Left ventricular noncompaction: a disorder with genotypic and phenotypic heterogeneity—a narrative review
Introduction
Left ventricular noncompaction (LVNC), also used synonymously as hypertrabeculation, is a cardiomyopathy characterized morphologically by two layers of highly thickened myocardium, numerous prominent trabecular processes, and deep intertrabecular recesses that connect to the left ventricular (LV) cavity (1,2). LVNC is the third most common cardiomyopathy after dilated cardiomyopathy (DCM) and hypertrophic cardiomyopathy (HCM) (3). LVNC has a broad morphologic spectrum and can appear alone or in association with DCM, HCM, early onset of arrhythmias, and congenital heart disease (CHD) (4). The clinical presentation of LVNC is highly variable, ranging from asymptomatic to severely symptomatic, including congestive heart failure (HF), arrhythmias, and thromboembolism (1,5-7). To date, genetic variants have been reported in patients with LVNC (5). Inheritance of LVNC is predominantly autosomal dominant, although X-linked recessive, autosomal recessive, and mitochondrial inheritance also occurs (6,8,9). However, despite the first clinical description of LVNC appearing 30 years ago (1), understanding of LVNC and its pathogenesis has not yet been fully elucidated, especially when compared to other cardiomyopathies such as HCM or DCM.
Therefore, we addressed the latest findings on genes reported to be associated with LVNC morphogenesis and possible pathologies in human or non-human model organisms to understand the diverse spectrum between genotype and phenotype in LVNC. Moreover, we summarized the latest findings and issues related to the diagnosis of LVNC. We present the following article in accordance with the Narrative Review reporting checklist (available at: https://cdt.amegroups.com/article/view/10.21037/cdt-22-198/rc).
Methods
Relevant studies published in the past 30 years to May 2022 were identified by PubMed searches using different combinations of the following search terms: “left ventricular noncompaction” and “hypertrabeculation”. The reference lists of relevant articles were also reviewed and additional articles were identified. Publications with relatively low reliability and non-English language publications were excluded. Data extraction was based on relevance to the topic rather than implementing a systematic approach to article extraction. This paper discusses existing data from the perspective of cardiologists caring for LVNC patients, is written as an expository narrative review, and provides an update on the current literature.
Epidemiology
The incidence of LVNC is reported to be 0.81 per 100,000 for infants, 0.12 per 100,000, and 50 per 100,000 for adults (3,10). Children are thought to account for 9.5% of all cardiomyopathies (5). LVNC is more common in males (62%) than in females (11).
Subtype
LVNC is often classified according to phenotype as follows (8,12,13).
- Isolated LVNC: normal cardiac function, with relatively good prognosis;
- LVNC with arrhythmias: normal cardiac function but with arrhythmias, often associated with ventricular arrhythmias, which is a poor prognostic factor. The arrhythmia phenotype is identified if a systolic function is preserved and LV size and wall thickness are normal but underlying arrhythmias are present, usually identified at diagnosis;
- Dilated LVNC: as in DCM, but with LV dilation and reduced contractility; often a poorer prognostic factor than DCM;
- Hypertrophic LVNC: as in HCM, but with LV thickening and impaired diastolic function. The prognosis is often similar to that of HCM;
- Restrictive LVNC: with enlargement of the left atrium or both atria and impairment of LV diastolic function. As with restrictive cardiomyopathy, the prognosis is often poor;
- LVNC associated with CHD: complications such as atrial septal defects, ventricular septal defects, and Epstein’s disease have been reported and present in 7% of patients (11). Compared to patients with CHD without LVNC, LV contractility is often reduced (14).
The MOGE(S) nomenclature may provide a clear basis for classifying phenotypic variations of LVNC. This nomenclature includes various aspects of cardiomyopathy, such as morphofunctional features (M), organ involvement (O), genetic patterns (G), etiologic annotation (E), and patient functional status by American college of cardiology/ American Heart association (ACC/AHA) staging or New York Heart Association (NYHA) functional class. MOGE(S) can be used to fix phenotypes at baseline and record follow-up data and may be useful to consider genotype-phenotype correlation (15).
Genetics
The genetic background of LVNC is both isolated and familial. A high percentage (28%) of familial cases were found to be characterized by genetic diversity, with autosomal dominant, autosomal recessive, or mitochondrial gene abnormalities as well as X-linked inheritance suspected in some families (11,16). Approximately 40% are thought to have constant genetic variants (17). Many genes should be considered that the association with LVNC is weak since these are based on single reports and the data have not been replicated. van Waning et al. conducted a systematic review and found that 80 genes were genetic causes of LVNC (Table 1) (16). Most genetic causes were single variants (85%), of which autosomal dominant missense variants were the most common (55%) (16). X-linked genetic abnormalities accounted for 7% of patients, mostly TAZ (6%). More than 50% of the genetic defects were reported in sarcomere genes, the most common being MYH7 (25%). Arrhythmia gene variants were found in 11%, especially in HCN4 (4%). Rojanasopondist et al. sought to systematically analyze and score the strength of all published evidence linking each gene to LVNC, and then verified which molecular pathways are likely to be involved in the pathogenesis of LVNC (18). They identified 189 genes associated with LVNC; 11 were classified as definitive, 21 as moderate, 140 as limited, and 17 were classified as no evidence. Of the 32 genes classified as definitive or moderate, the most common gene functions were sarcomere function (34%), transcription/translation regulator (19%), mitochondrial function (9%), and cytoskeletal proteins (9%) (Table 1). In addition, 18 genes (56%) were involved in the presentation of noncardiac syndromes. In addition, there were 20 genes shared between LVNC and DCM, and 18 genes shared between LVNC and HCM. Analysis of pathways and protein-protein interactions suggested that LVNC, more than DCM and HCM, is responsible for abnormalities in cardiomyocyte development and differentiation during cardiomyogenesis.
Table 1
Group according to the molecular function | Gene | Locus | Protein | Inheritance | Associated conditions | Frequency* |
---|---|---|---|---|---|---|
Sarcomere genes | ACTC1 | 15q14 | Actin alpha cardiac muscle 1 | AD | HCM, DCM, ASD | 7% |
DES | 2q35 | Desmin | AD | DCM, RCM | ||
LDB3 | 10q23.2 | LIM domain binding 3 | AD | HCM, DCM | ||
MYBPC3 | 11p11.2 | Myosin binding protein C3 | AD | HCM, DCM | 7% | |
MYH7 | 14q11.2 | Myosin heavy chain 7 | AD | HCM, DCM | 25% | |
OBSCN | 1q42.13 | Obscurin | AD | HCM, DCM | ||
PLN | 6q22.31 | Phospholamban | AD | HCM, DCM | ||
RYR2 | 1q43 | Ryanodine receptor 2 | AD | CPVT | 2% | |
TNNT2 | 1q32.1 | Troponin T2, cardiac type | AD | DCM, HCM, RCM | 2% | |
TPM1 | 15q22.2 | Tropomyosin 1 | AD | DCM, HCM | 3% | |
TTN | 2q31.2 | Titin | AD | DCM | 4% | |
Transcriptional/translational regulator genes | NKX2-5 | 5q35.1 | NK2 homeobox 5 | AD | CHD | |
NONO | Xq13.1 | Non-POU domain-containing octamer-binding protein | XL | Intellectual developmental disorder, X-linked syndromic 34 | ||
PRDM16 | 1p36.32 | PR/SET domain 16 | AD | DCM | ||
RBM20 | 10q25.2 | RNA binding motif protein 20 | AD | DCM | ||
TBX5 | 12q24.21 | T-box transcription factor 5 | AD | Holt-Oram syndrome | ||
TBX20 | 7p14.2 | T-box transcription factor 20 | CHD | |||
Mitochondrial function genes | NNT | 5p12 | Nicotinamide nucleotide transhydrogenase | AR | Glucocorticoid deficiency 4, with or without mineralocorticoid deficiency | |
TAZ | Xq28 | Taffazin | XL | Barth syndrome | 2% | |
TMEM70 | 8q21.11 | Transmembrane protein 70 | AR | Mitochondrial complex V (ATP synthase) deficiency, | ||
Cytoskeletal protein genes | DMD | Xp21.2-p21.1 | Dystrophin | XL | DCM, Duchenne/Becker muscular dystrophy | |
DTNA | 18q12.1 | Dystrobrevin alpha | AD | DCM | ||
LMNA | 1q22 | Lamin A/C | AD | DCM | ||
Cellular junction protein genes | DSP | 6p24.3 | Desmoplakin | AD | DCM | |
PKP2 | 12p11.21 | Plakophilin 2 | AR | ARVC | ||
Intracellular trafficking genes | LAMP2 | Xq24 | Lysosomal associated membrane protein 2 | XL | Danon disease | |
PLEKHM2 | 1p36.21 | Pleckstrin homology domain-containing family M member 2 | AD | |||
Ion channel genes | HCN4 | 15q24.1 | Hyperpolarization activated cyclic nucleotide gated potassium channel 4 | AD | MVP, bradycardia, Brugada syndrome | 4% |
SCN5A | 3p22.2 | Sodium voltage-gated channel alpha subunit 5 | AD | Brugada syndrome, LQTS, DCM, AF, SSS | 1% | |
Signal transduction genes | ALPK3 | 15q25.3 | Alpha kinase 3 | AR | HCM (signal transduction) | |
DMPK | 19q13.32 | DM1 protein kinase | AD | Myotonic dystrophy 1 | ||
Protein degradation gene | MIB1 | 18q11.2 | MIB E3 ubiquitin protein ligase 1 | AD | 5% |
*, proportion of all variants. AD, autosomal dominant; XL, X linked; AR, autosomal recessive; HCM, hypertrophic cardiomyopathy; DCM, dilated cardiomyopathy; ASD, atrial septal defect; RCM, restrictive cardiomyopathy; CPVT, catecholaminergic polymorphic ventricular tachycardia; CHD, congenital heart disease; ATP, adenosine triphosphate; ARVC, arrhythmogenic right ventricular cardiomyopathy; MVP, mitral valve prolapse; LQTS, long QT syndrome; AF, atrial fibrillation; SSS, sick sinus syndrome.
Some mitochondrial DNA (mtDNA) variants may be associated with LVNC (19-23). Cardiomyopathy has been reported in up to 20% of patients with mitochondrial disease (24). The cardiac phenotype typically seen in patients with mtDNA variants is characterized by mild, usually concentric LV enlargement, while LVNC occurs rarely (20,21). mtDNA variants include nuclear gene variants in 51%, mtDNA point variants in 40%, mtDNA single large deletions in 5%, and chromosomal abnormalities in 4% (25).
Neuromuscular disorders were present in an average of 5% (11). In neuromuscular disorders, the DMD genetic abnormality causing Duchenne and Becker muscular dystrophy also causes LVNC in boys.
Complex and rare monogenic syndromes with cardiac and non-cardiac abnormalities should be considered secondary to origin or cause of LVNC, such as Danon disease caused by LAMP2 gene variants and Anderson-Fabry disease caused by GLA gene variants, which do not show typical LVNC (26-28). Periventricular dysplasia and rare syndromes, such as microcephaly, glucocorticoid deficiency, and Coffin-Lowry syndrome caused by RPS6KA gene variants, may occasionally manifest LVNC (29-37).
In addition to single gene abnormalities, LVNC can be seen in association with chromosomal disorders. Syndromes with LVNC have been reported in patients with chromosomal disorders, including 1p36 deletion syndrome, interstitial 1q43-q43del, del(1)(q) syndrome, del5q35; 7p14.3p14.1 deletion, 8p23.1 deletion, 1 del syndrome, 18p subtelomere deletion, 22q11.2 deletion syndrome, 22q11.2 distal deletion syndrome, 13 and 18 trisomy, tetrasomy 5q35.2-5q35, Robertson translocation 13;14, 45,X/46XX, and 45,X/46,X,i(Y)(p11), among others. Chromosomal abnormalities accounted for 6% of patients, and 88% of them were diagnosed in childhood. Most chromosomal aberrations are isolated cases (38,39).
Pathogenesis
Two hypothesized pathogenesis mechanisms are proposed for LVNC: the congenital hypothesis during the embryonic period, and the acquired hypothesis after birth.
Congenital hypothesis
In congenital LVNC, the process of compaction is thought to be arrested, leaving behind trabeculated fetal myocardium and, conversely, a hypoplastic compacted myocardium. During mammalian heart development, the ventricle undergoes a series of morphogenetic events (40-42), including trabeculation and compaction (Figure 1) (43). Ventricular formation consists of four steps and involves multiple signals originating from the endocardium, myocardium, and epicardium. The first step is the formation of a single cell layer of myocardium in early development (e.g., E8.5 in mouse embryos, corresponding to E19 in humans). The second stage is the formation of the trabecular myocardium in early mid-gestation (e.g., E9.5–E10.5 in mouse embryos, corresponding to E22–E28 in humans). As the loops form and the myocardium thickens, cardiomyocytes in specific areas along the inner wall of the heart form sheet-like projections into the lumen, forming trabecular myocardium. The outer layer of the myocardium remains as a base for the formation of compact myocardium (43). The third stage is the compaction of the myocardium in the second half of gestation (e.g., E12.5–E13.5 in mouse embryos, corresponding to E40–E44 in humans). The fourth stage is the formation of the three-layered helical myocardium from late fetal to neonatal stages (41). Unlike skeletal muscle, ventricular myocardium is composed of a three-dimensional network of myocytes in the subendocardial, subepicardial, and intermediate layers (44-51). Patients with LVNC have little apical torsion and LV steric rotation, with basal and apical rotation in the same direction (52,53). The normal three-layer structure in the compacted myocardium may be disrupted because LVNC is a residual of embryonic trabecular myocardium.
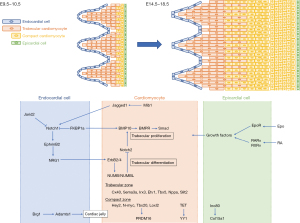
During this heart developmental stage, trabeculation and compaction of the LV require interactions between cardiomyocytes and their surrounding environments, such as the endocardium, epicardium, extracellular matrix, other cardiomyocyte factors, and epigenetic factors according to genetic studies in mice (Figure 1). Disruption of this developmental process by defects in cardiac proliferation, impaired trabecular coalescence, or defects in myofibrillogenesis and polarization of cardiomyocytes leads to an abnormal trajectory of trabeculogenesis and ventricular wall compression that is subsequently theorized to cause LVNC (4,54).
Trabeculae formation requires an interaction between the endocardium and myocardium, which, late in development, contributes to ventricular compaction. These endocardial-myocardial pathways are largely driven by signaling from the neuregulin1/ErbB, Notch, and bone morphogenetic protein (BMP) 10 pathways. The Notch1 and neureglin1 pathways interact dynamically to regulate extracellular matrix dynamics and trabecular growth, establishing a basis of trabecular architecture. The Dll4-Notch1 (55,56), Mib1 (57), BMP10 (58), and tumor growth factor (TGF)-β pathways (59), regulate trabecular compaction. Hey2 or Nppa systems are involved in the Dll4-Notch1 variant. Nrg1, Bmp10, and Hey2 have been reported to be Notch1-dependent (60,61). Recent studies have shown that Dll4-Notch1 signaling induces the formation of the trabeculae and coordinates their patterning and compaction with coronary formation to form mature chambers (55,56). Germline variants in human MIB1, encoding the E3 ubiquitin ligase that promotes endocytosis of the Notch ligands Delta and Jagged, cause LVNC, and those affected have decreased Notch1 activity and target genes (57). Kodo et al. showed that Tbx20 regulates the expression of TGF-β signaling regulators, including Prdm16 (59), a SMAD2/3 repressor of TGF-β signaling through binding (62-64). Downregulation of Erbb2 signaling in mature spongiosa has been shown to require the endocytosis-adaptive proteins Numb and Numbl (65).
The epicardium proliferates from the anterior pericardial organ at the venous pole of the heart beginning at E9.5 in mice (corresponding to E21 in humans), and the entire myocardial surface is covered by E11.0 (corresponding to E24 in humans) (66). The interaction between the epicardium and myocardium appears to be the primary driver of the compaction process (67,68). The epicardial-myocardial pathway includes retinoic acid (RA), erythropoietin (EPO), insulin-like growth factor (IGF-1), and fibroblast growth factor (FGF) signaling to support compaction. The underdevelopment of coronary artery is accompanied by abnormal growth of compact myocardium in several mouse models (55,69-72).
The extracellular matrix plays an important role in both trabeculae formation and compaction. The trabeculae require the chromatin-remodeling protein Brg1 to require to suppress the expression of Adamts1 in the endocardium above the developing trabeculae (73).
Other cardiomyocyte genes are involved in cardiomyocyte proliferation, but when or how their protein products facilitate interactions with surrounding cells remains unclear, although the cardiac transcription factor Nkx2-5 is an important regulator of ventricular trabecular and conduction system development (74-76).
The regulation of chromatin levels, particularly DNA methylation, adenosine triphosphate (ATP)-dependent chromatin remodeling that alters nucleosome structure, and covalent histone modifications that alter DNA-histone interactions (77). Regulation of chromatin levels contributes to gene expression in cardiac development, including cardiomyocyte proliferation, trabecular structure, and compaction. Jarid2 knockout mice exhibit HF, including trabecular hypertrophy with noncompaction of the ventricular wall. In Jarid2 knockout mice, failure to properly regulate Notch signaling may lead to defects in the developing ventricle (78). Mice deficient in endothelial Brg1, a chromatin remodeler, do not form proper trabeculations due to premature degradation of the cardiac jelly, the extracellular matrix between the myocardium and endocardium (73). Tet protein-mediated DNA hydroxymethylation regulates chromatin accessibility (79). Cardiac-specific deletion of Tet2 and Tet3 in mice leads to LVNC with embryonic lethality (79). Deletion of the Ino80 chromatin remodeler from embryonic endothelial cells has been shown to result in LVNC (80).
Acquired hypothesis
The acquired hypothesis is that LVNC may be secondary to the process of myocardial remodeling. Acquired LVNC may occur in athletes (81-83), as well as those with sickle cell anemia (84,85), pregnancy (86), renal failure (87), and other conditions. There are increasing reports of healthy subjects showing trabecular hypertrophy in the LV with normal size, function, and wall thickness. Many studies have concluded that LV trabeculae may develop or hidden trabeculae may be exposed in some situations of cardiac remodeling that occurs because of preload and increased cardiac output. Considering acquired LVNC, an increase in trabeculae may provide an adaptive advantage. LV compliance is highest in the absence of trabeculae, but the apex is subjected to higher wall stresses and strains. The presence of apical trabeculae may redistribute apical wall stresses and protect the thin-walled apex from adverse aneurysmal remodeling (88). Increasing stroke volume comes at a considerable energy cost and requires progressively higher longitudinal strain in smooth and non-trabecular LV, whereas, in trabecular LV, higher stroke volume can be achieved without exponentially increasing strain (89). These observations may support the pathogenesis of acquired LVNC.
Theoretical gap among human and non-human models or congenital and acquired hypothesis
In mouse models, many of the genes associated with cardiomyocyte proliferation result in LVNC-like phenotype. Since the products of these genes also function in other cells, gene deletions are expected to cause embryonic lethality. In contrast, many variants associated with human LVNC are sarcomere genes, part of myofibrillar structures, and are associated with contractile function, and alter the resulting protein rather than eliminate its function. Indeed, we reported that sarcomere variants are most commonly identified in fetus-onset patients, and fetus-onset patients with LVNC are more likely to develop HF (90). Moreover, we found a time-dependent increase in the ratio of noncompacted to compressible layers (N/C) of the LV wall in patients with fetal-onset LVNC, with the N/C ratio being greater than 2 before and after birth (91). This change is in contrast to patients with DCM and HCM, suggesting that the formation of the trabeculated layer proceeds late in gestation. Thus, studies focusing on fetal LVNC provide new clues to previous hypotheses of mouse models and the development of LVNC in humans. Recent advances in the generation of disease models of LVNC using genetically engineered mice may be expected to elucidate the etiology of LVNC.
LVNC exhibits several distinct forms, depending on the associated phenotype. Considering this vast etiologic heterogeneity, it makes sense that the genetics of LVNC are heterogeneous, with different causative genes and multiple disease-causing variants in each gene. The acquired and reversible morphology of LVNC poses a problem for the traditional interpretation that the noncompacted forms are genetic diseases (81,82,84-87).
Imaging
Echocardiography
Two-dimensional echocardiography is recommended as the first-line imaging modality for patients with LVNC because it is noninvasive, easy to perform, inexpensive, and, with the use of echo contrast agents, can delineate the presence of LV thrombus and trabeculae (92). Furthermore, as advances in tissue harmonics and image resolution in echocardiography have been made, there have been increasing reports of features consistent with even healthy populations. Excessive trabecular formation of the ventricular wall, deep recesses, and a two-layered structure with a trabeculated layer on the endocardial side and a compacted layer on the epicardial side can be observed by echocardiography (Figure 2). The trabeculated layer is often seen in the lateral, inferior, and posterior walls centered on the apex, and rarely on the ventricular septum or anterior wall (93). On color Doppler, blood flows in and out of the gaps in the reticular meatus formation centered on the apex, clearly differentiating this condition from apex HCM.
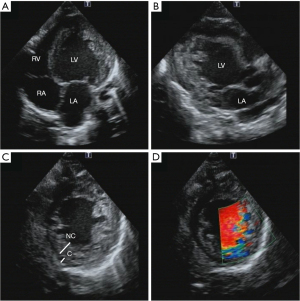
Cardiac magnetic resonance imaging (CMR)
CMR should be used to confirm the diagnosis of LVNC and rule out other cardiovascular diseases that may be present (94). CMR is capable of providing a detailed view of the cardiac structures in multiple image plans, including apical and lateral areas not easily seen on an echocardiogram (Figure 3). Multiple studies of late gadolinium enhancement (LGE) have been reported in LVNC on CMR. The most common LGE patterns are in the ventricular septum and right ventricular inflow region, similar to findings in DCM and HCM, and often differ from the apex, lateral, inferior, and posterior walls of the left ventricle, where trabeculation is most prominent (95). Moreover, the presence and extent of LGE are related to the clinical features and LV systolic function (96). Patients with isolated LVNC and no LGE have a better prognosis than patients with LVNC and LGE (95).
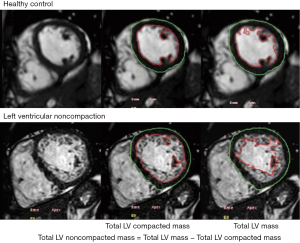
In recent years, native T1 mapping has proven to be a useful tool to quantitatively measure myocardial tissue characteristics without the administration of exogenous contrast agents (97). Myocardial T1 values in patients with diffuse myocardial fibrosis have been reported to correlate well with fibrosis confirmed by myocardial biopsy (98). Native T1 mapping is superior for characterizing diffuse fibrosis and subtle focal lesions in comparison with LGE (97,99). Previous studies that applied T1 mapping to patients with LVNC and without LGE showed that LVNC patients had elevated native myocardial T1 values compared to normal controls, suggesting that native T1 mapping is a more useful indicator of early myocardial fibrosis than LGE imaging in LVNC patients (100,101).
Thus, CMR provides morphological assessment and non-invasive tissue characterization and has been widely used in LVNC.
Electrocardiogram
Electrocardiographic abnormalities are present in approximately 90% of patients but are non-specific (54). LV hypertrophy, repolarization abnormalities, T wave negativity, ST changes, axial displacement, intraventricular conduction disturbances, and atrioventricular (AV) block are seen (102). Conduction disturbances such as AV block or bundle branch block are observed in 26% of patients, while supraventricular tachycardia is present in 17%. Sustained ventricular tachycardia occurs in 8%, and when non-sustained ventricular tachycardia is included, in 20% of cases (11). Certain arrhythmias are seen in patients with specific genetic variants, such as sinus failure syndrome in patients with LVNC with the HCN4 gene variant.
Myocardial biopsy
Endocardial fibrosis or fibroblasts were the most frequently reported abnormalities in adult and pediatric LVNC (1,103-106). It is unclear whether endocardial fibrosis is caused by an immune response, the result of local blood flow abnormalities, or induced by hemodynamic and mechanical factors around the trabecular myocardium (107).
Often a mural thrombus is seen on the endocardial surface in the fine-walled column area. The myocardial layer shows various degrees of degeneration, mainly subendocardial, and up to moderate fibrosis (108,109). However, there is no extensive myocardial desmoplasia or foci of mottled fibrosis, as is often seen in DCM, and the histological findings are rather similar to those of endocardial fibroelastosis.
Diagnosis
Imaging studies are essential for diagnosis. Diagnostic criteria based on echocardiography, CMR, and multidetector computed tomography (MDCT) have been proposed (Table 2) (1,5,52,110-124). Diagnostic criteria were created based on the ratio between noncompacted layer thickness (2,117), mass (118), volume (125), and compacted layer. The number of segments of the noncompacted layer provides information on LVNC dilation (119,126). Another approach integrates the assessment of counts or a global trabecular index (127). However, to date, there are no consensus criteria (128-130).
Table 2
Modality | First author | Patients | Morphologic criteria (measurement) (cut-off for positive criteria) | Cardiac phase | Recommended section |
---|---|---|---|---|---|
Echocardiography | Chin et al. (1), 1990 | 8 | • Distance (X) from the deepest trough of the trabecular recess to the epicardial surface • Distance (Y) peak of trabeculations to the epicardial surface • X/Y <0.5 considered potential LVNC |
• End-diastole, parasternal long axis, or apical four-chamber view • Sensitivity, 79–100%; specificity, 54–92% |
Long axis |
Jenni et al. (110), 2002 | 34 | • N/C >2 • Morphologic criteria: • Absence of coexisting cardiac abnormalities • Numerous excessively prominent trabeculations and deep intertrabecular recesses • Intertrabecular spaces filled by direct blood flow from the ventricular cavity |
• End systolic, parasternal short axis • Insensitive |
Short axis | |
Stöllberger et al. (111), 2002 | 62 | • N/C ≥2 • >3 Trabeculations protruding from the LV wall distal to the papillary muscles at the apex • Perfusion of the intertrabecular recesses from the ventricular cavity • Trabeculations must have the same echogenicity as the myocardium while moving in sync with ventricular contractions |
• End-diastole, apical four-chamber view • Sensitivity, 79–80%; specificity, 79–93% |
Lax (± short axis) | |
Pignatelli et al. (5), 2003 | 36 | • N/C >1.4 | • End-diastole | Long axis | |
Belanger et al. (112), 2008 | 60 | • N/C >2 + area >5 cm2 | • End-systole | Long axis + short axis | |
van Dalen et al. (52), 2008 | 10 | • LV twist—rigid body rotation | • Systole and diastole | Short axis | |
Paterick et al. (113), 2012 | 0 | • N/C ≥2 | • End-diastole | Short axis | |
Gebhard et al. (114), 2012 | 41 | • Max systolic C layer <8.1 mm | • End-systole | Short axis | |
Yubbu et al. (115), 2018 | 30 | • Circumferential strain <−24.5% | • Systole and diastole | Short axis apical | |
Caselli et al. (116), 2012 | 17 | • TV >15.8 mL or TV% >12.8% | • End-diastole | Long axis | |
CMR | Petersen et al. (117), 2005 | 7 | • N/C >2.3 in diastole • N/C ratio >2.3 in 43% of subjects with no cardiac disease or hypertension |
• End-diastole, long axis apical views• Sensitivity, 86%; specificity, 99% | Long axis |
Jacquier et al. (118), 2010 | 16 | • Total NC mass compared to the total LV mass • Total NC mass >20% of the total LV mass |
• End-diastole, short axis cine images• Sensitivity, 93.7%; specificity, 93.7% | Short axis | |
Grothoff et al. (119), 2012 | 12 | • Total NC mass index of 15 g/m2 of global LV mass with an NC mass >25% of the global LV mass | • End-diastole, short axis cine images• High sensitivity and specificity | Short axis | |
Stacey et al. (120), 2013 | 122 | • N/C ratio >2 in end-systole • End systolic criteria were shown to have a higher odds ratio for cardiac events compared to end diastolic criteria |
• End-systole using short axis SSFP images | Short axis | |
Captur et al. (121), 2013 | 30 | • FD max apical of ≥1.392 was considered diagnostic for LVNC • FD was significantly higher in patients with LVNC compared to healthy volunteers |
• Used fractal analysis to quantify the LVNC structure along the cardiac apex • CMR short axis sine images were reviewed using a box counting method |
Short axis | |
Choi et al. (122), 2016 | 54 | • Ratio of the thickness of apical trabeculation to that of C myocardium in the apical lateral segments (apex/C ratio) measured during end-diastole in all three long axis views • TV >35% total |
• End-systole | Short axis | |
MDCT | Melendez-Ramirez et al. (123), 2012 | 10 | • N/C ratio >2.2 at end-diastole seen in >2 segments | • Diastole, visualization of 17 heart segments• Sensitivity 100%, specificity 95% | Short axis |
Table adapted with permission; for full references, see source Abela and D’Silva (124). CMR, cardiac magnetic resonance; MDCT, multidetector computed tomography; X, distance of the epicardial layer to the trabecular trough; Y, distance of the epicardial layer to the trabecular peak; NC, noncompacted layer; LV, left ventricle; FD, fractal dimension; C, compacted layer; Lax, long axis view; TV, trabeculated left ventricular volume; TV%, percentage trabeculated left ventricular volume as a proportion of the left ventricular end diastolic cavity; SSFP, steady-state free precession.
Echocardiography
Most studies on echocardiography use the diagnostic criteria of Chin, Jenni, and Stöllberger et al. (Table 2) (1,131). The first echocardiographic criteria were proposed by Chin et al. (1). This criterion proposed the ratio of the distance from the epicardium to the trabecular trough (X) to the trabecular peak (Y) is diagnostic of LVNC if X/Y is less than 0.5. Jenni et al. proposed a second criterion (2). They proposed a maximum ratio of N/C >2 as a diagnostic criterion for LVNC. Measurements in this study were made on the parasternal short-axis image at end-systole. Currently, this criterion is the most widely accepted and clinically applied echocardiographic criterion for the diagnosis of LVNC. Stöllberger et al. proposed the third criterion (132). They proposed that the presence of three or more trabeculae at the tip of the papillary muscle and blood flow by color Doppler imaging or evidence of echo-enhancing agents in the interventricular recesses should be required.
Joong et al. compared the usefulness and reproducibility of the previously mentioned Chin, Jenni, and Stöllberger echocardiographic criteria and found that Chin’s criterion has the highest reproducibility and interrater reliability for counting the parasternal short axis and apical trabeculae using the X/Y ratio in the apical anterolateral region (133). Jenni and Stöllberger’s criteria are probably overdiagnosed in many adult HF cases that meet the diagnostic criteria. Moreover, echocardiography has several limitations. First, in the literature, echocardiographic diagnostic criteria vary widely and are based on a small number of studies using different research methods (134,135). Second, since myocardial thickness is greatest in systole and least in diastole, the cardiac cycle (end-systole or end-diastole) during which the uncompressed and compressed layers are measured is also important, which directly affects the N/C ratio. Paterick et al. suggest that the two-layer myocardium be measured at end-diastole because the thickness of each layer can be more accurately measured, and this approach is in line with the end-diastole. They proposed measuring the N/C ratio of the LV myocardium at end-diastole and diagnosing with a ratio >2 (136). This approach is consistent with the recommendation of the American Society of Echocardiography to measure wall thickness at the end of diastole (113).
Another common problem in echocardiographic criteria for LVNC is that interobserver reliability tends to be suboptimal. Saleeb et al. compared 104 LVNC cases with 100 controls and found that interobserver agreement in counts of trabeculations >3 and N/C ratio >2 ranged from 53% to 77% and did not statistically factor in the possibility of chance agreement (137). Stöllberger et al. recently evaluated the interobserver reliability among three observers evaluating 100 echocardiograms of LVNC patients and reported discordance in 35% of cases (128). Related to all LVNC diagnostic studies assessing the validity and inter-rater reliability is the lack of a gold standard for diagnosis, as many of these studies rely on the original echocardiographic diagnosis as their overarching criteria. Furthermore, these echocardiographic diagnostic tests may be too sensitive, as suggested by Kohli et al. They studied echocardiograms of patients referred for HF and found that 24% met one or more of the LVNC criteria (138).
CMR
If uncertainty still exists in the diagnosis of LVNC, additional diagnoses, such as CMR, are warranted (138). The most widely accepted criteria for CMR diagnosis of LVNC were proposed by Petersen et al. (117). They suggested a larger ratio (N/C >2.3) and measurements in diastole to better understand the bilayer myocardium and to improve accuracy. Jacquier et al. proposed another cross-sectional evaluation method by determining the mass of the trabecular LV (118). They proposed that trabecular LV mass be greater than 20% of the total hemispheric mass. Stacey et al. subsequently compared the Petersen and Jacquier criteria with the conventional end-systolic ratio N/C >2 proposed in the Jenni criterion and found the latter to be strongly associated with arrhythmia, HF, and subsequent hospitalization (120). Grothoff et al. presented a method to measure the LV myocardial mass index of uncompressed myocardium as an absolute value and as a percentage of total LV myocardial mass and proposed cutoffs of 15 g/m2 and 25%, respectively (119).
Positano et al. found that the Grothoff method could more accurately depict trabecular tissue compared to the Jacquier method, but both methods had adequate reproducibility (139). Similarly, Ivanov et al. investigated the prevalence of LVNC in 700 patients using the Peterson, Stacy, Jacquier, and Captur CMR criteria, and the prevalence was found to be 39% by Peterson criteria, 23% by Stacey criteria, 25% by Jacquier criteria, and 3% by Captur criteria, respectively.
The current LVNC criteria produce substantial false-positive results when the LVNC criteria are applied to healthy or HF populations (140). Echocardiography shows a prevalence of 1.3%, while CMR shows a prevalence of 14.8% (140). In athletes, echocardiography showed a prevalence of 3.2%, whereas CMR showed a higher prevalence of 27.3% (140). Zemrak et al. studied echocardiograms from an American multi-ethnic study of an atherosclerosis cohort and demonstrated that 26% met Petersen’s LVNC criterion. Interestingly, this population was reexamined during a 9.5-year follow-up period, which reaffirmed that the increased trabeculae in this healthy population were not associated with major adverse cardiac events (141). There have been several similar large cohort studies that have reached similar conclusions regarding possible overdiagnosis of imaging, poor consistency between techniques, and lack of association between trabecular severity and adverse clinical outcomes (142-145). Thus, while CMR can more easily identify changes in LVNC, it may also encompass healthy subjects.
Clinical symptoms
The major clinical manifestations of LVNC are HF, arrhythmias, and thromboembolism (10). HF is a complication in more than half of patients with LVNC, and LV systolic dysfunction is present in 84% of patients (146). LV diastolic dysfunction is also present and has been associated with marked trabeculation, myocardial ischemia, and abnormal relaxation and inflow limitation due to fibrosis (146). Hemodynamic features include decreased cardiac contractility with enlargement and thickening of the LV (146). The LV is also characterized by a high degree of cardiac contractility, with a high degree of LV hypertrophy. Arrhythmias are another common complication. Atrial fibrillation and ventricular tachycardia occur in 12.9% and 16.0% of adult patients, respectively (147). Sudden death occurs in approximately half of the patients with LVNC with arrhythmias (146). Wolff-Parkinson-White (WPW) syndrome has been reported in approximately 15% of pediatric patients with LVNC (6,146). Abnormal cardiac rhythm is frequently reported, with conduction defects in 26%, supraventricular tachycardia in 17%, and sustained or non-sustained ventricular tachycardia in 18% (11). Thromboembolism varies from 0% to 38% (5,6). Patients with reduced contractility and those with atrial fibrillation are at higher risk of systemic embolism, including cerebral emboli, and pulmonary infarction (11).
Chest pain and acute coronary syndromes are often present, which are thought to be due to decreased coronary blood flow and microcirculation (148-150). Recent studies have shown that LVNC has different symptoms depending on subtype and even genotype (16). These stratifications help predict the risk of LV systolic dysfunction and major adverse cardiac events.
Natural history
The onset of LVNC can range from neonatal to infancy, school age to adolescence, or adulthood, depending on the case, and the clinical presentation is diverse, making it easily overlooked. A recent meta-analysis estimates the prevalence, clinical presentation, natural history, and prognosis of LVNC. The cardiovascular mortality rate for children is 4.3 per 100 live births per year (151). The cardiovascular mortality rate for adults is 1.9 per 100 live births per year (152). The rate is 2.2 for those with LV ejection fraction (EF) <45% and 1.2 for those with LVEF ≥45% (152) The event rate for stroke and systemic embolism is 3.5 per 100 per year (152) The rate of ventricular arrhythmias is 2.2 per 100 person-years (152). We previously reported that neonatal and infantile-onset cases of dilated LVNC and LVNC with CHD are common, and some cases present with severe HF symptoms, subsequently half are indicated for heart transplantation or die within 10 to 15 years (17). The prognosis of newborns with mitochondrial disease or metabolic complications is worse, with a higher rate of heart transplantation compared to DCM in children (17). Additionally, fetal-onset cases have a high rate of HF and mortality (5,90,91). In contrast, young and adult-onset cases that are asymptomatic and detected by medical examination or incidentally often have isolated LVNC, are asymptomatic for a long time, and have a good prognosis (17). The arrhythmogenic form is also asymptomatic and is often diagnosed with an abnormal electrocardiogram, but the complication of lethal ventricular arrhythmias should be kept in mind (17). Screening of family members for LVNC by echocardiography and genetic testing is recommended.
Prognosis
Recent studies have reported risk factors for mortality in patients with LVNC. It appears that the prognosis may be different for children and adults. Kubik et al. suggested that the presence of atrial fibrillation, low LVEF, symptomatic HF, and enlargement of the dimensions and volume of the LV cavity, as in DCM and other cardiomyopathies were predictors of adverse outcomes in patients with LVNC (153). Sarma et al. noted that apart from lower LVEF (especially LVEF <31%), parameters such as atrial fibrillation, left atrial diameter >40 mm, advanced age, concomitant neuromuscular disease, and HF with LV dilation also lead to poor prognosis and increased mortality (154). Half of all deaths in patients with LVNC may be due to ventricular arrhythmias (155). Fragmented narrow or wide QRS complexes are associated with higher mortality and lower LVEF. The presence of a fragmented narrow QRS complex appears to be an independent predictor of all-cause mortality and heart transplantation (156). LBBB appears to be more frequent in patients with LVEF <35% (157). The LVEF, but not the degree of LV trabeculae, had an important influence on the variability of thrombolism. The risk of thromboembolism and ventricular arrhythmias in patients with LVNC was similar to that in patients with DCM (152). The presence of LGE on CMR was associated with a higher incidence of premature ventricular contractions and non-sustained ventricular tachycardia (158). Patients presenting with LGE often have a poor prognosis (95). NYHA functional class III/IV, hospitalization for HF symptoms, sustained ventricular arrhythmia, and systemic embolism are predictors of adverse outcomes (159). Of the patients with a cardioembolic cause of LVNC, approximately 93% had either atrial fibrillation or LV systolic dysfunction with a fractional shortening rate of less than 25%, and approximately 29% had both atrial fibrillation and LV dysfunction.
Genetic diagnosis may help predict the outcome of LVNC. MYH7 and ACTC1 variants have been shown to have a lower risk of major adverse cardiac events than MYBPC3 and TTN in adults (16). The cardiac phenotype of dilated LVNC was the most frequent subtype (56%) and was associated with an increased risk of major adverse cardiac events and a higher risk of LV systolic dysfunction. Moreover, MYBPC3, TTN, arrhythmia genes, and X-linked genes were genetic predictors of major adverse cardiac events (16). Recently, we found that genetic variants were linked to age at diagnosis, LV systolic dysfunction, and risk of major adverse cardiac events in children (Figure 4) (17). The existence of multiple variants is a significant risk factor for MACE occurrence (17). Children with a variant were frequently diagnosed at <1 year old, and they exhibited cardiac symptoms, LV systolic dysfunction, and a high risk of major adverse cardiac events. Interestingly, most of them had either sarcomere or mitochondrial gene variants. Furthermore, patients with double variants developed the disease in the fetal or neonatal period and had a very poor prognosis. Thus, risk factors for major adverse cardiac events were linked to genetic status. Although further large-scale studies on patients with LVNC with variants are needed to confirm and refine the identified genotype-phenotype correlations to improve patient-tailored clinical management, the broad phenotypic classification of LVNC is consistent with a more accurate genotype-phenotype.
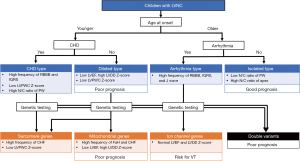
Management
There is no specific treatment for LVNC, and treatment for HF with diuretics, angiotensin-converting enzyme inhibitors, and beta-blockers should be similar to that for DCM if cardiac enlargement or reduced cardiac function is observed. Newer HF therapies, such as angiotensin receptors and neprilysin inhibitors, are expected in the future. A high rate of arrhythmias may require antiarrhythmic drugs, pacemakers, or implantable cardioverter-defibrillators. Several studies have recommended the implantation of implantable cardioverter-defibrillator devices in patients with LVNC and ventricular arrhythmias, especially those with reduced systolic function as determined by LVEF <31% (160). However, there is insufficient evidence for implantable cardioverter-defibrillators implantation in primary prevention solely due to the presence of LVNC (161). In patients with HF or atrial fibrillation, it is important to use antiplatelet and anticoagulant therapy to prevent thromboembolism (147). In some cases of LVNC, cardiac resynchronization therapy may be effective because of dyssynchrony at the site of the meatus. cardiac resynchronization therapy should be considered to reverse LV regeneration and reduce the incidence of fatal ventricular arrhythmias and sudden cardiac death in the presence of severe systolic dysfunction (162). An increasing number of patients with severe HF are eligible for heart transplantation and are receiving ventricular assist devices and awaiting heart transplantation. The number of patients who have received a ventricular assist device remains low, and more large-scale studies are needed to chart a treatment strategy for severe HF cases.
Conclusions
This present review addressed the latest findings on genes reported to be associated with LVNC morphogenesis and possible pathologies in human or non-human model organisms. It reflects the current lack of clarity regarding the pathogenesis and significance of LVNC. This is due to the complexity of imaging diagnostic criteria, interpretation of the role of LVNC as a cause or contributor to cardiomyopathy, and uncertainty regarding the specific genetic basis of LVNC. Hence, the current review highlights both the complexity and heterogeneity of the disease and the continuing need for higher quality evidence. Further extensive genetic and imaging profiling of the patient population would help clarify the risk of LVNC. We hope that this review will not only serve to help with the current status and limitations of LVNC findings but will also help identify areas where current research is lacking and where future efforts may be of interest.
Acknowledgments
Funding: None.
Footnote
Provenance and Peer Review: This article was commissioned by the Guest Editors (Yskert von Kodolitsch, Harald Kaemmerer and Koichiro Niwa) for the series “Current Management Aspects in Adult Congenital Heart Disease (ACHD): Part V” published in Cardiovascular Diagnosis and Therapy. The article has undergone external peer review.
Reporting Checklist: The authors have completed the Narrative Review reporting checklist. Available at: https://cdt.amegroups.com/article/view/10.21037/cdt-22-198/rc
Peer Review File: Available at: https://cdt.amegroups.com/article/view/10.21037/cdt-22-198/prf
Conflicts of Interest: Both authors have completed the ICMJE uniform disclosure form (available at https://cdt.amegroups.com/article/view/10.21037/cdt-22-198/coif). The series “Current Management Aspects in Adult Congenital Heart Disease (ACHD): Part V” was commissioned by the editorial office without any funding or sponsorship. The authors have no other conflicts of interest to declare.
Ethical Statement: The authors are accountable for all aspects of the work in ensuring that questions related to the accuracy or integrity of any part of the work are appropriately investigated and resolved.
Open Access Statement: This is an Open Access article distributed in accordance with the Creative Commons Attribution-NonCommercial-NoDerivs 4.0 International License (CC BY-NC-ND 4.0), which permits the non-commercial replication and distribution of the article with the strict proviso that no changes or edits are made and the original work is properly cited (including links to both the formal publication through the relevant DOI and the license). See: https://creativecommons.org/licenses/by-nc-nd/4.0/.
References
- Chin TK, Perloff JK, Williams RG, et al. Isolated noncompaction of left ventricular myocardium. A study of eight cases. Circulation 1990;82:507-13. [Crossref] [PubMed]
- Jenni R, Oechslin E, Schneider J, et al. Echocardiographic and pathoanatomical characteristics of isolated left ventricular non-compaction: a step towards classification as a distinct cardiomyopathy. Heart 2001;86:666-71. [Crossref] [PubMed]
- Lee TM, Hsu DT, Kantor P, et al. Pediatric Cardiomyopathies. Circ Res 2017;121:855-73. [Crossref] [PubMed]
- Liu Y, Chen H, Shou W. Potential Common Pathogenic Pathways for the Left Ventricular Noncompaction Cardiomyopathy (LVNC). Pediatr Cardiol 2018;39:1099-106. [Crossref] [PubMed]
- Pignatelli RH, McMahon CJ, Dreyer WJ, et al. Clinical characterization of left ventricular noncompaction in children: a relatively common form of cardiomyopathy. Circulation 2003;108:2672-8. [Crossref] [PubMed]
- Ichida F, Hamamichi Y, Miyawaki T, et al. Clinical features of isolated noncompaction of the ventricular myocardium: long-term clinical course, hemodynamic properties, and genetic background. J Am Coll Cardiol 1999;34:233-40. [Crossref] [PubMed]
- Ichida F, Tsubata S, Bowles KR, et al. Novel gene mutations in patients with left ventricular noncompaction or Barth syndrome. Circulation 2001;103:1256-63. [Crossref] [PubMed]
- Towbin JA, Lorts A, Jefferies JL. Left ventricular non-compaction cardiomyopathy. Lancet 2015;386:813-25. [Crossref] [PubMed]
- Klaassen S, Probst S, Oechslin E, et al. Mutations in sarcomere protein genes in left ventricular noncompaction. Circulation 2008;117:2893-901. [Crossref] [PubMed]
- Towbin JA, Jefferies JL. Cardiomyopathies Due to Left Ventricular Noncompaction, Mitochondrial and Storage Diseases, and Inborn Errors of Metabolism. Circ Res 2017;121:838-54. [Crossref] [PubMed]
- Kayvanpour E, Sedaghat-Hamedani F, Gi WT, et al. Clinical and genetic insights into non-compaction: a meta-analysis and systematic review on 7598 individuals. Clin Res Cardiol 2019;108:1297-308. [PubMed]
- Towbin JA. Left ventricular noncompaction: a new form of heart failure. Heart Fail Clin 2010;6:453-69. viii. [Crossref] [PubMed]
- Parent JJ, Towbin JA, Jefferies JL. Left ventricular noncompaction in a family with lamin A/C gene mutation. Tex Heart Inst J 2015;42:73-6. [Crossref] [PubMed]
- Hirono K, Hata Y, Miyao N, et al. Left Ventricular Noncompaction and Congenital Heart Disease Increases the Risk of Congestive Heart Failure. J Clin Med 2020;9:785. [Crossref] [PubMed]
- Arbustini E, Narula N, Tavazzi L, et al. The MOGE(S) classification of cardiomyopathy for clinicians. J Am Coll Cardiol 2014;64:304-18. [Crossref] [PubMed]
- van Waning JI, Moesker J, Heijsman D, et al. Systematic Review of Genotype-Phenotype Correlations in Noncompaction Cardiomyopathy. J Am Heart Assoc 2019;8:e012993. [Crossref] [PubMed]
- Hirono K, Hata Y, Miyao N, et al. Increased Burden of Ion Channel Gene Variants Is Related to Distinct Phenotypes in Pediatric Patients With Left Ventricular Noncompaction. Circ Genom Precis Med 2020;13:e002940. [Crossref] [PubMed]
- Rojanasopondist P, Nesheiwat L, Piombo S, et al. Genetic Basis of Left Ventricular Noncompaction. Circ Genom Precis Med 2022;15:e003517. [Crossref] [PubMed]
- Opdal SH, Vege A, Egeland T, et al. Possible role of mtDNA mutations in sudden infant death. Pediatr Neurol 2002;27:23-9. [Crossref] [PubMed]
- Tang S, Batra A, Zhang Y, et al. Left ventricular noncompaction is associated with mutations in the mitochondrial genome. Mitochondrion 2010;10:350-7. [Crossref] [PubMed]
- Zarrouk Mahjoub S, Mehri S, Ourda F, et al. Transition m.3308T>C in the ND1 gene is associated with left ventricular hypertrabeculation/noncompaction. Cardiology 2011;118:153-8. [Crossref] [PubMed]
- Liu S, Bai Y, Huang J, et al. Do mitochondria contribute to left ventricular non-compaction cardiomyopathy? New findings from myocardium of patients with left ventricular non-compaction cardiomyopathy. Mol Genet Metab 2013;109:100-6. [Crossref] [PubMed]
- Kogelnik AM, Lott MT, Brown MD, et al. MITOMAP: a human mitochondrial genome database. Nucleic Acids Res 1996;24:177-9. [Crossref] [PubMed]
- Holmgren D, Wåhlander H, Eriksson BO, et al. Cardiomyopathy in children with mitochondrial disease; clinical course and cardiological findings. Eur Heart J 2003;24:280-8. [Crossref] [PubMed]
- Imai-Okazaki A, Kishita Y, Kohda M, et al. Cardiomyopathy in children with mitochondrial disease: Prognosis and genetic background. Int J Cardiol 2019;279:115-21. [Crossref] [PubMed]
- Azevedo O, Gaspar P, Sá Miranda C, et al. Left ventricular noncompaction in a patient with fabry disease: overdiagnosis, morphological manifestation of fabry disease or two unrelated rare conditions in the same patient? Cardiology 2011;119:155-9. [Crossref] [PubMed]
- Stöllberger C, Finsterer J, Voigtländer T, et al. Is left ventricular hypertrabeculation/ noncompaction a cardiac manifestation of Fabry's disease? Z Kardiol 2003;92:966-9. [Crossref] [PubMed]
- Van Der Starre P, Deuse T, Pritts C, et al. Late profound muscle weakness following heart transplantation due to Danon disease. Muscle Nerve 2013;47:135-7. [Crossref] [PubMed]
- Yilmaz S, Gokben S, Serdaroglu G, et al. The expanding phenotypic spectrum of ARFGEF2 gene mutation: Cardiomyopathy and movement disorder. Brain Dev 2016;38:124-7. [Crossref] [PubMed]
- Davey KM, Parboosingh JS, McLeod DR, et al. Mutation of DNAJC19, a human homologue of yeast inner mitochondrial membrane co-chaperones, causes DCMA syndrome, a novel autosomal recessive Barth syndrome-like condition. J Med Genet 2006;43:385-93. [Crossref] [PubMed]
- Prada CE, Jefferies JL, Grenier MA, et al. Malonyl coenzyme A decarboxylase deficiency: early dietary restriction and time course of cardiomyopathy. Pediatrics 2012;130:e456-60. [Crossref] [PubMed]
- Profitlich LE, Kirmse B, Wasserstein MP, et al. High prevalence of structural heart disease in children with cblC-type methylmalonic aciduria and homocystinuria. Mol Genet Metab 2009;98:344-8. [Crossref] [PubMed]
- Bainbridge MN, Davis EE, Choi WY, et al. Loss of Function Mutations in NNT Are Associated With Left Ventricular Noncompaction. Circ Cardiovasc Genet 2015;8:544-52. [Crossref] [PubMed]
- Martinez HR, Belmont JW, Craigen WJ, et al. Left ventricular noncompaction in Sotos syndrome. Am J Med Genet A 2011;155A:1115-8. [Crossref] [PubMed]
- Facher JJ, Regier EJ, Jacobs GH, et al. Cardiomyopathy in Coffin-Lowry syndrome. Am J Med Genet A 2004;128A:176-8. [Crossref] [PubMed]
- Chang B, Gorbea C, Lezin G, et al. 14-3-3ε gene variants in a Japanese patient with left ventricular noncompaction and hypoplasia of the corpus callosum. Gene 2013;515:173-80. [Crossref] [PubMed]
- Martinez HR, Niu MC, Sutton VR, et al. Coffin-Lowry syndrome and left ventricular noncompaction cardiomyopathy with a restrictive pattern. Am J Med Genet A 2011;155A:3030-4. [Crossref] [PubMed]
- Stähli BE, Gebhard C, Biaggi P, et al. Left ventricular non-compaction: prevalence in congenital heart disease. Int J Cardiol 2013;167:2477-81. [Crossref] [PubMed]
- Digilio MC, Bernardini L, Gagliardi MG, et al. Syndromic non-compaction of the left ventricle: associated chromosomal anomalies. Clin Genet 2013;84:362-7. [Crossref] [PubMed]
- Bartman T, Hove J. Mechanics and function in heart morphogenesis. Dev Dyn 2005;233:373-81. [Crossref] [PubMed]
- Taber LA. Mechanical aspects of cardiac development. Prog Biophys Mol Biol 1998;69:237-55. [Crossref] [PubMed]
- Moorman AF, Christoffels VM, Anderson RH, et al. The heart-forming fields: one or multiple? Philos Trans R Soc Lond B Biol Sci 2007;362:1257-65. [Crossref] [PubMed]
- Sedmera D, Pexieder T, Vuillemin M, et al. Developmental patterning of the myocardium. Anat Rec 2000;258:319-37. [Crossref] [PubMed]
- Dorri F, Niederer PF, Redmann K, et al. An analysis of the spatial arrangement of the myocardial aggregates making up the wall of the left ventricle. Eur J Cardiothorac Surg 2007;31:430-7. [Crossref] [PubMed]
- Lunkenheimer PP, Redmann K, Kling N, et al. Three-dimensional architecture of the left ventricular myocardium. Anat Rec A Discov Mol Cell Evol Biol 2006;288:565-78. [Crossref] [PubMed]
- Anderson RH, Ho SY, Sanchez-Quintana D, et al. Heuristic problems in defining the three-dimensional arrangement of the ventricular myocytes. Anat Rec A Discov Mol Cell Evol Biol 2006;288:579-86. [Crossref] [PubMed]
- Lunkenheimer PP, Redmann K, Westermann P, et al. The myocardium and its fibrous matrix working in concert as a spatially netted mesh: a critical review of the purported tertiary structure of the ventricular mass. Eur J Cardiothorac Surg 2006;29:S41-9. [Crossref] [PubMed]
- Schmid P, Lunkenheimer PP, Redmann K, et al. Statistical analysis of the angle of intrusion of porcine ventricular myocytes from epicardium to endocardium using diffusion tensor magnetic resonance imaging. Anat Rec (Hoboken) 2007;290:1413-23. [Crossref] [PubMed]
- Anderson RH, Sanchez-Quintana D, Redmann K, et al. How are the myocytes aggregated so as to make up the ventricular mass? Semin Thorac Cardiovasc Surg Pediatr Card Surg Annu 2007;76-86. [Crossref] [PubMed]
- Anderson RH, Sanchez-Quintana D, Niederer P, et al. Structural-functional correlates of the 3-dimensional arrangement of the myocytes making up the ventricular walls. J Thorac Cardiovasc Surg 2008;136:10-8. [Crossref] [PubMed]
- Torrent-Guasp F, Kocica MJ, Corno AF, et al. Towards new understanding of the heart structure and function. Eur J Cardiothorac Surg 2005;27:191-201. [Crossref] [PubMed]
- van Dalen BM, Caliskan K, Soliman OI, et al. Left ventricular solid body rotation in non-compaction cardiomyopathy: a potential new objective and quantitative functional diagnostic criterion? Eur J Heart Fail 2008;10:1088-93. [Crossref] [PubMed]
- van Dalen BM, Caliskan K, Soliman OI, et al. Diagnostic value of rigid body rotation in noncompaction cardiomyopathy. J Am Soc Echocardiogr 2011;24:548-55. [Crossref] [PubMed]
- Choquet C, Kelly RG, Miquerol L. Defects in Trabecular Development Contribute to Left Ventricular Noncompaction. Pediatr Cardiol 2019;40:1331-8. [Crossref] [PubMed]
- D'Amato G, Luxán G, del Monte-Nieto G, et al. Sequential Notch activation regulates ventricular chamber development. Nat Cell Biol 2016;18:7-20. [Crossref] [PubMed]
- D'Amato G, Luxán G, de la Pompa JL. Notch signalling in ventricular chamber development and cardiomyopathy. FEBS J 2016;283:4223-37. [Crossref] [PubMed]
- Luxán G, Casanova JC, Martínez-Poveda B, et al. Mutations in the NOTCH pathway regulator MIB1 cause left ventricular noncompaction cardiomyopathy. Nat Med 2013;19:193-201. [Crossref] [PubMed]
- Wald R, Veldtman G, Golding F, et al. Determinants of outcome in isolated ventricular noncompaction in childhood. Am J Cardiol 2004;94:1581-4. [Crossref] [PubMed]
- Kodo K, Ong SG, Jahanbani F, et al. iPSC-derived cardiomyocytes reveal abnormal TGF-β signalling in left ventricular non-compaction cardiomyopathy. Nat Cell Biol 2016;18:1031-42. [Crossref] [PubMed]
- Grego-Bessa J, Luna-Zurita L, del Monte G, et al. Notch signaling is essential for ventricular chamber development. Dev Cell 2007;12:415-29. [Crossref] [PubMed]
- VanDusen NJ, Casanovas J, Vincentz JW, et al. Hand2 is an essential regulator for two Notch-dependent functions within the embryonic endocardium. Cell Rep 2014;9:2071-83. [Crossref] [PubMed]
- Takahata M, Inoue Y, Tsuda H, et al. SKI and MEL1 cooperate to inhibit transforming growth factor-beta signal in gastric cancer cells. J Biol Chem 2009;284:3334-44. [Crossref] [PubMed]
- Warner DR, Horn KH, Mudd L, et al. PRDM16/MEL1: a novel Smad binding protein expressed in murine embryonic orofacial tissue. Biochim Biophys Acta 2007;1773:814-20. [Crossref] [PubMed]
- Bjork BC, Turbe-Doan A, Prysak M, et al. Prdm16 is required for normal palatogenesis in mice. Hum Mol Genet 2010;19:774-89. [Crossref] [PubMed]
- Hirai M, Arita Y, McGlade CJ, et al. Adaptor proteins NUMB and NUMBL promote cell cycle withdrawal by targeting ERBB2 for degradation. J Clin Invest 2017;127:569-82. [Crossref] [PubMed]
- Jenkins SJ, Hutson DR, Kubalak SW. Analysis of the proepicardium-epicardium transition during the malformation of the RXRalpha-/- epicardium. Dev Dyn 2005;233:1091-101. [Crossref] [PubMed]
- Kwee L, Baldwin HS, Shen HM, et al. Defective development of the embryonic and extraembryonic circulatory systems in vascular cell adhesion molecule (VCAM-1) deficient mice. Development 1995;121:489-503. [Crossref] [PubMed]
- Pennisi DJ, Ballard VL, Mikawa T. Epicardium is required for the full rate of myocyte proliferation and levels of expression of myocyte mitogenic factors FGF2 and its receptor, FGFR-1, but not for transmural myocardial patterning in the embryonic chick heart. Dev Dyn 2003;228:161-72. [Crossref] [PubMed]
- Tevosian SG, Deconinck AE, Tanaka M, et al. FOG-2, a cofactor for GATA transcription factors, is essential for heart morphogenesis and development of coronary vessels from epicardium. Cell 2000;101:729-39. [Crossref] [PubMed]
- Tomanek RJ, Ishii Y, Holifield JS, et al. VEGF family members regulate myocardial tubulogenesis and coronary artery formation in the embryo. Circ Res 2006;98:947-53. [Crossref] [PubMed]
- Giordano FJ, Gerber HP, Williams SP, et al. A cardiac myocyte vascular endothelial growth factor paracrine pathway is required to maintain cardiac function. Proc Natl Acad Sci U S A 2001;98:5780-5. [Crossref] [PubMed]
- Wu B, Zhang Z, Lui W, et al. Endocardial cells form the coronary arteries by angiogenesis through myocardial-endocardial VEGF signaling. Cell 2012;151:1083-96. [Crossref] [PubMed]
- Stankunas K, Hang CT, Tsun ZY, et al. Endocardial Brg1 represses ADAMTS1 to maintain the microenvironment for myocardial morphogenesis. Dev Cell 2008;14:298-311. [Crossref] [PubMed]
- Jay PY, Berul CI, Tanaka M, et al. Cardiac conduction and arrhythmia: insights from Nkx2.5 mutations in mouse and humans. Novartis Found Symp 2003;250:227-38; discussion 238-41, 276-9.
- Tanaka M, Berul CI, Ishii M, et al. A mouse model of congenital heart disease: cardiac arrhythmias and atrial septal defect caused by haploinsufficiency of the cardiac transcription factor Csx/Nkx2.5. Cold Spring Harb Symp Quant Biol 2002;67:317-25. [Crossref] [PubMed]
- Choquet C, Nguyen THM, Sicard P, et al. Deletion of Nkx2-5 in trabecular myocardium reveals the developmental origins of pathological heterogeneity associated with ventricular non-compaction cardiomyopathy. PLoS Genet 2018;14:e1007502. [Crossref] [PubMed]
- Chang CP, Bruneau BG. Epigenetics and cardiovascular development. Annu Rev Physiol 2012;74:41-68. [Crossref] [PubMed]
- Mysliwiec MR, Bresnick EH, Lee Y. Endothelial Jarid2/Jumonji is required for normal cardiac development and proper Notch1 expression. J Biol Chem 2011;286:17193-204. [Crossref] [PubMed]
- Fang S, Li J, Xiao Y, et al. Tet inactivation disrupts YY1 binding and long-range chromatin interactions during embryonic heart development. Nat Commun 2019;10:4297. [Crossref] [PubMed]
- Rhee S, Chung JI, King DA, et al. Endothelial deletion of Ino80 disrupts coronary angiogenesis and causes congenital heart disease. Nat Commun 2018;9:368. [Crossref] [PubMed]
- Gati S, Chandra N, Bennett RL, et al. Increased left ventricular trabeculation in highly trained athletes: do we need more stringent criteria for the diagnosis of left ventricular non-compaction in athletes? Heart 2013;99:401-8. [Crossref] [PubMed]
- D'Ascenzi F, Pelliccia A, Natali BM, et al. Exercise-induced left-ventricular hypertrabeculation in athlete’s heart. Int J Cardiol 2015;181:320-2. [Crossref] [PubMed]
- Caselli S, Attenhofer Jost CH, Jenni R, et al. Left Ventricular Noncompaction Diagnosis and Management Relevant to Pre-participation Screening of Athletes. Am J Cardiol 2015;116:801-8. [Crossref] [PubMed]
- Piga A, Longo F, Musallam KM, et al. Left ventricular noncompaction in patients with β-thalassemia: uncovering a previously unrecognized abnormality. Am J Hematol 2012;87:1079-83. [Crossref] [PubMed]
- Gati S, Papadakis M, Van Niekerk N, et al. Increased left ventricular trabeculation in individuals with sickle cell anaemia: physiology or pathology? Int J Cardiol 2013;168:1658-60. [Crossref] [PubMed]
- Gati S, Papadakis M, Papamichael ND, et al. Reversible de novo left ventricular trabeculations in pregnant women: implications for the diagnosis of left ventricular noncompaction in low-risk populations. Circulation 2014;130:475-83. [Crossref] [PubMed]
- Markovic NS, Dimkovic N, Damjanovic T, et al. Isolated ventricular noncompaction in patients with chronic renal failure. Clin Nephrol 2008;70:72-6. [Crossref] [PubMed]
- Halaney DL, Sanyal A, Nafissi NA, et al. The Effect of Trabeculae Carneae on Left Ventricular Diastolic Compliance: Improvement in Compliance With Trabecular Cutting. J Biomech Eng 2017; [Crossref] [PubMed]
- Paun B, Bijnens B, Butakoff C. Relationship between the left ventricular size and the amount of trabeculations. Int J Numer Method Biomed Eng 2018; [Crossref] [PubMed]
- Hirono K, Hata Y, Ozawa SW, et al. A burden of sarcomere gene variants in fetal-onset patients with left ventricular noncompaction. Int J Cardiol 2021;328:122-9. [Crossref] [PubMed]
- Ozawa SW, Takarada S, Okabe M, et al. Clinical Characteristics and Prognosis of Fetal Left Ventricular Noncompaction in Japan. Circ J 2021;86:98-105. [Crossref] [PubMed]
- Zuccarino F, Vollmer I, Sanchez G, et al. Left ventricular noncompaction: imaging findings and diagnostic criteria. AJR Am J Roentgenol 2015;204:W519-30. [Crossref] [PubMed]
- Wang C, Takasaki A, Watanabe Ozawa S, et al. Long-Term Prognosis of Patients With Left Ventricular Noncompaction - Comparison Between Infantile and Juvenile Types. Circ J 2017;81:694-700. [Crossref] [PubMed]
- Finsterer J, Stöllberger C. Apical noncompaction in metabolic myopathy may be missed on echocardiography but visible on cardiac MRI or misinterpreted as apical hypokinesia. Int J Cardiol 2012;160:e15-7. [Crossref] [PubMed]
- Grigoratos C, Barison A, Ivanov A, et al. Meta-Analysis of the Prognostic Role of Late Gadolinium Enhancement and Global Systolic Impairment in Left Ventricular Noncompaction. JACC Cardiovasc Imaging 2019;12:2141-51. [Crossref] [PubMed]
- Nucifora G, Aquaro GD, Pingitore A, et al. Myocardial fibrosis in isolated left ventricular non-compaction and its relation to disease severity. Eur J Heart Fail 2011;13:170-6. [Crossref] [PubMed]
- Jellis CL, Kwon DH. Myocardial T1 mapping: modalities and clinical applications. Cardiovasc Diagn Ther 2014;4:126-37. [PubMed]
- Bull S, White SK, Piechnik SK, et al. Human non-contrast T1 values and correlation with histology in diffuse fibrosis. Heart 2013;99:932-7. [Crossref] [PubMed]
- Ferreira VM, Piechnik SK, Robson MD, et al. Myocardial tissue characterization by magnetic resonance imaging: novel applications of T1 and T2 mapping. J Thorac Imaging 2014;29:147-54. [Crossref] [PubMed]
- Zhou H, Lin X, Fang L, et al. Characterization of Compacted Myocardial Abnormalities by Cardiac Magnetic Resonance With Native T1 Mapping in Left Ventricular Non-Compaction Patients - A Comparison With Late Gadolinium Enhancement. Circ J 2016;80:1210-6. [Crossref] [PubMed]
- Araujo-Filho JAB, Assuncao AN Jr, Tavares de Melo MD, et al. Myocardial T1 mapping and extracellular volume quantification in patients with left ventricular non-compaction cardiomyopathy. Eur Heart J Cardiovasc Imaging 2018;19:888-95. [Crossref] [PubMed]
- Hirono K, Miyao N, Yoshinaga M, et al. A significance of school screening electrocardiogram in the patients with ventricular noncompaction. Heart Vessels 2020;35:985-95. [Crossref] [PubMed]
- Gerger D, Stöllberger C, Grassberger M, et al. Pathomorphologic findings in left ventricular hypertrabeculation/noncompaction of adults in relation to neuromuscular disorders. Int J Cardiol 2013;169:249-53. [Crossref] [PubMed]
- Ker J, Du Toit-Prinsloo L, Van Heerden WF, et al. Subendocardial fibrosis in left ventricular hypertrabeculation-cause or consequence? Clin Med Insights Cardiol 2011;5:13-6. [Crossref] [PubMed]
- Stöllberger C, Wegner C, Finsterer J. Fetal Ventricular Hypertrabeculation/Noncompaction: Clinical Presentation, Genetics, Associated Cardiac and Extracardiac Abnormalities and Outcome. Pediatr Cardiol 2015;36:1319-26. [Crossref] [PubMed]
- Finsterer J, Stöllberger C, Feichtinger H. Histological appearance of left ventricular hypertrabeculation/noncompaction. Cardiology 2002;98:162-4. [Crossref] [PubMed]
- Stehbens WE, Delahunt B, Zuccollo JM. The histopathology of endocardial sclerosis. Cardiovasc Pathol 2000;9:161-73. [Crossref] [PubMed]
- Guntheroth W, Komarniski C, Atkinson W, et al. Criterion for fetal primary spongiform cardiomyopathy: restrictive pathophysiology. Obstet Gynecol 2002;99:882-5. [Crossref] [PubMed]
- Moura C, Hillion Y, Daikha-Dahmane F, et al. Isolated non-compaction of the myocardium diagnosed in the fetus: two sporadic and two familial cases. Cardiol Young 2002;12:278-83. [Crossref] [PubMed]
- Jenni R, Wyss CA, Oechslin EN, et al. Isolated ventricular noncompaction is associated with coronary microcirculatory dysfunction. J Am Coll Cardiol 2002;39:450-4. [Crossref] [PubMed]
- Stöllberger C, Finsterer J, Blazek G. Left ventricular hypertrabeculation/noncompaction and association with additional cardiac abnormalities and neuromuscular disorders. Am J Cardiol 2002;90:899-902. [Crossref] [PubMed]
- Belanger AR, Miller MA, Donthireddi UR, et al. New classification scheme of left ventricular noncompaction and correlation with ventricular performance. Am J Cardiol 2008;102:92-6. [Crossref] [PubMed]
- Paterick TE, Tajik AJ. Left ventricular noncompaction: a diagnostically challenging cardiomyopathy. Circ J 2012;76:1556-62. [Crossref] [PubMed]
- Gebhard C, Stähli BE, Greutmann M, et al. Reduced left ventricular compacta thickness: a novel echocardiographic criterion for non-compaction cardiomyopathy. J Am Soc Echocardiogr 2012;25:1050-7. [Crossref] [PubMed]
- Yubbu P, Nawaytou HM, Calderon-Anyosa R, et al. Diagnostic value of myocardial deformation pattern in children with noncompaction cardiomyopathy. Int J Cardiovasc Imaging 2018;34:1529-39. [Crossref] [PubMed]
- Caselli S, Autore C, Serdoz A, et al. Three-dimensional echocardiographic characterization of patients with left ventricular noncompaction. J Am Soc Echocardiogr 2012;25:203-9. [Crossref] [PubMed]
- Petersen SE, Selvanayagam JB, Wiesmann F, et al. Left ventricular non-compaction: insights from cardiovascular magnetic resonance imaging. J Am Coll Cardiol 2005;46:101-5. [Crossref] [PubMed]
- Jacquier A, Thuny F, Jop B, et al. Measurement of trabeculated left ventricular mass using cardiac magnetic resonance imaging in the diagnosis of left ventricular non-compaction. Eur Heart J 2010;31:1098-104. [Crossref] [PubMed]
- Grothoff M, Pachowsky M, Hoffmann J, et al. Value of cardiovascular MR in diagnosing left ventricular non-compaction cardiomyopathy and in discriminating between other cardiomyopathies. Eur Radiol 2012;22:2699-709. [Crossref] [PubMed]
- Stacey RB, Andersen MM, St Clair M, et al. Comparison of systolic and diastolic criteria for isolated LV noncompaction in CMR. JACC Cardiovasc Imaging 2013;6:931-40. [Crossref] [PubMed]
- Captur G, Muthurangu V, Cook C, et al. Quantification of left ventricular trabeculae using fractal analysis. J Cardiovasc Magn Reson 2013;15:36. [Crossref] [PubMed]
- Choi Y, Kim SM, Lee SC, et al. Quantification of left ventricular trabeculae using cardiovascular magnetic resonance for the diagnosis of left ventricular non-compaction: evaluation of trabecular volume and refined semi-quantitative criteria. J Cardiovasc Magn Reson 2016;18:24. [Crossref] [PubMed]
- Melendez-Ramirez G, Castillo-Castellon F, Espinola-Zavaleta N, et al. Left ventricular noncompaction: a proposal of new diagnostic criteria by multidetector computed tomography. J Cardiovasc Comput Tomogr 2012;6:346-54. [Crossref] [PubMed]
- Abela M, D'Silva A. Left Ventricular Trabeculations in Athletes: Epiphenomenon or Phenotype of Disease? Curr Treat Options Cardiovasc Med 2018;20:100. [Crossref] [PubMed]
- André F, Burger A, Loßnitzer D, et al. Reference values for left and right ventricular trabeculation and non-compacted myocardium. Int J Cardiol 2015;185:240-7. [Crossref] [PubMed]
- Dawson DK, Maceira AM, Raj VJ, et al. Regional thicknesses and thickening of compacted and trabeculated myocardial layers of the normal left ventricle studied by cardiovascular magnetic resonance. Circ Cardiovasc Imaging 2011;4:139-46. [Crossref] [PubMed]
- Marchal P, Lairez O, Cognet T, et al. Relationship between left ventricular sphericity and trabeculation indexes in patients with dilated cardiomyopathy: a cardiac magnetic resonance study. Eur Heart J Cardiovasc Imaging 2013;14:914-20. [Crossref] [PubMed]
- Stöllberger C, Gerecke B, Engberding R, et al. Interobserver Agreement of the Echocardiographic Diagnosis of LV Hypertrabeculation/Noncompaction. JACC Cardiovasc Imaging 2015;8:1252-7. [Crossref] [PubMed]
- Gati S, Rajani R, Carr-White GS, et al. Adult left ventricular noncompaction: reappraisal of current diagnostic imaging modalities. JACC Cardiovasc Imaging 2014;7:1266-75. [Crossref] [PubMed]
- Captur G, Syrris P, Obianyo C, et al. Formation and Malformation of Cardiac Trabeculae: Biological Basis, Clinical Significance, and Special Yield of Magnetic Resonance Imaging in Assessment. Can J Cardiol 2015;31:1325-37. [Crossref] [PubMed]
- Jenni R, Rojas J, Oechslin E. Isolated noncompaction of the myocardium. N Engl J Med 1999;340:966-7. [Crossref] [PubMed]
- Stöllberger C, Gerecke B, Finsterer J, et al. Refinement of echocardiographic criteria for left ventricular noncompaction. Int J Cardiol 2013;165:463-7. [Crossref] [PubMed]
- Joong A, Hayes DA, Anderson BR, et al. Comparison of Echocardiographic Diagnostic Criteria of Left Ventricular Noncompaction in a Pediatric Population. Pediatr Cardiol 2017;38:1493-504. [Crossref] [PubMed]
- Gerecke BJ, Engberding R. Noncompaction Cardiomyopathy-History and Current Knowledge for Clinical Practice. J Clin Med 2021;10:2457. [Crossref] [PubMed]
- Cantinotti M, Scalese M, Giordano R, et al. Pediatric nomograms for left ventricle biplane 2D volumes in healthy Caucasian children. Echocardiography 2020;37:971-975. [Crossref] [PubMed]
- Paterick TE, Umland MM, Jan MF, et al. Left ventricular noncompaction: a 25-year odyssey. J Am Soc Echocardiogr 2012;25:363-75. [Crossref] [PubMed]
- Saleeb SF, Margossian R, Spencer CT, et al. Reproducibility of echocardiographic diagnosis of left ventricular noncompaction. J Am Soc Echocardiogr 2012;25:194-202. [Crossref] [PubMed]
- Kohli SK, Pantazis AA, Shah JS, et al. Diagnosis of left-ventricular non-compaction in patients with left-ventricular systolic dysfunction: time for a reappraisal of diagnostic criteria? Eur Heart J 2008;29:89-95. [Crossref] [PubMed]
- Positano V, Meloni A, Macaione F, et al. Non-compact myocardium assessment by cardiac magnetic resonance: dependence on image analysis method. Int J Cardiovasc Imaging 2018;34:1227-38. [Crossref] [PubMed]
- Ross SB, Jones K, Blanch B, et al. A systematic review and meta-analysis of the prevalence of left ventricular non-compaction in adults. Eur Heart J 2020;41:1428-36. [Crossref] [PubMed]
- Zemrak F, Ahlman MA, Captur G, et al. The relationship of left ventricular trabeculation to ventricular function and structure over a 9.5-year follow-up: the MESA study. J Am Coll Cardiol 2014;64:1971-80. [Crossref] [PubMed]
- Weir-McCall JR, Yeap PM, Papagiorcopulo C, et al. Left Ventricular Noncompaction: Anatomical Phenotype or Distinct Cardiomyopathy? J Am Coll Cardiol 2016;68:2157-65. [Crossref] [PubMed]
- Amzulescu MS, Rousseau MF, Ahn SA, et al. Prognostic Impact of Hypertrabeculation and Noncompaction Phenotype in Dilated Cardiomyopathy: A CMR Study. JACC Cardiovasc Imaging 2015;8:934-46. [Crossref] [PubMed]
- Andreini D, Pontone G, Bogaert J, et al. Long-Term Prognostic Value of Cardiac Magnetic Resonance in Left Ventricle Noncompaction: A Prospective Multicenter Study. J Am Coll Cardiol 2016;68:2166-81. [Crossref] [PubMed]
- Ivanov A, Dabiesingh DS, Bhumireddy GP, et al. Prevalence and Prognostic Significance of Left Ventricular Noncompaction in Patients Referred for Cardiac Magnetic Resonance Imaging. Circ Cardiovasc Imaging 2017;10:e006174. [Crossref] [PubMed]
- Oechslin EN, Attenhofer Jost CH, Rojas JR, et al. Long-term follow-up of 34 adults with isolated left ventricular noncompaction: a distinct cardiomyopathy with poor prognosis. J Am Coll Cardiol 2000;36:493-500. [Crossref] [PubMed]
- Hirono K, Takarada S, Miyao N, et al. Thromboembolic events in left ventricular non-compaction: comparison between children and adults - a systematic review and meta-analysis. Open Heart 2022;9:e001908. [Crossref] [PubMed]
- Emrich T, Emrich K, Abegunewardene N, et al. Cardiac MR enables diagnosis in 90% of patients with acute chest pain, elevated biomarkers and unobstructed coronary arteries. Br J Radiol 2015;88:20150025. [Crossref] [PubMed]
- Güvenç TS, Erer HB, Altay S, et al. 'Idiopathic' acute myocardial infarction in a young patient with noncompaction cardiomyopathy. Cardiol J 2012;19:429-33. [Crossref] [PubMed]
- Chen MA. Noncompaction cardiomyopathy and multiple coronary-cameral fistulae in an octogenarian. J Cardiol Cases 2020;21:227-30. [Crossref] [PubMed]
- Łuczak-Woźniak K, Werner B. Left Ventricular Noncompaction-A Systematic Review of Risk Factors in the Pediatric Population. J Clin Med 2021;10:1232. [Crossref] [PubMed]
- Aung N, Doimo S, Ricci F, et al. Prognostic Significance of Left Ventricular Noncompaction: Systematic Review and Meta-Analysis of Observational Studies. Circ Cardiovasc Imaging 2020;13:e009712. [Crossref] [PubMed]
- Kubik M, Dąbrowska-Kugacka A, Lewicka E, et al. Predictors of poor outcome in patients with left ventricular noncompaction: Review of the literature. Adv Clin Exp Med 2018;27:415-22. [Crossref] [PubMed]
- Sarma RJ, Chana A, Elkayam U. Left ventricular noncompaction. Prog Cardiovasc Dis 2010;52:264-73. [Crossref] [PubMed]
- Stöllberger C, Keller H, Blazek G, et al. Cardiac devices and neuromuscular disorders in left ventricular noncompaction. Int J Cardiol 2011;148:120-3. [Crossref] [PubMed]
- Ning XH, Tang M, Chen KP, et al. The prognostic significance of fragmented QRS in patients with left ventricular noncompaction cardiomyopathy. Can J Cardiol 2012;28:508-14. [Crossref] [PubMed]
- Akhbour S, Fellat I, Fennich N, et al. Electrocardiographic findings in correlation to magnetic resonance imaging patterns in African patients with isolated ventricular noncompaction. Anatol J Cardiol 2015;15:550-5. [Crossref] [PubMed]
- Wan J, Zhao S, Cheng H, et al. Varied distributions of late gadolinium enhancement found among patients meeting cardiovascular magnetic resonance criteria for isolated left ventricular non-compaction. J Cardiovasc Magn Reson 2013;15:20. [Crossref] [PubMed]
- Greutmann M, Mah ML, Silversides CK, et al. Predictors of adverse outcome in adolescents and adults with isolated left ventricular noncompaction. Am J Cardiol 2012;109:276-81. [Crossref] [PubMed]
- Kobza R, Jenni R, Erne P, et al. Implantable cardioverter-defibrillators in patients with left ventricular noncompaction. Pacing Clin Electrophysiol 2008;31:461-7. [Crossref] [PubMed]
- Priori SG, Blomström-Lundqvist C, Mazzanti A, et al. 2015 ESC Guidelines for the management of patients with ventricular arrhythmias and the prevention of sudden cardiac death: The Task Force for the Management of Patients with Ventricular Arrhythmias and the Prevention of Sudden Cardiac Death of the European Society of Cardiology (ESC). Endorsed by: Association for European Paediatric and Congenital Cardiology (AEPC). Eur Heart J 2015;36:2793-867. [Crossref] [PubMed]
- Okubo K, Sato Y, Matsumoto N, et al. Cardiac resynchronization and cardioverter defibrillation therapy in a patient with isolated noncompaction of the ventricular myocardium. Int J Cardiol 2009;136:e66-8. [Crossref] [PubMed]