), lowering the effective levels of the target miRNA, and miRNA mimics, increasing the target miRNA amount (101,102). miRNAs have been recognized as mediators of almost every pathophysiological step of atherosclerosis, diabetes and their culmination in vascular complications (103). To date, more than 2,500 human miRNAs are known, with the number still increasing. We will therefore only discuss selected miRNAs with relevance to vascular regenerative potential and T2D.
Circulating levels of the miR-126—involved in vascular integrity, angiogenesis and wound repair—and the anti-inflammatory miRNAs miR-146a and miR-155 are decreased in patients with T2D resulting in impaired endothelial repair capacity, increased chronic inflammation and atherosclerosis-associated vascular remodeling as well as failing glucose control (104-112). Interestingly, miR-155 also limits BAT genesis and function (113). MiR-155 might therefore potentially also exert negative effects on BAT-mediated glucose tolerance and insulin resistance (114,115). The miR-182 and miR-203 were furthermore identified to regulate BAT development and homeostasis by ablation of Dgcr8, a crucial regulator of microRNA biogenesis (116). Several studies give evidence that miRNAs affect the behavior of stem and progenitor cells which are essential for vascular repair processes (117). For instance, miR-99b, miR-181a and miR-181b contribute to the differentiation of human embryonic stem cells towards an endothelial cell phenotype and render them more efficient in therapeutic revascularization (118). Saphenous vein-derived pericyte progenitor cells depend on paracrine secretion of the pro-angiogenic miR-132 which improves post-infarct fibrosis, reparative angiogenesis and ventricular contractility (119). Caporali et al. demonstrated a significant increase in miR-503 expression in ischemic limb muscles of diabetic mice and of human diabetic muscle specimens (120). Therapeutic downregulation of miR-503 restored endothelial function and angiogenesis and might thus prove a potential regulator of post-ischemic neovascularization (120).
Taken together, modulation of miRNA levels by antagomiRs and miR mimics represents a promising therapy approach for the treatment of vascular dysfunction. Their targeting of multiple gene products, however, requires extensive in vitro and in vivo screening to prevent off-target effects. The same characteristic, on the other hand, could also result in a more efficient targeting of regeneration-specific signaling cascades, as is seen in the case of miR-126, thereby improving the efficiency of potential future miRNA-based drugs.
Dipeptidyl peptidase-4 (DPP-4) inhibition and glucagon-like-peptide-1 (GLP-1) (analogues)
Incretinergic therapies such as dipeptidyl peptidase-4 (DPP-4 or CD26) inhibitors and GLP-1 receptor antagonists or GLP-1 analogues have been proven to be vital new members of the anti-diabetic drug repertoire. Unlike other betacytotrope agents, such as sulfonylureas and glinides, DPP-4 inhibitors and GLP1-antagonists neither induce weight gain nor do they increase the risk of hypoglycaemia (121,122). Furthermore, they can be combined with biguanides if the initial monotherapy fails to sufficiently reduce blood glucose levels (123). DPP-4 inhibitors protect incretin hormones, such as GLP-1, from degradation by DPP-4. GLP-1 is produced and released by neuroendocrine L-cells in the ileum after a meal and exerts its beneficial effects by stimulating pancreatic insulin release, inhibiting glucagon release and by slowing down gastric passage (124). However, DPP-4 inhibitors do not only improve glucose metabolism, but may also hold favourable pleiotropic effects in the cardiovascular and immune system. This is due to the broad substrate affinity of DPP-4, which besides GLP-1 include MCP-1, IL-1, RANTES and SDF-1 (125). Several in vitro and in vivo studies demonstrate the impact of DPP-4 inhibition on the cardiovascular system, including the reduction of intimal hyperplasia formation, and faster regenerative response after experimental vascular injury in rodents (126-128). The protection of the SDF-1/CXCR4 pathway might therefore crucially contribute to the cardiovascular regenerative effects of DPP-4 inhibitors, which are also investigated in several clinical trials (128). Zaruba et al. describe the first treatment of a child with severe ischemic cardiomyopathy with granulocyte colony stimulating factor (G-CSF) and sitagliptin, a concept which they previously demonstrated to be efficient in mouse myocardial infarction (129). The mobilization of stem cells by G-CSF and the stabilization of their homing factor stromal derived factor-1 (SDF-1) resulted in an increase of ejection fraction from 27% to 33% and a decrease in the heart failure markers BNP and pro-BNP (130). In a small non-randomised controlled trial, a 4-week treatment with sitagliptin in addition to metformin or other insulin releasing agents compared to no additional therapy lead to significantly higher levels of circulating CD34+KDR+ cells. This was accompanied by an increase in plasma levels of SDF-1α and a decrease in MCP-1 (131). Furthermore, Tremblay et al. conducted a double-blind crossover study with T2D patients treated with 100 mg/d sitagliptin and were able to observe a significant decrease in plasma markers of low-grade inflammation, such as CRP, IL-18, IL-6, ICAM-1 and E-Selectin, thus demonstrating potentially beneficial off-target effects for this patient population (132). Finally, the retrospective analysis of the LifeLink database indicated that T2D patients had a significantly lower risk of experiencing cardiovascular complications when treated with the GLP-1 analogue exenatide in comparison to other glucose-lowering substances (133). Two large randomized controlled trials, SAVOR and EXAMINE, have assessed the cardiovascular safety profile of saxagliptin (SAVOR) and alogliptin (EXAMINE) and found no increase in adverse cardiovascular events compared to placebo (134,135). However, before implementing DPP-4 inhibition as a standard of care in primary or secondary prevention of cardiovascular events in patients with type diabetes results from double-blind randomised controlled trials such as the TECOS trial have to be awaited (136).
Conclusions
In the recent years, we have come to understand diabetes-associated vascular complications as a result of cascade failures of several interlinked, paracrinally active organ systems, including adipose tissue, the immune system, skeletal muscles and the liver. It appears that effective therapies need to act pleitropically, interfering with several points of this “downward spiral” (Figure 1). Hence, lifestyle interactions have been successful in primary and secondary cardiovascular prevention as well as for the improvement of metabolic parameters. Due to their cost-effectiveness and efficiency, they harbor great potential, if long-term adherence can be improved. Micro-RNA-based strategies have a significant potential, if safety concerns can be overcome. More personalized treatment strategies, such as autologous cell transplantation is met with various limitations, not least economically.
Acknowledgements
None.
Footnote
Conflicts of Interest: The authors have no conflicts of interest to declare.
References
- American Diabetes Association. Standards of medical care in diabetes--2014. Diabetes Care 2014;37 Suppl 1:S14-80. [PubMed]
- Morrish NJ, Wang SL, Stevens LK, et al. Mortality and causes of death in the WHO Multinational Study of Vascular Disease in Diabetes. Diabetologia. 2001;44:S14-21. [PubMed]
- Meliga E, Garcia-Garcia HM, Valgimigli M, et al. Diabetic patients treated for unprotected left main coronary artery disease with drug eluting stents: a 3-year clinical outcome study. The diabetes and drug eluting stent for LeFT main registry (D-DELFT). EuroIntervention 2008;4:77-83. [PubMed]
- Emdin CA, Rahimi K, Neal B, et al. Blood pressure lowering in type 2 diabetes: a systematic review and meta-analysis. JAMA 2015;313:603-15. [PubMed]
- Tu B, Rich B, Labos C, et al. Coronary revascularization in diabetic patients:a systematic review and Bayesian network meta-analysis. Ann Intern Med 2014;161:724-32. [PubMed]
- Farkouh ME, Domanski M, Sleeper LA, et al. Strategies for multivessel revascularization in patients with diabetes. N Engl J Med 2012;367:2375-84. [PubMed]
- BARI 2D Study Group, Frye RL, August P, et al. A randomized trial of therapies for type 2 diabetes and coronary artery disease. N Engl J Med 2009;360:2503-15.
- Scheen AJ, Warzée F. Diabetes is still a risk factor for restenosis after drug-eluting stent in coronary arteries. Diabetes Care 2004;27:1840-1. [PubMed]
- Park SJ, Ahn JM, Kim YH, et al. Trial of everolimus-eluting stents or bypass surgery for coronary disease. N Engl J Med 2015;372:1204-12. [PubMed]
- Bangalore S, Guo Y, Samadashvili Z, et al. Everolimus-eluting stents or bypass surgery for multivessel coronary disease. N Engl J Med 2015;372:1213-22. [PubMed]
- Vazquez-Benitez G, Desai JR, Xu S, et al. Preventable major cardiovascular events associated with uncontrolled glucose, blood pressure, and lipids and active smoking in adults wth diabetes with and without cardiovascular disease: a contemporary analysis. Diabetes Care 2015;38:905-12. [PubMed]
- Jain R, Nallamothu BK, Chan PS, et al. Body mass index and survival after in-hospital cardiac arrest. Circ Cardiovasc Qual Outcomes 2010;3:490-7. [PubMed]
- Unger RH, Scherer PE. Gluttony, sloth and the metabolic syndrome: a roadmap to lipotoxicity. Trends Endocrinol Metab 2010;21:345-52. [PubMed]
- Wang MY, Grayburn P, Chen S, et al. Adipogenic capacity and the susceptibility to type 2 diabetes and metabolic syndrome. Proc Natl Acad Sci U S A 2008;105:6139-44. [PubMed]
- Listenberger LL, Han X, Lewis SE, et al. Triglyceride accumulation protects against fatty acid-induced lipotoxicity. Proc Natl Acad Sci U S A 2003;100:3077-82. [PubMed]
- Kraakman MJ, Murphy AJ, Jandeleit-Dahm K, et al. Macrophage polarization in obesity and type 2 diabetes: weighing down our understanding of macrophage function? Front Immunol 2014;5:470. [PubMed]
- Nishimura S, Manabe I, Nagai R. Adipose tissue inflammation in obesity and metabolic syndrome. Discov Med 2009;8:55-60. [PubMed]
- Zeyda M, Farmer D, Todoric J, et al. Human adipose tissue macrophages are of an anti-inflammatory phenotype but capable of excessive pro-inflammatory mediator production. Int J Obes (Lond) 2007;31:1420-8. [PubMed]
- Boden G, She P, Mozzoli M, et al. Free fatty acids produce insulin resistance and activate the proinflammatory nuclear factor-kappaB pathway in rat liver. Diabetes 2005;54:3458-65. [PubMed]
- Hotamisligil GS, Shargill NS, Spiegelman BM. Adipose expression of tumor necrosis factor-alpha: direct role in obesity-linked insulin resistance. Science 1993;259:87-91. [PubMed]
- Nguyen MT, Favelyukis S, Nguyen AK, et al. A subpopulation of macrophages infiltrates hypertrophic adipose tissue and is activated by free fatty acids via Toll-like receptors 2 and 4 and JNK-dependent pathways. J Biol Chem 2007;282:35279-92. [PubMed]
- Odegaard JI, Chawla A. Alternative macrophage activation and metabolism. Annu Rev Pathol 2011;6:275-97. [PubMed]
- Kanda H, Tateya S, Tamori Y, et al. MCP-1 contributes to macrophage infiltration into adipose tissue, insulin resistance, and hepatic steatosis in obesity. J Clin Invest 2006;116:1494-505. [PubMed]
- Shi H, Kokoeva MV, Inouye K, et al. TLR4 links innate immunity and fatty acid-induced insulin resistance. J Clin Invest 2006;116:3015-25. [PubMed]
- Matter CM, Handschin C. RANTES (regulated on activation, normal T cell expressed and secreted), inflammation, obesity, and the metabolic syndrome. Circulation. 2007;115:946-8. [PubMed]
- Baker RG, Hayden MS, Ghosh S. NF. -κB, inflammation, and metabolic disease. Cell metab 2011;13:11-22. [PubMed]
- Xu H, Barnes GT, Yang Q, et al. Chronic inflammation in fat plays a crucial role in the development of obesity-related insulin resistance. J Clin Invest 2003;112:1821-30. [PubMed]
- Hand LE, Usan P, Cooper GJ, et al. Adiponectin induces A20 expression in adipose tissue to confer metabolic benefit. Diabetes 2015;64:128-36. [PubMed]
- Franckhauser S, Munoz S, Pujol A, et al. Increased fatty acid re-esterification by PEPCK overexpression in adipose tissue leads to obesity without insulin resistance. Diabetes 2002;51:624-30. [PubMed]
- Park M, Yi JW, Kim EM, et al. Triggering receptor expressed on myeloid cells 2 (TREM2) promotes adipogenesis and diet-induced obesity. Diabetes 2015;64:117-27. [PubMed]
- Hajer GR, van Haeften TW, Visseren FL. Adipose tissue dysfunction in obesity, diabetes, and vascular diseases. Eur Heart J 2008;29:2959-71. [PubMed]
- Okamoto Y, Folco EJ, Minami M, et al. Adiponectin inhibits the production of CXC receptor 3 chemokine ligands in macrophages and reduces T-lymphocyte recruitment in atherogenesis. Circ Res 2008;102:218-25. [PubMed]
- Yokota T, Oritani K, Takahashi I, et al. Adiponectin, a new member of the family of soluble defense collagens, negatively regulates the growth of myelomonocytic progenitors and the functions of macrophages. Blood 2000;96:1723-32. [PubMed]
- Yamaguchi N, Argueta JG, Masuhiro Y, et al. Adiponectin inhibits Toll-like receptor family-induced signaling. FEBS Lett 2005;579:6821-6. [PubMed]
- Antoniades C, Antonopoulos AS, Tousoulis D, et al. Adiponectin: from obesity to cardiovascular disease. Obes Rev 2009;10:269-79. [PubMed]
- Yang H, Zhang R, Mu H, et al. Adiponectin promotes endothelial cell differentiation from human peripheral CD14+ monocytes in vitro. J Cell Mol Med 2006;10:459-69. [PubMed]
- Winnik S, Stein S, Matter CM. SIRT1 - an anti-inflammatory pathway at the crossroads between metabolic disease and atherosclerosis. Curr Vasc Pharmacol 2012;10:693-6. [PubMed]
- de Kreutzenberg SV, Ceolotto G, Papparella I, et al. Downregulation of the longevity-associated protein sirtuin 1 in insulin resistance and metabolic syndrome: potential biochemical mechanisms. Diabetes 2010;59:1006-15. [PubMed]
- Escande C, Nin V, Pirtskhalava T, et al. Deleted in breast cancer 1 limits adipose tissue fat accumulation and plays a key role in the development of metabolic syndrome phenotype. Diabetes 2015;64:12-22. [PubMed]
- Chatterjee TK, Basford JE, Knoll E, et al. HDAC9 knockout mice are protected from adipose tissue dysfunction and systemic metabolic disease during high-fat feeding. Diabetes 2014;63:176-87. [PubMed]
- Gil A, Olza J, Gil-Campos M, et al. Is adipose tissue metabolically different at different sites? Int J Pediatr Obes 2011;6 Suppl 1:13-20. [PubMed]
- Cancello R, Tordjman J, Poitou C, et al. Increased infiltration of macrophages in omental adipose tissue is associated with marked hepatic lesions in morbid human obesity. Diabetes 2006;55:1554-61. [PubMed]
- Virdis A, Duranti E, Rossi C, et al. Tumour necrosis factor-alpha participates on the endothelin-1/nitric oxide imbalance in small arteries from obese patients: role of perivascular adipose tissue. Eur Heart J 2015;36:784-94. [PubMed]
- Wang D, Wang C, Wu X, et al. Endothelial dysfunction and enhanced contractility in microvessels from ovariectomized rats: roles of oxidative stress and perivascular adipose tissue. Hypertension 2014;63:1063-9. [PubMed]
- Lee MH, Chen SJ, Tsao CM, et al. Perivascular adipose tissue inhibits endothelial function of rat aortas via caveolin-1. PLoS One 2014;9:e99947. [PubMed]
- Manka D, Chatterjee TK, Stoll LL, et al. Transplanted perivascular adipose tissue accelerates injury-induced neointimal hyperplasia: role of monocyte chemoattractant protein-1. Arterioscler Thromb Vasc Biol 2014;34:1723-30. [PubMed]
- Gil-Ortega M, Condezo-Hoyos L, Garcia-Prieto CF, et al. Imbalance between pro and anti-oxidant mechanisms in perivascular adipose tissue aggravates long-term high-fat diet-derived endothelial dysfunction. PLoS One 2014;9:e95312. [PubMed]
- Antonopoulos AS, Margaritis M, Coutinho P, et al. Adiponectin as a link between type 2 diabetes mellitus and vascular NADPH-oxidase activity in the human arterial wall: the regulatory role of perivascular adipose tissue. Diabetes 2015;64:2207-19. [PubMed]
- Shimizu I, Aprahamian T, Kikuchi R, et al. Vascular rarefaction mediates whitening of brown fat in obesity. J Clin Invest 2014;124:2099-112. [PubMed]
- Villarroya J, Cereijo R, Villarroya F. An endocrine role for brown adipose tissue? Am J Physiol Endocrinol Metab 2013;305:E567-72. [PubMed]
- Bagchi M, Kim LA, Boucher J, et al. Vascular endothelial growth factor is important for brown adipose tissue development and maintenance. FASEB J 2013;27:3257-71. [PubMed]
- Lindström J, Ilanne-Parikka P, Peltonen M, et al. Sustained reduction in the incidence of type 2 diabetes by lifestyle intervention: follow-up of the Finnish Diabetes Prevention Study. Lancet 2006;368:1673-9. [PubMed]
- Diabetes Prevention Program Research Group, Knowler WC, Fowler SE. 10-year follow-up of diabetes incidence and weight loss in the diabetes prevention program outcomes study. Lancet 2009;374:1677-86. [PubMed]
- Boulé NG, Haddad E, Kenny GP, et al. Effects of exercise on glycemic control and body mass in type 2 diabetes mellitus: a meta-analysis of controlled clinical trials. JAMA 2001;286:1218-27. [PubMed]
- Mourier A, Gautier JF, De Kerviler E, et al. Mobilization of visceral adipose tissue related to the improvement in insulin sensitivity in response to physical training in NIDDM. Effects of branched-chain amino acid supplements. Diabetes Care 1997;20:385-91. [PubMed]
- Yokoyama H, Emoto M, Fujiwara S, et al. Short-term aerobic exercise improves arterial stiffness in type 2 diabetes. Diabetes Res Clin Pract 2004;65:85-93. [PubMed]
- Stewart KJ. Exercise training and the cardiovascular consequences of type 2 diabetes and hypertension: plausible mechanisms for improving cardiovascular health. JAMA 2002;288:1622-31. [PubMed]
- Knowler WC, Barrett-Connor E, Fowler SE, et al. Reduction in the incidence of type 2 diabetes with lifestyle intervention or metformin. N Engl J Med 2002;346:393-403. [PubMed]
- Herman WH, Hoerger TJ, Brandle M, et al. The cost-effectiveness of lifestyle modification or metformin in preventing type 2 diabetes in adults with impaired glucose tolerance. Ann Intern Med 2005;142:323-32. [PubMed]
- Diabetes Prevention Program Research Group. The 10-year cost-effectiveness of lifestyle intervention or metformin for diabetes prevention: an intent-to-treat analysis of the DPP/DPPOS. Diabetes Care 2012;35:723-30. [PubMed]
- Golbidi S, Badran M, Laher I. Antioxidant and anti-inflammatory effects of exercise in diabetic patients. Exp Diabetes Res 2012;2012:941868.
- Pedersen BK, Febbraio MA. Muscles, exercise and obesity: skeletal muscle as a secretory organ. Nat Rev Endocrinol 2012;8:457-65. [PubMed]
- Ennezat PV, Malendowicz SL, Testa M, et al. Physical training in patients with chronic heart failure enhances the expression of genes encoding antioxidative enzymes. J Am Coll Cardiol 2001;38:194-8. [PubMed]
- Niebauer J, Cooke JP. Cardiovascular effects of exercise: role of endothelial shear stress. J Am Coll Cardiol 1996;28:1652-60. [PubMed]
- Tikhonenko M, Lydic TA, Opreanu M, et al. N-3 polyunsaturated fatty acids prevent diabetic retinopathy by inhibition of retinal vascular damage and enhanced endothelial progenitor cell reparative function. PLoS One 2013;8:e55177. [PubMed]
- van Bussel BC, Henry RM, Ferreira I, et al. A healthy diet is associated with less endothelial dysfunction and less low-grade inflammation over a 7-year period in adults at risk of cardiovascular disease. J Nutr 2015;145:532-40. [PubMed]
- Merino J, Guasch-Ferre M, Martinez-Gonzalez MA, et al. Is complying with the recommendations of sodium intake beneficial for health in individuals at high cardiovascular risk? Findings from the PREDIMED study. Am J Clin Nutr 2015;101:440-8. [PubMed]
- Leo CH, Woodman OL. Flavonols in the prevention of diabetes-induced vascular dysfunction. J Cardiovasc Pharmacol 2015;65:532-44. [PubMed]
- Finucane OM, Lyons CL, Murphy AM, et al. Monounsaturated fatty acid-enriched high-fat diets impede adipose NLRP3 inflammasome-mediated IL-1beta secretion and insulin resistance despite obesity. Diabetes 2015;64:2116-28. [PubMed]
- Hare DL, Ryan TM, Selig SE, et al. Resistance exercise training increases muscle strength, endurance, and blood flow in patients with chronic heart failure. Am J Cardiol 1999;83:1674-7, A7.
- Miller WJ, Sherman WM, Ivy JL. Effect of strength training on glucose tolerance and post-glucose insulin response. Med Sci Sports Exerc 1984;16:539-43. [PubMed]
- Poehlman ET, Dvorak RV, DeNino WF, et al. Effects of resistance training and endurance training on insulin sensitivity in nonobese, young women: a controlled randomized trial. J Clin Endocrinol Metab 2000;85:2463-8. [PubMed]
- Umpierre D, Ribeiro PA, Kramer CK, et al. Physical activity advice only or structured exercise training and association with HbA1c levels in type 2 diabetes: a systematic review and meta-analysis. JAMA 2011;305:1790-9. [PubMed]
- Colberg SR, Sigal RJ, Fernhall B, et al. Exercise and type 2 diabetes: the American college of sports medicine and the American diabetes association: joint position statement. Diabetes Care 2010;33:e147-67. [PubMed]
- Sigal RJ, Kenny GP. Combined aerobic and resistance exercise for patients with type 2 diabetes. JAMA 2010;304:2298-9. [PubMed]
- Look AR, Wing RR, Bolin P, et al. Cardiovascular effects of intensive lifestyle intervention in type 2 diabetes. N Engl J Med 2013;369:145-54. [PubMed]
- Hattersley JG, Pfeiffer AF, Roden M, et al. Modulation of amino acid metabolic signatures by supplemented isoenergetic diets differing in protein and cereal fiber content. J Clin Endocrinol Metab 2014;99:E2599-609. [PubMed]
- Wang TJ, Larson MG, Vasan RS, et al. Metabolite profiles and the risk of developing diabetes. Nat Med 2011;17:448-53. [PubMed]
- Newgard CB, An J, Bain JR, et al. A branched-chain amino acid-related metabolic signature that differentiates obese and lean humans and contributes to insulin resistance. Cell Metab 2009;9:311-26. [PubMed]
- Lamping KG, Nuno DW, Coppey LJ, et al. Modification of high saturated fat diet with n-3 polyunsaturated fat improves glucose intolerance and vascular dysfunction. Diabetes Obes Metab 2013;15:144-52. [PubMed]
- Nettleton JA, Katz R. n-3 long-chain polyunsaturated fatty acids in type 2 diabetes: a review. J Am Diet Assoc 2005;105:428-40. [PubMed]
- Sénéchal M, Rempel M, Duhamel TA, et al. Fitness is a determinant of the metabolic response to endurance training in adolescents at risk of type 2 diabetes mellitus. Obesity (Silver Spring) 2015;23:823-32. [PubMed]
- Lavie CJ, McAuley PA, Church TS, et al. Obesity and cardiovascular diseases: implications regarding fitness, fatness, and severity in the obesity paradox. J Am Coll Cardiol 2014;63:1345-54. [PubMed]
- Pagliuca FW, Millman JR, Gürtler M, et al. Generation of functional human pancreatic β cells in vitro. Cell 2014;159:428-39. [PubMed]
- Friis T, Haack-Sørensen M, Mathiasen AB, et al. Mesenchymal stromal cell derived endothelial progenitor treatment in patients with refractory angina. Scand Cardiovasc J 2011;45:161-8. [PubMed]
- Fadini GP, Miorin M, Facco M, et al. Circulating endothelial progenitor cells are reduced in peripheral vascular complications of type 2 diabetes mellitus. J Am Coll Cardiol 2005;45:1449-57. [PubMed]
- Churdchomjan W, Kheolamai P, Manochantr S, et al. Comparison of endothelial progenitor cell function in type 2 diabetes with good and poor glycemic control. BMC Endocr Disord 2010;10:5. [PubMed]
- Hamed S, Brenner B, Roguin A. Nitric oxide: a key factor behind the dysfunctionality of endothelial progenitor cells in diabetes mellitus type-2. Cardiovasc Res 2011;91:9-15. [PubMed]
- Egan CG, Lavery R, Caporali F, et al. Generalised reduction of putative endothelial progenitors and CXCR4-positive peripheral blood cells in type 2 diabetes. Diabetologia 2008;51:1296-305. [PubMed]
- Kuliszewski MA, Ward MR, Kowalewski JW, et al. A direct comparison of endothelial progenitor cell dysfunction in rat metabolic syndrome and diabetes. Atherosclerosis 2013;226:58-66. [PubMed]
- Molgat AS, Tilokee EL, Rafatian G, et al. Hyperglycemia inhibitsc cardiac stem cell-mediated cardiac repair and angiogenic capacity. Circulation 2014;130:S70-6. [PubMed]
- Fadini GP, Albiero M, Vigili de Kreutzenberg S, et al. Hypoglycemia affects the changes in endothelial progenitor cell levels during insulin therapy in type 2 diabetic patients. J Endocrinol Invest 2015;38:733-8. [PubMed]
- Qayyum AA, Haack-Sørensen M, Mathiasen AB, et al. Adipose-derived mesenchymal stromal cells for chronic myocardial ischemia (MyStromalCell Trial): study design. Regen Med 2012;7:421-8. [PubMed]
- Perin EC, Sanz-Ruiz R, Sánchez PL, et al. Adipose-derived regenerative cells in patients with ischemic cardiomyopathy: The PRECISE Trial. Am Heart J 2014;168:88-95. [PubMed]
- Bura A, Planat-Benard V, Bourin P, et al. Phase I trial: the use of autologous cultured adipose-derived stroma/stem cells to treat patients with non-revascularizable critical limb ischemia. Cytotherapy 2014;16:245-57. [PubMed]
- Policha A, Zhang P, Chang L, et al. Endothelial differentiation of diabetic adipose-derived stem cells. J Surg Res 2014;192:656-63. [PubMed]
- Hong KU, Guo Y, Li QH, et al. c-kit+ cardiac stem cells alleviate post-myocardial infarction left ventricular dysfunction despite poor engraftment and negligible retention in the recipient heart. PLoS One 2014;9:e96725. [PubMed]
- Mackie AR, Klyachko E, Thorne T, et al. Sonic hedgehog-modified human CD34+ cells preserve cardiac function after acute myocardial infarction. Circ Res 2012;111:312-21. [PubMed]
- Giricz Z, Varga ZV, Baranyai T, et al. Cardioprotection by remote ischemic preconditioning of the rat heart is mediated by extracellular vesicles. J Mol Cell Cardiol 2014;68:75-8. [PubMed]
- Guo H, Ingolia NT, Weissman JS, et al. Mammalian microRNAs predominantly act to decrease target mRNA levels. Nature 2010;466:835-40. [PubMed]
- Caporali A, Emanueli C. MicroRNAs in postischemic vascular repair. Cardiol Res Pract 2012;2012:486702.
- van Rooij E. The Art of MicroRNA Research. Circ Res 2011;108:219-34. [PubMed]
- Nishiguchi T, Imanishi T, Akasaka T. MicroRNAs and cardiovascular diseases. BioMed Res Int 2015;2015:682857.
- Zampetaki A, Kiechl S, Drozdov I, et al. Plasma microRNA profiling reveals loss of endothelial miR-126 and other microRNAs in type 2 diabetes. Circ Res 2010;107:810-7. [PubMed]
- Fish JE, Santoro MM, Morton SU, et al. miR-126 regulates angiogenic signaling and vascular integrity. Dev Cell 2008;15:272-84. [PubMed]
- Wang S, Aurora AB, Johnson BA, et al. An endothelial-specific microRNA governs vascular integrity and angiogenesis. Dev Cell 2008;15:261-71. [PubMed]
- Jansen F, Yang X, Hoelscher M, et al. Endothelial microparticle-mediated transfer of microRNA-126 promotes vascular endothelial cell repair via SPRED1 and is abrogated in glucose-damaged endothelial microparticles. Circulation 2013;128:2026-38. [PubMed]
- Baldeón R L, Weigelt K, de Wit H, et al. Decreased serum level of miR-146a as sign of chronic inflammation in type 2 diabetic patients. PLoS One 2014;9:e115209. [PubMed]
- Corral-Fernández NE, Salgado-Bustamante M, Martínez-Leija ME, et al. Dysregulated miR-155 expression in peripheral blood mononuclear cells from patients with type 2 diabetes. Exp Clin Endocrinol Diabetes 2013;121:347-53. [PubMed]
- Fichtlscherer S, De Rosa S, Fox H, et al. Circulating microRNAs in patients with coronary artery disease. Circ Res 2010;107:677-84. [PubMed]
- Zhu N, Zhang D, Chen S, et al. Endothelial enriched microRNAs regulate antiotensin II-induced endothelial inflammation and migration. Atherosclerosis 2011;215:286-93. [PubMed]
- Huang RS, Hu GQ, Lin B, et al. MicroRNA-155 silencing enhances inflammatory response and lipid uptake in oxidized low-density lipoprotein-stimulated human THP-1 macrophages. J Investig Med 2010;58:961-7. [PubMed]
- Chen Y, Siegel F, Kipschull S, et al. miR-155 regulates differentiation of brown and beige adipocytes via a bistable circuit. Nat Commun 2013;4:1769. [PubMed]
- Poher AL, Altirriba J, Veyrat-Durebex C, et al. Brown adipose tissue activity as a target for the treatment of obesity/insulin resistance. Front Physiol 2015;6:4. [PubMed]
- Peirce V, Vidal-Puig A. Regulation of glucose homoeostasis by brown adipose tissue. Lancet Diabetes Endocrinol 2013;1:353-60. [PubMed]
- Kim HJ, Cho H, Alexander R, et al. MicroRNAs are required for the feature maintenance and differentiation of brown adipocytes. Diabetes 2014;63:4045-56. [PubMed]
- Lai WH, Ho JC, Chan YC, et al. Attenuation of hind-limb ischemia in mice with endothelial-like cells derived from different sources of human stem cells. PLoS One 2013;8:e57876. [PubMed]
- Kane NM, Howard L, Descamps B, et al. Role of microRNAs 99b, 181a, and 181b in the differentiation of human embryonic stem cells to vascular endothelial cells. Stem Cells 2012;30:643-54. [PubMed]
- Katare R, Riu F, Mitchell K, et al. Transplantation of human pericyte progenitor cells improves the repair of infarcted heart through activation of an angiogenic program involving micro-RNA-132. Circ Res 2011;109:894-906. [PubMed]
- Caporali A, Meloni M, Völlenkle C, et al. Deregulation of microRNA-503 contributes to diabetes mellitus-induced impairment of endothelial function and reparative angiogenesis after limb ischemia. Circulation 2011;123:282-91. [PubMed]
- Monami M, Iacomelli I, Marchionni N, et al. Dipeptydil peptidase-4 inhibitors in type 2 diabetes: a meta-analysis of randomized clinical trials. Nutr Metab Cardiovasc Dis 2010;20:224-35. [PubMed]
- Drucker DJ, Nauck MA. The incretin system: glucagon-like peptide-1 receptor agonists and dipeptidyl peptidase-4 inhibitors in type 2 diabetes. Lancet 2006;368:1696-705. [PubMed]
- American Diabetes Association. Standards of medical care in diabetes--2014. Diabetes Care 2014;37:S14-80. [PubMed]
- Drucker DJ. The biology of incretin hormones. Cell Metab 2006;3:153-65. [PubMed]
- Drucker DJ. Dipeptidyl peptidase-4 inhibition and the treatment of type 2 diabetes: preclinical biology and mechanisms of action. Diabetes Care 2007;30:1335-43. [PubMed]
- Goto H, Nomiyama T, Mita T, et al. Exendin-4, a glucagon-like peptide-1 receptor agonist, reduces intimal thickening after vascular injury. Biochem Biophys Res Commun 2011;405:79-84. [PubMed]
- Murthy SN, Hilaire RC, Casey DB, et al. The synthetic GLP-I receptor agonist, exenatide, reduces intimal hyperplasia in insulin resistant rats. Diab Vasc Dis Res 2010;7:138-44. [PubMed]
- Brenner C, Kränkel N, Kühlenthal S, et al. Short-term inhibition of DPP-4 enhances endothelial regeneration after acute arterial injury via enhanced recruitment of circulating progenitor cells. Int J Cardiol 2014;177:266-75. [PubMed]
- Zaruba MM, Theiss HD, Vallaster M, et al. Synergy between CD26/DPP-IV inhibition and G-CSF improves cardiac function after acute myocardial infarction. Cell Stem Cell 2009;4:313-23. [PubMed]
- Zaruba MM, Hiergeist L, Mechea A, et al. First treatment of a child suffering from severe ischemic cardiomyopathy with G-CSF and sitagliptin. Int J Cardiol 2013;170:e41-2. [PubMed]
- Fadini GP, Boscaro E, Albiero M, et al. The oral dipeptidyl peptidase-4 inhibitor sitagliptin increases circulating endothelial progenitor cells in patients with type 2 diabetes: possible role of stromal-derived factor-1. Diabetes Care 2010;33:1607-9. [PubMed]
- Tremblay AJ, Lamarche B, Deacon CF, et al. Effects of sitagliptin therapy on markers of low-grade inflammation and cell adhesion molecules in patients with type 2 diabetes. Metabolism 2014;63:1141-8. [PubMed]
- Best JH, Hoogwerf BJ, Herman WH, et al. Risk of cardiovascular disease events in patients with type 2 diabetes prescribed the glucagon-like peptide 1 (GLP-1) receptor agonist exenatide twice daily or other glucose-lowering therapies: a retrospective analysis of the LifeLink database. Diabetes Care 2011;34:90-5. [PubMed]
- White WB, Cannon CP, Heller SR, et al. Alogliptin after acute coronary syndrome in patients with type 2 diabetes. N Engl J Med 2013;369:1327-35. [PubMed]
- Scirica BM, Bhatt DL, Braunwald E, et al. Saxagliptin and cardiovascular outcomes in patients with type 2 diabetes mellitus. N Engl J Med 2013;369:1317-26. [PubMed]
- Green JB, Bethel MA, Paul SK, et al. Rationale, design, and organization of a randomized, controlled trial evaluating cardiovascular outcomes with sitagliptin (TECOS) in patients with type 2 diabetes and established cardiovascular disease. Am Heart J 2013;166:983-9. [PubMed]