Cardiac biomarkers: new tools for heart failure management
Introduction
Heart failure (HF) remains the leading cause of death in the US and worldwide, causing a significant burden on health care systems across the globe (1). According to the 2011 update on the heart disease and stroke statistics, the prevalence of HF in people aged 20 years and older in the United States is approximately 5.7 million (2). Clinically, HF is a complex disease process that can result from a variety of conditions preventing the left ventricle from properly filling and ejecting blood. Patients with acute HF commonly present to the emergency department with symptoms and signs of fluid retention, pulmonary congestion, peripheral edema, decreased exercise tolerance, dyspnea, and fatigue. However, these symptoms and signs can be misleading and have poor diagnostic sensitivity and specificity. In spite of major advances in therapy, prognosis for HF remains poor. Biomarkers, with their objectivity and widespread availability, have an indispensable role in improving HF management. Figure 1 represents various biomarkers reflecting distinct cardiac myocyte pathology along with biomarkers that have demonstrated strong evidence in detecting comorbidities such as acute kidney injury and pneumonia often seen in patients with HF. A multi-marker approach is required to adequately assess the risk profile of a given HF patient. As a result, significant effort has been placed on biomarker research, leading to the emergence of several promising novel cardiac biomarkers for HF diagnosis and risk stratification.
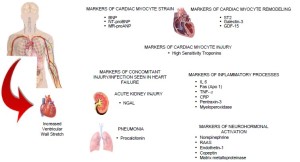
Markers of myocyte strain
The natriuretic peptides, which include B-type natriuretic peptide (BNP) and the N-terminal fragment of its prohormone (NT-proBNP), as well as atrial natriuretic peptide (ANP), adrenomedullin and the mid-regional fragment of the prohormone (MR-proANP), are currently the most widely used markers of myocardial strain. These prohormones are released in hemodynamic stress and processed into biologically active natriuretic peptides, which can counteract the stress by inducing vasodilation, natriuresis and diuresis (3).
BNP is produced from pre-proBNP, a 134 amino acid molecule released from myocytes under stress (4). Once released, plasma BNP binds to the NP receptor A causing a signaling cascade that initiates natriuresis, diuresis, arterial vasodilation, inhibition of cardiac and vascular myocyte growth. BNP has a half life of 20 minutes (5) and is cleared from the circulation via endocytosis, renal filtration or passive excretion. The utility of BNP has been demonstrated in several studies and is perhaps the most widely used biomarker in the assessment of acute HF (6,7).
NT-proBNP is also formed and released as a result of cleavage of its precursor form proBNP that undergoes enzymatic breakdown and processing by two paraprotein convertases, furin (8) and corin (9). NT-proBNP is formed in largest concentration in the left ventricle, but is also detectable to a certain amount in the right atrial and ventricular muscle. NT-proBNP has a half-life of 60-90 minutes and is excreted in its original form via the kidney (10).
While ANP has been slightly less consistent as a diagnostic marker than BNP due to its rapid clearance, the stable mid-regional fragment of proANP (MR-proANP) has been identified as a robust surrogate marker (11). MR-proANP degrades and clears the blood less quickly than ANP or proANP and thus is more reliable as a marker in the clinical setting (9).
Adrenomedullin (ADM) is a 52-amino-acid peptide thought to be upregulated due to increased volume overload and is mediated by vasoactive hormones (12,13). However, due its rapid clearance from the circulation and a short half-life (22 min), using ADM as a routine biomarker is impractical (14). MR-proADM, the mid-regional segment of ADM’s precursor pre-proadrenomedullin, is released in equimolar concentrations as adrenomedullin and thus is an effective substitute, and due its inactivity and longer half-life, MR-proADM is a better surrogate marker (13).
BNP
BNP plasma concentrations fluctuate depending on the disease. BNP increases particularly when there is an abnormal dilatation of the cardiac wall chamber, increased fluid volume or reduced elimination of peptides such as in kidney failure (15).
Factors such as age, BMI, renal function can alter NP levels resulting in “grey-zone” values, so accurate interpretation is critical (16). The grey-zone values are approximately 100-400 pg/mL for BNP (16). In the ED wherein the majority of acute HF patients present, a cut-off of 100 pg/mL to exclude HF is sufficient (in conjunction with other tests) regardless of age and sex (16). Attention to other causes of elevated NP levels such as pulmonary hypertension, right ventricular dysfunction, and valvular disorders must be considered (16,17). Other modalities such as chest X-ray, blood tests must be utilized to make a positive diagnosis (16-18). Close monitoring of such patients is crucial to identify associated comorbidities and modify HF-treatment according to level of risk. In those with high BMI (>35 kg/m2), more rapid clearance of NPs by increased clearance receptors in adipocytes results in a lower BNP (19,20), and thus a lower cut-off value of BNP (<50 pg/mL) can be used to exclude diagnosis of HF (16).
Most patients with acute HF will visit the ED due to an exacerbation of their symptoms or volume overload (21). Using BNP levels in the ED can assess the risk of death or readmission within 30 days (16). In a cohort of 464 patients presenting to the ED with symptoms of HF, Maisel et al. found that the 90-day combined event rate with BNP <200 pg/mL was 9% compared to those admitted with BNP >200 pg/mL, which was 29%. Prognosis of patients with BNP levels <200 pg/mL is excellent, even though the patients were perceived to be in a NYHA functional class III or IV (22).
In those presenting with acute decompensated HF (ADHF), Di Somma et al. (23) found that the optimal times to assess BNP levels are at admission, 24 hours after admission and at discharge. A reduction of 25% or more 24 hours after admission and a reduction of 46% or more of BNP levels at discharge compared with the admission, together with the absolute value of less than 300 pg/mL yielded a strong negative prognosis for future cardiovascular events including readmission or in-hospital mortality, independent of LVEF (23). Analysis of 48,629 patients in the ADHERE cohort showed that risk of mortality varied 3- to 4-fold based on the patient’s initial BNP (24).
In patients with chronic HF, BNP can predict future cardiac events and hospitalizations (25-27). Results from the Val-HeFT trial reported by Masson et al. (25) demonstrated that BNP and NT-proBNP were the strongest predictors of mortality and hospitalization for HF. Increase in BNP levels by 50 ng/mL from baseline carried a high risk compared to the pooled analysis by Doust et al. which demonstrated a 35% increase in risk of mortality with an increase of 100 pg/mL from baseline (26). In another study, BNP level >189 pg/mL after 2 months in addition to >15% change in BNP levels from baseline had worst survival despite being stable after discharge for HF (27). Thus, it is imperative that BNP must be closely monitored after discharge, even in stable patients with chronic HF to identify those at increased risk and judge response to therapy.
Therapy can be guided based on “dry” and “wet” BNP levels (21). The dry BNP level indicates the patient’s baseline euvolemic BNP level and the wet BNP indicates the level resulting from volume overload. Titration of HF therapy and use of diuretic can be modified judging by the decrease in wet BNP levels during treatment. Measuring pre-discharge BNP can help physicians in tailoring HF regimen as well as to stratify patients according to their risk and minimize future cardiac events and lower mortality (28).
NT-proBNP
NT-proBNP is released along with other NPs by the cardiac myocytes in response to increased wall stress due to HF and myocardial dysfunction. In recent years, the quantitative assessment of NT-proBNP has shown to be a useful tool in the identification or exclusion of HF.
In a prospective study assessing the value of NT-proBNP in aiding diagnosis in 221 patients presenting to the ED with acute dyspnea, there was significant correlation between NT-proBNP and the clinical estimate of HF (P<0.0005) (29). Without knowledge of NT-proBNP, HF was diagnosed in 24.0%, lung failure in 60.6%, a combination of heart and lung failure in 10.9% and 4.5% had another diagnosis. Adding NT-proBNP levels to the differential resulted in 24.4% with a diagnosis of HF, 47.5% with lung failure and 23.5% with combined heart and lung failure (29). This study concluded that the additive value of NT-proBNP helped diagnose 18% of patients presenting with acute dyspnea.
NT-proBNP is also prognostic in patients with suspected acute HF (30). In the Canadian multicenter IMPROVE CHF study, Moe et al. (30) demonstrated that NT-proBNP testing improved the management of patients presenting with dyspnea in EDs of Canada and reduced their re-hospitalization rates over 60 days (P=0.023). Adding NT-proBNP to clinical judgment increased the ROC curve to 0.90 from 0.83 (P<0.00001).
In some studies, NT-pro-BNP has been shown to be superior over other natriuretic peptides. BNP, NT-proBNP, and proBNP levels at hospital admission and discharge in patients with ADHF were compared for their predictive value of cardiac death and all cause mortality within 90 days post discharge. NT-proBNP had superior prognostic power for all cause mortality when compared with BNP and proBNP, suggesting that discharge values of NT-proBNP have the greatest diagnostic and prognostic potential of all natriuretic peptides (31).
These studies are just a few to point to the exceptional value of NT-proBNP for the diagnosis, prognosis, and management of patients with HF. While there are many studies suggesting that NT proBNP and BNP are similar in their potential as a marker for heart failure, there are some recent studies underscoring the fact that NT proBNP is a more discerning marker in many common clinical scenarios, such as diastolic HF (32,33). This versatile cardiovascular marker will help optimize the care of a wide range of patients with prevalent cardiovascular illnesses.
Mid-regional pro A-type natriuretic peptide
The diagnostic utility of MR-proANP as compared to BNP was recently demonstrated in the Biomarkers in ACute Heart Failure (BACH) study, a 15-center international trial (34). In the diagnosis of acute HF in those presenting to the ED with dyspnea, a MR-proANP level greater than the predefined cutpoint of 120 pmol/L was found to be non-inferior to BNP at the 100 pg/mL cut point. Table 1 shows the specificity and sensitivity for MR-proANP compared to BNP. Combining MR-proANP and BNP together increased diagnostic accuracy from 73.6% (BNP) to 76.6%. In areas where BNP and NT-proBNP could be less informative (obesity, old age, renal dysfunction, “grey zone” values), MR-proANP adds value when used in combination with each biomarker (34,35).
Table 1
MR-proANP (>120 pmol/L) | BNP (>100 pg/mL) | |
---|---|---|
Sensitivity | 95.56% | 96.98% |
Specificity | 59.85% | 61.90% |
Adapted from: Maisel A, Mueller C, Nowak R, et al. Mid-region prohormone markers for diagnosis and prognosis in acute dyspnea: results from the BACH (Biomarkers in Acute HF) trail. J Am Coll Cardiol 2010;55:2062-76
In the prospective Malmö Diet and Cancer Study, the prognostic values of MR-proANP and five other biomarkers in the development of HF or atrial fibrillation were examined. Of 5,187 participants, 112 developed HF and 284 developed atrial fibrillation within the 14 year follow-up. MR-proANP as well as CRP and NT-proBNP were predictive of the onset of HF, independent of risk factors or other markers (36). In a comparison of NT-proBNP and MR-proBNP in a sample of 525 chronic HF patients of all NYHA classes, MR-proANP was found to be positively correlated with NYHA class, and after correction for NT-proBNP, age, ejection fraction, NYHA class, creatinine, and BMI, MR-proANP was found to be a predictor of poor survival (37). Overall the study concluded that MR-proANP independently predicts mortality, and adds prognostic value when combined with NT-proBNP (37).
MR-proANP has both diagnostic and prognostic utility in the treatment of HF patients. Used correctly, MR-proANP can aid in risk stratification, particularly in unsure cases and in concert with BNP and other biomarkers.
Adrenomedullin
The BACH trial (34) also evaluated the prognostic utility of MR-proADM in acute HF patients presenting to the ED with dyspnea. Results revealed that MR-proADM performed better to BNP/NT-proBNP in predicting 90-day death or re-hospitalization due to cardiovascular causes. Survivors were found to have median MR-proADM level of 0.84 nmol/L compared to non-survivors-1.57 nmol/L (P<0.0001) (34). A follow-up study (38) to the BACH trial concluded that biomarkers that proved superior to natriuretic peptides in predicting 14-day mortality were Copeptin and MR-proADM. Furthermore, combining MR-proADM and copeptin in predicting 14-day mortality achieved the best AUC of 0.818 (38). Klip et al. (39) examining the prognostic potential of MR-proADM in patients developing HF after AMI demonstrated that those with elevated MR-proADM levels had a threefold increased risk of all-cause mortality.
Numerous studies conducted over the last decade have expanded our knowledge regarding the role of adrenomedullin in prognosticating patients with acute and chronic HF. Although the data suggests that MR-proADM is a robust marker in predicting mortality in patients with HF, further evidence determining its superiority to benchmark markers such as natriuretic peptides is warranted.
Markers of myocyte remodeling
ST2
ST2, an inflammatory cytokine and member of the interleukin (IL–1) receptor family, appears to predict mortality and heart failure in patients with acute MI and may play a vital role in cardiac pathophysiology (40-45). ST2 is thought to be involved in modifying immunologic processes, specifically mediated by T-helper 2 lymphocytes (46). Interleukin-33, a hormone which may protect against LVH and myocardial fibrosis (43) has recently been identified as the ligand for ST2 (42). The interaction between IL-33 and ST2L is necessary for the protective effect of IL-33 making it counterintuitive that high levels of sST2 have a deleterious effect. Because sST2 lacks both the transmembrane and the intracellular domains of its membrane-bound counterpart ST2L, excess levels of sST2 bind and neutralize IL-33 without subsequently activating the beneficial signaling cascade. In this way, sST2 acts as a decoy receptor, limiting the availability of IL-33 to bind and activate the protective effects of ST2L (44,45).
The PRIDE study found that in those presenting to the ED with dyspnea, ST2 levels were significantly higher in those diagnosed with acute HF versus those without (47). Higher median concentrations of ST2 were seen in patients with ADHF plus impaired left ventricular systolic function than those with non-systolic HF. ST2 was demonstrated to be at least as predictive of death as NT-proBNP. Even in patients with elevated values of NT-proBNP, the majority of events occurred in subjects with elevated ST2 levels at presentation. Patients with elevated levels of both ST2 and BNP are at a considerably high risk of death compared with patients with none or with only one marker elevated (47,48).
As shown by Shimpo et al. (49), serum ST2 levels are important predictors of death and heart failure in patients presenting with ST-elevation myocardial infarction (STEMI). For the combined cohort of 810 patients in the TIMI-14 and ENTIRE-TIMI-23 trial with acute MI, baseline levels of ST2 were significantly higher in those patients who died or developed new congestive heart failure by 30 days. Moreover, in an analysis by quartiles of ST2, the risk of death and the composite of death or congestive heart failure increased in a graded stepwise fashion with higher levels of ST2. Overall, serum ST2 may be of prognostic value in assessing risk for heart failure and death.
Growth differentiation factor-15
GDF-15 is a member of the transforming growth factor beta-cytokine superfamily and has been recognized as a potential biomarker of HF (50). GDF-15 is expressed at its highest levels in the liver (51), but under conditions of stress in the heart such as inflammation, GDF-15 is released by cardiac myocytes, with a normal circulating concentration of <1,200 ng/L (52-54). GDF-15 exhibits protective effects in the heart by inhibiting apoptosis, hypertrophy, and adverse remodeling in the injured heart (55). Overexpression of GDF-15 is also associated with other conditions including certain cancers, pregnancy (56), atherosclerosis, and vascular injury (57).
In post-MI patients, GDF correlates with NT-proBNP and together increased risk of death or HF above other clinical factors, even allowing patients to be stratified into high, medium, and low risk groups (58). GDF-15 appears to have a graded relationship with all-cause mortality (54,55). Higher levels of GDF-15 on admission had higher death rates at 1 year: those in the >1,800 ng/L range had a 14% death rate compared with 5% death (59). In the Val-HEFT study, elevated GDF-15 was associated with an increased risk of mortality or a first morbid event in those with symptomatic heart failure. Combined with BNP and TnT, GDF-15 allowed patients to be categorized into subgroups with very different risks of adverse events (ischemic vs. non-ischemic) (60). Higher levels were associated with features of worse heart failure and biomarkers of neurohormonal activation, inflammation, myocyte injury, and renal dysfunction (60).
In patients with stages A-C of early HF, GDF-15 was elevated compared to healthy controls, progressively increased with worsening heart disease, and correlated with several echocardiographic variables of structural abnormality (61). GDF-15 can be used to identify those patients who have HF with a preserved ejection fraction (HFpEF) (62). GDF-15 may also help distinguish between patients with normal diastolic function and patients with asymptomatic LV diastolic dysfunction (63).
Overall, GDF-15 is valuable as both a prognostic and diagnostic marker in HF, although its lack of specificity makes it unable to be used as a lone biomarker for HF. GDF-15 should be used in combination with other clinical factors and indicators for more specific information.
Galectin-3
Inflammatory and fibrotic processes are central to cardiac remodeling and the development of HF. Galectin-3, secreted by activated macrophages, causes cardiac fibrosis via proliferation of cardiac fibroblasts and deposition and irreversible cross-linking of collagen I in myocytes (64). Recent examinations of galectin-3 in the context of HF have revealed the potential clinical value of galectin-3 as a prognostic indicator (65-67). Most commonly known for its regulation in inflammation, immunity, and cancer, galectin-3 may be a player in cardiac pathophysiology and act as surrogate indicator of cardiac remodeling and fibrosis apparent in HF (67).
In the PROVE IT-TIMI 22 study (65), higher galectin-3 levels in patients hospitalized for ACS showed a positive graded relationship with the development of HF. After adjustment for prior HF, prior MI, hypertension, and diabetes, this correlation remained significant, though accounting for BNP slightly attenuated the relationship (65). In the COACH trial, doubling of galectin-3 was associated with twice the risk of death or rehospitalization over a mean follow-up period of 18 months. Those with NYHA class III and IV had higher galectin-3 than NYHA II patients (P<0.001). Galectin-3 was also found to be correlated with the inflammatory cytokines CRP, interleukin-6 (IL-6), and vascular endothelial growth factor (VEGF) (66). In another study, plasma galectin-3 was not very valuable in the diagnosis of HF, but had strong prognostic value in the prediction of death and recurrent HF within 60 days (68).
Expression of galectin-3 appears to occur before evident HF and thus may be more useful to predict and prevent disease. Ideally, future applications of galectin-3 may be to classify and risk stratify the type of HF into “remodeling” (high risk) and “non-remodeling” (low risk) to help physicians tailor their treatment plans accordingly.
Markers of myocyte injury
High sensitivity troponin
Patients with acute HF often have ischemic events as the predominant cause (69). When comparing high sensitive cTnI and NT-proBNP to cardiac troponins measured using conventional assay in 258 patients with congestive HF (EF<45%), high sensitive cTnI (>0.03 ng/mL, P=0.016) and NT-proBNP (>627 pg/mL, P=0.0063) were single best independent prognostic predictors where as cardiac troponins (>0.03 ng/mL) measured using conventional assays was not. On multivariate analysis in predicting mortality, those with elevated high sensitivity cTnI and NT-proBNP had a hazard ratio of 5.74 (P<0.0001) (70).
High sensitivity assays have certainly improved diagnostic capabilities in patients presenting with acute HF. In a study done by Xue et al. (71) in 144 acute HF patients demonstrated that those with troponin I levels >23.25 ng/mL were at increased risk of mortality and HF-related readmission (P=0.003) and elevated troponin I and BNP had the highest combined event rate of 39% (71).
Several studies have assessed the importance of measuring high sensitive cardiac troponins that are detected in the serum in patient with stable-chronic HF in order to establish prognostic potential and future cardiovascular events (72,73).
The utility of cardiac troponinT (cTnT) in detecting sub-myocardial injury was demonstrated by Miller et al. (73) in 172 asymptomatic patients with class III and IV HF. Results revealed that in patients with consistently elevated troponin levels (>0.01 ng/mL) even in the absence of clinical symptoms were at an increased risk of mortality and cardiac transplantation.
The advent of high sensitive assays has made it easier to detect previously undetectable levels of cardiac troponins. Latini et al. (74) demonstrated elevations in high sensitive assay cardiac troponin T (Hs-cTnT) in 90% of participants with a 35.6% associated mortality risk (P<0.0001). Furthermore, the addition of BNP and/or Hs-cTnT improved prognostic potential in patients with chronic HF. Expanding on the role of NPs and cTn in risk stratification of chronic HF patients, Tsutamoto et al. (75) demonstrated that elevations in NT-proBNP (>627 pg/mL) and Hs-cTnI (>0.03 ng/mL) carried the highest risk of mortality (P<0.0001).
Pascual-Figal et al. (76) demonstrated that in 107 patients hospitalized with ADHF, sST2, high sensitive troponin T and NT-proBNP were all independently predictive of higher risk of death and 1-year mortality and measuring their levels individually or in combination may provide additional prognostic information in patients with ADHF.
Cardiac troponins measured using high sensitive assays have proved to be superior to conventional assays. Future studies may benefit from exploring the importance of serial monitoring of high sensitive troponins in larger populations with emphasis on accurate interpretation of troponin levels in order to detect all patients with myocardial injury and heart failure.
Markers in comorbidities associated with HF
Neutrophil gelatinase-associated lipocalin
Neutrophil gelatinase-associated lipocalin (NGAL) is a protein of the lipocalin family that consists of polypeptide chain of 178-amino-acids with a molecular mass of 25 kDa. It is expressed by neutrophils and various epithelial cells (77,78). Being an acute phase protein its expression is up-regulated under diverse conditions (79-82).
There is evidence that NGAL is one of the earliest kidney biomarkers of nephrotoxic and ischemic injury in animal models and is elevated in the urine and blood of humans soon after acute kidney injury (AKI) (83). NGAL has gained attention as a structural biomarker in the urine and plasma for an early AKI diagnosis and for the prediction of clinical outcomes including mortality and the need for renal-replacement therapy. Serum and urine levels of NGAL have been studied in both chronic and acute HF and have shown much superior performance to serum creatinine in the rapid and early detection of acute kidney injury (84-86).
Chronic HF patients have been found to have significantly higher levels of both serum and urine NGAL when compared with control subjects, despite having only modest reductions in estimated glomerular filtration rates (eGFR) (84,85,87-89). Damman et al. (90) demonstrated that urinary NGAL was significantly associated with primary outcome after adjustment for risk factors and clinical variables (P=0.042). This relationship remained significant even in those with normal GFR. Perhaps urine NGAL is a better marker of prognosis in chronic HF patients due to the fact that these patients have a high risk of cardiorenal insults from not only prerenal decreases in renal blood flow but also from nephrotoxic therapies such as diuretics.
NGAL also seems to have a role as a biomarker in cases of acute HF. In the (OPTIMAAL) study done by Dickstein et al. (91) in a subgroup of 236 patients with acute HF following MI, serum NGAL levels were found to be elevated both at baseline and on followup in patients with NYHA class III (vs. NYHA I/II). Patients with baseline serum NGAL levels above the median showed a trend to higher incidence of the composite endpoint of nonfatal MI, CV death, all-cause death, and stroke.
The recent GALLANT study (92) demonstrated the prognostic utility of plasma NGAL along with BNP in 186 patients with ADHF. Patients with higher NGAL levels had significantly more HF-related adverse outcomes in 30-days than those with lower levels (134 vs. 84 ng/mL; P<0.001) and those with elevations in both NGAL and BNP were at significant risk for HF events (P=0.006) as was also the case for those with high NGAL but low BNP (P=0.036). These results suggest that using both markers can serve as powerful tool in the risk stratification for those with acute HF (92).
Future research can benefit by further exploring the role of NGAL in combination with NPs in order to reduce diuretic overuse and timely discharge patients with acute and chronic HF.
Procalcitonin
In the ED, the diagnosis and management of patients suspected of HF presenting with dyspnea can be an incredible challenge if pneumonia is present. Low sensitivity and specificity of clinical symptoms/ signs and chest X-ray imaging make it difficult to distinguish cardiac from non-cardiac dyspnea (93).
Procalcitonin (PCT) is a serum calcitonin precursor and has been shown to be elevated in both gram-positive and gram-negative bacterial infections while being attenuated in viral infections (93-96). Studies suggest that PCT levels strongly correlate with the severity of infections and are thus able to guide antibiotic therapy in patients with respiratory tract infections (96).
The recently conducted follow-up study to the BACH trial (97) demonstrated the diagnostic utility of PCT in 1,641 patients presenting with dyspnea to the ED. PCT was significantly associated with all-cause 90-day mortality in those with acute HF (P=0.0024) and was found to be very useful in guiding antibiotic therapy in those with pneumonia and acute HF. When divided into groups based on PCT levels, various survival trends were found. Those with PCT >0.21 ng/mL not treated with antibiotics had lower survival rates (P=0.046). Moreover, those with PCT <0.05 ng/mL, had increased mortality if receiving antibiotic therapy (P=0.049).
While these results need to be validated in a large randomized control trial, early indications imply PCT could be a valuable diagnostic marker in patients with undifferentiated dyspnea presenting to the ED able to diagnose pneumonia and guide antibiotic treatment.
Inflammatory markers in heart failure
Inflammatory markers have been identified as potential indicators of heart failure and future adverse events. Proinflammatory cytokines may represent a class of biological mediators that are activated in CHF, akin to but distinct from neurohormones and the natriuretic peptide pathways. Plasma levels of these inflammatory markers are useful in prediction of new heart failure as well as in risk stratification of established heart failure.
IL-6
IL-6 is a pleiotropic cytokine with a broad range of humoral and cellular immune effects (98-102). IL-6 is produced not only by immune cells and immune accessory cells but also by cardiovascular components, such as endothelial cells, vascular smooth-muscle cells, and ischemic myocytes (103-106). Elevated levels of IL-6 have also been found in patients suffering from acute and chronic heart failure. Raised levels of IL-6 correlate with severity of NYHA functional class, lowered ejection fraction, and are associated with poor prognosis (107-110). Pudil et al. (111) explored the prognostic role of IL-6 in patients presenting with acute decompensated heart failure (ADHF). Plasma levels of IL-6 were found to be significantly elevated in patients with ADHF as compared to normal controls. Significant positive correlations were also found between plasma IL-6 and NT-proBNP levels. Both remained strong independent predictor of 1-year mortality.
In another study (112), Maeda et al. demonstrated that among the neurohumoral factors and cytokines measured at baseline and three months after optimized treatment for heart failure, only sustained high levels of BNP and IL-6 at three months are independent risk factors of mortality in patients with CHF, despite improvements in left ventricular ejection fraction and symptoms.
Fas (Apo 1)
Fas, also called apoptosis antigen-1 (Apo1), is a member of the TNF family, which mediates apoptosis. Fas is found in various tissues including the thymus, liver, ovary, lung and heart (113-115). In cardiomyocytes, Fas is upregulated as a result of apoptotic cell death due to hypoxia (115) and overstretching (116). A soluble form of Fas (sFas) lacking transmembrane domain was also found in serum of human subjects (117) & is thought to be increased in patients with cardiovascular diseases.
Okuyama et al. found that the circulating sFas level was higher in 61 patients with varying degrees of CHF and actually increased in relation to severity of CHF (118). It was also demonstrated that sFas was related to soluble forms of the similar receptor family, sTNF-R1 and sTNF-R2 and may play an important role in determining severity of CHF. Tsutamoto et al. (119) investigated the relationship between plasma levels of cardiac natriuretic peptides and those of sFas and TNF-α. In 96 patients with HF, they found no significant correlation between sFas levels and those of ANP or BNP. Plasma levels of sFas were significantly higher in patients with severe CHF than in patients with mild CHF. Also, stepwise multivariate analysis demonstrated that high levels of sFas and BNP and a low ejection fraction were independent significant prognostic predictors. This study clearly demonstrated that patients with high sFas levels had a significantly higher death rate than those with low sFas levels and plasma sFas is a useful prognostic marker in pathogenesis of CHF.
TNF-α
Tumor necrosis factor alpha, a classic biomarker of inflammatory processes, has displayed clinical utility as a marker in cases of heart failure (120). In fact, myocardial TNF-α and its receptors (type 1 and type 2) are intricately involved in the pathogenesis of HF. Specifically, excessive expression of TNF-α and type-1 receptor stimulation can lead to cardiac hypertrophy, fibrosis, contractile dysfunction and apoptosis while lower TNF-α and type-2 receptor activation is cardioprotective (121). A study of 1200 HF patients found that TNF-α levels were related directly to NYHA stage and higher levels were associated with poorer prognosis (122). A more recent study found that in those with recently diagnosed HF, TNF-α level were associated with abnormal left atrial function and advanced left systolic and diastolic dysfunction (123). Interestingly, soluble type-1 TNF-α receptor was the only independent predictor of future HF or death after adjustment for clinical and baseline characteristics in a study of patients with recent MI (124). While much of the research on TNF-α and HF is promising, most clinicians would seem to demand a biomarker more specific to the HF disease process.
CRP
Another classic marker of inflammation, the acute phase protein C-reactive protein (CRP) has been associated with presence of heart failure (125-128) and cardiovascular risk (129). Several studies to date have examined what role, CRP may play in cases of HF. Cesari et al. demonstrated that elevated CRP values in elderly patients can predict future HF onset (128). A large study by Engstrom et al. on members of the general population found that those with a CRP ≥3 mg/L were twice as likely to be hospitalized with HF over a mean follow-up of 13 years (130). Unfortunately, CRP lacks specificity to HF and cardiovascular disease, perhaps even more so than TNF-α. CRP's correlation with functional parameters such as EF has been inconsistent however as some studies have found significant correlation whiles other haves not (131). CRP may have some clinical use in cases of HF, but it is not an ideal biomarker for the disease.
Pentraxin-3
Pentraxin-3 (PTX3), a member of the pentraxin family is believed to be a more specific marker of vascular inflammation than CRP and other members of its family (132). PTX3 has also been shown to predict unfavorable clinical outcomes in those with HF (133,134). A recent study by Matsubara et al. (135) demonstrated that PTX3, TNF-α and IL-6 but not hsCRP were elevated significantly in those with HFPEF (HF with preserved EF) but not in those without HF (136). Furthermore, multivariate analysis showed that only elevated PTX3 among inflammatory markers correlated with HFPEF (P<0.01) and in those with LV diastolic dysfunction but without HF (P<0.05). Another study demonstrated that patients with stable CAD, PTX3 had a HR of 1.5 (P=0.21) after adjustment for eGFR; perhaps showing some merit in this study population (132). Research so far indicates that PTX3 could be a significant marker of vascular inflammation, diastolic dysfunction and HF. Large randomized controlled trials are necessary to confirm and elaborate on these early findings.
Myeloperoxidase
Myeloperoxidase (MPO) is an enzyme derived from leukocytes and endothelial cells that catalyzes the creation of several reactive oxidant molecules (including LDL oxidation) and contributes to endothelial dysfunction by reducing levels of nitric oxide (136-138). It has demonstrated prognostic value in cases of ACS and chest pain (139-141) and has recently been studied successfully in cases of HF demonstrating positive correlation with both NYHA stage and diastolic dysfunction (142,143). In a study of 140 patients with chronic systolic HF, the combination of MPO and BNP increased the prognostic accuracy of future events to AUC 0.70 (P=0.0004) compared to AUC 0.66 (P=0.0003) for BNP alone (144). Tang et al. came across an interesting finding when examining a population of 3,733 healtly elderly subjects: the relation between HF risk and elevated MPO was greater in patients without traditional risk factors (i.e. systolic BP <136 mmHg, age <75, no diabetes) (137). This could be a valuable utility in clinical situations as current screening methods do not have the ability to risk stratify those lacking traditional CV risk factors. With further research, MPO could perhaps fill this void and help countless clinicians better manage HF risk.
Markers of neurohormonal activation
Norepinephrine; renin-angiotensin-aldosterone system; endothelin-1/CT-proET-1
Heart failure has long been considered to be primarily a hemodynamic disorder, in which heart pumps insufficient blood and characterized by symptoms of congestion. In recent years, neurohormonal activation has been found in heart failure, particularly of the sympathetic nervous system (145,146) and the renin-angiotensin-aldosterone system (147). However, the utility of neurohormones as diagnostic or prognostic biomarkers in heart failure is varied, particularly due to the influence of blocking agents commonly used in heart failure, including beta-blockers, ACE inhibitors, and aldosterone receptor blockers (ARBs) (148).
Increased levels of plasma and urinary excretion of norepinephrine were found to be independent predictors of mortality (145) and led to the investigation of the deleterious effects of neurohormonal activation present in HF (146). In the Val-HeFT trial, the prognostic utility of BNP, norepinephrine, renin activity (PRA), aldosterone, and endothelin were compared in 4,300 patients with stable heart failure of NYHA II-IV severity. Aside from BNP, which was most strongly associated, in multivariate analysis norepinephrine was the only marker significantly associated with poor outcome after an average follow-up period of 23 months (149). However, Givertz and Braunwald comment that the use of norepinephrine as a routine clinical biomarker is impractical given the need for high-performance liquid chromatography, and thus is not a marker to be relied on particularly at points of care (150). In the VERITAS study, CRP, BNP-32, endothelin-1, and norepinephrine among other markers were measured at baseline, 24 and 48 h and 7 and 30 days in patients with acute heart failure. On univariate analysis, CRP, BNP and endothelin-1 predicted worse outcomes at 30 days; on multivariate analysis, only CRP and BNP were found to be significantly associated with this outcome. After consideration of other variables including age, baseline blood pressure, serum sodium and creatinine, only age and BNP were significant (151).
In an examination of the predictive value of plasma renin activity (PRA) among other neurohormone biomarkers, ejection fraction, NT-proBNP, and PRA were independent predictors of cardiac death in those with heart failure. Elevated levels of both NT-proBNP and high PRA indicated greatest risk of cardiac death. Overall, PRA resulted to be an independent prognostic marker in those with systolic heart failure, and added prognostic value to NT-proBNP and ejection fraction. PRA may be most useful in identifying those needing better therapy, particularly RAAS blockade (152).
Endothelin-1, a potent vasoconstrictor and potentiator of sympathetic neurohormones produced in the endothelial cells of blood vessels, is also a marker of sympathetic activation. Plasma levels of both endothelin-1 and its precursor, big endothelin-1, are increased in heart failure and correlated with pulmonary artery pressure, disease severity, and mortality (153,154). A recently identified stable surrogate marker of endothelin-1, C-terminal pro-endothelin-1 (CT-proET-1), has also been suggested as a prognosticator. In a recent study on outcomes in 3,717 patients with stable coronary artery disease, after adjustment for clinical cardiovascular risk predictors and ejection fraction, elevated levels of CT-proET-1 as well as MR-proANP and MR-proADM were independently correlated with risk of cardiac death or heart failure (155). In another sample of 491 patients with systolic heart failure, increasing CT-proET-1 was correlated with increased mortality at 12 months (156). However, endothelin-1 or CT-proET-1 has not yet become an established marker of prognosis (157). Furthermore, recent investigations into endothelin-1 receptor blockers as a potential therapy have not demonstrated significant clinical benefits or preventive effects (154,157).
Overall, due to clinical impracticality and widespread use of diuretics and neurohormonal blockade, norepinephrine, angiotensin, and aldosterone are not ideal prognosticators of disease. Renin may prove to be useful in assessing the effectiveness of neurohormone blockade therapies. CT-proET-1 may be an emerging biomarker with prognostic potential, though further research is necessary.
AVP/Copeptin
Arginine vasopressin, released from the posterior pituitary and a major player in fluid homeostasis, is also noted to be 2-3 times higher in heart failure patients (158). However, AVP as a biomarker has not been used due to short half life and impracticality in measuring. The precursor of AVP, preprovasopressin, is also the parent hormone to a more stable fragment, C-terminal proAVP or copeptin (159). Copeptin is stable in serum at room temperature, is quicker to assay, and is generally a robust surrogate marker of AVP.
Several studies recently have indicated the possible prognostic value of copeptin in heart failure patients. In the BACH trial, copeptin was associated with significantly worse outcomes including increased mortality, readmissions, and emergency department visits at 90 days, particularly in those with hyponatremia. Copeptin was highly prognostic for adverse events and added prognostic value to clinical indicators, natriuretic peptide markers, and serum sodium (160). In another recent study done in Danish heart failure clinic on 340 patients, copeptin was found to be a significant predictor of hospitalization or death but did not predict mortality independent from NT-proBNP (161). Copeptin has also been shown to be associated with NYHA functional class and was the strongest predictor of mortality in patients with class II or III heart failure. In this study, copeptin was stronger than BNP and NT-proBNP, but they appear to be closely related (162).
Copeptin as a surrogate marker of AVP appears to be an emerging biomarker with good prognostic potential and may add information to natriuretic peptide biomarkers.
Matrix metalloproteinases
As mediators of collagen metabolism and extracellular matrix (ECM) homeostasis (163), the extensive matrix metalloproteinase family has rightly come to the attention of researchers seeking biomarkers for heart failure. Some precursors to clinical HF such as LVH involve ventricular remodeling and the enzymes behind this pathophysiology can offer unique insight into the disease process and HF risks within each individual (164).
The strength of MMP’s as biomarkers may come from their diversity. They are organized into several different classes such as the collagenases (MMP-1, MMP-8), the gelatinases (MMP-2, MMP-9) and the stomelysins (MMP-3, MMP-7) (162). These enzymes have been analyzed individually to varying degrees of success. For example, MMP-2 has been suggested to be more useful than BNP in the identification of HFPEF (165), while its fellow gelatinase MMP-9 was found to be inferior to both BNP as a marker of remodeling (166) and to tissue inhibitor of MMP-1 (TIMP-1) as a predictor of mortality in those with chronic HF (167).
While stand-alone marker results for MMP’s have varied, there may be strength in numbers as far as the utilization of a MMP biomarker panel. In a study of 144 subjects with LVH but no HF, Zile et al. found that panels of MMP's along with clinical covariates (such as BP, age, sex, BMI) performed better than individual markers, clinical covariates alone or NT-proBNP alone. Specifically, for detection of LVH, the panel of MMP-7, MMP-9, tissue inhibitor of MMP-1 (TIMP-1), collagen III N-terminal polypeptide (PIIINP) and NT-proBNP when combined with clinical covariates had an AUC of 0.80 (164). Similar success was found in individuals with LVH that had progressed to HFPEF (164). The authors suggest that panels such as these could better reflect the complex pathophysiology occurring in cases of ventricular remodeling and allow those at early stages of these changes to be identified before the progression to overt HF or before the worsening of existing HFPEF.
Panels such as these, if validated could become a powerful tool in identifying risk and simplifying the diagnosis of a condition (HFPEF) that currently requires costly investigations and subspecialist interpretations.
Conclusions
Table 2 shows a hypothesized strength of evidence table for different biomarkers based on a review of previous supporting literature. Cardiac biomarkers with their objectivity, reproducibility and accessibility are excellent adjuncts to physical examination and imaging studies in HF diagnosis and risk stratification. With advances in medical research, new biomarkers representing different physiological processes continue to emerge, providing an ever clearer risk profile for patients with HF. Biomarkers not only serve as traditional predictors of prognosis, they can also help to identify high-risk patients who need closer monitoring and more aggressive therapy. By continually enhancing our understanding of underlying pathophysiology of HF, improving our ability to identify high risk patients, and helping us to tailor therapies to an individual’s unique risk profile, biomarkers will undoubtedly improve the effectiveness of HF therapy and lead to better patient outcomes.
Table 2
Biomarker | Diagnostic Capability | Prognostic Capability |
---|---|---|
BNP | +++ | +++ |
NT-proBNP | +++ | +++ |
ST2 | - | ++ |
GDF-15 | - | + |
Galectin | - | + |
MR-proANP | ++ | ++ |
NGAL | +++ | +++ |
Hs-Tn | +++ | +++ |
MR-proADM | - | +++ |
Procalcitonin | +++ | +++ |
+: indicates strength; -: indicates lack of data suggesting strength; NT-proBNP: Amino terminal pro B-type natriuretic peptide; GDF-15: growth-differentiation factor-15; Hs-Tn: high sensitivity troponins; MR-proANP: Mid-regional atrial natriuretic peptide; MR-proADM: Mid-regional pro adrenomedullin
Acknowledgements
Disclosure: The authors declare no conflict of interest.
References
- Haldeman GA, Croft JB, Giles WH, et al. Hospitalization of Patients with HF: National Hospital Discharge Survey, 1985 to 1995. Am Heart J 1999;137:352-60. [PubMed]
- Roger VL, Go AS, Lloyd-Jones DM, et al. Heart Disease and stroke statistics: 2011 update: a report from American Heart Association. Circulation 2011;123:e18-e209. [PubMed]
- Nakao K, Ogawa Y, Suga S, et al. Molecular biology and biochemistry of the natriuretic peptide system. II: Natriuretic peptide receptors. J Hypertens 1992;10:1111-4. [PubMed]
- Jortani SA, Prabhu SD, Valdes R Jr. Strategies for developing biomarkers of HF. Clin Chem 2004;50:265-78. [PubMed]
- Saremi A, Gopal D, Maisel AS. Brain natriuretic peptide-guided therapy in the inpatient management of decompensated HF. Expert Rev Cardiovasc Ther 2012;10:191-203. [PubMed]
- Mueller C, Scholer A, Laule-Kilian K, et al. Use of B-type natriuretic peptide in the evaluation and management of acute dyspnea. N Engl J Med 2004;350:647-54. [PubMed]
- Xu-Cai YO, Wu Q. Molecular forms of natriuretic peptides in HF and their implications. Heart 2010;96:419-24. [PubMed]
- McCullough PA, Omland T, Maisel AS. B-type Natriuretic Peptides: A Diagnostic Breakthrough for Clinicians. Rev Cardiovasc Med 2003;4:72-80. [PubMed]
- Semenov AG, Tamm NN, Seferian KR, et al. Processing of Pro-B-Type Natriuretic Peptide Furin and Corin as Candidate Convertases. Clin Chem 2010;56:1166-76. [PubMed]
- Mueller T, Gegenhuber A, Poelz W, et al. Diagnostic accuracy of B-type natriuretic peptide and amino terminal proBNP in the emergency diagnosis of HF. Heart 2005;91:606-12. [PubMed]
- Moertl D, Berger R, Struck J, et al. Comparison of midregional pro-atrial and B-type natriuretic peptides in chronic HF: influencing factors, detection of left ventricular systolic dysfunction, and prediction of death. J Am Coll Cardiol 2009;53:1783-90. [PubMed]
- Daggubati S, Parks JR, Overton RM, et al. Adrenomedullin, endothelin, neuropeptide Y, atrial, brain, and C-natriuretic prohormone peptides compared as early HF indicators. Cardiovasc Res 1997;36:246-55. [PubMed]
- Jougasaki M, Grantham JA, Redfield MM, et al. Regulation of cardiac adrenomedullin in HF. Peptides 2001;22:1841-50. [PubMed]
- Meeran K, O’Shea D, Upton PD, et al. Circulating adrenomedullin does not regulate systemic blood pressure but increases plasma prolactin after intravenous infusion in humans: a pharmacokinetic study. J Clin Endocrinol Metab 1997;82:95-100. [PubMed]
- Cowie MR, Jourdain P, Maisel AS, et al. Clinical applications of B-type natriuretic peptide (BNP) testing. Eur Heart J 2003;24:1710-8. [PubMed]
- Maisel A, Muller C, Adams K Jr, et al. State of the art: Using natriuretic peptide levels in clinical practice. Eur J Heart Fail 2008;10:824-39. [PubMed]
- Januzzi Jr JL. The role of natriuretic peptide testing in guiding chronic heart failure management: Review of available data and recommendations for use. Archives of cardiovascular disease 2012;105:40-50.
- Leuchte HH, Holzapfel M, Baumgartner RA, et al. Clinical significance of brain natriuretic peptide in primary pulmonary hypertension. J Am Coll Cardiol 2004;43:764-70. [PubMed]
- Daniels LB, Clopton P, Bhalla V, et al. How obesity affects the cut-points for B-type natriuretic peptide in the diagnosis of acute heart failure. Results from the Breathing Not Properly Study. Am Heart J 2006;151:999-1005. [PubMed]
- Horwich TB, Hamilton MA, Fonarow GC. B-type natriuretic peptide levels in obese patients with advanced heart failure. J Am Coll Cardiol 2006;47:85-90. [PubMed]
- Maisel AS, Choudhary R. Biomarkers in acute heart failure-state of the art. Nat Rev Cardiol 2012. [Epub ahead of print].
- Maisel A, Hollander JE, Guss D, et al. Primary results of the Rapid ED HF Outpatient Trial (REDHOT). J Am Coll Cardiol 2007;49:1943-50. [PubMed]
- Di Somma S, Magrini L, Pittoni V, et al. In-hospital percentage BNP reduction is highly predictive for adverse events in patients admitted for acute HF: the Italian RED Study. Crit Care 2010;14:R116. [PubMed]
- Fonarow GC, Peacock WF, Phillips CO, et al. Admission B-type Natriuretic Peptide Levels and In-Hospital Mortality in Acute Decompensated HF. J Am Coll Cardiol 2007;49:1943-50. [PubMed]
- Masson S, Latini R, Anand IS, et al. Direct comparison of B-Type Natriuretic Peptide (BNP) and Amino-Terminal proBNP in a Large Population of Patients with Chronic and Symptomatic Heart Failure: The Valsartan Heart Failure (Val-HeFT) Data. Clin Chem 2006;52:1528-38. [PubMed]
- Doust JA, Pietrzak E, Dobson A, et al. How well does B-type natriuretic peptide predict death and cardiac events in patients with heart failure: systematic review. BMJ 2005;330:625. [PubMed]
- Nishiyama K, Tsutamoto T, Yamaji M, et al. Biological Variation of Brain Natriuretic Peptide and Cardiac Events in Stable Outpatients with Nonischemic Chronic Heart Failure. Circ J 2011;75:341-7. [PubMed]
- Di Somma S, De Berardinis B, Bongiovanni C, et al. Use of BNP and bioimpedance to drive therapy in heart failure patients. Congest Heart Fail 2010;16:S56-61. [PubMed]
- van der Burg-de Graauw N, Cobbaert CM, Middelhoff CJ, et al. The additive value of N-terminal pro-B-type natriuretic peptide testing at the ED in patients with acute dyspnea. Eur J Intern Med 2009;20:301-6. [PubMed]
- Moe GW, Howlett J, Januzzi JL, et al. N-Terminal Pro-B-Type Natriuretic Peptide Testing Improves the Management of Patients With Suspected Acute HF: Primary Results of the Canadian Prospective Randomized Multicenter IMPROVE-CHF Study. Circulation 2007;115:3103-10. [PubMed]
- Waldo SW, Beede J, Isakson S, et al. Pro-B-Type Natriuretic Peptide Levels in Acute Decompensated Heart Failure. J Am Coll Cardiol 2008;51:1874-82. [PubMed]
- Cleland JGF, Taylor J, Freemantle N, et al. Relationship between plasma concentrations of N-terminal pro brain natriuretic peptide and the characteristics and outcome of patients with a clinical diagnosis of diastolic heart failure: a report from PEP-CHF study. Eur J Heart Fail 2012;14:487-94. [PubMed]
- Betti I, Castelli G, Barchielli A, et al. The role of N-terminal PRO- brain natriuretic peptide and echocardiography for screening asymptomatic left ventricular dysfunction in a population at high risk for heart failure. The PROBE-HF study. J Card Fail 2009;15:377-84. [PubMed]
- Maisel A, Mueller C, Nowak R, et al. Mid-region pro-hormone markers for diagnosis and prognosis in acute dyspnea:results from the BACH (Biomarkers in Acute HF) trial. J Am Coll Cardiol 2010;55:2062-76. [PubMed]
- Daniels LB, Clopton P, Potocki M, et al. Influence of age, race, sex, and body mass index on interpretation of midregional pro atrial natriuretic peptide for the diagnosis of acute heartfailure: results from the BACH multinational study. Eur J Heart Fail 2012;14:22-31. [PubMed]
- Smith JG, Newton-Cheh C, Almgren P, et al. Assessment of conventional cardiovascular risk factors and multiple biomarkers for the prediction of incident HF and atrial fibrillation. J Am Coll Cardiol 2010;56:1712-9. [PubMed]
- von Haehling S, Jankowska EA, Morgenthaler NG, et al. Comparison of midregional pro-atrial natriuretic peptide with N-terminal pro-B-type natriuretic peptide in predicting survival in patients with chronic HF. J Am Coll Cardiol 2007;50:1973-80. [PubMed]
- Peacock WF, Nowak R, Christenson R, et al. Short-term Mortality Risk in ED Acute HF. Acad Emerg Med 2011;18:947-58. [PubMed]
- Klip IT, Voors AA, Anker SD, et al. Prognostic value of mid-regional pro-adrenomedullin in patients with HF after an acute myocardial infarction. Heart 2011;97:892-8. [PubMed]
- Weinberg EO, Shimpo M, De Keulenaer GW, et al. Expression and regulation of ST2, an interleukin-1 receptor family member, in cardiomyocites and myocardial infarction. Circulation 2002;106:2961-6. [PubMed]
- Iwahana H, Yanagisawa K, Ito-Kosaka A, et al. Different promoter usage and multiple transcription initiation sites of the interleukin-1 receptor related human ST2 gene in UT-7 and TMI2 cells. Eur J Biochem 1999;264:397-406. [PubMed]
- Coyle AJ, Lloyd C, Tian J, et al. Crucial role of the interleukin-1 receptor family member T1/ST2 in T Helper cell type-2 mediated lung mucosal immune responses. J Exp Med 1999;190:895-902. [PubMed]
- Sanada S, Hakuno D, Higgins LJ, et al. IL-33 and ST2 comprise a critical biomechanically induced and cardioprotective signaling system. J Clin Invest 2007;117:1538-49. [PubMed]
- Schmitz J, Owyang A, Oldham E, et al. IL-33, an interleukin-1 like cytokine that signals via the IL-1 receptor related protein ST2 and induces T helper type-2 associated cytokines. Immunity 2005;23:479-90. [PubMed]
- Sanada S, Hakuno D, Higgins LJ, et al. IL-33 and ST2 comprise a critical biomechanically induced and cardioprotective signaling system. J Clin Invest 2007;117:1538-49. [PubMed]
- Weinberg EO, Shimpo M, De Keulenaer GW, et al. Expression and regulation of ST2, an interleukin-1 receptor family member, in cardiomyocites and myocardial infarction. Circulation 2002;106:2961-6. [PubMed]
- Januzzi JL, Peacock WF, Maisel AS, et al. Measurement of the interleukin family member ST2 in patients with acute dyspnea. J Am Coll Cardiol 2007;50:607-13. [PubMed]
- Daniels LB, Maisel AS, Clopton P, et al. Association of ST2 levels with cardiac structure and function and mortality in outpatients. Am Heart J 2010;160:721-8. [PubMed]
- Shimpo M, Morrow DA, Weinberg EO, et al. Serum levels of the Interleukin-1 receptor family member ST2 predict mortality and clinical outcome in acute myocardial infarction. Circulation 2004;109:2186-90. [PubMed]
- Ago T, Sadoshima J. GDF-15, a cardioprotective TGF-B superfamily protein. AHA Journals. 2011.
- Hsiao EC, Koniaris LG, Zimmers-Koniaris T, et al. Characterization of growth-differentiation factor 15, a transforming growth factor beta superfamily member induced following liver injury. Mol Cell Biol 2000;20:3742-51. [PubMed]
- Secchiero P, Corallini F, Gonelli A, et al. Atniangiogenic activity of the MDM2 antagonist nutlin-3. Circ Res 2007;100:61-9. [PubMed]
- Bermúdez B, Lopez S, Pacheco YM, et al. Influence of postprandial triglyceride-rich lipoproteins on lipid-mediated gene expression in smooth muscle cells of the human coronary artery. Cardiovasc Res 2008;79:294-303. [PubMed]
- Ding Q, Mracek T, Gonzalez-Muniesa P, et al. Identification of macrophage inhibitory cytokine-1 in adipose tissue and its secretion as an adipokine by human adipocytes. Endocrinology 2009;150:1688-96. [PubMed]
- Kempf T, von Haehling S, Peter T, et al. Prognostic utility of growth differentiation factor-15 in patients with chronic HF. J Am Coll Cardiol 2007;50:1054-60. [PubMed]
- Moore AG, Brown DA, Fairlie WD, et al. The transforming growth factor-ss superfamily cytokine macrophage inhibitory cytokine-1 is present in high concentrations in the serum of pregnant women. J Clin Endocrinol Metab 2000;85:4781-8. [PubMed]
- Eggers KM, Kempf T, Lind L, et al. Relations of growth-differentiation factor-15 to biomarkers reflecting vascular pathologies in a population-based sample of elderly subjects. Scand J Clin Lab Invest 2012;72:45-51. [PubMed]
- Khan SQ, Ng K, Dhillon O, et al. Growth differentiation factor-15 as a prognostic marker in patients with acute myocardial infarction. Eur Heart J 2009;30:1057-65. [PubMed]
- Kempf T, Bjorklund E, Olofsson S, et al. Growth differentiation factor-15 improves risk stratification in ST-segment elevation myocardial infarction. Eur Heart J 2007;28:2858-65. [PubMed]
- Anand IS, Kempf T, Rector TS, et al. Serial measurement of growth differentiation factor-15 in HF. Circulation 2010;122:1387-95. [PubMed]
- Wang F, Guo Y, Yu H, et al. Growth differentiation factor 15 in different stages of HF: potential screening implications. Biomarkers 2010;15:671-6. [PubMed]
- Stahrenberg R, Edelmann F, Mende M, et al. The novel biomarker gowth differentiation factor 15 in HF with normal ejection fraction. Eur J Heart Fail 2010;12:1309-16. [PubMed]
- Dinh W, Futh R, Lankisch M, et al. Growth-differentiation factor-15: a novel biomarker in patients with diastolic dysfunction? Sociedade Brasileira de Cardologia. 2011.
- McCullough PA, Olobatoke A, Vanhecke TE. Galectin-3: a novel blood test for the evaluation and management of patients with HF. Rev Cardiovasc Med 2011;12:200-10. [PubMed]
- Grandin EW, Jarolim P, Murphy SA, et al. Galectin-3 and the development of HF after acute coronary syndrome: pilot experience from PROVE IT-TIMI 22. Clin Chem 2012;58:267-73. [PubMed]
- de Boer RA, Lok DJ, Jaarsma T, et al. Predictive value of plasma galectin-3 levels in HF with reduced and preserved ejection fraction. Ann Med 2011;43:60-8. [PubMed]
- Lok DJ, Van Der Meer P, de la Porte PW, et al. Prognostic value of galectin-3, a novel marker of fibrosis, in patients with chronic HF: data from the DEAL-HF study. Clin Res Cardiol 2010;99:323-328. [PubMed]
- van Kimmenade RR, Januzzi JL Jr, Ellinor PT, et al. Utility of amino-terminal pro-brain natriuretic peptide, galectin-3, and apelin for the evaluation of patients with acute HF. J Am Coll Cardiol 2006;48:1217-24. [PubMed]
- Daubert MA, Jeremias A. The utility of troponin measurement to detect myocardial infarction: review of the current findings. Vasc Health Risk Manag 2010;6:691-9. [PubMed]
- Tsutamoto T, Kawahara MD, Yamaji M, et al. Prognostic role highly sensitive cardiac troponin I in patients with systolic HF. Am Heart J 2010;159:63-7. [PubMed]
- Xue Y, Clopton P, Peacock W, et al. Serial changes in high-sensitive troponin I predict outcome in patients with decompensated HF. Eur J Heart Fail 2011;13:37-42. [PubMed]
- Masson S, Anand I, Favero C, et al. Serial Measurement of Cardiac Troponin T Using a Highly Sensitive Assay in Patients With Chronic HF. Circulation 2012;125:280-8. [PubMed]
- Miller WL, Hartman KA, Burritt MF, et al. Profiles of serial changes in cardiac troponin T concentrations and outcome in ambulatory patients with chronic heart failure. J Am Coll Cardiol 2009;54:1715-21. [PubMed]
- Latini R, Masson S, Anand IS, et al. Prognostic value of very low plasma concentrations of troponin T in patients with stable chronic heart failure. Circulation 2007;116:1242-9. [PubMed]
- Tsutamoto T, Kawahara C, Nishiyama K, et al. Prognostic role of highly sensitive cardiac troponin I in patients with systolic heart failure. Am Heart J 2010;159:63-7. [PubMed]
- Pascual-Figal DA, Manzano-Fernandez S, Boronat M, et al. Soluble ST2, high-sensitivity troponin T-and N-terminal pro-B-type natriuretic peptide: compementary role for risk stratification in acutely decompensated HF. Eur J Heart Fail 2011;13:718-25. [PubMed]
- Cowland JB, Borregaard N. Molecular characterization and pattern of tissue expression of the gene for neutrophil gelatinase-associated lipocalin from humans. Genomics 1997;45:17-23. [PubMed]
- Friedl A, Stoesz SP, Buckley P, et al. Neutrophil gelatinase-associated lipocalin in normal and neoplastic human tissues. Cell type-specific pattern of expression. Histochem J 1999;31:433-41. [PubMed]
- Soni SS, Cruz D, Bobek I, et al. NGAL: a biomarker of acute kidney injury and other systemic conditions. Int Urol Nephrol 2010;42:141-50. [PubMed]
- Gwira JA, Wei F, Ishibe S, et al. Expression of neutrophil gelatinase-associated lipocalin regulates epithelial morphogenesis in vitro. J Biol Chem 2005;280:7875-82. [PubMed]
- Mori K, Lee HT, Rapoport D, et al. Endocytic delivery of lipocalin-siderophore-iron complex rescues the kidney from ischemia-reperfusion injury. J Clin Invest 2005;115:610-21. [PubMed]
- Goetz DH, Holmes MA, Borregaard N, et al. The neutrophil lipocalin NGAL is a bacteriostatic agent that interferes with siderophore-mediated iron acquisition. Mol Cell 2002;10:1033-43. [PubMed]
- Devarajan P. NGAL in acute kidney injury: from serendipity to utility. Am J Kidney Dis 2008;52:395-9. [PubMed]
- Yndestad A, Landro L, Ueland T, et al. Increased systemic and myocardial expression of neutrophil gelatinase-associated lipocalin in clinical and experimental HF. Eur Heart J 2009;30:1229-36. [PubMed]
- Aghel A, Shrestha K, Mullens W, et al. Serum neutrophil gelatinase-associated lipocalin (NGAL) in predicting worsening renal function in acute decompensated HF. J Card Fail 2010;16:49-54. [PubMed]
- Alvelos M, Pimentel R, Pinho E, et al. Neutrophil Gelatinase-Associated Lipocalin in the Diagnosirs of Type 1 Cardio-Renal Syndrome in the General Ward. Clin J Am Soc Nephrol 2011;6:476-81. [PubMed]
- Damman K, van Veldhuisen DJ, Navis G, et al. Urinary neutrophil gelatinase associated lipocalin (NGAL), a marker of tubular damage, is increased in patients with chronic HF. Eur J Heart Fail 2008;10:997-1000. [PubMed]
- Poniatowski B, Malyszko J, Bachorzewska-Gajewska H, et al. Serum neutrophil gelatinase-associated lipocalin as a marker of renal function in patients with chronic HF and coronary artery disease. Kidney Blood Press Res 2009;32:77-80. [PubMed]
- Bolignano D, Basile G, Parisi P, et al. Increased plasma neutrophil gelatinase-associated lipocalin levels predict mortality in elderly patients with chronic HF. Rejuvenation Res 2009;12:7-14. [PubMed]
- Damman K, Masson S, Hillege HL, et al. Clinical outcome of renal tubular damage in chronic HF. Eur Heart J 2011;32:2705-12. [PubMed]
- Dickstein K, Kjekshus J. Effects of losartan and captopril on mortality and morbidity in high-risk patients after acute myocardial infarction: the OPTIMAAL randomised trial. Optimal Trial in Myocardial Infarction with Angiotensin II Antagonist Losartan. Lancet 2002;360:752-60. [PubMed]
- Maisel AS, Mueller C, Fitzgerald R, et al. Prognostic utility of plasma neutrophil gelatinase-associated lipocalin in patients with acute HF: the NGAL EvaLuation Along with B-type NaTriuretic Peptide in acutely decompensated HF (GALLANT) trial. Eur J Heart Fail 2011;13:846-51. [PubMed]
- Macfarlane JT, Colville A, Guion A, et al. Prospective study of aetiology and outcome of adult lower-respiratory-tract infections in the community. Lancet 1993;341:511-4. [PubMed]
- Iversen KK, Kjaergaard J, Akkan D, et al. The prognostic importance of lung function in patients admitted with HF. Eur J Heart Fail 2010;12:685-91. [PubMed]
- Sandek A, Springer J, Habedank D, et al. Procalcitonin-guided antibiotic treatment in HF. Lancet 2004;363:1555-author reply 1555-6. [PubMed]
- Christ-Crain M, Jaccard-Stolz D, Bingisser R, et al. Effect of procalcitonin-guided treatment on antibiotic use and outcome in lower respiratory tract infections: cluster-randomised, single-blinded intervention trial. Lancet 2004;363:600-7. [PubMed]
- Maisel A, Neath SX, Landsberg J, et al. Use of procalcitonin for the diagnosis of pneumonia in patients presenting with a chief complaint of dyspnoea: results from the BACH (Biomarkers in Acute HF) trial. Eur J Heart Fail 2012;14:278-86. [PubMed]
- Hirano T, Yasukawa K, Harada H, et al. Complementary DNA for a novel human interleukin (BSF-2) that induces B lymphocytes to produce immunoglobulin. Nature 1986;324:73-6. [PubMed]
- Lotz M, Jirik F, Kabouridis P, et al. B cell stimulating factor 2/interleukin 6 is a costimulant for human thymocytes and T lymphocytes. J Exp Med 1988;167:1253-8. [PubMed]
- Mulé JJ, McIntosh JK, Joblons DM, et al. Antitumor activity of recombinant interleukin-6 in mice. J Exp Med 1990;171:629-36. [PubMed]
- Gauldie J, Richards C, Harnich D, et al. Interferon s2/BSF-2 shares identity with monocyte- derived hepatocyte stimulating factor (HSF) and regulates the major acute phase protein response in liver cells. Proc Natl Acad Sci USA 1987;84:7251-5. [PubMed]
- Luger TA, Krutmann J, Kirnbaner R, et al. IFN-s2/IL-6 augments the activity of human natural killer cells. J Immunol 1989;143:1206-9. [PubMed]
- Mesri M, Altieri DC. Endothelial cell activation by leukocyte microparticles. J Immunol 1998;161:4382-7. [PubMed]
- Loppnow H, Libby P. Proliferating or interleukin 1-activated human vascular smooth muscle cells secrete copious interleukin 6. J Clin Invest 1990;85:731-8. [PubMed]
- Gwechenberger M, Mendoza LH, Youker KA, et al. Cardiac myocytes produce interleukin-6 in culture and inviable border zone of reperfused infarctions. Circulation 1999;99:546-51. [PubMed]
- Kaneko K, Kanda T, Yokoyama T, et al. Expression of interleukin-6 in the ventricles and coronary arteries of patients with myocardial infarction. Res Commun Mol Pathol Pharmacol 1997;97:3-12. [PubMed]
- Raymond RJ, Dehmer GJ, Theoharides TC, et al. Elevated interleukin-6 levels in patients with asymptomatic left ventricular systolic dysfunction. Am Heart J 2001;141:435-8. [PubMed]
- Tsutamoto T, Hisanaga T, Wada A, et al. Interleukin-6 spillover in the peripheral circulation increases with the severity of heart failure, and the high plasma level of interleukin-6 is an important prognostic predictor in patients with congestive heart failure. J Am Coll Cardiol 1998;31:391-8. [PubMed]
- Birks EJ, Yacoub MH. The role of nitric oxide and cytokines in heart failure. Coron Artery Dis 1997;8:389-402. [PubMed]
- Kubota T, Miyagishima M, Alvarez RJ, et al. Expression of proinflammatory cytokines in the failing human heart: comparison of recent-onset and end-stage congestive heart failure. J Heart Lung Transplant 2000;19:819-24. [PubMed]
- Pudil R, Tichy M, Andrys C, et al. Plasma interleukin-6 is associated with NT-proBNP level and predict short and long term mortality in patients with acute heart failure. Acta Med (Hradec Kralove) 2010;53:225-8.
- Maeda K, Tsutamoto T, Wada A, et al. High levels of plasma brain natriuretic peptide and interleukin-6 after optimized treatment for heart failure are independent risk factors for morbidity and mortality in patients with congestive heart failure. J Am Coll Cardiol 2000;36:1587-93. [PubMed]
- Itoh N, Yonehara S, Ishii A, et al. The polypeptide encoded by the cDNA for human cell surface antigen Fas can mediate apoptosis. Cell 1991;66:233-43. [PubMed]
- Oehm A, Behrmann I, Falk W, et al. Purification and molecular cloning of the Apo-1 cell surface antigen, a member of the tumor necrosis factor/nerve growth receptor superfamily. J Biol Chem 1992;267:10709-15. [PubMed]
- Tanaka M, Itoh H, Adachi S, et al. Hypoxia induces apoptosis with enhanced expression of Fas antigen messenger RNA in cultured neonatal rat cardiomyocytes. Circ Res 1994;75:426-33. [PubMed]
- Cheng W, Li B, Kajstura J, et al. Stretch induced programmed myocyte cell death. L clin Invest 1995; 96:2247-59.
- Cheng J, Zhou T, Liu C, et al. Protection from Fas mediated apoptosis by a soluble form of the Fas molecule. Science 1994;263:1759-62. [PubMed]
- Okuyama M, Yamaguchi S, Nozaki N, et al. Serum levels of soluble form of Fas molecule in patients with congestive heart failure. Am J Cardiol 1997;79:1698-701. [PubMed]
- Tsutamoto T, Wada A, Maeda K, et al. Relationship between plasma levels of cardiac natriuretic peptides and soluble Fas: Plasma soluble Fas as a prognostic predictor in patients with congestive heart failure. J Card Fail 2001;7:322-8. [PubMed]
- Levine B, Kalman J, Mayer L, et al. Elevated circulating levels of tumor necrosis factor in severe chronic heart failure. N Engl J Med 1990;323:236-41. [PubMed]
- Kleinbongard P, Schulz R, Heusch G. TNFα in myocardial ischemia/reperfusion, remodeling and heart failure. Heart Fail Rev 2011;16:49-69. [PubMed]
- Deswal A, Petersen NJ, Feldman AM, et al. Cytokines and cytokine receptors in advanced heart failure: an analysis of the cytokine database from the Vesnarinone trial (VEST). Circulation 2001;103:2055-9. [PubMed]
- Chrysohoou C, Pitsavos C, Barbetseas J, et al. Chronic systemic inflammation accompanies impaired ventricular diastolic function, detected by Doppler imaging, in patients with newly diagnosed systolic heart failure (Hellenic Heart Failure Study). Heart Vessels 2009;24:22-6. [PubMed]
- Valgimigli M, Ceconi C, Malagutti P, et al. Tumor necrosis factor-alpha receptor 1 is a major predictor of mortality and new-onset heart failure in patients with acute myocardial infarction: the Cytokine-Activation and Long-Term Prognosis in Myocardial Infarction (C-ALPHA) study. Circulation 2005;111:863-70. [PubMed]
- Berton G, Cordiano R, Palmieri R, et al. C-reactive protein in acute myocardial infarction: association with heart failure. Am Heart J 2003;145:1094-101. [PubMed]
- Yin WH, Chen JW, Jen HL, et al. Independent prognostic value of elevated high-sensitivity C-reactive protein in chronic heart failure. Am Heart J 2004;147:931-8. [PubMed]
- Chirinos JA, Zambrano JP, Chakko S, et al. Usefulness of C-reactive protein as an independent predictor of death in patients with ischemic cardiomyopathy. Am J Cardiol 2005;95:88-90. [PubMed]
- Cesari M, Penninx BW, Newman AB, et al. Inflammatory markers and onset of cardiovascular events: results from the Health ABC study. Circulation 2003;108:2317-22. [PubMed]
- Boekholdt SM, Hack CE, Sandhu MS, et al. C-reactive protein levels and coronary artery disease incidence and mortality in apparently healthy men and women: the EPIC-Norfolk prospective population study 1993-2003. Atherosclerosis 2006;187:415-22. [PubMed]
- Engström G, Melander O, Hedblad B. Carotid intima-media thickness, systemic inflammation, and incidence of heart failure hospitalizations. Arterioscler Thromb Vasc Biol 2009;29:1691-5. [PubMed]
- Oikonomou E, Tousoulis D, Siasos G, et al. The role of inflammation in heart failure: new therapeutic approaches. Hellenic J Cardiol 2011;52:30-40. [PubMed]
- Dubin R, Li Y, Ix JH, et al. Associations of pentraxin-3 with cardiovascular events, incident heart failure, and mortality among persons with coronary heart disease: data from the Heart and Soul Study. Am Heart J 2012;163:274-9. [PubMed]
- Suzuki S, Takeishi Y, Niizeki T, et al. Pentraxin 3, a new marker for vascular inflammation, predicts adverse clinical outcomes in patients with heart failure. Am Heart J 2008;155:75-81. [PubMed]
- Kotooka N, Inoue T, Aoki S, et al. Prognostic value of pentraxin 3 in patients with chronic heart failure. Int J Cardiol 2008;130:19-22. [PubMed]
- Matsubara J, Sugiyama S, Nozaki T, et al. Pentraxin 3 is a new inflammatory marker correlated with left ventricular diastolic dysfunction and heart failure with normal ejection fraction. J Am Coll Cardiol 2011;57:861-9. [PubMed]
- Vita JA, Brennan ML, Gokce N. Serum myeloperoxidase levels independently predict endothelial dysfunction in humans. Circulation 2004;110:1134-9. [PubMed]
- Tang WH, Katz R, Brennan ML. Usefulness of myeloperoxidase levels in healthy elderly subjects to predict risk of developing heart failure. Am J Cardiol 2009;103:1269-74. [PubMed]
- Reichlin T, Socrates T, Egli P. Use of myeloperoxidase for risk stratification in acute heart failure. Clin Chem 2010;56:944-51. [PubMed]
- Brennan ML, Penn MS, Van Lente F, et al. Prognostic value of myeloperoxidase in patients with chest pain. N Engl J Med 2003;349:1595-604. [PubMed]
- Cavusoglu E, Ruwende C, Eng C, et al. Usefulness of baseline plasma myeloperoxidase levels as an independent predictor of myocardial infarction at two years in patients presenting with acute coronary syndrome. Am J Cardiol 2007;99:1364-8. [PubMed]
- Baldus S, Heeschen C, Meinertz T, et al. Myeloperoxidase serum levels predict risk in patients with acute coronary syndromes. Circulation 2003;108:1440-5. [PubMed]
- Tang WH, Brennan ML, Philip K, et al. Plasma myeloperoxidase levels in patients with chronic heart failure. Am J Cardiol 2006;98:796-9. [PubMed]
- Michowitz Y, Kisil S, Guzner-Gur H, et al. Usefulness of serum myeloperoxidase in prediction of mortality in patients with severe heart failure. Isr Med Assoc J 2008;10:884-8. [PubMed]
- Tang WH, Tong W, Troughton RW, et al. Prognostic value and echocardiographic determinants of plasma myeloperoxidase levels in chronic heart failure. J Am Coll Cardiol 2007;49:2364-70. [PubMed]
- Cohn JN, Levine TB, Olivari MT, et al. Plasma norepinephrine as a guide to prognosis in patients with chronic congestive heart failure. N Engl J Med 1984;311:819-23. [PubMed]
- Packer M. The neurohormonal hypothesis: a theory to explain the mechanism of disease progression in heart failure. J Am Coll Cardiol 1992;20:248-54. [PubMed]
- Swedberg K, Eneroth P, Kjekshus J, et al. Hormones regulating cardiovascular function in patients with severe congestive heart failure and their relation to mortality. CONSENSUS Trial Study Group. Circulation 1990;82:1730-6. [PubMed]
- Francis GS. Neurohormonal control of heart failure. Cleve Clin J Med 2011;78:S75-9. [PubMed]
- Latini R, Masson S, Anand IVal-HeFT Investigators, et al. The comparative prognostic value of plasma neurohormones at baseline in patients with heart failure enrolled in Val-HeFT. Eur Heart J 2004;25:292-9. [PubMed]
- Givertz MM, Braunwald E. Neurohormones in heart failure: predicting outcomes, optimizing care. Eur Heart J 2004;25:281-2. [PubMed]
- Milo-Cotter O, Cotter-Davison B, Lombardi C, et al. Neurohormonal activation in acute heart failure: results from VERITAS. Cardiology 2011;119:96-105. [PubMed]
- Vergaro G, Emdin M, Iervasi A, et al. Prognostic value of plasma renin activity in heart failure. Am J Cardiol 2011;108:246-51. [PubMed]
- Hülsmann M, Stanek B, Frey B, et al. Value of cardiopulmonary exercise testing and big endothelin plasma levels to predict short-term prognosis of patients with chronic heart failure. J Am Coll Cardiol 1998;32:1695-700. [PubMed]
- Braunwald E. Biomarkers in heart failure. N Engl J Med 2008;358:2148-59. [PubMed]
- Sabatine MS, Morrow DA, de Lemos JA, et al. Evaluation of multiple biomarkers of cardiovascular stress for risk prediction and guiding medical therapy in patients with stable coronary disease. Circulation 2012;125:233-40. [PubMed]
- Jankowska EA, Filippatos GS, von Haehling S, et al. Identification of chronic heart failure patients with a high 12-month mortality risk using biomarkers including plasma C-terminal pro-endothelin-1. PLoS ONE 2011;6:e14506. [PubMed]
- Teerlink JR. The role of endothelin in the pathogenesis of heart failure. Curr Cardiol Rep 2002;4:206-12. [PubMed]
- Goldsmith SR. The role of vasopressin in congestive heart failure. Cleve Clin J Med 2006;73:S19-23. [PubMed]
- Morgenthaler NG. Copeptin: a biomarker of cardiovascular and renal function. Congest Heart Fail 2010;16:S37-44. [PubMed]
- Maisel A, Xue Y, Shah K, et al. Increased 90-day mortality in patients with acute heart failure with elevated copeptin: secondary results from the Biomarkers in Acute Heart Failure (BACH) study. Circ Heart Fail 2011;4:613-20. [PubMed]
- Balling L, Kistorp C, Schou M, et al. Plasma copeptin levels and prediction of outcome in heart failure outpatients: relation to hyponatremia and loop diuretic doses. J Card Fail 2012;18:351-8. [PubMed]
- Neuhold S, Huelsmann M, Strunk G, et al. Comparison of copeptin, B-type natriuretic peptide, and amino-terminal pro-B-type natriuretic peptide in patients with chronic heart failure: prediction of death at different stages of the disease. J Am Coll Cardiol 2008;52:266-72. [PubMed]
- Spinale FG. Myocardial matrix remodeling and the matrix metalloproteinases: influence on cardiac form and function. Physiol Rev 2007;87:1285-342. [PubMed]
- Zile MR, Desantis SM, Baicu CF, et al. Plasma biomarkers that reflect determinants of matrix composition identify the presence of left ventricular hypertrophy and diastolic heart failure. Circ Heart Fail 2011;4:246-56. [PubMed]
- Martos R, Baugh J, Ledwidge M, et al. Diagnosis of heart failure with preserved ejection fraction: improved accuracy with the use of markers of collagen turnover. Eur J Heart Fail 2009;11:191-7. [PubMed]
- Vorovich EE, Chuai S, Li M, et al. Comparison of matrix metalloproteinase 9 and brain natriuretic peptide as clinical biomarkers in chronic heart failure. Am Heart J 2008;155:992-7. [PubMed]
- Frantz S, Störk S, Michels K, et al. Tissue inhibitor of metalloproteinases levels in patients with chronic heart failure: an independent predictor of mortality. Eur J Heart Fail 2008;10:388-95. [PubMed]