Dual energy CT based scoring in chronic thromboembolic pulmonary hypertension and correlation with clinical and hemodynamic parameters: a retrospective cross-sectional study
Introduction
Chronic thromboembolic pulmonary hypertension (CTEPH), classified as a group 4 disorder in the updated clinical classification of pulmonary hypertension (PH), is defined as mean resting pulmonary arterial pressure (mPAP) of >20 mmHg on right heart catheterization with pulmonary vascular resistance of ≥3 Wood units (RHC) (1). The disease process is triggered by various predisposing factors, some known and others unclear, that result in incomplete resolution of acute pulmonary embolism (PE); this causes vascular remodeling resulting in elevation of mPAP and pulmonary vascular resistance (PVR) (2,3). With increased understanding of the disease, it is now clear that CTEPH is part of a heterogeneous group of loosely clustered processes that are along a continuum, labeled as post-PE syndrome (4). Timely diagnosis of CTEPH is critical since it is a potentially treatable condition with surgical pulmonary thromboendarterectomy (PTE) being the treatment of choice (5,6). Balloon pulmonary angioplasty has emerged as a promising alternative for surgically inaccessible disease or non-operable patients (7).
Dual-energy computed tomography (DECT) is a novel imaging technique that utilizes differences in attenuation of specific materials such as iodine at different energy levels (8-10). These material specific images, called perfused blood volume (PBV) maps, can be used in chest imaging to map the amount of iodine in a voxel of lung tissue. Several studies have shown that these can serve as a surrogate marker of lung perfusion (11-13). Studies have highlighted the incremental benefits of PBV maps in evaluation of CTEPH and in assessment of surgical candidacy. Qualitative and quantitative information from PBV maps help in diagnosis, provide indirect information on hemodynamic state, and also have the potential to indirectly reflect the state of underlying vascular bed (14-16).
As noted above, CTEPH is a potentially treatable disease, provided it is timely diagnosed and medical, interventional, and surgical methods can be successfully implemented in isolation or in combination for treatment. CTEPH patients can develop small vessel vasculopathy over time, which can worsen the prognosis of the disease (3). Imaging forms a crucial component of the diagnostic pathway with CT being one of the tests of choice for disease characterization and preoperative evaluation. Specific goals of diagnostic workup in CTEPH are to evaluate if the thrombus is surgically accessible, to assess the degree of increased PVR, and to evaluate if there is likelihood of underlying small vessel disease. The anatomic and perfusion information obtained from DECT offers a “one stop shop” technique that can address most of the required goals listed above. In this study, we tried to implement a simple DECT based scoring model in patients with known CTEPH and assess if it helps in noninvasive assessment of functional and hemodynamic information. We calculated anatomic and perfusion scores as well as a combined score for this purpose and correlated the scoring system with functional parameters (such as pulmonary function test indices) as well as invasive hemodynamic information obtained from RHC. We present the following article in accordance with the STROBE reporting checklist (available at https://cdt.amegroups.com/article/view/10.21037/cdt-21-686/rc).
Methods
Study population
The study was conducted in accordance with the Declaration of Helsinki (as revised in 2013). This retrospective cross-sectional study was performed with Cleveland Clinic institutional review board (IRB) approval and a waiver of individual informed consent. A waiver of informed consent was granted since CT scans were obtained as part of a diagnostic workup with little or no additional risk. Between March 2014 to March 2019, 298 patients with a known diagnosis of CTEPH were identified using a Cleveland Clinic registry. The diagnosis of CTEPH was reached at a multidisciplinary meeting utilizing a combination of clinical, functional, invasive and imaging data. Patients with no DECT imaging or incomplete hemodynamic information were excluded from the study. Also, patients with significant artifacts on DECT images were not included in the study population. Finally, four of these patients had two preoperative DECT scans as part of their evaluation. Final number in the study group was 78. The patient characteristics are summarized in Table 1. Also, details of patient enrollment are listed in Figure 1.
Table 1
Characteristic | Values |
---|---|
Age | 19–78 years, mean of 52 years |
Sex | Male 36 (46%), female 42 (54%) |
Weight | 60.2–135.6 kg, mean of 91 kg |
Comorbidities | |
Diabetes mellitus | 15/78 (19%) |
Hypertension | 42/78 (54%) |
Dyslipidemia | 14/78 (18%) |
Chronic obstructive pulmonary disease | 24/78 (31%) |
Smoking history | 24/78 (31%) |
Coronary artery disease/atrial fibrillation | 41/78 (53%) |
Renal failure | 6/78 (8%) |
Risk factors for CTEPH | |
Venous thromboembolism | 37/78 (47%) |
Cancer | 16/78 (21%) |
Thyroid abnormality | 11/78 (14%) |
Thrombophilia | 10/78 (13%) |
Pregnancy | 0 |
CTEPH, chronic thromboembolic pulmonary hypertension.
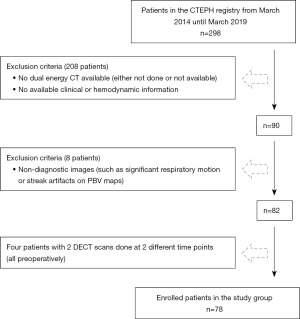
CT protocol
DECT scans were performed either on a Somatom Definition Flash or Definition Force CT scanner (Siemens Healthcare, Forchheim, Germany) equipped with automated tube voltage selection (CARE kV; Siemens Healthcare). Default kilovoltage (kVp) settings for the DECT protocol were 100 and 140 Sn kVp on the Flash scanner and 80 and 150 Sn kVp on the Force scanner. Quality reference milliampere (mAs) values was 170 for tube A on both scanners. Other settings of the CT protocol were 0.28 and 0.2 seconds rotation time on Flash and Force scanners respectively, and pitch of 0.55 on both scanners. A weight-based P3T soft-ware (MedRad, Bayer Healthcare, Germany) was used to calculate the contrast volume, which was injected at a rate of 5 mL/s. A region of interest (ROI) was placed on the main pulmonary artery. A Hounsfield Unit (HU) threshold of 150 was used. A caudocranial scan direction was used.
Image reconstruction and data analysis
Both the low-kVp and high-kVp data were reconstructed at 1-mm slice thickness using 1-mm increments in the axial plane. A mixed image dataset was created using low and high kVp image datasets using a commercially available workstation (syngo CT Workplace, VA44A; Siemens Healthcare). Also, using 3-material decomposition (iodine, soft tissue, and air), color-coded lung PBV images were reconstructed. At our institution, these are reconstructed at 3×3-mm intervals in axial and coronal planes. The ROI of at least 0.5 cm2 was placed in the main pulmonary trunk to calibrate the color coding.
For data analysis, the mixed dataset images were evaluated for morphologic indices. First, clot score was calculated. Chronic clots were characterized using morphologic features such as atretic and poorly opacified arteries, bands and webs, and areas of stenosis and luminal narrowing. Clot score was calculated by assigning a following score to presence of arterial clot: pulmonary trunk—5, each main pulmonary artery—4, each lobar branch—3, each segmental branch—2, and subsegmental—1 per lobe. Total clot burden score was calculated for both lungs. Next, DECT based perfusion defect (PD) score was calculated using PBV color coded maps. One point was assigned to each wedge shape segmental perfusion defect (Figure 2). For the purpose of simplicity, mottled defects were not included in the calculation of the PD score. A combined score was calculated by addition of clot and PD scores.
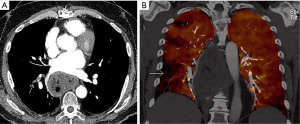
All of the clot burden scoring and DECT based calculation was done by two radiologists, with 2 and 1 year of experience in thoracic imaging. In cases with discrepant readings, thoracic radiologist with 10 years of experience reviewed the cases, and the diagnosis was reached by consensus.
Clinical and hemodynamic data collection
Using CTEPH registry, clinical and hemodynamic parameters typically used for assessment of CTEPH disease severity were collected. Clinical and functional data included functional class as determined by New York Heart Association (NYHA) classification, 6-minute walk distance in feet (6MWD) and % predicted value, and pulmonary function testing parameters such as forced expiratory volume in one second (FEV1) including % of predicted value, forced vital capacity (FVC) including % of predicted value and diffusing capacity of the lung for carbon monoxide (DLCO%). Hemodynamic parameters measured via RHC examination included the pulmonary arterial pressure (PAP) [systolic PAP (sPAP), diastolic PAP (dPAP) and mean PAP (mPAP)], right atrial pressure (RAP), cardiac output (CO) and cardiac index (CI). The PVR was calculated using the following formula: PVR = [mean PAP − PCWP (Pulmonary capillary wedge pressure)]/CO × 80 (dynes-s/cm5). All DECT, clinical, functional and hemodynamic information were obtained as part of a pre-operative evaluation and were measured within approximately a month of each other. In case of 4 patients who had two DECT scans done as preoperative evaluation, we correlated the CT data with the most recent functional and hemodynamic data (closest to the DECT scanning date).
Statistical analysis
The primary aim of the study was to investigate a DECT based score for preoperative evaluation of CTEPH; for this purpose, we studied the association between DECT-based scoring and various clinical/hemodynamic parameters. The associations between 3 DECT-based scores and 15 hemodynamic/clinical parameters were characterized using Spearman’s rank correlation coefficient. A significance level of 0.05 was used. All analyses were performed in R version 3.6.0. Correlation figure made use of the package ‘ggcorrplot’.
Results
This sample consisted of 82 CTs from 78 patients (36 female, 42 male). In this exploratory study, clot burden score, PD score, and combined score all positively correlated with sPAP (rs=0.25, 0.34, 0.34), PVR (rs=0.27, 0.30, 0.34), and mPAP (rs=0.28, 0.31, 0.36) (Table 2, Figures 3,4). Though statistically significant, the magnitude of these correlations were fairly weak. The combined score had the highest magnitude of correlations with the aforementioned parameters. There was no statistically significant correlation of clot burden score, PD score and combined score with functional indices such as 6MWT (rs=0.09, −0.03, 0.03), % predicted 6MWT (rs=−0.10, −0.12, −0.18), FEV1 (rs=0.10, −0.03, 0.04), FEV1% (rs=0.02, −0.14, −0.04), FVC (rs=0.20, 0.14, 0.17), FVC% (rs=0.05, 0.15, 0.06), DLCO% (rs=−0.09, −0.06, −0.13) and NYHA functional class (rs=0.17, 0.14, 0.22).
Table 2
Hemodynamic/clinical parameters | N | Clot burden | Perfusion score | Combined score | |||||
---|---|---|---|---|---|---|---|---|---|
rs | P | rs | P | rs | P | ||||
mPAP | 71 | 0.28 | 0.018* | 0.31 | 0.008* | 0.36 | 0.002* | ||
PVR | 66 | 0.27 | 0.026* | 0.30 | 0.013* | 0.34 | 0.005* | ||
RAP | 76 | −0.02 | 0.881 | 0.08 | 0.493 | 0.04 | 0.731 | ||
sPAP | 73 | 0.25 | 0.030* | 0.34 | 0.003* | 0.34 | 0.003* | ||
dPAP | 73 | 0.13 | 0.287 | 0.22 | 0.058 | 0.19 | 0.100 | ||
CO | 49 | −0.16 | 0.284 | 0.00 | 0.984 | −0.15 | 0.291 | ||
CI | 72 | −0.17 | 0.166 | −0.04 | 0.737 | −0.18 | 0.125 | ||
6MWT | 68 | 0.09 | 0.471 | −0.03 | 0.796 | 0.03 | 0.816 | ||
% predicted | 65 | −0.10 | 0.436 | −0.12 | 0.329 | −0.18 | 0.155 | ||
FEV1 | 76 | 0.10 | 0.397 | −0.03 | 0.787 | 0.04 | 0.763 | ||
FEV1% | 76 | 0.02 | 0.889 | −0.14 | 0.244 | −0.04 | 0.738 | ||
FVC | 76 | 0.20 | 0.083 | 0.14 | 0.217 | 0.17 | 0.141 | ||
FVC% | 76 | 0.05 | 0.668 | 0.15 | 0.193 | 0.06 | 0.593 | ||
DLCO% | 75 | −0.09 | 0.431 | −0.06 | 0.592 | −0.13 | 0.285 | ||
NYHA | 68 | 0.17 | 0.158 | 0.14 | 0.267 | 0.22 | 0.074 |
*, P<0.05. DECT, dual energy computed tomography; rs, correlation coefficient; mPAP, mean pulmonary arterial pressure; PVR, pulmonary vascular resistance; RAP, right atrial pressure; sPAP, systolic pulmonary arterial pressure; dPAP, diastolic pulmonary arterial pressure; CO, cardiac output; CI, cardiac index; 6MWT, 6-minute walk test; FEV1, forced expiratory volume in the first second; FVC, forced vital capacity; DLCO, diffusing capacity of the lung for carbon monoxide; NYHA, New York Heart Association.
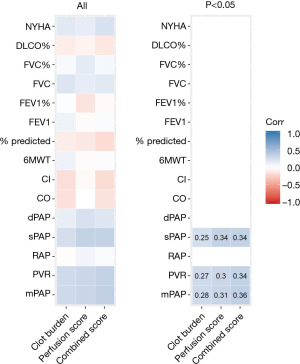
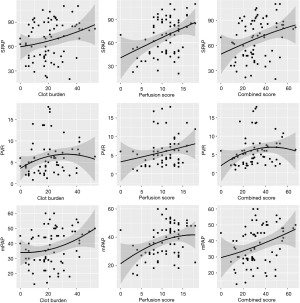
Discussion
In this study, we used a simple DECT based scoring system to noninvasively assess the functional and hemodynamic status in patients with CTEPH. Results demonstrated a positive correlation of all the three scores with sPAP, PVR and mPAP, although the magnitude of correlations was fairly weak. Of the three scores, the combined score had the highest magnitude of correlation.
Diagnosis and evaluation of CTEPH is often not straight forward and requires a significant level of expertise and multidisciplinary collaboration. Once the diagnosis of CTEPH is confirmed, the major questions in the diagnostic algorithm are if the patient is a good surgical candidate, and when to intervene on the patient. Deciding on surgical candidacy is dependent on a lot of factors such as surgically accessible disease, degree of increased PVR, presence or absence of small vessel disease, and presence of comorbidities. Usually, these necessitate a combination of noninvasive tools such as CT and echocardiography, and invasive tests such as RHC and pulmonary angiography. Noninvasive assessment of functional and hemodynamic status can be extremely beneficial especially in long term follow-up, and might obviate repeating invasive procedures. With the ability to provide anatomic and perfusion information on a single scan, DECT seems to be ideally suited for this purpose. So far, a handful of studies have been published that have shown promise in noninvasive assessment of hemodynamics, although with mixed conclusions. In a study on 46 patients, the authors used a 3-point scoring criteria and calculated a lung PBV score (17). The lung PBV score significantly correlated with the PAP (mean, rho =0.48; systolic, rho =0.47; diastolic, rho =0.39), PVR (rho =0.47), and RVP (rho =0.48) (all P<0.01). Multivariable linear regression analyses showed that only the lung PBV score was significantly associated with both the mean PAP and PVR (17). In another study by Hoey et al., they correlated the visual PBV findings and clinical measures of CTEPH severity (18) and did not find significant correlation with the mean PAP or PVR. Another small study on 25 patients used automated quantification values of PBV; these PBV values inversely correlated with systolic (r=−0.64, P=0.001) and mean (r=−0.57, P=0.004) pulmonary arterial pressure (19). There was also a trend for PBV values to inversely correlate with pulmonary vascular resistance (r=−0.20, P=0.35) (19). Similarly, Leone et al. tried to use a CT based score on 145 patients and used the following parameters: unilateral or bilateral disease, main pulmonary artery diameter, mosaic perfusion pattern and tricuspid regurgitation (20). They found a high statistical correlation between the devised CT-score and mPAP and PVR. While these studies do show promise, there are some limitations in these scoring systems. Firstly, some of the scoring models are complex and are not suited for routine clinical practice. Secondly, most of the scoring models have focused either on anatomic disease burden or perfusion information. Hence, we tried to devise a simple scoring system that takes into account both the anatomic and perfusion parameters. Since, we wanted to keep the scoring model simple, we did not include secondary biomarkers of CTEPH such as mosaic attenuation and bronchial artery collaterals (21). Our observations suggest that DECT based scoring does positively correlate with some of the hemodynamic parameters such as sPAP, mPAP and PVR. The combined score had the best magnitude of correlation with the aforementioned parameters highlighting the strength of both anatomic and physiologic information.
This study has some limitations that need to be acknowledged. It is a single center retrospective study with a relatively small sample size. CTEPH is a rare disease, and many patients did not have DECT imaging, and needed to be excluded, hence accounting for the relatively small sample size. Secondly, we did not make adjustments for multiple comparisons, and these results should be interpreted as exploratory. Lastly, we did not take into account a lot of biomarkers that have been discussed in prediction of CTEPH and assessment of severity (21). However, as mentioned above, the goal was to create a simple scoring model that can provide a quick noninvasive functional and hemodynamic assessment.
In conclusion, DECT based scoring system in assessment of severity of CTEPH is feasible, and fairly easy to use. It does correlate with invasive hemodynamic parameters such as sPAP, mPAP and PVR, with combined score having the best magnitude of correlation. This simple scoring model can be used as an ancillary tool in noninvasive preoperative evaluation of CTEPH, and can also be useful in longitudinal follow-up. Further studies with larger patient populations would be helpful in corroborating these observations.
Acknowledgments
Funding: None.
Footnote
Reporting Checklist: The authors have completed the STROBE reporting checklist. Available at https://cdt.amegroups.com/article/view/10.21037/cdt-21-686/rc
Data Sharing Statement: Available at https://cdt.amegroups.com/article/view/10.21037/cdt-21-686/dss
Conflicts of Interest: All authors have completed the ICMJE uniform disclosure form (available at https://cdt.amegroups.com/article/view/10.21037/cdt-21-686/coif). The authors have no conflicts of interest to declare.
Ethical Statement: The authors are accountable for all aspects of the work in ensuring that questions related to the accuracy or integrity of any part of the work are appropriately investigated and resolved. The study was conducted in accordance with the Declaration of Helsinki (as revised in 2013). This retrospective cross-sectional study was performed with Cleveland Clinic institutional review board (IRB) approval and a waiver of individual informed consent. A waiver of informed consent was granted since CT scans were obtained as part of a diagnostic workup with little or no additional risk.
Open Access Statement: This is an Open Access article distributed in accordance with the Creative Commons Attribution-NonCommercial-NoDerivs 4.0 International License (CC BY-NC-ND 4.0), which permits the non-commercial replication and distribution of the article with the strict proviso that no changes or edits are made and the original work is properly cited (including links to both the formal publication through the relevant DOI and the license). See: https://creativecommons.org/licenses/by-nc-nd/4.0/.
References
- Simonneau G, Montani D, Celermajer DS, et al. Haemodynamic definitions and updated clinical classification of pulmonary hypertension. Eur Respir J 2019;53:1801913. [Crossref] [PubMed]
- Moser KM, Bloor CM. Pulmonary vascular lesions occurring in patients with chronic major vessel thromboembolic pulmonary hypertension. Chest 1993;103:685-92. [Crossref] [PubMed]
- Fedullo P, Kerr KM, Kim NH, et al. Chronic thromboembolic pulmonary hypertension. Am J Respir Crit Care Med 2011;183:1605-13. [Crossref] [PubMed]
- Klok FA, van der Hulle T, den Exter PL, et al. The post-PE syndrome: a new concept for chronic complications of pulmonary embolism. Blood Rev 2014;28:221-6. [Crossref] [PubMed]
- Tscholl D, Langer F, Wendler O, et al. Pulmonary thromboendarterectomy--risk factors for early survival and hemodynamic improvement. Eur J Cardiothorac Surg 2001;19:771-6. [Crossref] [PubMed]
- Mayer E, Jenkins D, Lindner J, et al. Surgical management and outcome of patients with chronic thromboembolic pulmonary hypertension: results from an international prospective registry. J Thorac Cardiovasc Surg 2011;141:702-10. [Crossref] [PubMed]
- Mizoguchi H, Ogawa A, Munemasa M, et al. Refined balloon pulmonary angioplasty for inoperable patients with chronic thromboembolic pulmonary hypertension. Circ Cardiovasc Interv 2012;5:748-55. [Crossref] [PubMed]
- Boroto K, Remy-Jardin M, Flohr T, et al. Thoracic applications of dual-source CT technology. Eur J Radiol 2008;68:375-84. [Crossref] [PubMed]
- Kang MJ, Park CM, Lee CH, et al. Dual-energy CT: clinical applications in various pulmonary diseases. Radiographics 2010;30:685-98. [Crossref] [PubMed]
- Karçaaltıncaba M, Aktaş A. Dual-energy CT revisited with multidetector CT: review of principles and clinical applications. Diagn Interv Radiol 2011;17:181-94. [PubMed]
- Pontana F, Faivre JB, Remy-Jardin M, et al. Lung perfusion with dual-energy multidetector-row CT (MDCT): feasibility for the evaluation of acute pulmonary embolism in 117 consecutive patients. Acad Radiol 2008;15:1494-504. [Crossref] [PubMed]
- Le Faivre J, Duhamel A, Khung S, et al. Impact of CT perfusion imaging on the assessment of peripheral chronic pulmonary thromboembolism: clinical experience in 62 patients. Eur Radiol 2016;26:4011-20. [Crossref] [PubMed]
- Renard B, Remy-Jardin M, Santangelo T, et al. Dual-energy CT angiography of chronic thromboembolic disease: can it help recognize links between the severity of pulmonary arterial obstruction and perfusion defects? Eur J Radiol 2011;79:467-72. [Crossref] [PubMed]
- Nakazawa T, Watanabe Y, Hori Y, et al. Lung perfused blood volume images with dual-energy computed tomography for chronic thromboembolic pulmonary hypertension: correlation to scintigraphy with single-photon emission computed tomography. J Comput Assist Tomogr 2011;35:590-5. [Crossref] [PubMed]
- Renapurkar RD, Bolen MA, Shrikanthan S, et al. Comparative assessment of qualitative and quantitative perfusion with dual-energy CT and planar and SPECT-CT V/Q scanning in patients with chronic thromboembolic pulmonary hypertension. Cardiovasc Diagn Ther 2018;8:414-22. [Crossref] [PubMed]
- Nallasamy N, Bullen J, Karim W, et al. Evaluation of Vascular Parameters in Patients With Pulmonary Thromboembolic Disease Using Dual-energy Computed Tomography. J Thorac Imaging 2019;34:367-72. [Crossref] [PubMed]
- Takagi H, Ota H, Sugimura K, et al. Dual-energy CT to estimate clinical severity of chronic thromboembolic pulmonary hypertension: Comparison with invasive right heart catheterization. Eur J Radiol 2016;85:1574-80. [Crossref] [PubMed]
- Hoey ET, Mirsadraee S, Pepke-Zaba J, et al. Dual-energy CT angiography for assessment of regional pulmonary perfusion in patients with chronic thromboembolic pulmonary hypertension: initial experience. AJR Am J Roentgenol 2011;196:524-32. [Crossref] [PubMed]
- Meinel FG, Graef A, Thierfelder KM, et al. Automated quantification of pulmonary perfused blood volume by dual-energy CTPA in chronic thromboembolic pulmonary hypertension. Rofo 2014;186:151-6. [PubMed]
- Leone MB, Giannotta M, Palazzini M, et al. A new CT-score as index of hemodynamic changes in patients with chronic thromboembolic pulmonary hypertension. Radiol Med 2017;122:495-504. [Crossref] [PubMed]
- Lorenz G, Saeedan MB, Bullen J, et al. CT-Based Biomarkers for Prediction of Chronic Thromboembolic Pulmonary Hypertension After an Acute Pulmonary Embolic Event. AJR Am J Roentgenol 2020;215:800-6. [Crossref] [PubMed]