Left ventricular remodeling and systolic function changes in patients with obstructive sleep apnea: a comprehensive contrast-enhanced cardiac magnetic resonance study
Introduction
Obstructive sleep apnea (OSA), characterized by repetitive partial or complete upper respiratory tract obstruction during sleep, is a highly prevalent and under-treated respiratory disorder (1). OSA has emerged as a risk factor for a variety of cardiovascular diseases (CVD) (2), including coronary artery disease, arterial hypertension, heart failure and cardiac arrhythmia (3,4).
OSA is associated with cardiac hypertrophy and left ventricular (LV) dysfunction (5,6). Previous studies have shown that compared to individuals without OSA, patients with OSA have a higher LV mass index (LVMI) (7,8) and elevated odds of developing concentric LV hypertrophy (9). Left ventricular ejection function (LVEF) is a conventional parameter for the evaluation of LV systolic function in both echocardiography and cardiac magnetic resonance (CMR) field, while myocardial deformation analysis could detect more subtle LV systolic function disorders. Some studies have demonstrated that patients with OSA have a lower LVEF than controls (5,10), whereas others have shown these patients have normal LVEF values, and impaired LV deformation ability (11).
CMR is the gold standard imaging technique for cardiac structure, volume, and function measurements. In addition to assessing the cardiac morphology, CMR is a unique tool for characterizing myocardial tissue changes. T1 mapping is an emerging noninvasive technique that quantifies T1 relaxation times. Native T1 and extracellular volume (ECV) provide useful information for measuring diffuse myocardial fibrosis (12,13). Furthermore, T1 mapping can dichotomize myocardial tissue into cellular and extracellular compartments (14-16), which provides a detailed description of cardiac composition. Moreover, the late gadolinium enhancement technique (LGE) not only provides valuable information for the differential diagnosis of cardiomyopathy but also qualifies and quantifies myocardial fibrosis burden. The feature tracking CMR (FT-CMR) technique can generate valuable information about myocardial deformation for subclinical LV systolic function evaluation even in condition when LVEF is normal and correlates well with speckle tracking echocardiography-derived parameters (17). To the best of our knowledge, T1 mapping and FT-CMR techniques have not been previously used to illustrate LV myocardium characteristics and assess changes in systolic function in patients with OSA.
Therefore, the present study aimed to elucidate myocardial tissue characteristics and explore LV systolic function changes in subjects with OSA using a comprehensive contrast-enhanced CMR imaging technique. We present the following article in accordance with the STROBE reporting checklist (available at https://cdt.amegroups.com/article/view/10.21037/cdt-22-38/rc).
Methods
Study design and population
Patients who underwent polysomnography (PSG) for snoring or sleeping problems at the Sleep Center of Guangdong Provincial People’s Hospital between March 2019 and February 2021 were recruited. The inclusion criteria were as follows: (I) 18–65 years of any sex; (II) first-time examination with PSG. After a brief echocardiography test and detailed clinical inquiry, patients with any of the following conditions were excluded: (I) previous history of major CVD, including coronary artery disease, congenital heart disease, cardiomyopathy, moderate-to-severe cardiac valve disease, heart failure, and significant arrhythmia; (II) lung disease or other sleep disorders; (III) diabetes mellitus or other metabolic diseases; and (IV) any contraindications to CMR or allergy to gadolinium-based contrast agents. Twenty age- and sex-matched healthy participants who had never complained of snoring or sleep problems were also enrolled as controls.
All patients enrolled in this case-control study underwent overnight PSG, blood pressure measurement, and CMR examination. CMR scans were performed within 1 week of PSG examination. Blood sampling for hematocrit measurement was performed on the same day as the CMR scan. The study was conducted in accordance with the Declaration of Helsinki (as revised in 2013) and was approved by the Institutional Review Board of Guangdong Provincial People’s Hospital (ethical code: 2017079H[R1]). Written informed consent was obtained from each patient before CMR imaging.
PSG parameters
All patients underwent full-night PSG examination using the Compumedics Grael HD-PSG system (Compumedics, Abbotsford, Victoria, Australia). Surface electrodes incorporated six-channel electroencephalography, electrooculography, submental electromyography, and electrocardiography were performed. Chest and abdominal belts were used to record respiratory movements. Airflow was monitored with an oronasal transducer, and arterial oxygen saturation was measured using finger pulse oximetry. According to the standard criteria of the American Academy of Sleep Medicine (AASM) (18), apnea was defined as a ≥90% drop in airflow lasting for >10 s. Hypopnea was defined as a ≥30% decrease in airflow for at least 10 s from the baseline level accompanied by a ≥3% reduction in arterial oxygen saturation or arousal. The apnea-hypopnea index (AHI) was calculated as the average number of apneas and hypopneas per hour of sleep. Based on AHI, patients were divided into non-OSA (AHI <5 events/h), mild-to-moderate OSA (5≤ AHI ≤30 events/h), and severe OSA (AHI >30 events/h) groups. The oxygen desaturation index (ODI), overall arousal index, nocturnal average oxygen saturation (A-SPO2) and minimum oxygen saturation (M-SPO2) and sleep duration were also recorded.
CMR imaging
CMR acquisition
All participants underwent a standard CMR imaging protocol using a 3.0 T clinical scanner (Ingenia, Philips Medical Systems, Best, the Netherlands) with 32 coil elements.
LV volume, function, and structure were assessed using a stack of short-axis electrocardiogram (ECG)-gated balanced steady-state free precession (bSSFP) cine sequences with whole ventricle coverage. Long-axis planes (including two-, three-, and four-chamber views) were obtained by employing the same sequences. The FT-CMR technique is based on bSSFP cine images. For LGE imaging, a stack of short-axis images by phase-sensitive inversion recovery sequence was acquired approximately 10–11 min after contrast administration to detect patients with any pattern of delayed enhancement. The detailed parameters for cine and LGE sequences can be found in Supplementary Material 1 (Appendix 1). T1 mapping images were obtained using a 5s(3s)3s scheme for native T1 at pre-contrast, and a 4s(1s)3s(1s)2s scheme for post T1 15–17 min after administration of contrast agent with the modified Look-Locker inversion recovery sequences in a single short-axis view of the mid-ventricle (12). The imaging parameters were as follows: field of view, 230 mm × 230 mm; voxels, 2 mm × 2 mm × 8 mm; sense factor, 2; minimum inversion time, 105 ms; flip angle, 20°.
CMR image analysis
All CMR image analyses were performed using a commercially validated software (version 3.2, Medis, Leiden, the Netherlands). LV structure [LV mass (LVM), LVMI, maximal wall thickness (MWT), and LVM/volume ratio (LVMVR)], LV volume, and global function parameters were acquired. The software was employed to delineate the endocardial and epicardial borders in the end-diastolic phase of the LVM calculation with papillary muscle exclusion.
For FT analysis, global circumferential strain (GCS), GCS rate, and global radial strain (GRS) and GRS rate analyses were derived by sketching endocardial and epicardial borders on the basal, middle, and apical planes of the LV short-axis view, while the global longitudinal strain (GLS) and GLS rate were obtained from the two-, three-, and four-chamber views of the LV. The FT-CMR protocol is described in Supplementary Material 1 (Appendix 1).
T1 mapping images were analyzed using the QMapECV ver. 2.2.18 (Medis) workstation by contouring the endocardial and epicardial borders on the entire mid-ventricle (Figure 1). Additionally, a region of interest was placed in the LV cavity for quantitation of blood pool T1 values without containing the papillary muscle. Myocardial tissue was divided into cell and matrix components using ECV (%) which was calculated as follows:
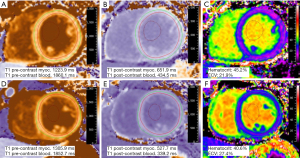
Two components of myocardium tissue calculation:
Inter- and intraobserver variability
Inter- and intraobserver variabilities for T1 mapping measurements were tested in randomly selected 20 participants of all by two experienced radiologists. One observer measured the interobserver variability once, and the second blinded to the results and clinical information measured the intraobserver variability twice at two time points at an interval of 2 weeks.
Statistical analysis
Statistical analyses were performed using SPSS (version 19.0, IBM Inc., Chicago, IL, USA) and GraphPad Prism software (version 8.3, San Diego, California, USA). All patients were classified into non-OSA, mild-moderate OSA, and severe OSA groups. After normality assessment using the Shapiro-Wilk test, normally distributed continuous variables are expressed as mean ± standard deviation, whereas skewed variables are presented as median [interquartile range (IQR)]. Categorical variables are reported as number and frequency (%). Comparisons between all OSA and non-OSA groups were performed using Student’s t-test, Mann-Whitney U test, Fisher’s exact test, or chi-square test, as appropriate. Three-group comparisons were performed by one-way analysis of variance test with post-hoc Bonferroni testing; the Kruskal-Wallis test with Mann-Whitney U for multiple comparisons; the Fisher’s exact test or chi-square test, as appropriate. The AHI and LV structure parameter correlations were assessed using Spearman’s correlation after the normality test. To determine the significant impact of OSA on LV structural parameters, including LVMI, LVMVR, and iCV, multiple linear regression analyses were performed in patients with two different models after adjustment for confounders, including age, sex, body mass index (BMI), smoking history, hyperlipidemia, and blood pressure, which were displayed either as systolic blood pressure (SBP) or hypertension. Smoking history and hyperlipidemia variables were excluded from the univariate correlation analyses. Intra- and interobserver reliabilities were tested using Bland-Altman plot analysis and intra-class correlation coefficients. Differences were considered statistically significant at two-sided P values <0.05.
Results
A total of 519 patients underwent PSG examination during the study period. Of these, 56 patients completed both PSG and CMR scans. Of the five patients that were excluded from the CMR criteria, one exhibited delayed subendocardial enhancement for a suspected myocardial infarction. Eventually, 32 patients with OSA and 19 non-OSA were included in the analysis (Figure 2). OSA patients were further divided into 13 mild-to-moderate and 19 severe OSA groups.
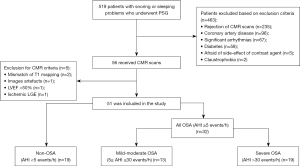
Population characteristics
Basic patients’ characteristics and PSG parameters are presented in Tables 1,2, respectively. The patients were predominantly middle-aged males; participants with mild-moderate OSA were significantly older than those with severe OSA (47.8±9.4 vs. 39.0±10.0 years, P<0.05). Patients with OSA had slightly elevated BMI (P<0.05) than non-OSA patients, especially in the severe OSA group. Eleven patients with OSA (34.4%) also had hypertension. The OSA group had a longer duration of snoring [median 9.5 (4.0 to 16.8) vs. median 1.5 years (0.1 to 4.5), P<0.001] than the non-OSA group. Besides AHI, OSA patients had significantly lower A-SPO2 and M-SPO2 and higher ODI values (P<0.001).
Table 1
Variables | All OSA (n=32) | Non-OSA (n=19) | Mild-moderate OSA (n=13) | Severe OSA (n=19) | P value1 | P value2 |
---|---|---|---|---|---|---|
Age (years) | 42.5±10.6 | 40.7±8.0 | 47.8±9.4 | 39.0±10.0∆ | 0.515 | 0.029 |
Male | 26 (81.3) | 12 (63.2) | 9 (69.2) | 17 (89.5) | 0.192 | 0.172 |
BMI (kg/m2) | 26.7±3.6 | 24.1±2.6 | 26.2±3.7 | 26.8±3.5* | 0.010 | 0.032 |
BSA (m2) | 1.88±0.18 | 1.76±0.16 | 1.86±0.20 | 1.90±0.17 | 0.020 | 0.053 |
Hypertension | 11 (34.4) | 0 | 3 (23.1) | 8 (42.1) | – | 0.003 |
SBP (mmHg) | 126.6±13.6 | 120.0±7.1 | 126.6±10.2 | 126.5±15.8 | 0.027 | 0.162 |
DBP (mmHg) | 84.1±10.8 | 81.2± 6.2 | 84.0±10.9 | 84.2±11.0 | 0.280 | 0.560 |
Medication | ||||||
Beta-blocker | 7 (63.6) | 0 | 1 (33.3) | 6 (75.0) | – | – |
Calcium blocker | 8 (72.7) | 0 | 3 (100.0) | 5 (62.5) | – | – |
ACE inhibitor/ARB | 4 (36.4) | 0 | 0 | 4 (50.0) | – | – |
Heart rate (beats/min) | 74.9±10.9 | 71.3±8.8 | 74.5±11.3 | 75.2±11.0 | 0.233 | 0.486 |
Hyperlipidemia | 19 (59.4) | 12 (63.2) | 7 (53.8) | 12 (63.2) | 0.789 | 0.838 |
Smoking history | 6 (18.8) | 2 (10.5) | 1 (7.7) | 5 (26.3) | 0.694 | 0.420 |
Hematocrit (%) | 43.2±3.4 | 42.1±3.6 | 43.0±3.8 | 43.3±3.2 | 0.311 | 0.590 |
Duration of snoring (years) | 9.5 [4.0, 16.8] | 1.5 [0.1, 4.5] | 10 [1.0, 20.5]# | 9 [4.5, 16.0]* | <0.001 | <0.001 |
PSG parameters | ||||||
AHI (events/h) | 36.7±20.1 | 1.9±1.2 | 16.0±4.7# | 51.0±12.6*∆ | <0.001 | <0.001 |
ODI (events/h) | 28.7 [16.0, 49.9] | 1.2 [0.8, 1.9] | 16 [11.4, 18.0]# | 44.1 [32.9, 54.9]*∆ | <0.001 | <0.001 |
Overall arousal index | 20.3 [14.1, 27.9] | 16.9 [9.9, 23.9] | 22.7 [12.3, 46.0] | 19.2 [13.8, 24.1] | 0.079 | 0.174 |
A-SPO2 (%) | 94 [93, 95] | 96 [96, 97] | 95 [94, 96]# | 93 [91, 95]*∆ | <0.001 | <0.001 |
M-SPO2 (%) | 77 [71, 83] | 90 [89, 92] | 81 [74, 85]# | 73 [66, 82]* | <0.001 | <0.001 |
Sleep duration (min) | 413 [390, 453] | 448 [390, 496] | 396 [349, 429]# | 428 [396, 456] | 0.102 | 0.073 |
Data are mean ± standard deviation for normally distributed continuous variables, median [interquartile range] for skewed variables, and n (%) for binary variables. 1, comparation between all OSA and the non-OSA group; 2, comparation within the non-OSA, the mild-moderate OSA and the severe OSA groups; *, P<0.05 between the severe OSA and the non-OSA groups; ∆, P<0.05 between the severe OSA and the mild-moderate OSA groups; #, P<0.05 between the mild-moderate OSA and the non-OSA groups. OSA, obstructive sleep apnea; BMI, body mass index; BSA, body surface area; SBP, systolic blood pressure; DBP, diastolic blood pressure; PSG, polysomnography; ACE, angiotensin-converting enzyme; ARB, angiotensin receptor blocker; AHI, apnea-hypopnea index; ODI, oxygen desaturation index; A-SPO2, average oxygen saturation; M-SPO2, minimum oxygen saturation.
Table 2
Variables | Healthy controls (n=20) | Non-OSA (n=19) | All OSA (n=32) | P value |
---|---|---|---|---|
Age (years) | 41.8±11.0 | 40.7±8.0 | 42.5±10.6 | 0.820 |
Male | 15 (75.0) | 12 (63.2) | 26 (81.3) | 0.343 |
BMI (kg/m2) | 23.8±2.9 | 24.1±2.6 | 26.7±3.6* | 0.003 |
BSA (m2) | 1.77±0.14 | 1.76±0.16 | 1.88±0.18 | 0.015 |
Hypertension (%) | 0 (0) | 0 (0) | 11 (34.4) | – |
SBP (mmHg) | 119.6±6.9 | 120.0±7.1 | 126.6±13.6 | 0.031 |
DBP (mmHg) | 74.1±7.4 | 81.2±6.2# | 84.1±10.8* | 0.001 |
Heart rate (beats/min) | 70.4±11.1 | 71.3±8.8 | 74.9±10.9 | 0.268 |
Hyperlipidemia | 0 (0) | 12 (63.2) | 19 (59.4) | – |
Smoking history | 0 (0) | 2 (10.5) | 6 (18.8) | – |
Hematocrit (%) | 41.6±1.3 | 42.1±3.6 | 43.2±3.4 | 0.194 |
Data are mean ± standard deviation for normally distributed continuous variables and n (%) for binary variables. P value denotes comparation within the healthy controls, the non-OSA group and all OSA patients; *, P<0.05 between all OSA and healthy controls; #, P<0.05 between the non-OSA group and healthy controls. OSA, obstructive sleep apnea; BMI, body mass index; BSA, body surface area; SBP, systolic blood pressure; DBP, diastolic blood pressure.
The demographics and sleep data of the study participants included in Supplementary Material 1 (Appendix 1) are displayed according to two additional grouping methods: hypertension (Table S1) and obesity (Table S2). Based on the hypertension and OSA conditions (Table S1), OSA patients with or without hypertension had a higher BMI than those without these two conditions (P<0.05). In Table S2, participates were grouped into three groups with BMI ≥25 kg/m2 as the boundary. The age of patients in the OSA-without-obese group was higher than those in other groups (P<0.05).
CMR results
CMR findings are presented in Tables 3,4. There was no significant difference in LV systolic function as reflected by LVEF, LV stroke volume index (LVSVi), LV cardiac index (LVCi), LV strain (GLS, GCS, and GRS), and strain rate indices between patients with non-OSA, mild-to-moderate OSA, and severe OSA. However, compared with healthy controls, patients with OSA had a slightly decreased LVSVi and GCS (P<0.05), while LVEF was preserved. Patients with OSA had significantly higher LV remodeling parameters than those without OSA with respect to LVMI (21.0±3.8 vs. 16.4±3.1 g/m2.7, P<0.001), MWT (P=0.008), and LVMVR (P=0.001) (Table 3 and Figure 3). There were no significant differences in the conventional right ventricular CMR parameters between the OSA and non-OSA groups.
Table 3
Variables | All OSA (n=32) | non-OSA (n=19) | Mild-moderate OSA (n=13) | Severe OSA (n=19) | P value1 | P value2 |
---|---|---|---|---|---|---|
Function and structure | ||||||
LVEDVi (mL/m2) | 77.4±7.4 | 78.6±10.1 | 76.8±6.3 | 77.8±8.2 | 0.637 | 0.846 |
LVESVi (mL/m2) | 30.3±5.9 | 30.9±7.2 | 29.8±7.0 | 30.7±5.1 | 0.763 | 0.785 |
LVEF (%) | 61.0±5.8 | 61.1±5.1 | 61.4±7.5 | 60.7±4.5 | 0.949 | 0.931 |
LVSVi (mL/m2) | 47.1±5.5 | 47.7±4.9 | 47.0±5.6 | 47.2±5.6 | 0.685 | 0.924 |
LVCi (L/min per m2) | 3.3 [2.9, 3.5] | 3.2 [3.0, 3.5] | 3.4 [3.1, 3.7] | 3.2 [2.9, 3.6] | 0.785 | 0.655 |
LVM (g) | 86.5±18.5 | 66.5±18.4 | 80.8±19.0 | 90.4±19.6* | <0.001 | 0.001 |
LVMI (g/m2.7) | 21.0±3.8 | 16.4±3.1 | 19.7±3.0# | 21.9±4.0* | <0.001 | <0.001 |
LVMVR (g/mL) | 0.59 [0.53, 0.66] | 0.45 [0.39, 0.52] | 0.58 [0.47, 0.64]# | 0.59 [0.54, 0.68]* | 0.001 | 0.002 |
MWT (mm) | 10.9±2.0 | 9.7±1.2 | 10.3±1.9 | 11.3±2.0* | 0.008 | 0.016 |
RVEDVi (mL/m2) | 83.8±14.3 | 83.2±11.8 | 77.1±10.6 | 88.4±14.9 | 0.863 | 0.056 |
RVESVi (mL/m2) | 40.8±10.6 | 40.6±8.1 | 36.1±7.8 | 44.1±11.1 | 0.936 | 0.066 |
RVEF (%) | 50.3 [47.4, 56.8] | 50.2 [47.4, 53.3] | 51.3 [49.3, 61.0] | 48.7 [45.8, 53.4] | 0.922 | 0.272 |
RVSVi (mL/m2) | 43.0±7.4 | 42.6±6.0 | 41.1±6.3 | 44.4±7.9 | 0.824 | 0.404 |
RVCi (L/min per m2) | 3.2±0.6 | 3.0±0.3 | 3.1±0.8 | 3.3±0.5 | 0.137 | 0.134 |
T1 mapping parameters | ||||||
Native T1 (ms) | 1,254 [1,234, 1,262] | 1,257 [1,237, 1,280] | 1,261 [1,245, 1,272] | 1,243 [1,224, 1,257]∆ | 0.360 | 0.066 |
Post T1 (ms) | 606.9±41.0 | 591.0±44.0 | 593.8±43.3 | 615.9±37.9 | 0.199 | 0.152 |
ECV (%) | 24.4±1.9 | 26.2±2.5 | 24.4±1.5 | 24.5±2.1* | 0.006 | 0.022 |
iECV (mL/m2.7) | 4.9±0.8 | 4.1±0.6 | 4.6±0.6 | 5.1±0.9* | 0.001 | 0.005 |
iCV (mL/m2.7) | 15.1±2.9 | 11.6±2.4 | 14.2±2.3# | 15.8±3.1* | <0.001 | <0.001 |
iCV-iECV (mL/m2.7) | 10.3±2.2 | 7.5±2.0 | 9.6±1.8# | 10.7±2.4* | <0.001 | <0.001 |
LV strain | ||||||
GLS (%) | −21.4 [−23.7, −20.2] | −22.5 [−23.9, −21.8] | −21.3 [−24.3, −20.0] | −21.5 [−23.4, −20.3] | 0.141 | 0.321 |
GCS (%) | −20.0±2.9 | −21.5±2.8 | −20.3±3.9 | −19.7±2.0 | 0.056 | 0.132 |
GRS (%) | 78.3±21.1 | 93.3±31.2 | 79.3±21.9 | 77.6±21.2 | 0.074 | 0.138 |
GLS rate (s−1) | −1.0 [−1.1, −0.9] | −1.0 [−1.1, −1.0] | −1.0 [−1.1, −0.9] | −1.0 [−1.1, −0.9] | 0.295 | 0.578 |
GCS rate (s−1) | −1.0 [−1.1, −0.9] | −1.0 [−1.2, −0.9] | −1.0 [−1.1, −0.9] | −1.0 [−1.1, −0.9] | 0.348 | 0.642 |
GRS rate (s−1) | 2.3±0.5 | 2.3±0.5 | 2.3±0.5 | 2.3±0.5 | 0.956 | 0.993 |
Data are mean ± standard deviation for normally distributed continuous variables and median [interquartile range] for skewed variables. 1, comparation between all OSA and the non-OSA group; 2, comparation within the non-OSA, the mild-moderate OSA and the severe OSA groups; *, P<0.05 between the severe OSA and the non-OSA group; ∆, P<0.05 between the severe OSA and the mild-moderate OSA groups; #, P<0.05 between the mild-moderate OSA and the non-OSA group. CMR, cardiac magnetic resonance; OSA, obstructive sleep apnea; LV, left ventricle; RV, right ventricle; LVEDVi, LV end-diastolic volume index; LVESVi, LV end-systolic volume index; LVEF, LV ejection fraction; SVi, stroke volume index; Ci, cardiac index; LVM, LV mass; LVMI, LV mass indexed to height2.7; LVMVR, left ventricular mass/volume ratio; MWT, maximal wall thickness; ECV, extracellular volume; iECV, indexed extracellular volume; iCV, indexed cellular volume; GLS, global longitudinal strain; GCS, global circumferential strain; GRS, global radial strain.
Table 4
Variables | Healthy controls (n=20) | Non-OSA (n=19) | All OSA (n=32) | P value |
---|---|---|---|---|
Function and structure | ||||
LVEDVi (mL/m2) | 82.3±9.2 | 78.6±10.1 | 77.4±7.4 | 0151 |
LVESVi (mL/m2) | 30.1±6.8 | 30.9±7.2 | 30.3±5.9 | 0.934 |
LVEF (%) | 62.8±6.4 | 61.1±5.1 | 61.0±5.8 | 0.506 |
LVSVi (mL/m2) | 52.1±7.3 | 47.7±4.9 | 47.1±5.5* | 0.012 |
LVCi (L/min per m2) | 3.6 [3.2, 3.9] | 3.2 [3.0, 3.5]# | 3.3 [2.9, 3.5] | 0.048 |
LVM (g) | 65.8±13.6 | 66.5±18.4 | 86.5±18.5* | <0.001 |
LVMI (g/m2.7) | 16.3±3.2 | 16.4±3.1 | 21.0±3.8* | <0.001 |
LVMVR (g/mL) | 0.44 [0.39, 0.52] | 0.45 [0.39, 0.52] | 0.59 [0.53, 0.66]* | <0.001 |
MWT (mm) | 9.7±1.4 | 9.7±1.2 | 10.9±2.0* | 0.011 |
RVEDVi (mL/m2) | 77.6±14.1 | 83.2±11.8 | 83.8±14.3 | 0.248 |
RVESVi (mL/m2) | 35.3±9.5 | 40.6±8.1 | 40.8±10.6 | 0.109 |
RVEF (%) | 54.7 [48.1, 60.3] | 50.2 [47.4, 53.3] | 50.3 [47.4, 56.8] | 0.167 |
RVSVi (mL/m2) | 42.3±8.4 | 42.6±6.0 | 43.0±7.4 | 0.936 |
RVCi (L/min per m2) | 3.0±0.8 | 3.0±0.3 | 3.2±0.6 | 0.367 |
T1 mapping parameters | ||||
Native T1 (ms) | 1,281 [1,258, 1,294] | 1,257 [1,237, 1,280]# | 1,254 [1,234, 1,262]* | 0.002 |
Post T1 (ms) | 565.3±59.9 | 591.0±44.0 | 606.9±41.0* | 0.013 |
ECV (%) | 26.5±2.3 | 26.2±2.5 | 24.4±1.9* | 0.002 |
iECV (mL/m2.7) | 4.1±0.8 | 4.1±0.6 | 4.9±0.8* | <0.001 |
iCV (mL/m2.7) | 11.4±2.3 | 11.6±2.4 | 15.1±2.9* | <0.001 |
iCV-iECV (mL/m2.7) | 7.3±1.7 | 7.5±2.0 | 10.3±2.2* | <0.001 |
LV strain | ||||
GLS (%) | −24.1 [−25.6, −20.5] | −22.5 [−23.9, −21.8] | −21.4 [−23.7, −20.2] | 0.077 |
GCS (%) | −24.6±3.5 | −21.5±2.8# | −20.0±2.9* | <0.001 |
GRS (%) | 101.7±30.1 | 93.3±31.2 | 78.3±21.1* | 0.009 |
GLS rate (s−1) | −1.0 [−1.1, −0.8] | −1.0 [−1.1, −1.0] | −1.0 [−1.1, −0.9] | 0.469 |
GCS rate (s−1) | −1.0 [−1.1, −0.8] | −1.0 [−1.2, −0.9] | −1.0 [−1.1, −0.9] | 0.628 |
GRS rate (s−1) | 2.0±0.5 | 2.3±0.5 | 2.3±0.5 | 0.046 |
Data are mean ± standard deviation for normally distributed continuous variables and median [interquartile range] for skewed variables. P denotes comparation within healthy controls, the non-OSA group and all OSA patients; *, P<0.05 between all OSA and healthy controls; #, P<0.05 between the non-OSA group and healthy controls. CMR, cardiac magnetic resonance; OSA, obstructive sleep apnea; LV, left ventricle; RV, right ventricle; LVEDVi, LV end-diastolic volume index; LVESVi, LV end-systolic volume index; LVEF, LV ejection fraction; SVi, stroke volume index; Ci, cardiac index; LVM, LV mass; LVMI, LV mass indexed to height2.7; LVMVR, left ventricular mass/volume ratio; MWT, maximal wall thickness; ECV, extracellular volume; iECV, indexed extracellular volume; iCV, indexed cellular volume; GLS, global longitudinal strain; GCS, global circumferential strain; GRS, global radial strain.
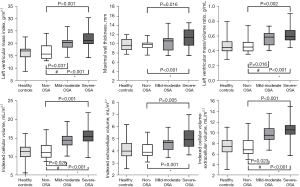
Focal replacement fibrosis was not detected by LGE in any of the participants following assessment by two experienced radiologists. Regarding T1 mapping-derived parameters, the severe OSA group had lower native T1 relaxation times than the mild-to-moderate OSA group (P<0.05). Interestingly, compared to non-OSA patients and healthy controls, a decrease in ECV was seen in OSA patients (24.4%±1.9% vs. 26.2%±2.5%, P=0.006; 24.4%±1.9% vs. 26.5%±2.3%, P=0.004). This observation was similar to that noted in the severe OSA group in comparison with the non-OSA group. With respect to iCV and iCV-iECV values, the non-OSA group had lower values and the severe OSA had the highest (Table 3 and Figure 3). In the OSA group, the expansion of the cellular component was greater than that of the extracellular component, as demonstrated by the values of iCV-iECV.
The CMR data has been displayed in greater detail in Supplementary Material 1 (Appendix 1); wherein our cohort has been divided according to two additional grouping methods, as mentioned above. Based on OSA and hypertension states (Table S3), all patients were divided into three groups: no-OSA-and-no-hypertension, OSA-without-hypertension, and OSA-with-hypertension groups. LV structural parameter values were greater in the OSA with and without hypertension group than in controls without these two conditions. LVMI was 16.4±3.1 g/m2.7 for control subjects, 19.7±3.4 g/m2.7 for the OSA-without-hypertension group, and 23.4±3.3 g/m2.7 for the OSA-with-hypertension group (P<0.001). The ECV was lower in the OSA with and without-hypertension groups. iCV and iCV-iECV values increased in the OSA-without-hypertension group, and a further significant increase was observed in the OSA-with-hypertension group (P<0.05). When patients were grouped by OSA and obese states (Table S4), LV structural parameters were also higher in OSA with or without obese than in controls, especially in the OSA-with-obese group. The increase/decrease patterns of ECV, iCV, and iCV-iECV values (Table S4) were similar to the above grouping method.
Factors related to LV remodeling indexes
In the correlation analysis, AHI values were found to correlate well with LVMI, LVMVR, iCV, and iCV-iECV values (Figure 4). Multiple linear regression analysis was performed to determine the association between OSA and LV remodeling parameters after adjusting for confounders. As blood pressure is a vital factor of LV hypertrophy, SBP values (Table 5) and hypertension (Table 6) were the analyzed factors that could influence LV remodeling parameters. As shown in Table 5, the independent factors that correlated with LVMI were OSA (β=0.348, P=0.004), male sex (β=0.292, P=0.009), BMI (β=0.252, P=0.026), and SBP (β=0.226, P=0.037). Table 6 shows that the independent correlated factors for LVMI were the same (OSA, β=0.233 and P=0.048; male sex, β=0.297 and P=0.005; BMI, β=0.274 and P=0.01; having hypertension β=0.363, P=0.001). Multiple linear regression analysis also showed significant associations between OSA and LVMVR (β=0.392, P=0.003; Table 5) and iCV (β=0.337, P=0.004; Table 5 and β=0.231, P=0.047; Table 6).
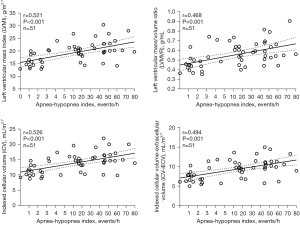
Table 5
Dependent variables | Independent variables | Unadjusted | Multivariate adjusted | |||
---|---|---|---|---|---|---|
r | P value | β | P value | |||
LVMI | ||||||
Age (years) | −0.151 | 0.289 | – | – | ||
Sex (0= female, 1= male) | 0.431 | 0.001 | 0.292 | 0.009 | ||
BMI (kg/m2) | 0.461 | 0.001 | 0.252 | 0.026 | ||
SBP (mmHg) | 0.345 | 0.013 | 0.226 | 0.037 | ||
Hyperlipidemia (0= no, 1= yes) | 0.259 | 0.066 | – | – | ||
OSA grade | 0.560 | <0.001 | 0.348 | 0.004 | ||
LVMVR | ||||||
Age (years) | −0.170 | 0.233 | – | – | ||
Sex (0= female, 1= male) | 0.434 | 0.001 | 0.312 | 0.015 | ||
BMI (kg/m2) | 0.399 | 0.004 | – | – | ||
SBP (mmHg) | 0.279 | 0.047 | – | – | ||
Hyperlipidemia (0= no, 1= yes) | 0.248 | 0.079 | – | – | ||
OSA grade | 0.492 | <0.001 | 0.392 | 0.003 | ||
iCV | ||||||
Age (years) | −0.140 | 0.329 | – | – | ||
Sex (0= female, 1= male) | 0.474 | <0.001 | 0.326 | 0.003 | ||
BMI (kg/m2) | 0.472 | <0.001 | 0.260 | 0.019 | ||
SBP (mmHg) | 0.318 | 0.023 | 0.218 | 0.039 | ||
Hyperlipidemia (0= no, 1= yes) | 0.289 | 0.040 | – | – | ||
OSA grade | 0.571 | <0.001 | 0.337 | 0.004 |
Multiple linear regression analyses were applied in patients after adjustment for clinically relevant factors including age, sex, BMI, SBP, smoking history and hyperlipidemia. LV structure parameters were as the dependent variables including LVMI, LVMVR, and iCV. LV, left ventricle; LVMI, LV mass indexed to height2.7; LVMVR, left ventricular mass/volume ratio; iCV, indexed cellular volume; BMI, body mass index; OSA, obstructive sleep apnea; SBP, systolic blood pressure.
Table 6
Dependent variables | Independent variables | Unadjusted | Multivariate adjusted | |||
---|---|---|---|---|---|---|
r | P value | β | P value | |||
LVMI | ||||||
Age (years) | −0.151 | 0.289 | – | – | ||
Sex (0= female, 1= male) | 0.431 | 0.001 | 0.297 | 0.005 | ||
BMI (kg/m2) | 0.461 | 0.001 | 0.274 | 0.010 | ||
Hypertension (0= no, 1= yes) | 0.541 | <0.001 | 0.363 | 0.001 | ||
Hyperlipidemia (0= no, 1= yes) | 0.259 | 0.066 | – | – | ||
OSA grade | 0.560 | <0.001 | 0.233 | 0.048 | ||
LVMVR | ||||||
Age (years) | −0.170 | 0.233 | – | – | ||
Sex (0= female, 1= male) | 0.434 | 0.001 | 0.321 | 0.010 | ||
BMI (kg/m2) | 0.399 | 0.004 | – | – | ||
Hypertension (0= no, 1= yes) | 0.453 | 0.001 | 0.282 | 0.033 | ||
Hyperlipidemia (0= no, 1= yes) | 0.248 | 0.079 | – | – | ||
OSA grade | 0.492 | <0.001 | 0.266 | 0.050 | ||
iCV | ||||||
Age (years) | −0.140 | 0.329 | – | – | ||
Sex (0= female, 1= male) | 0.474 | <0.001 | 0.331 | 0.002 | ||
BMI (kg/m2) | 0.472 | <0.001 | 0.282 | 0.008 | ||
Hypertension (0= no, 1= yes) | 0.512 | <0.001 | 0.338 | 0.002 | ||
Hyperlipidemia (0= no, 1= yes) | 0.289 | 0.040 | – | – | ||
OSA grade | 0.571 | <0.001 | 0.231 | 0.047 |
Multiple linear regression analyses were applied in patients after adjustment for clinically relevant factors including age, sex, BMI, hypertension, smoking history and hyperlipidemia. LV structure parameters were as the dependent variables including LVMI, LVMVR, and iCV. LV, left ventricle; LVMI, LV mass indexed to height2.7; LVMVR, left ventricular mass/volume ratio; iCV, indexed cellular volume; BMI, body mass index; OSA, obstructive sleep apnea.
Reproducibility of T1 mapping
Intraobserver and interobserver reliabilities were very good for the T1 mapping parameters in 20 randomly selected patients (Table 7).
Table 7
Variables | Reproducibility | Bias | 95% CI | ICC | 95% CI |
---|---|---|---|---|---|
Native T1 (ms) | Intra-observer variability | −1.6 | −4.7, 1.4 | 0.983 | 0.959, 0.993 |
Inter-observer variability | −8.8 | −19.5, 1.8 | 0.809 | 0.576, 0.920 | |
Post T1 (ms) | Intra-observer variability | 0.1 | −3.5, 3.7 | 0.984 | 0.960, 0.994 |
Inter-observer variability | 1.7 | −1.0, 4.4 | 0.990 | 0.976, 0.996 | |
ECV (%) | Intra-observer variability | 0.1 | −0.1, 0.3 | 0.982 | 0.956, 0.993 |
Inter-observer variability | −0.2 | −0.6, 0.2 | 0.930 | 0.835, 0.971 |
Reproducibility analysis was performed by Bland-Altman analyses and ICCs. Results were expressed as bias, ICC, and 95% CI, respectively. CI, confidence interval; ICC, intraclass correlation coefficients; ECV, extracellular volume.
Discussion
In the present study, we took advantage of comprehensive contrast-enhanced CMR imaging in patients with OSA to determine LV systolic function and myocardial tissue characteristics. Our main finding was that patients with OSA have elevated LVMI, which is mainly derived from the expansion of the cellular compartment. We also noted that LV subclinical systolic function was slightly decreased in these patients, whereas LVEF was preserved.
The impact of OSA on cardiac consequences is intricate. Multiple underlying pathophysiological mechanisms account for the impairment of cardiac function and structure in OSA remained uncertain. Intermittent nocturnal hypoxia is believed to be the initial trigger that causes hyperactivity of the sympathetic nervous system, oxidative stress, inflammatory cascade reactions, endothelial dysfunction, and arterial stiffness (19-21). These factors work together and eventually increase the cardiac afterload. Moreover, the collapse of the upper airway causes OSA patients to forcibly inhale during sleep, which increases the intrathoracic pressure; consequently, more blood flows in the right heart system, and the ventricular septum shifts to the left during diastole, ultimately leading to a reduction in left ventricle output. Therefore, there is no doubt that OSA causes the pathological cardiac hypertrophy, rather than the physiological cardiac hypertrophy. In fact, the aforementioned mechanisms could not account for the changes in LV structure and myocardial characteristics as observed in OSA, for the results have not been explored and illustrated previously.
With the aid of CMR imaging, T1 mapping, and the LGE technique, myocardial fibrosis with disease processes can be detected. Many studies have demonstrated that native T1 and ECV values derived from T1 mapping that reflect diffuse myocardial fibrosis correlate well with histological results (13). LGE represents the replaced fibrosis, which is a powerful prognostic predictor for major adverse cardiovascular events. However, contrary to previous studies, OSA does not result in focal fibrosis, as LGE could detect and diffuse myocardial fibrosis as reflected by elevated ECV. A PubMed database search showed only four studies related to cardiac fibrosis in OSA which were associated with increased fibrosis in the left ventricle or left atrium (22-25). However, instead of enrolled “purely” OSA patients, these studies focused on hypertrophic obstructive cardiomyopathy, atrial fibrillation patients or community-based cohort of the multi-ethnic study of atherosclerosis patients. Moreover, the study cohorts were generally older with more comorbidities, such as coronary heart disease, hypertension, diabetes, arrhythmia, and unhealthy living habits. In the present study, our participants were mostly middle-aged men, with no related cardiovascular risk factors or diseases other than hypertension. Our results also showed that patients with OSA had significantly lower ECV levels than those without OSA. Furthermore, the severe OSA group showed significantly lower native T1 values. Similar CMR results were reported for cardiac parameters in athletes (26), wherein lower native T1 and ECV values were noted than in untrained controls; the values were particularly lower in athletes with high performance. The difference between an athletic and OSA patient is that the former represents physiological stresses that cause LV physiological hypertrophy, while OSA induces disease-related triggers which lead to LV pathological hypertrophy (27). The specific stimulation to the cardiovascular system by OSA is analogous to hypertension in some respects by increasing cardiac afterload (28). Hypertension persists throughout the day; however, the damage to the LV caused by OSA is alleviated during the daytime because the collapse of the upper airway occurs only during sleep. Therefore, the enrolled patients and the OSA-specific pathogenic mechanism may explain the negative T1 mapping results, in contrast to eccentric or concentric left ventricular hypertrophy (LVH) with higher native T1 and ECV values observed in hypertensive patients (16). Many studies have highlighted the negative effects of pathological hypertrophy on CVD. However, it should be noted that LVH is a dynamic course, developing from the compensatory stage in short-term to the maladaptive phase, and ultimately resulting in LV dysfunction (27,29). The short-term stimulation of diseases may be beneficial by minimizing wall stress and reducing oxygen consumption. Nevertheless, considering the magnitude and duration of stimulation, long-term detrimental effects can arise. In an animal study even revealed that chronic cardiac overload rats model, animals developed cardiomyocyte hypertrophy without fibrosis after 8 weeks with dobutamine administration, as those running on treadmill did (30). As the authors mentioned, the features of cardiac overload may essentially be important to LVH, independent of the nature of stimulation.
Taking the ECV parameter a step further, myocardial tissue can be divided into cellular and extracellular compartments. Thomas and colleagues found that LVH in athletes was mainly caused by cellular hypertrophy and predominantly derived from extracellular matrix expansion in patients with cardiac amyloidosis (15). Rodrigues et al. (16) comprehensively characterized hypertensive heart disease using CMR and showed that concentric and eccentric LVH patterns were associated with elevated cellular components and extracellular matrix expansion. The greatest interstitial volume in eccentric LVH assists explaining the poor cardiovascular outcomes. Notably, our results showed that the elevated LVMI in patients with OSA was mainly due to cellular hypertrophy. To the best of our knowledge, to date, this is the first study to explore the myocardial tissue composition of OSA patients using the T1 mapping technique. Studies have revealed that not only LV cellular mass regresses but also diffuse fibrosis retreats after aortic valve replacement in aortic stenosis (14,29). With respect to OSA, this will stimulate an appropriate and relevant clinical approach for treatment and patient compliance with continuous positive airway pressure therapy.
The etiology and pathogenesis of OSA are frequently associated with cardiovascular and metabolic complications. The prevalence of comorbid OSA in hypertensive patients ranges from 30% to 50% (31). Obesity is a major risk factor for OSA through fat deposition within the upper airway and reduction in lung volume (28,31). Therefore, OSA commonly coexists with obesity with a frequency of up to 60% (28). The influence of OSA on LV is inevitably affected by obesity.
In this context, it is debatable whether OSA has an impact on elevated LVM. After excluding patients with major CVD, 11 out of 51 participants were hypertensive and received antihypertensive treatment in the current study. To eliminate the interference of confounding factors, we applied different grouping methods to maximize the usage of our data. Furthermore, we found that patients with OSA had a higher LVMI in every grouping method. Multivariate analysis showed that OSA, male sex, BMI, SBP, and hypertension were significantly associated with increased LVMI.
Consistent with previous studies, these results show that OSA is associated with LVMI (5,6,8). After adjusting for confounders, Huang et al. (6) found that among 1,053 men with coronary artery disease, those with concurrent severe OSA had a higher risk of developing LVH than those without OSA. Furthermore, in both men and women aged ≤65 years, higher LVM was significantly associated with more severe OSA in a cross-sectional analysis using CMR (5). These two large-scale studies provided strong evidence toward the association between OSA and LVMI. On the other hand, Koga et al. (8) found that OSA patients not only had higher LVMI but also showed a greater prevalence of concentric LV hypertrophy. However, some authors hold different views. A cross-sectional study that included 533 patients without prior CVD found that OSA was not associated with increased LVM after controlling for obesity and hypertension (32). Varol et al. (33) found no statistically significant difference between OSA and control groups, although a gradual upward trend between LVMI and OSA grade was observed. Similar findings were reported by Roubille et al. (34) in a CMR study. This study had a small sample size and more comorbidities in patients with OSA than the voluntary enrolment of healthy participants. In addition, in patients with OSA, the severity and duration of OSA may also explain the contradictory results of the association between OSA and LVMI.
Our results showed that LVMVR was also sensitive in detecting early LV remodeling in OSA patients (Table 2 and Figure 3). Even in the mild-to-moderate OSA group, the LVMVR was higher than that in the controls. The data showed that OSA is independently associated with an elevated LVMVR index. For both LVM and LVMVR parameter considerations, LV phenotypes were defined as normal LV, concentric remodeling, concentric LVH, or eccentric LVH (16). As per a meta-analysis OSA was more frequently associated with concentric LVH than eccentric LVH (9). However, the OSA group in our study did not meet the concentric remodeling or concentric LVH criteria and rather had a normal LV phenotype. This suggests that our study participants consisted of a sample of a healthy population without major CVD comorbidities.
This is the first study to analyze LV strain using CMR imaging. Our results showed that OSA patients had lower GCS values than healthy controls, although LVEF was preserved, and comparable findings were found within different OSA groups. LV strain studies remain controversial because of the difficulty in determining the definite effect of OSA on LV mechanics, without potential confounding factors. Some researchers found reduced LV GLS in OSA patients (35,36), which gradually decreased with the severity process (10,11). Zhou et al. further revealed that the three-layer longitudinal and circumferential LV strains deteriorated in OSA (11). Wang et al. found that GCS and GRS scores were significantly reduced only in patients with severe OSA (10). However, some studies failed to show impairment of GCS and GRS values, even in the severe OSA group (36). Regarding of limited CMR exploration in OSA domain, much more CMR research needs to be done to confirm our findings and reveal how LV mechanics impaired.
This study has several limitations. First, although we detected significant differences in CMR data between the OSA and non-OSA groups and positive multilinear analysis results, this was a single-center cohort study with a relatively small sample size. Second, the participants were diagnosed with PSG for the first time in this study, and whether the duration of OSA would have an additional effect on LVMI or other CMR indices remains unclear. Finally, the iCV and iECV measured by T1 mapping do not exclusively denote the total mass of cardiomyocytes or fibrillar collagens, respectively. Additionally, the histological assessment of endomyocardial biopsies, undoubtedly the most precise method for confirming the current insights into myocardial configuration was not performed in this study.
Conclusions
LV remodeling in OSA with preserved LVEF is prominent with cellular hypertrophy which may retreat after proper clinical management. Longitudinal analyses and long-term follow-up of OSA with CMR scans are required for a comprehensive understanding of the LV remodeling process.
Acknowledgments
Funding: The study was supported by the National Natural Science Foundation of China (Grant Nos. 81974262 and 81970288 to Hui Liu; grant No. 81870022 to Qiong Ou), Natural Science Foundation of Guangdong Province (Grant No. 2020A1515010650 to Hui Liu), and Guangdong Cardiovascular Institute Project (Grant No. 2020XXG009 to Hui Liu).
Footnote
Reporting Checklist: The authors have completed the STROBE reporting checklist. Available at https://cdt.amegroups.com/article/view/10.21037/cdt-22-38/rc
Data Sharing Statement: Available at https://cdt.amegroups.com/article/view/10.21037/cdt-22-38/dss
Peer Review File: Available at https://cdt.amegroups.com/article/view/10.21037/cdt-22-38/prf
Conflicts of Interest: All authors have completed the ICMJE uniform disclosure form (available at https://cdt.amegroups.com/article/view/10.21037/cdt-22-38/coif). The authors have no conflicts of interest to declare.
Ethical Statement: The authors are accountable for all aspects of the work in ensuring that questions related to the accuracy or integrity of any part of the work are appropriately investigated and resolved. The study was conducted in accordance with the Declaration of Helsinki (as revised in 2013) and was approved by the Institutional Review Board of Guangdong Provincial People’s Hospital (ethical code: 2017079H[R1]). Written informed consent requirement was obtained from all participants before CMR imaging.
Open Access Statement: This is an Open Access article distributed in accordance with the Creative Commons Attribution-NonCommercial-NoDerivs 4.0 International License (CC BY-NC-ND 4.0), which permits the non-commercial replication and distribution of the article with the strict proviso that no changes or edits are made and the original work is properly cited (including links to both the formal publication through the relevant DOI and the license). See: https://creativecommons.org/licenses/by-nc-nd/4.0/.
References
- Chen X, Wang R, Zee P, et al. Racial/Ethnic Differences in Sleep Disturbances: The Multi-Ethnic Study of Atherosclerosis (MESA). Sleep 2015;38:877-88. [Crossref] [PubMed]
- Yoshihisa A, Takeishi Y. Sleep Disordered Breathing and Cardiovascular Diseases. J Atheroscler Thromb 2019;26:315-27. [Crossref] [PubMed]
- Lee CH, Sethi R, Li R, et al. Obstructive Sleep Apnea and Cardiovascular Events After Percutaneous Coronary Intervention. Circulation 2016;133:2008-17. [Crossref] [PubMed]
- Wang H, Parker JD, Newton GE, et al. Influence of obstructive sleep apnea on mortality in patients with heart failure. J Am Coll Cardiol 2007;49:1625-31. [Crossref] [PubMed]
- Javaheri S, Sharma RK, Wang R, et al. Association between Obstructive Sleep Apnea and Left Ventricular Structure by Age and Gender: the Multi-Ethnic Study of Atherosclerosis. Sleep 2016;39:523-9. [Crossref] [PubMed]
- Huang Z, Wang L, Liu Y, et al. Impact of obstructive sleep apnea on left ventricular mass index in men with coronary artery disease. J Clin Sleep Med 2020;16:1675-82. [Crossref] [PubMed]
- Drager LF, Bortolotto LA, Figueiredo AC, et al. Obstructive sleep apnea, hypertension, and their interaction on arterial stiffness and heart remodeling. Chest 2007;131:1379-86. [Crossref] [PubMed]
- Koga S, Ikeda S, Nakata T, et al. Effects of nasal continuous positive airway pressure on left ventricular concentric hypertrophy in obstructive sleep apnea syndrome. Intern Med 2012;51:2863-8. [Crossref] [PubMed]
- Cuspidi C, Tadic M, Sala C, et al. Targeting Concentric Left Ventricular Hypertrophy in Obstructive Sleep Apnea Syndrome. A Meta-analysis of Echocardiographic Studies. Am J Hypertens 2020;33:310-5. [Crossref] [PubMed]
- Wang D, Ma GS, Wang XY, et al. Left ventricular subclinical dysfunction associated with myocardial deformation changes in obstructive sleep apnea patients estimated by real-time 3D speckle-tracking echocardiography. Sleep Breath 2016;20:135-44. [Crossref] [PubMed]
- Zhou NW, Shu XH, Liu YL, et al. A Novel Method for Sensitive Determination of Subclinical Left-Ventricular Systolic Dysfunction in Subjects With Obstructive Sleep Apnea. Respir Care 2016;61:366-75. [Crossref] [PubMed]
- Chen R, Wang J, Du Z, et al. The comparison of short-term prognostic value of T1 mapping with feature tracking by cardiovascular magnetic resonance in patients with severe dilated cardiomyopathy. Int J Cardiovasc Imaging 2019;35:171-8. [Crossref] [PubMed]
- Nakamori S, Dohi K, Ishida M, et al. Native T1 Mapping and Extracellular Volume Mapping for the Assessment of Diffuse Myocardial Fibrosis in Dilated Cardiomyopathy. JACC Cardiovasc Imaging 2018;11:48-59. [Crossref] [PubMed]
- Treibel TA, Kozor R, Schofield R, et al. Reverse Myocardial Remodeling Following Valve Replacement in Patients With Aortic Stenosis. J Am Coll Cardiol 2018;71:860-71. [Crossref] [PubMed]
- Treibel TA, Kozor R, Menacho K, et al. Left Ventricular Hypertrophy Revisited: Cell and Matrix Expansion Have Disease-Specific Relationships. Circulation 2017;136:2519-21. [Crossref] [PubMed]
- Rodrigues JC, Amadu AM, Dastidar AG, et al. Comprehensive characterisation of hypertensive heart disease left ventricular phenotypes. Heart 2016;102:1671-9. [Crossref] [PubMed]
- Obokata M, Nagata Y, Wu VC, et al. Direct comparison of cardiac magnetic resonance feature tracking and 2D/3D echocardiography speckle tracking for evaluation of global left ventricular strain. Eur Heart J Cardiovasc Imaging 2016;17:525-32. [Crossref] [PubMed]
- Berry RB, Budhiraja R, Gottlieb DJ, et al. Rules for scoring respiratory events in sleep: update of the 2007 AASM Manual for the Scoring of Sleep and Associated Events. Deliberations of the Sleep Apnea Definitions Task Force of the American Academy of Sleep Medicine. J Clin Sleep Med 2012;8:597-619. [Crossref] [PubMed]
- Bodez D, Damy T, Soulat-Dufour L, et al. Consequences of obstructive sleep apnoea syndrome on left ventricular geometry and diastolic function. Arch Cardiovasc Dis 2016;109:494-503. [Crossref] [PubMed]
- Bradley TD, Floras JS. Obstructive sleep apnoea and its cardiovascular consequences. Lancet 2009;373:82-93. [Crossref] [PubMed]
- Mehra R. Sleep apnea and the heart. Cleve Clin J Med 2019;86:10-8. [Crossref] [PubMed]
- Shah NA, Reid M, Kizer JR, et al. Sleep Disordered Breathing and Left Ventricular Scar on Cardiac Magnetic Resonance: Results of the Multi-Ethnic Study of Atherosclerosis. J Clin Sleep Med 2020;16:855-62. [Crossref] [PubMed]
- Wang S, Cui H, Ji K, et al. Relationship Between Obstructive Sleep Apnea and Late Gadolinium Enhancement and Their Effect on Cardiac Arrhythmias in Patients with Hypertrophic Obstructive Cardiomyopathy. Nat Sci Sleep 2021;13:447-56. [Crossref] [PubMed]
- de Oliveira FG, Pinto I, Valdigem B, et al. Evaluation of late atrial enhancement by cardiac magnetic resonance imaging in patients with obstructive sleep apnea. Sleep Med 2020;74:204-10. [Crossref] [PubMed]
- Neilan TG, Farhad H, Dodson JA, et al. Effect of sleep apnea and continuous positive airway pressure on cardiac structure and recurrence of atrial fibrillation. J Am Heart Assoc 2013;2:e000421. [Crossref] [PubMed]
- McDiarmid AK, Swoboda PP, Erhayiem B, et al. Athletic Cardiac Adaptation in Males Is a Consequence of Elevated Myocyte Mass. Circ Cardiovasc Imaging 2016;9:e003579. [Crossref] [PubMed]
- Schiattarella GG, Hill JA. Inhibition of hypertrophy is a good therapeutic strategy in ventricular pressure overload. Circulation 2015;131:1435-47. [Crossref] [PubMed]
- Javaheri S, Barbe F, Campos-Rodriguez F, et al. Sleep Apnea: Types, Mechanisms, and Clinical Cardiovascular Consequences. J Am Coll Cardiol 2017;69:841-58. [Crossref] [PubMed]
- Bing R, Cavalcante JL, Everett RJ, et al. Imaging and Impact of Myocardial Fibrosis in Aortic Stenosis. JACC Cardiovasc Imaging 2019;12:283-96. [Crossref] [PubMed]
- Moreira-Gonçalves D, Henriques-Coelho T, Fonseca H, et al. Intermittent cardiac overload results in adaptive hypertrophy and provides protection against left ventricular acute pressure overload insult. J Physiol 2015;593:3885-97. [Crossref] [PubMed]
- Yeghiazarians Y, Jneid H, Tietjens JR, et al. Obstructive Sleep Apnea and Cardiovascular Disease: A Scientific Statement From the American Heart Association. Circulation 2021;144:e56-67. [Crossref] [PubMed]
- Niroumand M, Kuperstein R, Sasson Z, et al. Impact of obstructive sleep apnea on left ventricular mass and diastolic function. Am J Respir Crit Care Med 2001;163:1632-6. [Crossref] [PubMed]
- Varol E, Akcay S, Ozaydin M, et al. Influence of obstructive sleep apnea on left ventricular mass and global function: sleep apnea and myocardial performance index. Heart Vessels 2010;25:400-4. [Crossref] [PubMed]
- Roubille F, Fischer K, Guensch DP, et al. Impact of hyperventilation and apnea on myocardial oxygenation in patients with obstructive sleep apnea - An oxygenation-sensitive CMR study. J Cardiol 2017;69:489-94. [Crossref] [PubMed]
- Varghese MJ, Sharma G, Shukla G, et al. Longitudinal ventricular systolic dysfunction in patients with very severe obstructive sleep apnea: A case control study using speckle tracking imaging. Indian Heart J 2017;69:305-10. [Crossref] [PubMed]
- Vitarelli A, D'Orazio S, Caranci F, et al. Left ventricular torsion abnormalities in patients with obstructive sleep apnea syndrome: an early sign of subclinical dysfunction. Int J Cardiol 2013;165:512-8. [Crossref] [PubMed]