Prediction of cardiovascular outcomes by imaging coronary atherosclerosis
Identification of specific findings has more impact on clinical decision-making when they are closely associated with prognosis or outcomes. As detailed reviews on various invasive and non-invasive imaging modalities for coronary atherosclerosis have been reported in this issue of the journal (1,2), this article will focus on their prognostic value. The main outcomes can be divided into long-term outcomes, such subsequent major adverse cardiovascular event (MACE); and more immediate peri-procedural outcomes such as distal embolization, peri-procedural myocardial infraction and no reflow.
Predicting outcomes
In general, study outcomes should be clinically relevant, easily ascertainable, sensitive to the effects under evaluation (3). Thus, the outcome measure must be defined carefully, measured accurately, and ascertained completely to provide a reliable basis for evaluation of any risk assessment tool (4). The subsequent discussion about imaging modalities and their role in prediction of cardiovascular outcomes is heavily influenced by several factors including: pre-test probability (e.g., patient populations or characteristics), study design (duration of follow-up in cohort study, e.g., 30-day, years or a decade), definition of outcome [and the distribution of each component of outcomes if composite endpoint (e.g., MACE) is used], as well as the technology under investigation.
Pre-test probability
The goal of performing diagnostic tests, either invasive or non-invasive, is to utilize the test results to guide appropriate management and to improve clinical consequences (4). Before discussing diagnostic accuracy or prognostic value in detail, it is very useful and sometimes inevitable to incorporate pre-test probability in order to enable sound post-imaging risk estimation. There are some scenarios where a decision can be made without performing any tests (e.g., smoking cessation recommendation for smokers). Use of appropriate tests requires balancing risks and benefits. For a coronary artery calcium (CAC) score between 101 and 300, post-test risk of observed cardiac death or non-fatal myocardial infarction (MI) varies from 0% to 13.2% based on Framingham risk score (FRS) (5). Importantly, a CAC of zero with high FRS (≥21) indicated greater risk than a low FRS [0−9] with any other levels of CAC. Reflecting this, non-invasive imaging modalities are most utilized in low-intermediate risk population, and invasive techniques in high risk (6).
Outcome measure, sample size and follow-up measure
Clinicians should pay extra caution when interpreting or utilizing outcome studies, not only because they are important but also because of the following potential pitfalls. The definition of study endpoints is variable and consequently must inform the analysis of any outcome data. Study endpoints should be clinically relevant, easily ascertainable, sensitive to the effects under evaluation, and verifiable (3). Hard endpoints, like all-cause death, is often preferable but this also has limitations. Imaging findings associated with all-cause death in the elderly often reflect different underlying bio-physiological signals from those seen in the young. Physician-influenced outcomes (i.e., soft endpoints) are significantly limited by the propensity of bias towards to treatment or positive findings. Composite endpoints like MACE are getting more and more popular. Although they are often relevant, they are not perfect because of numerous limitations. In a computed tomography coronary angiography (CTCA) study, the composite endpoint of cardiac death, MI, unstable angina, and revascularization was used (7). Revascularizations dominated the outcome, 24 out of 33 events (73%), CTCA therefore actually predicted revascularization. Thus, it can be misleading when a study reports composite endpoints dominated by a single component of the composite definition. Care must be taken when inferring the predictive ability of other endpoints.
The common outcomes reported in the coronary atherosclerosis are summarized in Tables 1 and 2, which illustrate significant heterogeneity in the definition of outcomes, not only the variety in definitions of MACE but the inclusion or exclusion of all-cause mortality and unstable angina in various trials. There is also further fragmentation of definitions with some trials including only angina requiring hospitalization vs. requiring intervention vs. all angina. Clearly the presence of “stenosis >50%” on angiography does not carry the same weight as “cardiac death”. Given predictive value of a test is clearly influenced by the outcome measured it should serve as a caution pertaining to the subsequent discussion and indeed in any assessment of outcome measures.
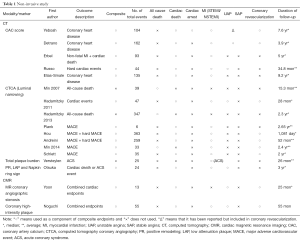
Full table
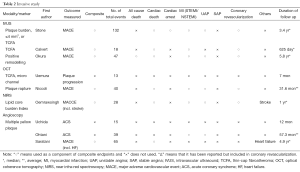
Full table
A clinical trial should be designed based on a sound rationale with a proper outcome measure as well as an appropriate sample size calculation. The sample size should be minimally required number of participants but large enough to have a high probability (power) of detecting a true effect of a given magnitude, should it exist. The protocol should generally include the following: the outcome, the values assumed for the outcome in each study group, the statistical test, alpha (type 1 error), power and the calculated sample size per group-both assuming no loss of data and, if relevant, after any inflation for anticipated missing data (8).
The follow-up duration in a clinical study closely parallels the era when a modality becomes available for widespread-use. Table 1 clearly illustrates how CAC/CA score, CTCA, Plaque assessment and cMRA all have progressively lower/shorter durations of follow-up. As such any differences in event rates need to be interpreted in light of this follow-up period. From an invasive perspective, this difference is mirrored in the paucity of predictive supporting OCT compared to IVUS (Table 2).
Imaging modalities for coronary atheroma
Identification of the “vulnerable plaque” can be achieved both invasively and non-invasively. Choice of modality depends on the target patient population and outcomes to be measured. The non-invasive modalities to visualize atherosclerosis are CT coronary angiography (CTCA)/CAC score and magnetic resonance imaging (MRI). Though applicable to both primary and secondary prevention, the non-invasive modalities are best suited to a low-to-intermediate risk population, often in primary prevention setting, to facilitate further risk stratification and identify those who may benefit from invasive treatment in addition to medical management. Whereas, the secondary prevention group or those requiring coronary angiography present an opportunity to study the atherosclerotic burden and vulnerability of plaques invasively. Intravascular ultrasound (IVUS), optical coherence tomography (OCT), near infra-red spectroscopy (NIRS), angioscopy, thermography and intravascular MRI have all been studied in the assessment of vulnerable plaques. The aim of all above modalities is to identify the “vulnerable plaque”.
The imaging modality used to investigate atherosclerosis may be only adequate to assess disease at certain locations in the coronary tree (e.g., cMRA-proximal and mid artery, IVUS/OCT-detailed assessment of individual lesions) and at a certain time during the evolution of atherosclerosis (CAC/CTCA in subclinical/asymptomatic patients). They may also provide total atherosclerotic disease burden (CAC, total plaque volume). Clearly each of these strengths and limitations will impact on the predictive ability.
Non-invasive modalities
Computed tomography (CT)
The association with radiological detection of calcium and atherosclerosis has been described in the early ‘70s (9). In 1990, Agatston created the score using calcified coronary lesions with more than 130 Hounsfield units (HU) intensity and an area over 1 mm2, which became the standard approach for measuring CAC (10). The radiation dose associated with a CAC score is comparable to that of mammography at less than 1 mSv (11) and newer methods of iterative reconstruction have resulted in even lower radiation exposure.
Coronary artery calcium (CAC)
Prediction of outcomes using new tools involves more than association, discrimination, and calibration. Consequently current body of literature also emphasizes the importance of reclassification, such as net reclassification improvement (NRI) and integrated discrimination improvement (IDI). Analyzing the Multi-Ethnic Study of Atherosclerosis (MESA) cohort, Yeboah demonstrated that CAC provided superior discrimination and risk reclassification over FRS, ankle-brachial index, high-sensitivity CRP and family history, though all were independent predictors of cardiovascular disease in intermediate risk individuals. Hecht and Narula summarized three studies (MESA, Heinz Nixdof, and Rotterdam) (12-14) and found an NRI of 19−25% (15) (Table 3). Hecht has also surmised from multiple studies that a CAC of 0 is associated with a 10-year event of 1.1−1.7%. There is an incremental risk with increasing CAC score of: 1−100 (2.3−5.9%), 101−400 (12.8−16.4%), >400 (22.5−28.6%) and >1,000 (37%) (16). Valenti has shown that in low to intermediate risk individuals, a CAC scores of 0 confers a 15-year warranty of <1% annual mortality (17). In summary, the CAC primarily serves as a risk stratification tool for primary prevention cohorts to provide incremental prognostic value and once evidence catches up- influence management decisions.
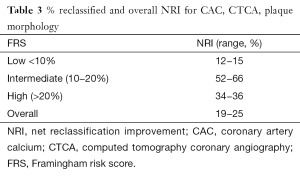
Full table
Computed tomography coronary angiography (CTCA)
Despite the robust prognostic information provided by the CAC, CT coronary angiography (CTCA) permits assessment of the coronary lumen and also enables visualization and characterization (qualitative and quantitative) of atherosclerotic plaque, incorporating calcified or low attenuation structures outside the lumen as part of the plaque. Based on these characteristics, plaques are classified as calcified, mixed and non-calcified/soft plaques. Min has demonstrated the prognostic value of CTCA in patients with chest pain, utilizing disease extent, location and distribution. Hadamitzky initially demonstrated the significantly higher event rate in patients with obstructive CAD to those without obstruction [2.9%/year (95% CI, 2.1−4.0) vs. 0.3%/year (95% CI, 6.7−27.2), P<0.001], providing predictive value over and above conventional risk scores and calcium scoring (18). A recent study using the CONFIRM registry showed that the best prognostic markers were number of proximal segments with mixed or calcified plaques (c-index 0.64, P<0.0001) and the number of proximal segments with stenosis >50% (c-index 0.56, P=0.002) (19). Hulten meta-analyzed 18 studies of symptomatic patients with chest pain and suspected coronary artery disease (CAD), and found with a negative CTCA, the negative likely hood ratio was 0.008 for MACE (95% CI, 0.0004−0.17, P<0.001). Compared to a normal CTCA, any vessel with >50% luminal stenosis had an annualized event rate of 8.8% for MACE and 3.2% for death or MI (20). Plank et al. have studied the asymptomatic high risk population using both CAC and CTCA. They utilized a coronary segment involvement score (SIS) and found that SIS ≥5 had a hazard ratio (HR) of 6.5 (95% CI, 1.6−25.8; P=0.013) for MACE. However, of the 711 patients studied, 300 had a CAC of zero but still 32% of these had non calcified plaque. The presence of a non-calcified sis (ncSIS) >1 was associated with HR of 2.4 (95% CI, 1.2−4.6, P<0.01) for the secondary composite endpoint (revascularization or invasive stenosis >50%).
This ability of CTCA to visualize not only calcific but soft plaque and determine luminal narrowing possesses incremental prognostic value. Hou et al. used CAC, CTCA and plaque characteristics in a study of 5007 patients, of whom 363 developed MACE. They found the cumulative probability of 3-year MACE increased across CT strata for CACS, CTCA and plaque characteristics as shown in Table 4. The areas under the ROC curves were 0.72 using clinical markers, 0.82 (P<0.001) with the addition of CAC and 0.93 (P<0.001) with CTCA (21).
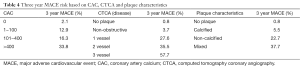
Full table
The evaluation of CTCA for long-term prognosis (52±22 months) was studied by Andreini, demonstrating cumulative event-free survival of 100% for patients with normal coronary arteries, 88% for hard events in patients with non-obstructive CAD, and 54% in patients with obstructive CAD. The long term follow of the ROMICAT cohort (emergency presentations) demonstrated prognosis across CTCA pathology with MACE of 0%, 4.6% and 30.3% with a normal CTCA, non-obstructive and obstructive coronary disease, respectively (P<0.0001) (22). Min demonstrated incremental value of CTCA over CAC in a 400 asymptomatic diabetic patients. After adjusting for clinical risk factors and CAC the severity of stenosis, number of stenosis and segment stenosis score provided by CTCA added prognostic value (23). Similar incremental prognostic value was also demonstrated by Russo (24).
Vulnerable plaque identification by CTCA
The sub-millimeter spatial resolution of CTCA enables the assessment of plaque characteristics including identification of the “vulnerable plaque”. Firstly, plaque volume has been shown to be an independent predictor of acute coronary syndrome (ACS). Unlike the above trials, a study of 1,650 patients found no difference among CAC score, lesion severity score and segment stenosis score when predicting ACS. However, it did demonstrate ACS patients had higher total plaque volume (median: 94 vs. 29 mm3) and total non-calcified volume (28 vs. 4 mm3, P<0.001) using a semi-automated technique. The addition of semi-automated plaque quantification improved the area under the ROC curve from 0.64 (FRS and CTCA) to 0.79 (P<0.05) (25). Further work by Motoyama and colleagues targeted positive remodeling (PR) (defined as diameter of plaque 10% larger than reference vessel) and low attenuation plaque (LAP) (less than 30 HU) as potential predictors of ACS. Patients with both factors had a 22.2% chance of developing ACS vs. 3.7% for only one of the above and 0.5% chance if a plaque had none of the above features. PR and/or LAP independently predicted acute coronary events HR 22.8 (95% CI, 6.9−75.2; P<0.001). As seen in the previous study plaque volume, LAP volume and ratio of it to total plaque volume were all associated with ACS. Previous work by the group had also identified spotty calcification to be associated with ACS however this was not reproduced in the present study (26).
The Napkin ring sign describes a LAP surrounded by a rim of higher attenuation. Otsuka studied the CT features of a vulnerable plaque in 895 patients and found that the HR on a segment based analysis for predicting ACS was 5.55 (2.10−14.7, P<0.001) for the Napkin ring sign, 3.75 (1.43−9.79, P=0.007) for LAP, 5.25 (2.12−12.69, P<0.001) for PR (27). These high risk features are shown in Figure 1. Motoyama recently demonstrated the incremental value of the high risk plaque in 4,423 patients with known or suspected CAD. A multivariate regression model demonstrated a HR of 8.24 (95% CI, 5.26−12.96, P<0.0001) for high-risk plaque and 1.61 (1.01−2.53, P=0.044) for severe stenosis (>70%) for predicting development of ACS (28).
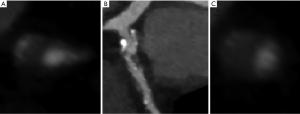
In addition to the morphological characteristics discussed above there is growing interest regarding the functional assessment of a coronary plaque, There has been a fundamental shift from anatomic to physiology guided revascularization heralded by the FAME trials (29). The FFR may also reflect not only distal ischemic burden but hemodynamic venerability of the atherosclerotic plaque. CT FFR was compared to invasive FFR and had a per-vessel accuracy of 84.3%, sensitivity of 87.9%, and specificity of 82.2% in the discover FLOW trial (30). The DEFACTO trial revealed that FFR CT better discriminates coronary stenosis with Ischemia AUC 0.81 (95% CI, 0.75−0.86; P<0.0001) compared with CT alone AUC 0.68 (0.62−0.74) (31). Finally modelling the coronary flow dynamics with CT FFR can be used to predict response of a coronary tree after virtual stent implantation (32).
In summary, the strength of CTCA is a result of the ability to document significant stenosis and characterize high risk plaque, there is incremental prognostic value over and above a CAC though the magnitude of this benefit varies across studies. Currently, a CTCA should be reserved for low to intermediate risk symptomatic patients for whom coronary angiography is not deemed appropriate. Its value in the primary prevention population in the face of overwhelming prognostic data for the CAC score would be in the younger high risk cohort as CAC is a function of age and gender (33). Importantly as radiation doses for CTCA are minimized an ultra-low radiation complete scan may be the standard of care in the future.
Cardiac magnetic resonance imaging (CMR)
Visualization of coronary atherosclerosis using CMR is desirable given the lack of ionizing radiation and contrast, because of an intrinsically high blood signal intensity (34). It can also present a unique opportunity to longitudinally follow up plaque burden. For proximal vessels, CMR and CT have shown similar diagnostic accuracy for detecting coronary stenosis >50% (35). Further validation with invasive angiography by Yang showed that on a per-person basis the sensitivity, specificity and accuracy were 94.1% 82.1% and 88.7% (36). Kato demonstrated the high sensitivity (88%) of MRA against the gold standard of coronary angiography in a multicenter study of 138 patients (37). Despite validation against invasive and non-invasive modalities, CMR has been mainly used as a functional tool rather than an anatomical tool. Reasons include the lower spatial resolution of CMR compared with CTCA, although recent work by Gharib on a 3T scanner shows that this gap can be bridged (38). Secondly, CTCA allows 3 dimensional volume acquisition rather than accumulating multiple 2 dimensional slices in CMR, which can be time consuming. However, time to acquisition of a Coronary MRI has been reduced to approximately 5 minutes in large units and recent work by Iyama in healthy patients shows the feasibility of even quicker acquisition (39,40).
Compared to the plethora of data on CAC, CTCA, plaque volume on Cardiac CT, there is relatively scant prognostic data on coronary MRA. Yoon studied 207 patients who had coronary MRA and reviewed all vessel segments irrespective of image quality (41). Over a follow up of 25 months [inter quartile range (IQR), 16−33] 1 out of 123 patients without a significant stenosis and 10 of the 84 patients with stenosis >50% met the combined cardiac endpoint. The annual event rate was 3.9% vs. 0% for severe cardiac events (P=0.003) and 6.3% vs. 0.3% for all cardiac events (P<0.001). The presence of significant stenosis on CMR was associated with a HR of 20.78 (95% CI, 2.65−162; P=0.001) (41). The authors noted, despite their findings, there has been restrained utilization of the CMR due to its spatial resolution (1−1.5 mm) and acquisition time. The generalizability of this small intermediate risk cohort is limited and further prognostic studies with large cohorts are required before CTCA is dethroned as the primary non-invasive imaging modality for visualizing coronary atherosclerosis.
The feasibility of coronary plaque imaging was demonstrated by Fayad and expanded upon by Botnar (42,43). Since then using knowledge from carotid plaques [where plaque hemorrhage and lipid rich necrotic cores manifest as high signal intensity on T 1 weighted (T1W) sequences], Kawasaki has evaluated the components of High intensity plaque (HIP) on MRI and compared these with IVUS/CTCA demonstrating higher frequency of PR by both CTCA (89% vs. 0%, P<0.0001) and IVUS (94% vs. 14%, P<0.001) and ultrasound attenuation (100% vs. 14.3%, P<0.0001) (44). Importantly, Noguchi et al have shown the prognostic value of these HIPs. In a study of 568 patients with known or suspected CAD, the plaque to myocardium signal intensity ratio (PMR) was calculated. Plaques with PMR ≥1.4 independently predicted coronary events [HR: 3.96 (95% CI, 1.92−8.17), P<0.001] compared with the presence of CAD [HR: 3.56 (95% CI, 1.76−7.20), P<0.001] and traditional risk factors (45). Although plaque characterization by T1W MRI is technically demanding and its use is currently limited to research centers, corroborating data has been published by this group, where they showed that statin therapy reduces the PMR by comparison between propensity-matched cohorts (46).
Despite the premise of this article being prediction of cardiovascular events by visualizing atherosclerosis, it would be incomplete to mention CMR without highlighting the significant outcome data supporting the prognostic value of functional assessment of ischemia with this modality. Indeed, when coupled with the anatomical assessment, it presents the sought after “one stop shop” approach to ischemic heart disease. Lipinski reviewed 19 studies evaluating stress CMR and found a higher incidence of MI [odds ratio (OR): 7.7, P<0.0001], cardiovascular death (OR: 7.0, P<0.0001), and the combined endpoint (OR: 6.5, P<0.0001) in those with demonstrable ischemia compared with those with a negative study (47). An earlier meta-analysis of studies involving intermediate risk patients found that adenosine stress perfusion MRI demonstrated a sensitivity of 0.91 (95% CI, 0.88−0.94) and specificity of 0.81 (95% CI, 0.77−0.85) (48). Song and colleagues have put this anatomical and functional approach to the test in a retrospective study of 332 patients. The combined technique detected significant CAD in 13 patients (3.8%), 11 of whom had stenosis >50% and a corresponding stress perfusion defect (49).
An editorial in 2011 by Schoenhagen and Nagel stated that both CTCA and MRA can be used to assess coronary artery stenosis with a high negative predictive value (50). However, their respective strengths are anatomical assessment and physiological assessment, respectively. This holds true as the standard approach in most institutions. Further prospective trials incorporating both anatomical and functional assessment and improving coronary imaging protocols may lead to a change in practice in the future. MRA may be a good option for younger patients in whom radiation dose or serial follow are concerns. Developments which may change this paradigm are improved spatial resolution, time of acquisition and lastly molecular imaging, which holds tremendous promise (51) as does the future prospect of multimodality imaging.
Invasive plaque assessment.
Intravascular ultrasound (IVUS)
Over the decades, IVUS has been used as a primary invasive tool for coronary plaque assessment, utilizing its superiority over coronary luminography (i.e., coronary angiography). With an axial resolution of 150 to 250 µm and a lateral resolution of 250 µm, several findings of vulnerability have been identified (Figures 2,3). In addition to conventional grayscale IVUS, new generation of IVUS are available. Virtual histology (VH)-IVUS uses auto regression model to generate spectral parameters for the backscattered signal which are then color coded (Figure 2), whereas integrated backscatter (IB)-IVUS uses Fast Fourier Transformation to analyze the radiofrequency backscatter and create a color map. Given the inherent limitations placed on IVUS by its spatial resolution, a necrotic core >10% without evident overlying fibrous tissue and a plaque volume of >40% is required as a surrogate for thin-cap fibroatheroma (TCFA) (53). IVUS elastography and palpography have also been used to characterize the stress strain relationship on coronary arteries and plaques.
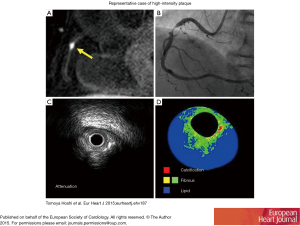
There is significant prognostic data supporting the use of IVUS, furthermore direct relationships among atherosclerotic burden, progression and adverse cardiovascular events have been documented. The strength of IVUS is to facilitate real time decision making. Acutely risk of peri-procedural myocardial infarct (PMI) is a concern and this is discussed in detail below. However immediate decisions have long-term prognostic outcomes. Ahn performed a meta-analysis of 3 randomized controlled trials (RCTs) and 14 observational studies showing that IVUS guided revascularization resulted in more stents, longer stents and larger stents-reflecting true assessment of atherosclerotic disease burden and vessel and plaque size. Importantly, these changes which favored a more aggressive interventional strategy were associated with lower risk of TLR, death, MI and stent thrombosis (54). The PROSPECT trial, which enrolled 697 ACS patients (secondary prevention), identified three characteristics predictive of subsequent cumulative MACE rate: plaque burden ≥70% (HR: 5.03; 95% CI, 2.51−10.11, P<0.001), a minimal lumen diameter (MLA) ≤4.0 (HR: 3.21; 95% CI, 1.61−6.42, P=0.001) or TCFA identified by RF IVUS (HR: 3.35; 95% CI, 1.77−6.36, P<0.001). It must be noted that in the absence of any of these three IVUS parameters, the risk of MACE was 0.3%. However, if ≥2 of these features are seen, the MACE risk is 10% to 18% over the next three years (55). VIVA study corroborates these findings, which reported that VH-TCFA (HR: 8.16, P=0.007), plaque burden >70% (HR: 7.48, P<0.001), and MLA <4 mm2 (HR: 2.91, P=0.036) were associated with total MACE on individual plaque analysis. Thirteen of the 19 MACE outcomes were at non-culprit lesion events (56). This study also assessed patients with stable angina in addition to the ACS population seen in PROSPECT. Despite this and other fundamental differences in the two trials, their findings are remarkably similar.
Not only inner plaque characteristics but also outer wall per see has prognostic information. Okura reviewed the prognostic value of PR at the target lesion in short (~2 yrs) (57) and long term (~6 yrs) follow-up (58). MACE free survival was significantly lower in the group with PR at target lesion (HR: 2.4, P=0.02). Specifically, however, the rates of target lesion revascularization (TLR) were higher in patients with PR (33.3% vs. 13.7%, P=0.01) (58).
A review of six clinical trials documenting plaque volume and progression was performed with MACE as the endpoint. Baseline percent atheroma volume was associated with MACE. Serial follow-up documented greater increases in the percent atheroma volume in the MACE cohort. This difference was largely driven by the need for coronary revascularization (59).
Finally, VH-IVUS has also been used to follow up effect of statin therapy on plaque characteristics offering not only initial prognostic information but data on how the vulnerable characteristics of a plaque can be modified by therapy (60).
Optical coherence tomography (OCT)
OCT has been used initially in ophthalmology and is now clinically available in cardiology. Compared with IVUS, OCT offers more precise assessment of coronary plaques, utilizing a 10-time greater resolution than that of IVUS (a spatial resolution of 10 µm axially and 20−40 µm laterally). OCT enables the visualization of several important plaque features assisting in determining plaque vulnerability: TCFA, plaque rupture, plaque erosion, lipid core, micro-calcification and neo-vascularization. The description of TCFA incorporates a fibrous cap <70 µm and also requires the arc of the lipid pool to subtend an angle >90 degrees in certain trials as seen in Figure 4. However, there is no strict definition of TCFA and a consensus statement rather ambiguously suggested, OCT-TCFA should reflect the histological definition of a TCFA (61).
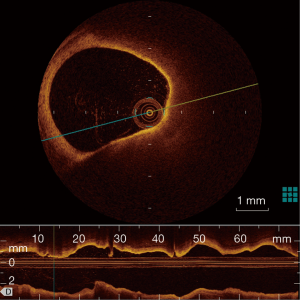
Unlike the IVUS studies, there is limited data on the long term predictive value of OCT TCFA lesions as it is a younger technology. A study of 67 patients with OCT showed thinner fibrous cap, larger areas of TCFA and more inflammation was evident on STEMI patients compared to those with SAP (62). Uemura et al. have shown that TCFA and micro-channel images showed a high correlation with subsequent luminal progression (OR: 20.0, P<0.01 for both) (63). Niccoli found MACE occurred more frequently in patients with plaque rupture at the target lesion when compared with those having intact fibrous cap (39.0% vs. 14.0%, P=0.001) in an ACS cohort. Plaque rupture was an independent predictor of outcome on multivariable analysis (OR: 3.74; 95% CI, 1.36−9.74) (64). OCT has been used to follow TCFA and demonstrated an increase in the fibrous cap thickness of patients who were treated with lipid lowering therapy (65).
OCT also allows visualization of vasa vasorum. After initial validation study (66), Taruya has recently demonstrated that vasa vasorum volume is positively correlated with plaque volume, and that intra-plaque neovascularization is associated with features of plaque vulnerability, especially noting the coral tree pattern is more frequent in ruptured plaques.
Given the limited long term data, currently the evidence from PROSPECT and other IVUS trials following up VH-TCFA are used as surrogate to support outcomes with OCT. Though OCT is superior in regards to assessment of fibrous cap thickness and endothelial assessment, it is unable to give us vessel dimensions, plaque area, or “necrotic core” area, due to limited penetration. The extra details obtained by OCT have not translated to better short term outcomes compared to IVUS and its long-term predictive and prognostic data is lacking. Further long term studies need to be conducted to assess the predictive value of OCT in regards to long term outcomes of non-target lesions.
Near infra-red spectroscopy (NIRS)
Near Infrared light can penetrate arterial tissue and the reflected spectral pattern is used to assess the lipid content of arterial walls, as lipid core burden index (LCBI). NIR-defined lipid-rich plaque has been assessed in various settings (67). NIRS can be also used to assess coronary lesions not apparent on angiography, such as ulcerated plaques with a re-cannalized lumen following treatment, or identifying culprit vessel in the context of an infarct with multivessel disease. As is the case with OCT and IVUS, a large lipid core is an essential component of the NIRS-identified vulnerable plaque. In stable coronary disease, a high LCBI and lipid chemogram can be used to assess vulnerability of a lesion. Oemrawsingh showed that a LCBI value greater than the median of 43 was associated with a 4-fold increase in MACE at 12 months follow up in a prospective manner (68). Currently six further prognostic trials/registers assessing the utility of NIRS are recruiting or planned (Table 5) (67).
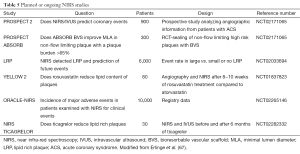
Full table
Angioscopy
Coronary Angioscopy allows visualization of the coronary luminal surface with color images. The color of plaques can be used to assess their vulnerability. A comparison between angiography and OCT, plaque color progressing from white to yellow is accompanied by a decrease in fibrous cap thickness (69). Despite more than two decades of research in this field, prognostic data is limited. Itoh demonstrated in a small cohort the risk of re-stenosis was greater in yellow plaques compared to white plaques (70). Initial work by Uchida showed that Angioscopy can be used to predict ACS events (71). Ohtani demonstrated that number of yellow plaques (NYP) was higher in patients with ACS (72). Gradation within yellowness of plaques is also associated with prognosis in AMI (73). Recently, Angioscopy has also been used to follow up neointimal coverage, thrombi and plaque color after drug-eluting stent deployment (74). The subjectivity and complexity of Angioscopy has resulted in limited uptake of this technology.
Prediction of peri-procedure events
The role of both non-invasive and invasive modalities as an aid to planning of percutaneous coronary intervention (PCI) is predicated on its ability to foresee acute peri-procedural events and our ability to appropriately reduce this risk. After the initial promising result of the TAPAS study, more recent evidence does not support the routine use of aspiration thrombectomy in ACS (75,76). Similarly, the role of statins, high dose antiplatelets, and distal protection devices have been evaluated with currently mixed results. The lack of definitive benefit may reflect our inability to target lesions at high risk of distal embolization.
The primary use of IVUS has been in assessing culprit lesions and vessels with view to PCI. Perhaps the utility of real-time information and prediction of immediate peri-procedural outcomes is its more tangible benefit. Claessen reviewed 11 articles discussing the use of IVUS in predicting distal embolization and no reflow phenomenon (77). A necrotic core volume of 33.4 mm3 as judged by VH-IVUS (78) had a sensitivity of 81.7% and specificity of 63.6% in regards to predicting no reflow in STEMI patients. Hong found that the percentage necrotic core volume remained an independent predictor of no-reflow (OR: 1.13, P<0.01). Moreover, at least 1 VH-TCFA, as well as multiple VH-TCFA, were significantly more common in patients with no-reflow (71% vs. 36%, P<0.01) (79). A lipid volume of 45.6 mm3 was found to be a predictor of PMI as defined by a troponin rise (80). Furthermore, as mentioned earlier, IVUS guided stent implantation is associated with a lower risk of long-term events. There is also evidence to suggest acute events such as death/MI within 30 days are reduced from an IVUS guided imaging strategy (Matrix Registry) (81).
OCT TCFA is an independent predictor of post procedural MI (OR: 4.68, P<0.01) and OCT derived lipid arc can be used to predict no reflow (82,83). Recent data combining TCFA as assessed by OCT (OR: 3.4; 95% CI, 1.3−8.9, P=0.012) and echo attenuated plaque as assessed by IVUS (OR: 3.6; 95% CI, 1.7−7.7, P<0.001) predicted PMI in stable angina. The combination of the two modalities had a positive predictive value of 82.4%. Furthermore PMI was an independent predictor of adverse cardiac events during 1-year follow-up illustrating the inextricable link between short and long term outcomes.
NIRS-derived Lipid rich coronary plaques are at risk of embolization during PCI (84). Patients with a high lipid content on Chemogram have a higher risk of PMI. CANARY trial showed that a maximal LCBI over any 4-mm length was higher in patients with MI than without [481.5 (425.6 to 679.6) vs. 371.5 (228.9 to 611.6), P=0.05]. As part of this pilot study of 31 patients with max LCBI 4 mm >600 were randomized to PCI with or without a distal protection device and there was no difference between the two groups (35.7% vs. 23.5%; relative risk: 1.52; 95% CI, 0.50−4.60, P=0.69) (85).
Non- Invasive disease assessment may also play a role in planning interventional strategies in the future. CTCA can be used to predict post elective angioplasty troponin rise. Watanabe showed both PR (OR: 4.54; 95% CI, 1.36−15.9, P=0.014) and spotty calcification (OR: 4.27; 95% CI, 1.30−14.8, P=0.016) were statistically significant independent predictors for cTnT elevation. When the above two were coupled with LAP <55 HU, the presence of all three risk factors was associated with a high positive predictive value of 94%, and their absence showed a high negative predictive value of 90% for PMI (86). Similar predictive value was shown in regards to volume of LAP and predicting post PCI troponin rise (87).
Hoshi and colleagues demonstrated the feasibility of CMR findings in predicting PMI, where PMI occurred more frequently in the HIP than non-HIP group (58.1% vs. 10.9%, P<0.001) and that using a PMR >1.4 was a significant predictor of PMI (OR: 5.63; 95% CI, 1.28−24.7, P=0.022) (52). A representative image of HIP (A), angiographic stenosis (B), IVUS attenuation (C) and IB-IVUS (D) is shown in Figure 2 (52). These results further validated by Asaumi showing a PMR >1.3 cutoff (OR) predicted PMI with a sensitivity and specificity of 67% and 86% respectively (88).
Despite the 2013 ACC/AHA guidelines assigning a class III indication for revascularization of non-culprit lesions, the PRAMI trial and the CvLPRIT trial have both advocated the role of complete revascularization following STEMI presentations (89-91). These non-culprit lesions could first be assessed by one of the above modalities particularly OCT. Kato has demonstrated the utility of OCT in assessing plaque characteristics and shown that the non-culprit lesions in an ACS have more vulnerable plaque characteristics than those studied in non ACS patients (92).
The strengths and limitations of invasive modalities have been summarized. Knowledge gap in the literature here is systematic implementation studies, which maximize the benefits of each diagnostic modality and minimize the drawbacks and costs. It should be warranted to conduct such studies how to implement these modalities by balancing their advantages and disadvantages in medical, economic and social contexts. It could be guided by the primary reason for the use of imaging modalities i.e., priority of the subsequent outcomes: what the interventionist wants to know is whether causes of ACS (plaque rupture, erosion, spasm, or spontaneous coronary dissection) for subsequent management; size of stent/balloon for better long term patency; existence of lipid core to avoid peri-procedure distal emboli; or other reasons. Given the rapid update cycle of scientific evidence with continuous implementation of new devices, life expectancy of the systematic implementation scheme might not be long enough to be widely used, especially when without considering economic factors for affordability and sustainability. By comparing new service with existing or competing services, a cost-effective analysis may be constructed. The process of conducting and reporting these studies is currently under review its results will guide such studies (93). With the assistance of clinical outcome trials, systematic implementation studies, and various registries, we will be able to better allocate the “right test” for the “right patient”.
From vulnerable plaque to vulnerable patient
All modalities discussed above assess similar aspects of coronary atherosclerosis, so-called “popcorn plaque” (94), to predict both long and short term outcomes. Underlying hypothesis, though biological plausible, is that “there is a 1-to-1 relationship between TCFA and a high-risk plaque” (95). Despite the above evidence supporting the vulnerable plaque there is also a body of evidence asking us to consider not the vulnerable plaque but the vulnerable patient. While it is true that autopsy studies suggest that TCFAs are most often found in the culprit lesions in ACS (96), it is quite another story to show the presence of this morphology as a harbinger of ACS prospectively. Arbab-Zadeh and Fuster have recently summarized the predictive value of the vulnerable plaque (97). They argue that both non-invasive and invasive studies targeting vulnerable plaques do not account for overall atherosclerotic disease burden as a potential confounder (97). Plaque rupture and healing may also be a silent event thus not all vulnerable plaques which rupture lead to acute coronary events. Maehara et al. reported that 20−25% of patients with plaque rupture has SAP or were asymptomatic (98). Furthermore, recent work by Kubo supports the dynamic view of vulnerability. Of 216 non culprit lesions studied 75% of VH IVUS identified TCFAs healed during one year follow up whereas new lesions also developed (99). There is an argument for a shift in paradigm from lesion focused approach to elucidate the disease burden as “pan-coronaritis” (100). Rioufol et al. performed three-vessel IVUS imaging on 24 ACS cases and found that among 50 ruptured plaques, nine within culprit lesion and 41 at non-culprit one including 19 patients with ruptures in ≥2 arteries (101). This was substantiated by IVUS (102) and OCT studies (103-105). The robust predictive value of the CAC score likely reflects its assessment of total atherosclerotic disease burden (16). Physiological assessment of ischemia invasively and non-invasively is another component of this discussion which deserves equal weight in regards to predicting cardiovascular outcomes. The utility of multimodality imaging, concomitant functional/anatomical assessment and mathematical modelling are all tools being investigated for their predictive value in regards to coronary atherosclerosis. Integrating this plethora of information clinically and ultimately developing a score which takes into account the vulnerable plaque, the vulnerable circulation and the vulnerable patient should be the goal of both invasive and non-invasive imaging.
Acknowledgements
The authors appreciate the courtesy of Dr Hiroko Tsuchiya, for providing Figures 3,4. The authors received no specific funding for this work. Kazuaki Negishi, MD, PhD, is supported by an award from the Select Foundation, which had no role in study design, data collection and analysis, decision to publish, or preparation of the manuscript.
Footnote
Conflicts of Interest: The authors have no conflicts of interest to declare.
References
- Shishikura D. Noninvasive imaging modalities to visualize atherosclerotic plaques. Cardiovasc Diagn Ther 2016;6:340-53.
- Honda S, Kataoka Y, Kanaya T, et al. Characterization of coronary atherosclerosis by intravascular imaging modalities. Cardiovasc Diagn Ther 2016;6:368-81.
- Hachamovitch R, Di Carli MF. Methods and limitations of assessing new noninvasive tests: Part II: Outcomes-based validation and reliability assessment of noninvasive testing. Circulation 2008;117:2793-801. [Crossref] [PubMed]
- Hlatky MA, Greenland P, Arnett DK, et al. Criteria for evaluation of novel markers of cardiovascular risk: a scientific statement from the American Heart Association. Circulation 2009;119:2408-16. [Crossref] [PubMed]
- Greenland P, LaBree L, Azen SP, et al. Coronary artery calcium score combined with Framingham score for risk prediction in asymptomatic individuals. JAMA 2004;291:210-5. [Crossref] [PubMed]
- Taylor AJ, Cerqueira M, Hodgson JM, et al. ACCF/SCCT/ACR/AHA/ASE/ASNC/NASCI/SCAI/SCMR 2010 appropriate use criteria for cardiac computed tomography. A report of the American College of Cardiology Foundation Appropriate Use Criteria Task Force, the Society of Cardiovascular Computed Tomography, the American College of Radiology, the American Heart Association, the American Society of Echocardiography, the American Society of Nuclear Cardiology, the North American Society for Cardiovascular Imaging, the Society for Cardiovascular Angiography and Interventions, and the Society for Cardiovascular Magnetic Resonance. J Am Coll Cardiol 2010;56:1864-94. [Crossref] [PubMed]
- Pundziute G, Schuijf JD, Jukema JW, et al. Prognostic value of multislice computed tomography coronary angiography in patients with known or suspected coronary artery disease. J Am Coll Cardiol 2007;49:62-70. [Crossref] [PubMed]
- Chan AW, Tetzlaff JM, Gotzsche PC, et al. SPIRIT 2013 explanation and elaboration: guidance for protocols of clinical trials. BMJ 2013;346:e7586. [Crossref] [PubMed]
- Hamby RI, Tabrah F, Wisoff BG, et al. Coronary artery calcification: Clinical implications and angiographic correlates. Am Heart J 1974;87:565-70. [Crossref] [PubMed]
- Agatston AS, Janowitz WR, Hildner FJ, et al. Quantification of coronary artery calcium using ultrafast computed tomography. J Am Coll Cardiol 1990;15:827-32. [Crossref] [PubMed]
- Morin RL, Gerber TC, McCollough CH. Radiation Dose in Computed Tomography of the Heart. Circulation 2003;107:917-22. [Crossref] [PubMed]
- Detrano R, Guerci AD, Carr JJ, et al. Coronary calcium as a predictor of coronary events in four racial or ethnic groups. N Engl J Med 2008;358:1336-45. [Crossref] [PubMed]
- Erbel R, Möhlenkamp S, Moebus S, et al. Coronary Risk Stratification, Discrimination, and Reclassification Improvement Based on Quantification of Subclinical Coronary Atherosclerosis: The Heinz Nixdorf Recall Study. J Am Coll Cardiol 2010;56:1397-406. [Crossref] [PubMed]
- Elias-Smale SE, Proenca RV, Koller MT, et al. Coronary calcium score improves classification of coronary heart disease risk in the elderly: the Rotterdam study. J Am Coll Cardiol 2010;56:1407-14. [Crossref] [PubMed]
- Hecht HS, Narula J. Coronary artery calcium scanning in asymptomatic patients with diabetes mellitus: a paradigm shift. J Diabetes 2012;4:342-50. [Crossref] [PubMed]
- Hecht HS. Coronary Artery Calcium Scanning: Past, Present, and Future. JACC Cardiovasc Imaging 2015;8:579-96. [Crossref] [PubMed]
- Valenti V. A 15-Year Warranty Period for Asymptomatic Individuals Without Coronary Artery Calcium: A Prospective Follow-Up of 9,715 Individuals. JACC Cardiovasc Imaging 2015;8:900-9. [Crossref] [PubMed]
- Hadamitzky M, Distler R, Meyer T, et al. Prognostic Value of Coronary Computed Tomographic Angiography in Comparison With Calcium Scoring and Clinical Risk Scores. Circ Cardiovasc Imaging 2011;4:16-23. [Crossref] [PubMed]
- Hadamitzky M, Achenbach S, Al-Mallah M, et al. Optimized prognostic score for coronary computed tomographic angiography: results from the CONFIRM registry (COronary CT Angiography EvaluatioN For Clinical Outcomes: An InteRnational Multicenter Registry). J Am Coll Cardiol 2013;62:468-76. [Crossref] [PubMed]
- Hulten EA, Carbonaro S, Petrillo SP, et al. Prognostic Value of Cardiac Computed Tomography AngiographyA Systematic Review and Meta-Analysis. J Am Coll Cardiol 2011;57:1237-47. [Crossref] [PubMed]
- Hou ZH, Lu B, Gao Y, et al. Prognostic value of coronary CT angiography and calcium score for major adverse cardiac events in outpatients. JACC Cardiovasc Imaging 2012;5:990-9. [Crossref] [PubMed]
- Schlett CL, Banerji D, Siegel E, et al. Prognostic value of CT angiography for major adverse cardiac events in patients with acute chest pain from the emergency department: 2-year outcomes of the ROMICAT trial. JACC Cardiovasc Imaging 2011;4:481-91. [Crossref] [PubMed]
- Min JK, Labounty TM, Gomez MJ, et al. Incremental prognostic value of coronary computed tomographic angiography over coronary artery calcium score for risk prediction of major adverse cardiac events in asymptomatic diabetic individuals. Atherosclerosis 2014;232:298-304. [Crossref] [PubMed]
- Russo V, Zavalloni A, Bacchi Reggiani ML, et al. Incremental Prognostic Value of Coronary CT Angiography in Patients With Suspected Coronary Artery Disease. Circ Cardiovasc Imaging 2010;3:351-9. [Crossref] [PubMed]
- Versteylen MO, Kietselaer BL, Dagnelie PC, et al. Additive value of semiautomated quantification of coronary artery disease using cardiac computed tomographic angiography to predict future acute coronary syndrome. J Am Coll Cardiol 2013;61:2296-305. [Crossref] [PubMed]
- Motoyama S, Sarai M, Harigaya H, et al. Computed Tomographic Angiography Characteristics of Atherosclerotic Plaques Subsequently Resulting in Acute Coronary Syndrome. J Am Coll Cardiol 2009;54:49-57. [Crossref] [PubMed]
- Otsuka K, Fukuda S, Tanaka A, et al. Napkin-ring sign on coronary CT angiography for the prediction of acute coronary syndrome. JACC Cardiovasc Imaging 2013;6:448-57. [Crossref] [PubMed]
- Motoyama S, Ito H, Sarai M, et al. Plaque Characterization by Coronary Computed Tomography Angiography and the Likelihood of Acute Coronary Events in Mid-Term Follow-Up. J Am Coll Cardiol 2015;66:337-46. [Crossref] [PubMed]
- De Bruyne B, Pijls NH, Kalesan B, et al. Fractional flow reserve-guided PCI versus medical therapy in stable coronary disease. N Engl J Med 2012;367:991-1001. [Crossref] [PubMed]
- Koo BK, Erglis A, Doh JH, et al. Diagnosis of ischemia-causing coronary stenoses by noninvasive fractional flow reserve computed from coronary computed tomographic angiograms. Results from the prospective multicenter DISCOVER-FLOW (Diagnosis of Ischemia-Causing Stenoses Obtained Via Noninvasive Fractional Flow Reserve) study. J Am Coll Cardiol 2011;58:1989-97. [Crossref] [PubMed]
- Min JK, Leipsic J, Pencina MJ, et al. DIagnostic accuracy of fractional flow reserve from anatomic CT angiography. JAMA 2012;308:1237-45. [Crossref] [PubMed]
- Kim KH, Doh JH, Koo BK, et al. A novel noninvasive technology for treatment planning using virtual coronary stenting and computed tomography-derived computed fractional flow reserve. JACC Cardiovasc Interv 2014;7:72-8. [Crossref] [PubMed]
- Raggi P, Callister TQ, Shaw LJ. Progression of coronary artery calcium and risk of first myocardial infarction in patients receiving cholesterol-lowering therapy. Arterioscler Thromb Vasc Biol 2004;24:1272-7. [Crossref] [PubMed]
- Finn JP, Nael K, Deshpande V, et al. Cardiac MR imaging: state of the technology. Radiology 2006;241:338-54. [Crossref] [PubMed]
- Hamdan A, Asbach P, Wellnhofer E, et al. A prospective study for comparison of MR and CT imaging for detection of coronary artery stenosis. JACC Cardiovasc Imaging 2011;4:50-61. [Crossref] [PubMed]
- Yang Q, Li K, Liu X, et al. Contrast-enhanced whole-heart coronary magnetic resonance angiography at 3.0-T: a comparative study with X-ray angiography in a single center. J Am Coll Cardiol 2009;54:69-76. [Crossref] [PubMed]
- Kato S, Kitagawa K, Ishida N, et al. Assessment of coronary artery disease using magnetic resonance coronary angiography: a national multicenter trial. J Am Coll Cardiol 2010;56:983-91. [Crossref] [PubMed]
- Gharib AM, Abd-Elmoniem KZ, Ho VB, et al. The feasibility of 350 mum spatial resolution coronary magnetic resonance angiography at 3 T in humans. Invest Radiol 2012;47:339-45. [Crossref] [PubMed]
- Makowski MR, Henningsson M, Spuentrup E, et al. Characterization of Coronary Atherosclerosis by Magnetic Resonance Imaging. Circulation 2013;128:1244-55. [PubMed]
- Iyama Y, Nakaura T, Kidoh M, et al. Single-breath-hold whole-heart coronary MRA in healthy volunteers at 3.0-T MRI. Springerplus 2014;3:667. [Crossref] [PubMed]
- Yoon YE, Kitagawa K, Kato S, et al. Prognostic Value of Coronary Magnetic Resonance Angiography for Prediction of Cardiac Events in Patients With Suspected Coronary Artery Disease. J Am Coll Cardiol 2012;60:2316-22. [Crossref] [PubMed]
- Fayad ZA, Fuster V, Fallon JT, et al. Noninvasive in vivo human coronary artery lumen and wall imaging using black-blood magnetic resonance imaging. Circulation 2000;102:506-10. [Crossref] [PubMed]
- Botnar RM, Stuber M, Kissinger KV, et al. Noninvasive coronary vessel wall and plaque imaging with magnetic resonance imaging. Circulation 2000;102:2582-7. [Crossref] [PubMed]
- Kawasaki T, Koga S, Koga N, et al. Characterization of hyperintense plaque with noncontrast T(1)-weighted cardiac magnetic resonance coronary plaque imaging: comparison with multislice computed tomography and intravascular ultrasound. JACC Cardiovasc Imaging 2009;2:720-8. [Crossref] [PubMed]
- Noguchi T, Kawasaki T, Tanaka A, et al. High-intensity signals in coronary plaques on noncontrast T1-weighted magnetic resonance imaging as a novel determinant of coronary events. J Am Coll Cardiol 2014;63:989-99. [Crossref] [PubMed]
- Noguchi T, Tanaka A, Kawasaki T, et al. Effect of Intensive Statin Therapy on Coronary High-Intensity Plaques Detected by Noncontrast T1-Weighted Imaging: The AQUAMARINE Pilot Study. J Am Coll Cardiol 2015;66:245-56. [Crossref] [PubMed]
- Lipinski MJ, McVey CM, Berger JS, et al. Prognostic value of stress cardiac magnetic resonance imaging in patients with known or suspected coronary artery disease: a systematic review and meta-analysis. J Am Coll Cardiol 2013;62:826-38. [Crossref] [PubMed]
- Nandalur KR, Dwamena BA, Choudhri AF, et al. Diagnostic performance of stress cardiac magnetic resonance imaging in the detection of coronary artery disease: a meta-analysis. J Am Coll Cardiol 2007;50:1343-53. [Crossref] [PubMed]
- Song KD, Kim SM, Choe YH, et al. Integrated cardiac magnetic resonance imaging with coronary magnetic resonance angiography, stress-perfusion, and delayed-enhancement imaging for the detection of occult coronary artery disease in asymptomatic individuals. Int J Cardiovasc Imaging 2015;31 Suppl 1:77-89. [Crossref] [PubMed]
- Schoenhagen P, Nagel E. Noninvasive assessment of coronary artery disease anatomy, physiology, and clinical outcome. JACC Cardiovasc Imaging 2011;4:62-4. [Crossref] [PubMed]
- Hyafil F, Cornily JC, Feig JE, et al. Noninvasive detection of macrophages using a nanoparticulate contrast agent for computed tomography. Nat Med 2007;13:636-41. [Crossref] [PubMed]
- Hoshi T, Sato A, Akiyama D, et al. Coronary high-intensity plaque on T1-weighted magnetic resonance imaging and its association with myocardial injury after percutaneous coronary intervention. Eur Heart J 2015;36:1913-22. [Crossref] [PubMed]
- Suh WM, Seto AH, Margey RJP, et al. Intravascular Detection of the Vulnerable Plaque. Circ Cardiovasc Imaging 2011;4:169-78. [Crossref] [PubMed]
- Ahn JM, Kang SJ, Yoon SH, et al. Meta-analysis of outcomes after intravascular ultrasound-guided versus angiography-guided drug-eluting stent implantation in 26,503 patients enrolled in three randomized trials and 14 observational studies. Am J Cardiol 2014;113:1338-47. [Crossref] [PubMed]
- Stone GW, Maehara A, Lansky AJ, et al. A Prospective Natural-History Study of Coronary Atherosclerosis. N Engl J Med 2011;364:226-35. [Crossref] [PubMed]
- Calvert PA, Obaid DR, O’Sullivan M, et al. Association between IVUS findings and adverse outcomes in patients with coronary artery disease: the VIVA (VH-IVUS in Vulnerable Atherosclerosis) Study. JACC Cardiovasc Imaging 2011;4:894-901. [Crossref] [PubMed]
- Okura H, Taguchi H, Kubo T, et al. Impact of arterial remodelling and plaque rupture on target and non-target lesion revascularisation after stent implantation in patients with acute coronary syndrome: an intravascular ultrasound study. Heart 2007;93:1219-25. [Crossref] [PubMed]
- Okura H, Kataoka T, Matsushita N, et al. Culprit lesion remodelling and long-term prognosis in patients with acute coronary syndrome: an intravascular ultrasound study. Eur Heart J Cardiovasc Imaging 2013;14:758-64. [Crossref] [PubMed]
- Nicholls SJ, Hsu A, Wolski K, et al. Intravascular ultrasound-derived measures of coronary atherosclerotic plaque burden and clinical outcome. J Am Coll Cardiol 2010;55:2399-407. [Crossref] [PubMed]
- Nozue T, Yamamoto S, Tohyama S, et al. Statin treatment for coronary artery plaque composition based on intravascular ultrasound radiofrequency data analysis. Am Heart J 2012;163:191-9.e1. [Crossref] [PubMed]
- Tearney GJ, Regar E, Akasaka T, et al. Consensus standards for acquisition, measurement, and reporting of intravascular optical coherence tomography studies: a report from the International Working Group for Intravascular Optical Coherence Tomography Standardization and Validation. J Am Coll Cardiol 2012;59:1058-72. [Crossref] [PubMed]
- Galon MZ, Wang Z, Bezerra HG, et al. Differences determined by optical coherence tomography volumetric analysis in non-culprit lesion morphology and inflammation in ST-segment elevation myocardial infarction and stable angina pectoris patients. Catheter Cardiovasc Interv 2015;85:E108-15. [Crossref] [PubMed]
- Uemura S, Ishigami K, Soeda T, et al. Thin-cap fibroatheroma and microchannel findings in optical coherence tomography correlate with subsequent progression of coronary atheromatous plaques. Eur Heart J 2012;33:78-85. [Crossref] [PubMed]
- Niccoli G, Montone RA, Di Vito L, et al. Plaque rupture and intact fibrous cap assessed by optical coherence tomography portend different outcomes in patients with acute coronary syndrome. Eur Heart J 2015;36:1377-84. [Crossref] [PubMed]
- Hattori K, Ozaki Y, Ismail TF, et al. Impact of Statin Therapy on Plaque Characteristics as Assessed by Serial OCT, Grayscale and Integrated Backscatter−IVUS. JACC Cardiovasc Imaging 2012;5:169-77. [Crossref] [PubMed]
- Nishimiya K, Matsumoto Y, Uzuka H, et al. Accuracy of optical frequency domain imaging for evaluation of coronary adventitial vasa vasorum formation after stent implantation in pigs and humans - a validation study. Circ J 2015;79:1323-31. [Crossref] [PubMed]
- Erlinge D. Near-infrared spectroscopy for intracoronary detection of lipid-rich plaques to understand atherosclerotic plaque biology in man and guide clinical therapy. J Intern Med 2015;278:110-25. [Crossref] [PubMed]
- Oemrawsingh RM, Cheng JM, Garcia-Garcia HM, et al. Near-infrared spectroscopy predicts cardiovascular outcome in patients with coronary artery disease. J Am Coll Cardiol 2014;64:2510-8. [Crossref] [PubMed]
- Kubo T, Imanishi T, Takarada S, et al. Implication of plaque color classification for assessing plaque vulnerability: a coronary angioscopy and optical coherence tomography investigation. JACC Cardiovasc Interv 2008;1:74-80. [Crossref] [PubMed]
- Itoh A, Miyazaki S, Nonogi H, et al. Ang Angioscopic prediction of successful dilatation and of restenosis in percutaneous transluminal coronary angioplasty. Significance of yellow plaque. Circulation. 1995;91:1389-96. [Crossref] [PubMed]
- Uchida Y, Nakamura F, Tomaru T, et al. Prediction of acute coronary syndromes by percutaneous coronary angioscopy in patients with stable angina. Am Heart J 1995;130:195-203. [Crossref] [PubMed]
- Ohtani T, Ueda Y, Mizote I, et al. Number of yellow plaques detected in a coronary artery is associated with future risk of acute coronary syndrome: detection of vulnerable patients by angioscopy. J Am Coll Cardiol 2006;47:2194-200. [Crossref] [PubMed]
- Saratani K, Iwanaga Y, Hayashi T, et al. Impact of plaque color by angioscopic evaluation on long-term clinical outcomes in patients with acute myocardial infarction undergoing percutaneous coronary intervention. Atherosclerosis 2010;211:170-5. [Crossref] [PubMed]
- Nishino M, Yoshimura T, Nakamura D, et al. Comparison of angioscopic findings and three-year cardiac events between sirolimus-eluting stent and bare-metal stent in acute myocardial infarction. Am J Cardiol 2011;108:1238-43. [Crossref] [PubMed]
- Vlaar PJ, Svilaas T, van der Horst IC, et al. Cardiac death and reinfarction after 1 year in the Thrombus Aspiration during Percutaneous coronary intervention in Acute myocardial infarction Study (TAPAS): a 1-year follow-up study. Lancet 2008;371:1915-20. [Crossref] [PubMed]
- Fröbert O, Lagerqvist B, Olivecrona GK, et al. Thrombus Aspiration during ST-Segment Elevation Myocardial Infarction. N Engl J Med 2013;369:1587-97. [Crossref] [PubMed]
- 8. Claessen BE, Maehara A, Fahy M, et al. Plaque composition by intravascular ultrasound and distal embolization after percutaneous coronary intervention. JACC Cardiovasc Imaging 2012;5:S111-S8.
- Kawaguchi R, Oshima S, Jingu M, et al. Usefulness of virtual histology intravascular ultrasound to predict distal embolization for ST-segment elevation myocardial infarction. J Am Coll Cardiol 2007;50:1641-6. [Crossref] [PubMed]
- Hong YJ, Mintz GS, Kim SW, et al. Impact of plaque composition on cardiac troponin elevation after percutaneous coronary intervention: an ultrasound analysis. JACC Cardiovasc Imaging 2009;2:458-68. [Crossref] [PubMed]
- Uetani T, Amano T, Ando H, et al. The correlation between lipid volume in the target lesion, measured by integrated backscatter intravascular ultrasound, and post-procedural myocardial infarction in patients with elective stent implantation. Eur Heart J 2008;29:1714-20. [Crossref] [PubMed]
- Claessen BE, Mehran R, Mintz GS, et al. Impact of intravascular ultrasound imaging on early and late clinical outcomes following percutaneous coronary intervention with drug-eluting stents. JACC Cardiovasc Interv 2011;4:974-81. [Crossref] [PubMed]
- Tanaka A, Imanishi T, Kitabata H, et al. Lipid-rich plaque and myocardial perfusion after successful stenting in patients with non-ST-segment elevation acute coronary syndrome: an optical coherence tomography study. Eur Heart J 2009;30:1348-55. [Crossref] [PubMed]
- Yonetsu T, Kakuta T, Lee T, et al. Impact of plaque morphology on creatine kinase-MB elevation in patients with elective stent implantation. Int J Cardiol 2011;146:80-5. [Crossref] [PubMed]
- Goldstein JA, Maini B, Dixon SR, et al. Detection of lipid-core plaques by intracoronary near-infrared spectroscopy identifies high risk of periprocedural myocardial infarction. Circ Cardiovasc Interv 2011;4:429-37. [Crossref] [PubMed]
- Stone GW, Maehara A, Muller JE, et al. Plaque Characterization to Inform the Prediction and Prevention of Periprocedural Myocardial Infarction During Percutaneous Coronary Intervention: The CANARY Trial (Coronary Assessment by Near-infrared of Atherosclerotic Rupture-prone Yellow). JACC Cardiovasc Interv 2015;8:927-36. [Crossref] [PubMed]
- Watabe H, Sato A, Akiyama D, et al. Impact of coronary plaque composition on cardiac troponin elevation after percutaneous coronary intervention in stable angina pectoris: a computed tomography analysis. J Am Coll Cardiol 2012;59:1881-8. [Crossref] [PubMed]
- Uetani T, Amano T, Kunimura A, et al. The association between plaque characterization by CT angiography and post-procedural myocardial infarction in patients with elective stent implantation. JACC Cardiovasc Imaging 2010;3:19-28. [Crossref] [PubMed]
- Asaumi Y, Noguchi T, Morita Y, et al. High-Intensity Plaques on Noncontrast T1-Weighted Imaging as a Predictor of Periprocedural Myocardial Injury. JACC Cardiovasc Imaging 2015;8:741-3. [Crossref] [PubMed]
- Wald DS, Morris JK, Wald NJ, et al. Randomized trial of preventive angioplasty in myocardial infarction. N Engl J Med 2013;369:1115-23. [Crossref] [PubMed]
- Gershlick AH, Khan JN, Kelly DJ, et al. Randomized trial of complete versus lesion-only revascularization in patients undergoing primary percutaneous coronary intervention for STEMI and multivessel disease: the CvLPRIT trial. J Am Coll Cardiol 2015;65:963-72. [Crossref] [PubMed]
- American College of Emergency Physicians; Society for Cardiovascular Angiography and Interventions, O’Gara PT, et al. 2013 ACCF/AHA guideline for the management of ST-elevation myocardial infarction: a report of the American College of Cardiology Foundation/American Heart Association Task Force on Practice Guidelines. J Am Coll Cardiol 2013;61:e78-e140. [Crossref] [PubMed]
- Kato K, Yonetsu T, Kim SJ, et al. Nonculprit plaques in patients with acute coronary syndromes have more vulnerable features compared with those with non-acute coronary syndromes: a 3-vessel optical coherence tomography study. Circ Cardiovasc Imaging 2012;5:433-40. [Crossref] [PubMed]
- Pinnock H, Epiphaniou E, Sheikh A, et al. Developing standards for reporting implementation studies of complex interventions (StaRI): a systematic review and e-Delphi. Implement Sci 2015;10:42. [Crossref] [PubMed]
- Narula J, Strauss HW. The popcorn plaques. Nat Med 2007;13:532-4. [Crossref] [PubMed]
- Nissen SE. The vulnerable plaque “hypothesis”: promise, but little progress. JACC Cardiovasc Imaging 2009;2:483-5. [Crossref] [PubMed]
- Virmani R, Kolodgie FD, Burke AP, et al. Lessons from sudden coronary death: a comprehensive morphological classification scheme for atherosclerotic lesions. Arterioscler Thromb Vasc Biol 2000;20:1262-75. [Crossref] [PubMed]
- Arbab-Zadeh A, Fuster V. The Myth of the “Vulnerable Plaque”Transitioning From a Focus on Individual Lesions to Atherosclerotic Disease Burden for Coronary Artery Disease Risk Assessment. J Am Coll Cardiol 2015;65:846-55. [Crossref] [PubMed]
- Maehara A, Mintz GS, Bui AB, et al. Morphologic and angiographic features of coronary plaque rupture detected by intravascular ultrasound. J Am Coll Cardiol 2002;40:904-10. [Crossref] [PubMed]
- Kubo T, Maehara A, Mintz GS, et al. The dynamic nature of coronary artery lesion morphology assessed by serial virtual histology intravascular ultrasound tissue characterization. J Am Coll Cardiol 2010;55:1590-7. [Crossref] [PubMed]
- Choi SY, Mintz GS. What have we learned about plaque rupture in acute coronary syndromes? Curr Cardiol Rep 2010;12:338-43. [Crossref] [PubMed]
- Rioufol G, Finet G, Ginon I, et al. Multiple atherosclerotic plaque rupture in acute coronary syndrome: a three-vessel intravascular ultrasound study. Circulation 2002;106:804-8. [Crossref] [PubMed]
- Tanaka A, Shimada K, Sano T, et al. Multiple plaque rupture and C-reactive protein in acute myocardial infarction. J Am Coll Cardiol 2005;45:1594-9. [Crossref] [PubMed]
- Tanaka A, Imanishi T, Kitabata H, et al. Distribution and frequency of thin-capped fibroatheromas and ruptured plaques in the entire culprit coronary artery in patients with acute coronary syndrome as determined by optical coherence tomography. Am J Cardiol 2008;102:975-9. [Crossref] [PubMed]
- Kubo T, Imanishi T, Kashiwagi M, et al. Multiple coronary lesion instability in patients with acute myocardial infarction as determined by optical coherence tomography. Am J Cardiol 2010;105:318-22. [Crossref] [PubMed]
- Fujii K, Masutani M, Okumura T, et al. Frequency and predictor of coronary thin-cap fibroatheroma in patients with acute myocardial infarction and stable angina pectoris a 3-vessel optical coherence tomography study. J Am Coll Cardiol 2008;52:787-8. [Crossref] [PubMed]