Protective effects of Shen Yuan Dan on myocardial ischemia-reperfusion injury via the regulation of mitochondrial quality control
Highlight box
Key findings
• Shen Yuan Dan (SYD) might be a promising drug for the treatment of myocardial ischemia/reperfusion injury (MIRI).
What is known and what is new?
• SYD plays a protective role in H/R-injured cardiomyocytes by regulating mitochondrial quality.
• SYD inhibits mitophagy activity through inhibiting the PINK1/Parkin pathway.
What is the implication, and what should change now?
• The application of SYD might be effective for the amelioration of MIRI.
Introduction
Cardiovascular diseases are major contributors to death worldwide. According to published data, the incidence, mortality, and disability rates of acute myocardial infarction (MI) are still increasing (1). Cardiovascular diseases leverage a considerable burden upon society and individuals and thus have become a major public health problem (2). When myocardial ischemia occurs, restoring myocardial blood supply as soon as possible is the most important means of treatment. However, regardless of whether treatment consists of emergency drug thrombolytic therapy, interventional therapy, or cardiac bypass surgery, when the ischemic myocardial tissue is restored to blood perfusion, if the myocardial injury caused by ischemia is not completely recovered, even more serious damage may occur (3). This paradoxically kills (as opposed to rescuing) previously abundant ischemic myocytes in a process referred to as lethal myocardial ischemia-reperfusion injury (MIRI) (4-6). Fortunately, many studies have been performed to explore the drugs to improve MIRI. For example, polyphyllin I was evidenced to protect against MIRI via the suppression of inflammatory response and oxidative stress (7). The protective role of curcumin in MIRI was testified by Mokhtari-Zaer and co-workers (8).
Recent researches have proposed several molecular mechanisms that may account for the pathological changes in MIRI, including rapid reactive oxygen species (ROS) release, energy depletion, dysfunctional mitochondria, and programmed cell death trigger (9-12). Among these, mitochondria have been considered to be the critical trigger of MIRI. It has been reported that cardiomyocytes highly depend on mitochondria, which determine more than 90% of the energy supply of cardiomyocytes (13). In the course of ischemia-reperfusion (I/R), the large-scale damage of mitochondrial quality in cardiomyocytes can lead to a sharp increase in ROS level and the triggering of the mitochondrial apoptosis pathway, which are key factors in inducing MIRI (14,15). At the same time, other pathologic conditions, such as oxidative stress, immune responses, and endoplasmic reticulum stress can be triggered, integrated, or enhanced via dysfunctional mitochondria (16). The dysfunctional mitochondria can result in I/R-mediated endothelial cell damage; therefore, clearing out injured mitochondria and generating new and healthy mitochondria is pivotal for the amelioration of I/R damage (17,18).
Mitochondrial quality control (MQC) is an important mechanism to ensure mitochondrial homeostasis and keep cardiomyocytes functionally viable (19,20). MQC system is involved in a range of processes such as mitochondrial dynamics/mitophagy and mitochondria-mediated cell death (21-23), the function of which is mainly reflected in regulating the quantity and quality of mitochondria. Well-organized MQC promotes the regeneration of mitochondria, improves the biochemical processes and signaling transduction of mitochondria, and modulates the related cell death of mitochondria (24-26). Interestingly, strategies to promote mitochondrial recovery are modulated in part through the enhancement of MQC, thereby improving mitochondrial turnover as well as protecting against mitochondria-induced cell death (10,27-29). It was also reported that the regulation of mitochondrial function by COX6B1 could relieve cardiomyocytes hypoxia-reoxygenation (H/R) injury (30). Existing study considered mitochondrial dysfunction as a hallmark behind the development of cardiac malfunction induced by MIRI (31). Therefore, initiating MQC and improving dysfunctional mitochondria are pivotal approaches to preventing and treating MIRI.
Shen Yuan Dan (SYD) consists of eight crude Chinese medicinal agents named Salvia miltiorrhiza Bge, Astragalus membranaceus Bge, root of Pilose Asiabell, Radix Scrophulariae, Hirudo nipponica (Whitman), Lumbricus, Eupolyphaga sinensis (Walker), and Rhizoma Corydalis (7). As a traditional Chinese medicine (TCM) compound preparation, SYD has been shown to be effective in the clinical treatment of ischemic cardiomyopathy (32). A previous study has demonstrated that SYD can increase superoxide dismutase (SOD) activity and decrease methane dicarboxylic aldehyde (MDA) level in MIRI rats, while exerting myocardial protection through antioxidative stress (33); meanwhile, SYD was also shown to effectively maintain Na+-K+-ATPase and Ca2+-Mg2+-ATPase at relatively high levels and ameliorate the energy metabolism of ischemic myocardial tissue (33). Nevertheless, the mechanism underlying the effect of SYD on the myocardial energy metabolism is obscure. In view of this, the present study investigated the efficacy of SYD pretreatment on dysfunctional mitochondria in myocardial cells injured by hypoxia-reoxygenation (H/R) and determined whether SYD could improve energy metabolism and protect against MIRI by regulating MQC. We present the following article in accordance with the MDAR reporting checklist (available at https://cdt.amegroups.com/article/view/10.21037/cdt-23-86/rc).
Methods
Reagents and instruments
TRNzol (#DP424) was acquired from Tiangen Biotech Co., Ltd. (Beijing, China); PrimeScript RT reagent Kit with gDNA Eraser (#RR047B), SYBR Premix Ex Taq II (Tli RNaseH Plus), and ROX plus (#RR82LR) were acquired from Takara Bio (Tokyo, Japan); an methyl thiazolyl tetrazolium (MTT) assay kit, bicinchoninic acid (BCA) protein quantification kit, and a NanoDrop 2000 spectrophotometer were acquired from Thermo Fisher Scientific (Waltham, MA, USA); a RIPA Total Protein Extraction Kit was acquired from Sigma-Aldrich (St. Louis, MI, USA); a mitochondria extract kit, enzyme-linked immunosorbent assay (ELISA) kits, and mitochondrial membrane potential JC-1 kit were acquired from Solarbio Science & Technology (Beijing, China); an adenosine triphosphate (ATP) kit, superoxide dismutase (SOD) kit, and methane dicarboxylic aldehyde (MDA) kit were acquired from Nanjing Jiancheng Bioengineering Institute (Nanjing, China); a FACSCalibur II Flow Cytometer was acquired from BD Biosciences (Franklin Lakes, NJ, USA); an XDS-2B inverted fluorescence microscope was acquired from Chongqing Optical & Electrical Instrument Co., Ltd. (Chongqing, China); and an ABI 7500 real-time PCR instrument was acquired from Applied Biosystems (Thermo Fisher Scientific); the autophagy inhibitor 3-methyladenine (3MA) was purchased from Selleck Chemicals (Houston, TX, USA).
Drugs and concentration selection
The preparation of SYD lyophilized powder was completed by the Institute of Traditional Chinese Medicine, Chinese Academy of Traditional Chinese Medicine, under the following parameters: cold trap temperature, −45 ℃; and vacuum, −0.1. Furthermore, 4 mL of Dulbecco’s modified Eagle medium (DMEM) was applied to dissolve 100 mg of SYD freeze-dried powder, which was followed by filtration and sterilization. Subsequently, the storage of the collected power was conducted at 4 ℃. The dilution of powder to favorable concentration was implemented prior to experimentation (34). MTT was employed to assess the nontoxic concentration of the drug, and the results indicated that 100 µg/mL was the optimal nontoxic concentration of SYD.
Cardiomyocyte resuscitation culture and H/R model establishment
H9c2 cardiomyocytes provided by Shanghai Beinuo Biotechnology Co., Ltd. (Shanghai, China) were incubated in DMEM with high glucose containing 10% fetal bovine serum (FBS) at 37 ℃ and 5% CO2. To establish H/R model in vitro, H9c2 cells were subjected to hypoxic conditions equilibrated with 0.1% O2, 5% CO2 and 95% N2 at 37 ℃ for 6 h. After that, H9c2 cells were reoxygenated under normoxic conditions supplemented with 95% air and 5% CO2 for 12 h at 37 ℃. Following the implementation of H/R, further experiments were conducted.
Methyl thiazolyl tetrazolium (MTT) assay
The toxicity of SYD with varying concentrations (0.78, 1.56, 3.13, 6.25, 12.5, 25, 50, 100, 200 µg/mL) on H9c2 cells was detected using MTT assay kit according to the manufacturer’s instructions. Briefly, the H9c2 cells were injected into 96-well plates at the density of 2×103/well and then incubated for 24 h. Subsequently, MTT solution was added into each well and the cells were incubated for another 4 h. After that, MTT solution was discarded and the cells were exposed to dimethyl sulfoxide. Finally, the absorbance at 570 nm was measured with a spectrophotometer.
Experimental grouping and cell processing
H9c2 cells were incubated in DMEM supplemented with 10% FBS at 37 ℃ and 5% CO2. All cells were divided into four groups: (I) control group (without any treatment), (II) H/R model group [hypoxia (6 h, 0.1% O2, 5% CO2 and 95% N2) and reoxygenation (12 h, 95% air and 5% CO2)], (III) H/R model + 3MA group: (H9c2 cells were pre-treated with 2 mM 3MA for 6 h and then exposed to H/R induction, (IV) H/R model + SYD group (H9c2 cells were pre-treated with 100 µg /mL SYD for 6 h and then exposed to H/R induction).
Evaluation of change in mitochondrial membrane potential of cardiomyocytes with JC-1 staining
After the rinse with PBS, 100 µL sample cells were collected for staining. Subsequently, 1 µL of JC-1 reagent was placed into each tube and then reacted for 30 min at room temperature. After suspension, 400 µL of PBS was added, and flow cytometry was employed to count the number of JC-1 cells, with the JC1-1 fluorescence channel being FL1 and JC1-2 fluorescence channel being FL2. Data were analyzed by CellQuest software (BD Biosciences): the membrane potential was considered to be the numerical ratio of JC1-1 + JC1-2+ to JC1-1 + JC1-2−.
ATP measurement
The concentration of ATP in H9c2 cells was detected using ATP assay kits according to manufacturer’s instructions. In brief, H9c2 cells were mixed with cell lysis buffer for 10 min and then centrifugated at 12,000 g at 4 ℃ for 5 min. After the incubation with 100 µL kit solution at room temperature for 5 min, the ATP level in cell supernatant was detected.
The measurement of SOD activity and MDA content
All operations were implemented according to the manufacturer’s instructions. Following ultrasonic treatment, cell lysate was centrifugated and subsequently, the supernatant was collected to measure the levels of SOD and MDA with SOD assay kits and MDA assay kits.
Detection mitochondrial quality and dynamic changes via laser confocal microscopy
Following treatment, cells were rinsed with PBS or normal saline twice (200 µL each time) and mixed with 1:100 diluted Mito-Tracker Green dye (MTH; 150 µL/dish) away from light for 1 h. After the subjection to 4',6-diamidino-2-phenylindole (DAPI) working solution (150 µL/dish), the cells were cultivated away from light for 15 min. Finally, confocal microscopy-green-blue fluorescence was applied to capture the fluorescence of PBS-rinsed cells.
Reverse transcription-quantitative polymerase chain reaction (RT-qPCR)
Following the extraction with TRNzol, the RNA was synthesized into complement DNA (cDNA) by QuantiTect Reverse Transcription kit (Qiagen GmbH, Hilden, Germany) according to established protocols. PCR reaction was implemented using SYBR Green PCR Master Mix (Takara Bio) on a 7500 Fast Real-time PCR system. 2−ΔΔCT was employed for the determination of relative gene expression (35). The primer sequences are listed in Table 1.
Table 1
Genes | Primer | Primer sequence (5' to 3') |
---|---|---|
Mfn1 | Mfn1-F | CTTCCCTTGTACATCGATTCCT |
Mfn1-R | GGGTTAGAAGGAGCAGTAGGAG | |
Mfn2 | Mfn2-F | ATTTCTCCCTTGGATGGACTA |
Mfn2-R | AGAGAGGCCAGGCCAGTAA | |
Opa1 | Opa1-F | AGTGATGAGATTACCACAGTCC |
Opa1-R | CCTCTCCGACAAAGGTTACAGT | |
Drp1 | Drp1-F | TCTTCTAAAGTTCCAAGTGCTTTG |
Drp1-R | GAAGTTTTCAGCATTCCTCTCC | |
Fis1 | Fis1-F | AGGAAATTTCAGTCTGAGCAGG |
Fis1-R | GCCAGGTAGAAGACATAATCCC | |
PGC-1a | PGC-1a-F | CCAAACCAACAACTTTATCTCTTC |
PGC-1a-R | CTGCAGTTCCAGAGAGTTCCAC |
Mfn1, mitofusin 1; Mfn2, mitofusin 2; Opa1, optic atrophy protein 1; Drp1, dynamin-related protein 1; Fis1, fission 1; PGC-1α, peroxisome proliferator-activated receptor gamma coactivator-1α.
Western blot analysis
The quantification of total proteins, which were isolated with RIPA lysis buffer, was conducted by means of the BCA method. Following separation with 10% sodium dodecyl sulfate polyacrylamide gel electrophoresis (SDS-PAGE), 50 µg of protein lysates was transferred to a polyvinylidene fluoride (PVDF) membrane. The overnight cultivation of membranes, which were blocked by 5% skimmed milk, was operated at 4 ℃ with primary antibodies targeting LC3-I/LC3-II (ab128025; 1:1,000; Abcam), PINK1 (ab186303; 1:1,000; Abcam), Parkin (ab77924; 1:2,000; Abcam) or GAPDH (ab8245; 1:1,000; Abcam) and followed by exposure to rabbit anti-mouse IgG H&L (ab6728; 1:2,000; Abcam) or goat anti-rabbit IgG H&L antibodies (ab6721; 1:2,000; Abcam) at room temperature for 1 h. Finally, enhanced chemiluminescence (ECL) was employed for the visualization of protein blots, and the images were captured using ImageJ software (National Institutes of Health, Bethesda, MD, USA).
Statistical analysis
SPSS software version 27.0 (IBM Corp., Armonk, NY, USA) was applied for the analysis of data derived from at least 3 independent experiments and expressed as mean ± standard error of mean (SEM). To determine the differences between 2 groups, Student t-test was applied; to determine the differences across multiple groups, 1-way analysis of variance (ANOVA) Tukey’s post-hoc test was applied. The experimental data were considered to be statistically significant at a P value less than 0.05.
Results
Determination of SYD concentration and the effects of SYD on mitochondrial membrane potential of cardiomyocytes injured by H/R
As shown in Figure 1A, 100 µg/mL was the optimal nontoxic concentration of SYD. In view of this, we chose 100 µg/mL SYD for following experiments. In relation with the Control group, the mitochondrial membrane potential (MMP) in H/R group was markedly reduced, indicating that H/R caused significant damage to the mitochondria of cardiomyocytes. SYD pretreatment evidently alleviated the MMP of cardiomyocytes after H/R stimulation, suggesting that SYD administration could alleviate cardiomyocyte damage induced by H/R stimulation while the effect was not as good as that in the H/R + 3MA group when compared with the H/R + SYD group (Figure 1B,1C).
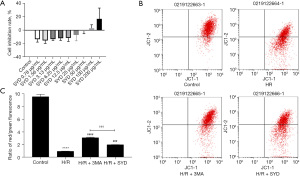
Effects of SYD on ATP content in H/R-injured cardiomyocytes
As shown in Figure 2A, ATP content in the H/R group was markedly reduced relative to the Control group. Compared with that in the H/R group, the content of ATP in the H/R-induced cardiomyocytes was dramatically increased in the H/R + SYD group and H/R + 3MA group. Nevertheless, SYD pretreatment group caused lower expression level of ATP compared to the H/R + 3MA group.
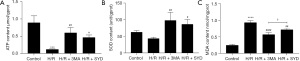
Effects of SYD on SOD and MDA in H/R-injured cardiomyocytes
As shown in Figure 2B, SOD activity was reduced by H/R stimulation, indicating that H/R could impair the antioxidant capacity of cardiomyocytes. Compared with the H/R group, both the H/R + SYD and H/R + 3MA groups showed improved SOD activity in cardiomyocytes after H/R. The high activity of SOD in the H/R + SYD group suggested that SYD could improve the antioxidant effect of H/R damage to cardiomyocytes.
Compared with the Control group, MDA content was conspicuously elevated in H/R group, indicating that H/R could significantly aggravate oxidative damage of cardiomyocytes. However, in comparison with that in the H/R group, MDA level in both the H/R + SYD and H/R + 3MA groups was diminished, indicating that SYD and 3MA could alleviate H/R-induced oxidative damage of cardiomyocytes, with the protective effect in the H/R + 3MA group being greater (Figure 2C).
Effects of SYD on the mitochondrial quality and mitochondrial dynamics in H/R-injured cardiomyocytes
In order to investigate the impact of SYD on mitochondrial quality and the dynamic changes in cardiomyocytes resulting from H/R stimulation, a mitochondria-specific fluorescent mito-tracker green (MTG) probe was applied for the co-staining of cardiomyocytes. As seen in Figure 3A,3B, the degree of mitochondrial networking was remarkably diminished in H/R group compared with the Control group, suggesting that mitochondrial division and fusion might be affected, resulting in a decreased number of mitochondria. This further indicated that H/R might lead to mitochondrial dysfunction in the cardiomyocytes and precipitate mitochondrial biosynthesis. Nevertheless, SYD strengthened the degree of mitochondrial networking in H/R-injured cardiomyocytes, and the distribution and number of mitochondria were greatly increased, suggesting that SYD regulated the number and network morphology of mitochondria by regulating the dynamic changes of mitochondria. In comparison with that in the H/R + 3MA group, the degree of mitochondrial improvement was slightly lower in H/R + SYD group.
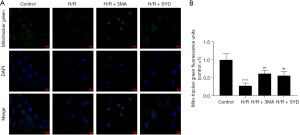
Effects of SYD on mRNA expression levels of key regulatory factors of mitochondrial fission, fusion, and mitochondrial biogenesis in H/R-injured cardiomyocytes
The role of SYD in mitochondrial fission and fusion in H/R-injured cardiomyocytes was examined. Results obtained from RT-qPCR demonstrated that the H/R induction reduced the mRNA levels of mitofusin 1 (Mfn1), mitofusin 2 (Mfn2), optic atrophy protein 1 (Opa1), and increased the mRNA levels of dynamin-related protein 1 (Drp1), and fission 1 (Fis1) relative with the Control group (Figure 4A,4B). Following the treatment with SYD, the mRNA levels of Mfn1, Mfn2 and Opa1 were increased while the expressions of Drp1 and Fis1 were reduced by contrast with those in H/R group.
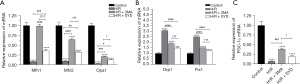
Additionally, results obtained from RT-qPCR also revealed that the level of peroxisome proliferator-activated receptor gamma coactivator-1α (PGC-1α) mRNA in H/R + SYD group was dramatically increased compared with the H/R group, suggesting that SYD promoted the transcription of PGC-1α in H/R-induced cardiomyocytes (Figure 4C).
Effects of SYD on the expression levels of LC3-II, PINK1, and Parkin in H/R-injured cardiomyocytes
As seen in Figure 5, by contrast with the Control group, LC3-II content was markedly increased in H/R group, implying that mitophagy was induced after H/R. Relative with the H/R group, the content of LC3-II in H/R + 3MA or H/R + SYD group was markedly decreased, suggesting that 3MA or SYD could suppress the mitophagy activity of H/R-damaged cardiomyocytes.
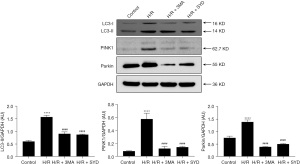
To clarify the role of PINK1/Parkin, a key regulatory pathway of mitophagy, in H/R-injured cardiomyocytes, PINK1/Parkin expression in cardiomyocytes was evaluated. As seen in Figure 5, the levels of PINK1 and Parkin proteins in cardiomyocytes were increased considerably after H/R stimulation, indicating that H/R could induce elevated mitophagy activity. Nevertheless, the levels of PINK1 and Parkin in H/R + SYD group were markedly reduced in comparison with the H/R group, indicating that SYD could inhibit the overexpression of PINK1 and Parkin in H/R-injured cardiomyocytes, thereby suppressing the occurrence of mitophagy.
Discussion
In our study, it was discovered that SYD pretreatment increased levels of MMP; raised cellular ATP content; activated mitochondrial antioxidant SOD activity; reduced MDA level; elevated the mRNA levels of Mfn1, Mfn2, Opa1 and PGC-1α; decreased the mRNA levels of Drp1 and Fis1; and reduced the protein levels of LC3 in H/R-injured cardiomyocytes. Therefore, our results demonstrated that SYD can reduce cardiomyocyte H/R injury by regulating MQC. Additionally, this study confirmed that SYD could downregulate the expressions of PINK1 and Parkin proteins in H/R-injured cardiomyocytes, thus confirming that SYD inhibited mitophagy activity by downregulating the PINK1/Parkin pathway, thereby exerting a protective effect against MIRI.
As a clinical condition that has a close relation with MI, MIRI contributes to ventricular arrhythmias, contractile dysfunction, and mortality (36). MI results from the occlusion or blockage of one or more coronary vessels supplying a region of the heart (37). As the coronary blood flow recanalizes or the collateral branches open, reperfusion of the ischemic myocardium and the immediate reintroduction of oxygen and nutrients become vital for the rescue of the reversibly injured myocardium and the limitation of infarct progression. Nevertheless, for subpopulations of reversibly injured cardiomyocytes, recovery of blood flow facilitates the death of necrotic and apoptotic cells (6,38,39). Myocardial cell death resulting from I/R is a prominent contributor to morbidity and mortality worldwide. Despite the fact that great efforts have been made, the mechanisms of lethal I/R injury, which are complicated and multifactorial, still remain obscure (40-44). Research results show that the mitochondria-centered mechanism plays an important role in the formation of I/R injury (45,46). It was also evidenced that mitochondrial dysfunction has currently been considered as a promising therapeutic target (47).
As an adaptive response, MQC adjusts the morphology and function of mitochondria in the course of MIRI (48). Following exposure to stress, mitochondrial fission is activated, and with the cooperation of mitophagy, part of the damaged mitochondria can be removed from the mitochondrial network, with small mitochondrial fragments being integrated into healthy long mitochondria. In this way, the resistance of the whole mitochondria is enhanced. Therefore, MQC is predominantly modulated by mitochondrial dynamics and mitophagy (49), while mitochondrial biogenesis is accompanied by mitophagy; these different mechanisms maintain mitochondrial quality in cells. They coordinate with each other to retain healthy mitochondria to the maximum extent while removing irreversible mitochondrial damage, and finally complete the dual regulation of mitochondrial quantity and quality. Nevertheless, it has been found that while autophagy confers protective effects to cells in the ischemic stage, in the reperfusion stage, excessive autophagy will aggravate cell damage, which may result in cell death in severe cases (50,51). The occurrence of MIRI is often accompanied by the pathological overexpression of mitochondrial fission and mitophagy, which can exacerbate the damage to heart muscle cells and even lead to cell death. Therefore, via the modulation of MQC, the trigger of mitophagy that promotes specificity, targeting, and balance may be the key factor for cardiac protection (52-54). In this study, MQC was used as the entry point to investigate the protective impacts of SYD on H/R-injured cardiomyocytes, which is expected to clarify the mechanism of SYD’s anti-MIRI action.
In TCM, the typical feature of MIRI is qi deficiency and clinical blood stasis (55). SYD was prepared in the Beijing Hospital of Traditional Chinese Medicine Capital Medical University and was registered in the Beijing Medical Products Administration (approval document No. 99 Jingwei Zi [056] F-203; National Invention Patent No. ZL201410363400.9). SYD has been broadly applied in clinical for the treatment of coronary heart disease by invigorating qi and removing blood stasis. A previous case study reported that SYD exerted myocardial protection through antioxidative stress and clearly relieved energy metabolism of the ischemic myocardial tissue (33). Liu and co-workers have evidenced that SYD could increase SOD expression in MIRI rats (7). In one study, SYD was shown to promote autophagy via the inactivation of PI3K/Akt/mTORC1 signaling pathway, thus attenuating atherosclerosis and foam cell formation (34). Additionally, SYD reduced myocardial cell injury and apoptosis in rats with acute myocardial infarction (AMI) via modulating mitochondrial apoptosis signaling pathway (56). Our study showed that SYD pretreatment could increase MMP in H/R-injured cardiomyocytes, elevate ATP content, trigger SOD activity, and reduce the MDA level in H/R-injured cardiomyocytes. This suggests that SYD can reduce oxidative stress injury and ameliorate mitochondrial function, thereby imparting a protective impact on H/R-injured cardiomyocytes. In addition, we investigated the molecular mechanism of SYD’s regulation of mitochondrial quality and anti-MIRI by observing the regulation of SYD pretreatment on mitochondrial division and fusion, mitochondrial biogenesis, and mitophagy in H/R-injured cardiomyocytes, the results from which may lay pharmacological basis for SYD’s prevention and treatment of MIRI. The Figure 6 is about the description of MQC system.
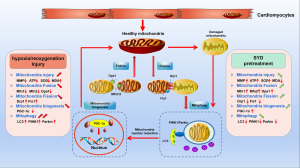
The mitochondrial fusion process is primarily regulated by 3 dynamin-related guanosine triphosphatases (GTPases) (57). Mitochondrial Fis1 protein and Drp1 are critical regulators in mitochondrial fission (58). PGC-1α is the most pivotal regulatory factor in the PPAR γ coactivator (PGC) (59,60). This study showed that SYD could regulate the mRNA levels of mitochondrial fission and fusion joint regulatory factors in H/R-injured cardiomyocytes, inhibit mitochondrial fission and promote fusion, imped the fission of the damaged mitochondria and mitochondrial network, repress substance exchange between mitochondria, and regulated mitochondrial quality. SYD also elevated PGC-1α expression in H/R-injured cardiomyocytes, thereby promoting mitochondrial biogenesis and modulating mitochondrial quality.
The classic understanding for the mechanism of mitophagy includes the mitochondrial serine/threonine PINK1/Parkin (61-63). A study has shown that the PINK1/Parkin pathway may induce excessive mitophagy in myocardial I/R, thereby promoting cell death (64), which plays an important role in maintaining the normal function of cardiomyocytes and myocardial protection in MIRI by mediating mitophagy activity (3). A growing number of studies have revealed that the regulation of PINK1/Parkin pathway is critical for the alleviation of MIRI. For example, Yang and co-workers have found that the suppression of PINK1/Parkin pathway by VDAC1 downregulation could attenuate MIRI (65). Additionally, the regulation of PINK1/Parkin pathway by the inhibition of myosin IIA-actin interaction-induced actomyosin contractility could impede MIRI (66). In this study, we found that SYD pretreatment can reduce the expression of LC-II in H/R-injured cardiomyocytes, reduce mitophagy activity, and ameliorate the injury to cardiomyocytes. Moreover, SYD pretreatment downregulated the expressions of PINK1 and Parkin proteins in H/R-injured cardiomyocytes. Thus, it is speculated that SYD may suppress excessive mitophagy activity by regulating PINK1/Parkin pathway to alleviate the myocardial injury effect of MIRI.
Conclusions
The results of our study demonstrate that SYD pretreatment significantly increases mitochondrial antioxidant capacity, alleviates the mitochondrial damage caused by H/R, regulates mitochondrial fission and fusion, upregulates mitochondrial biogenesis, and downregulates excessive mitophagy; therefore, it exerts a protective effect against H/R injury to cardiomyocytes by regulating mitochondrial quality. Meanwhile, SYD may inhibit mitophagy activity by inhibiting the PINK1/Parkin pathway, thereby protecting cardiomyocytes from I/R injury. This study offers novel insights into the underlying mechanism of the SYD alleviation of myocardial I/R injury and may provide a theoretical basis for the clinical strategies involving SYD treatment in MIRI.
Acknowledgments
Funding: This work was funded by Key Medical Professional Development Program Fund of Beijing Municipal Hospital Administration (No. ZYLX201817) and Beijing Municipal Administration of Hospitals Incubating Program (No. PZ2022023).
Footnote
Reporting Checklist: The authors have completed the MDAR reporting checklist. Available at https://cdt.amegroups.com/article/view/10.21037/cdt-23-86/rc
Data Sharing Statement: Available at https://cdt.amegroups.com/article/view/10.21037/cdt-23-86/dss
Peer Review File: Available at https://cdt.amegroups.com/article/view/10.21037/cdt-23-86/prf
Conflicts of Interest: All authors have completed the ICMJE uniform disclosure form (available at https://cdt.amegroups.com/article/view/10.21037/cdt-23-86/coif). The authors have no conflicts of interest to declare.
Ethical Statement: The authors are accountable for all aspects of the work in ensuring that questions related to the accuracy or integrity of any part of the work are appropriately investigated and resolved.
Open Access Statement: This is an Open Access article distributed in accordance with the Creative Commons Attribution-NonCommercial-NoDerivs 4.0 International License (CC BY-NC-ND 4.0), which permits the non-commercial replication and distribution of the article with the strict proviso that no changes or edits are made and the original work is properly cited (including links to both the formal publication through the relevant DOI and the license). See: https://creativecommons.org/licenses/by-nc-nd/4.0/.
References
- Gallone G, Baldetti L, Pagnesi M, et al. Medical Therapy for Long-Term Prevention of Atherothrombosis Following an Acute Coronary Syndrome: JACC State-of-the-Art Review. J Am Coll Cardiol 2018;72:2886-903. [Crossref] [PubMed]
- Mensah GA, Roth GA, Fuster V. The Global Burden of Cardiovascular Diseases and Risk Factors: 2020 and Beyond. J Am Coll Cardiol 2019;74:2529-32. [Crossref] [PubMed]
- Ren PP, Ren CF, Li HJ, et al. P Pink1-parkin pathway and its relationship with myocardial ischemia-reperfusion injury. J Med Res 2020;49:179-82.
- Jennings RB, Reimer KA. The cell biology of acute myocardial ischemia. Annu Rev Med 1991;42:225-46. [Crossref] [PubMed]
- Jennings RB. Historical perspective on the pathology of myocardial ischemia/reperfusion injury. Circ Res 2013;113:428-38. [Crossref] [PubMed]
- Bell RM, Yellon DM. There is more to life than revascularization: therapeutic targeting of myocardial ischemia/reperfusion injury. Cardiovasc Ther 2011;29:e67-79. [Crossref] [PubMed]
- Liu H, Shang J, Chu F, et al. Protective Effects of Shen-Yuan-Dan, a Traditional Chinese Medicine, against Myocardial Ischemia/Reperfusion Injury In Vivo and In Vitro. Evid Based Complement Alternat Med 2013;2013:956397. [Crossref] [PubMed]
- Mokhtari-Zaer A, Marefati N, Atkin SL, et al. The protective role of curcumin in myocardial ischemia-reperfusion injury. J Cell Physiol 2018;234:214-22. [Crossref] [PubMed]
- Zhou H, Wang S, Hu S, et al. ER-Mitochondria Microdomains in Cardiac Ischemia-Reperfusion Injury: A Fresh Perspective. Front Physiol 2018;9:755. [Crossref] [PubMed]
- Lochner A, Marais E, Huisamen B. Melatonin and cardioprotection against ischaemia/reperfusion injury: What's new? A review. J Pineal Res 2018;65:e12490. [Crossref] [PubMed]
- Wang M, Smith K, Yu Q, et al. Mitochondrial connexin 43 in sex-dependent myocardial responses and estrogen-mediated cardiac protection following acute ischemia/reperfusion injury. Basic Res Cardiol 2019;115:1. [Crossref] [PubMed]
- Zhang HF, Wang YL, Tan YZ, et al. Enhancement of cardiac lymphangiogenesis by transplantation of CD34(+)VEGFR-3(+) endothelial progenitor cells and sustained release of VEGF-C. Basic Res Cardiol 2019;114:43. [Crossref] [PubMed]
- Vela D. Keeping heart homeostasis in check through the balance of iron metabolism. Acta Physiol (Oxf) 2020;228:e13324. [Crossref] [PubMed]
- Sluijter JP, Condorelli G, Davidson SM, et al. Novel therapeutic strategies for cardioprotection. Pharmacol Ther 2014;144:60-70. [Crossref] [PubMed]
- Murphy E, Steenbergen C. Mechanisms underlying acute protection from cardiac ischemia-reperfusion injury. Physiol Rev 2008;88:581-609. [Crossref] [PubMed]
- Maneechote C, Palee S, Chattipakorn SC, et al. Roles of mitochondrial dynamics modulators in cardiac ischaemia/reperfusion injury. J Cell Mol Med 2017;21:2643-53. [Crossref] [PubMed]
- Tang X, Luo YX, Chen HZ, et al. Mitochondria, endothelial cell function, and vascular diseases. Front Physiol 2014;5:175. [Crossref] [PubMed]
- Reichard A, Asosingh K. The role of mitochondria in angiogenesis. Mol Biol Rep 2019;46:1393-400. [Crossref] [PubMed]
- Thomas RL, Gustafsson AB. Mitochondrial autophagy--an essential quality control mechanism for myocardial homeostasis. Circ J 2013;77:2449-54. [Crossref] [PubMed]
- Dorn GW 2nd, Vega RB, Kelly DP. Mitochondrial biogenesis and dynamics in the developing and diseased heart. Genes Dev 2015;29:1981-91. [Crossref] [PubMed]
- Leites EP, Morais VA. Mitochondrial quality control pathways: PINK1 acts as a gatekeeper. Biochem Biophys Res Commun 2018;500:45-50. [Crossref] [PubMed]
- Zhou H, Toan S. Pathological Roles of Mitochondrial Oxidative Stress and Mitochondrial Dynamics in Cardiac Microvascular Ischemia/Reperfusion Injury. Biomolecules 2020;10:85. [Crossref] [PubMed]
- Agarwal B, Stowe DF, Dash RK, et al. Mitochondrial targets for volatile anesthetics against cardiac ischemia-reperfusion injury. Front Physiol 2014;5:341. [Crossref] [PubMed]
- Kohlhauer M, Pell VR, Burger N, et al. Protection against cardiac ischemia-reperfusion injury by hypothermia and by inhibition of succinate accumulation and oxidation is additive. Basic Res Cardiol 2019;114:18. [Crossref] [PubMed]
- Ma S, Dong Z. Melatonin Attenuates Cardiac Reperfusion Stress by Improving OPA1-Related Mitochondrial Fusion in a Yap-Hippo Pathway-Dependent Manner. J Cardiovasc Pharmacol 2019;73:27-39. [Crossref] [PubMed]
- Shi X, Cheng S, Wang W. Suppression of CCT3 inhibits malignant proliferation of human papillary thyroid carcinoma cell. Oncol Lett 2018;15:9202-8. [Crossref] [PubMed]
- Aanhane E, Schulkens IA, Heusschen R, et al. Different angioregulatory activity of monovalent galectin-9 isoforms. Angiogenesis 2018;21:545-55. [Crossref] [PubMed]
- Sajib S, Zahra FT, Lionakis MS, et al. Mechanisms of angiogenesis in microbe-regulated inflammatory and neoplastic conditions. Angiogenesis 2018;21:1-14. [Crossref] [PubMed]
- Zhou H, Shi C, Hu S, et al. BI1 is associated with microvascular protection in cardiac ischemia reperfusion injury via repressing Syk-Nox2-Drp1-mitochondrial fission pathways. Angiogenesis 2018;21:599-615. [Crossref] [PubMed]
- Zhang W, Wang Y, Wan J, et al. COX6B1 relieves hypoxia/reoxygenation injury of neonatal rat cardiomyocytes by regulating mitochondrial function. Biotechnol Lett 2019;41:59-68. [Crossref] [PubMed]
- Walters AM, Porter GA Jr, Brookes PS. Mitochondria as a drug target in ischemic heart disease and cardiomyopathy. Circ Res 2012;111:1222-36. [Crossref] [PubMed]
- Zhang Z, Xing W, Liu H, et al. Effects of Shen-Yuan-Dan on Periprocedural Myocardial Injury and the Number of Peripheral Blood Endothelial Progenitor Cells in Patients with Unstable Angina Pectoris Undergoing Elective Percutaneous Coronary Intervention. Evid Based Complement Alternat Med 2022;2022:9055585. [Crossref] [PubMed]
- Liu DP, Liao L, Li SP, et al. Effect of Shenyuan Yiqi Huoxue Capsule on myocardial ischemia-reperfusion injury in rats. Beijing J Trad Chin Med 2022;41:132-5.
- Zhou M, Ren P, Zhang Y, et al. Shen-Yuan-Dan Capsule Attenuates Atherosclerosis and Foam Cell Formation by Enhancing Autophagy and Inhibiting the PI3K/Akt/mTORC1 Signaling Pathway. Front Pharmacol 2019;10:603. [Crossref] [PubMed]
- Livak KJ, Schmittgen TD. Analysis of relative gene expression data using real-time quantitative PCR and the 2(-Delta Delta C(T)) Method. Methods 2001;25:402-8. [Crossref] [PubMed]
- King DR, Padget RL, Perry J, et al. Elevated perfusate [Na+] increases contractile dysfunction during ischemia and reperfusion. Sci Rep 2020;10:17289. [Crossref] [PubMed]
- Kulek AR, Anzell A, Wider JM, et al. Mitochondrial Quality Control: Role in Cardiac Models of Lethal Ischemia-Reperfusion Injury. Cells 2020;9:214. [Crossref] [PubMed]
- Garcia-Dorado D, Piper HM. Postconditioning: reperfusion of "reperfusion injury" after hibernation. Cardiovasc Res 2006;69:1-3. [Crossref] [PubMed]
- Braunwald E, Kloner RA. Myocardial reperfusion: a double-edged sword? J Clin Invest 1985;76:1713-9. [Crossref] [PubMed]
- Tullio F, Angotti C, Perrelli MG, et al. Redox balance and cardioprotection. Basic Res Cardiol 2013;108:392. [Crossref] [PubMed]
- Buja LM, Vander Heide RS. Pathobiology of Ischemic Heart Disease: Past, Present and Future. Cardiovasc Pathol 2016;25:214-20. [Crossref] [PubMed]
- McCully JD, Wakiyama H, Hsieh YJ, et al. Differential contribution of necrosis and apoptosis in myocardial ischemia-reperfusion injury. Am J Physiol Heart Circ Physiol 2004;286:H1923-35. [Crossref] [PubMed]
- Kuznetsov AV, Javadov S, Margreiter R, et al. The Role of Mitochondria in the Mechanisms of Cardiac Ischemia-Reperfusion Injury. Antioxidants (Basel) 2019;8:454. [Crossref] [PubMed]
- Caccioppo A, Franchin L, Grosso A, et al. Ischemia Reperfusion Injury: Mechanisms of Damage/Protection and Novel Strategies for Cardiac Recovery/Regeneration. Int J Mol Sci 2019;20:5024. [Crossref] [PubMed]
- Zhou M, Yu Y, Luo X, et al. Myocardial Ischemia-Reperfusion Injury: Therapeutics from a Mitochondria-Centric Perspective. Cardiology 2021;146:781-92. [Crossref] [PubMed]
- Wang J, Toan S, Zhou H. New insights into the role of mitochondria in cardiac microvascular ischemia/reperfusion injury. Angiogenesis 2020;23:299-314. [Crossref] [PubMed]
- Gong Z, Pan J, Shen Q, et al. Mitochondrial dysfunction induces NLRP3 inflammasome activation during cerebral ischemia/reperfusion injury. J Neuroinflammation 2018;15:242. [Crossref] [PubMed]
- Wang J, Zhou H. Mitochondrial quality control mechanisms as molecular targets in cardiac ischemia-reperfusion injury. Acta Pharm Sin B 2020;10:1866-79. [Crossref] [PubMed]
- Lin KL, Chen SD, Lin KJ, et al. Quality Matters? The Involvement of Mitochondrial Quality Control in Cardiovascular Disease. Front Cell Dev Biol 2021;9:636295. [Crossref] [PubMed]
- Kamisawa T, Wood LD, Itoi T, et al. Pancreatic cancer. Lancet 2016;388:73-85. [Crossref] [PubMed]
- Schmitt AM, Chang HY. Long Noncoding RNAs in Cancer Pathways. Cancer Cell 2016;29:452-63. [Crossref] [PubMed]
- Sciarretta S, Maejima Y, Zablocki D, et al. The Role of Autophagy in the Heart. Annu Rev Physiol 2018;80:1-26. [Crossref] [PubMed]
- Sciarretta S, Hariharan N, Monden Y, et al. Is autophagy in response to ischemia and reperfusion protective or detrimental for the heart? Pediatr Cardiol 2011;32:275-81. [Crossref] [PubMed]
- Przyklenk K, Undyala VV, Wider J, et al. Acute induction of autophagy as a novel strategy for cardioprotection: getting to the heart of the matter. Autophagy 2011;7:432-3. [Crossref] [PubMed]
- Xi RX, Chen KJ, Shi DZ, et al. Evaluation of standard of TCM syndrome diagnosis of coronary heart disease after interventional surgery. Chin J Integrated Trad West Med 2013;33:1036-41.
- Chu F, Yan X, Xiong X, et al. Traditional Chinese Medicine Shen-Yuan-Dan (SYD) Improves Hypoxia-Induced Cardiomyocyte Apoptosis in Neonatal Rats by Upregulating miR-24/Bim Pathway. Evid Based Complement Alternat Med 2022;2022:5804187. [Crossref] [PubMed]
- Picca A, Mankowski RT, Burman JL, et al. Mitochondrial quality control mechanisms as molecular targets in cardiac ageing. Nat Rev Cardiol 2018;15:543-54. [Crossref] [PubMed]
- Ishihara N, Eura Y, Mihara K. Mitofusin 1 and 2 play distinct roles in mitochondrial fusion reactions via GTPase activity. J Cell Sci 2004;117:6535-46. [Crossref] [PubMed]
- Gleyzer N, Scarpulla RC. PGC-1-related coactivator (PRC), a sensor of metabolic stress, orchestrates a redox-sensitive program of inflammatory gene expression. J Biol Chem 2011;286:39715-25. [Crossref] [PubMed]
- Villena JA. New insights into PGC-1 coactivators: redefining their role in the regulation of mitochondrial function and beyond. FEBS J 2015;282:647-72. [Crossref] [PubMed]
- Narendra DP, Jin SM, Tanaka A, et al. PINK1 is selectively stabilized on impaired mitochondria to activate Parkin. PLoS Biol 2010;8:e1000298. [Crossref] [PubMed]
- Jin SM, Lazarou M, Wang C, et al. Mitochondrial membrane potential regulates PINK1 import and proteolytic destabilization by PARL. J Cell Biol 2010;191:933-42. [Crossref] [PubMed]
- Jin SM, Youle RJ. The accumulation of misfolded proteins in the mitochondrial matrix is sensed by PINK1 to induce PARK2/Parkin-mediated mitophagy of polarized mitochondria. Autophagy 2013;9:1750-7. [Crossref] [PubMed]
- Huang J, Li R, Wang C. The Role of Mitochondrial Quality Control in Cardiac Ischemia/Reperfusion Injury. Oxid Med Cell Longev 2021;2021:5543452. [Crossref] [PubMed]
- Yang X, Zhou Y, Liang H, et al. VDAC1 promotes cardiomyocyte autophagy in anoxia/reoxygenation injury via the PINK1/Parkin pathway. Cell Biol Int 2021;45:1448-58. [Crossref] [PubMed]
- Li F, Fan X, Zhang Y, et al. Inhibition of myosin IIA-actin interaction prevents ischemia/reperfusion induced cardiomyocytes apoptosis through modulating PINK1/Parkin pathway and mitochondrial fission. Int J Cardiol 2018;271:211-8. [Crossref] [PubMed]
(English Language Editor: J. Gray)