Inhibition of ERK or Akt ameliorates intimal hyperplasia via up-regulation of Cx37 and down-regulation of Cx43 in balloon injury rat model
Introduction
Vascular endothelial injury can trigger the development of atherosclerosis, carotid artery stenosis, and percutaneous coronary intervention (PCI) induced restenosis, which could be attributed to the occurrence of intimal hyperplasia (IH) (1,2). When the vascular endothelium is injured, vascular smooth muscle cells (VSMCs) can migrate from the medial to the intimal layer, proliferate, and secrete extracellular matrix to form restenotic lesions (3). Although drug-eluting stents are designed to decrease the incidence of mechanical injury associated stenosis, it remains a bane to high-risk patients with atherosclerosis, hypertension, and diabetes. It is estimated that almost 40–50% of patients with stenosis will endure restenosis within one year after angioplasty intervention at previous atheromatous lesions. Therefore, it is vital to understand the mechanisms underlying the IH process to develop novel approaches to control the restenosis.
Heterocellular communications, such as gap junction, between VSMCs and endothelial cells (ECs) are essential to the function of the vascular wall (4,5). It is further testified that gap junctions can be homotypic or formed from combinations of the 21 connexin isoforms. In the development of atherosclerosis, a progressive change in connexins (Cxs) expression can be observed at the point of contact between EC and VSMC, and altered Cxs expression pattern can be detected in atherosclerotic plaques (6-8). All of these indicate that Cxs take part in the development of atherosclerosis, whether such molecules are also involved in the balloon injury (BI) induced IH and restenosis is less reported.
In this investigation, restenosis after BI is utilized as a model to decipher the relevant mechanism associated with Cxs in the development of IH.
We present the following article in accordance with the ARRIVE reporting checklist (available at http://dx.doi.org/10.21037/cdt-20-345).
Methods
Animals
Male Sprague-Dawley rats (250 g) were purchased from Peking Vital River Laboratory Animal Ltd. (Beijing, China), which were maintained with a standard 12 hours light-dark cycle. All experimental procedures and animal feeding were approved by the Animal Care and Use Committee of the First Affiliated Hospital of Wenzhou Medical University (#WZYKDX-311), in compliance with institutional guidelines of Wenzhou Medical University for the care and use of animals.
Induction of BI rat model
In this research, 48 rats were randomly assigned into BI group and not injured (NI) group. BI rats were anesthetized with isoflurane, and the left external carotid artery was exposed and temporally blocked with arterial clamps on both proximal and distal sides (9). A balloon catheter (ϕ =1.5 mm, Sprinter Legend™, Medtronic, Inc., MN, USA) was inserted into the lumen and inflated to 6 atm (Dolphin inflation device, Perouse Medical, France), which was further pulled back and forth three times for a short distance to produce endothelial denudation (10). NI rats underwent a sham surgery was utilized as a control. Pain relievers and antibiotics were given subcutaneously, and the rats were monitored routinely during recovery. Rats were sacrificed by cervical dislocation at 6 hours, 24 hours, 7 days, and 14 days post the surgery for further assessments.
Histopathological assessment
After harvest, carotid arteries were fixed with paraformaldehyde (4%) and embedded with paraffin. Serial cross-sections (2 µm) were performed along the entire injured segment, then hematoxylin and eosin (H&E) staining was utilized to detect neointimal hyperplasia. Intima, media, and lumen areas quantification was obtained with NIH-Image J1.51p 22 (National Institutes of Health, Bethesda, MD, USA).
Proliferating cell nuclear antigen (PCNA) was stained in carotid sections. Microwave-heating was applied for antigen retrieval, and nonspecific blocking was performed by 4% normal goat serum plus 1% bovine serum albumin (BSA) in phosphate-buffered saline for 30 min. Then, an anti-rat PCNA rabbit polyclonal antibody (1:100; Santa Cruz Biotechnology Inc., Santa Cruz, CA) was used to incubate the sections. Biotinylated secondary antibody, avidin:biotinylated enzyme complex, and 3,3'-diaminobenzidine substrate (Zhongshan Goldenbridge Biotechnology, Guangzhou, China) were utilized to develop the signal. Finally, the slides were counterstained with hematoxylin and analyzed.
Treatment
Each group (N=6) BI and NI rats were administrated with U0216 (ERK-inhibitor, 50 mg/kg body weight) or MIK2206 (Akt-inhibitor, 100 mg/kg body weight) via tail vein per day until day 14, which were then sacrificed for assessment. The dose chosen was based on the previous report (11).
Western blots
Carotid tissues were homogenized with IKA disperser in SDS-lysis buffer (10 mM Tris-HCl, 2 mM EDTA, 1% SDS), which was further separated by 12% sodium dodecyl sulphate-polyacrylamide gel electrophoresis and transferred to a polyvinyl difluoride membrane. After block with 5% non-fat dry milk, the membranes were incubated with the primary antibodies of Cx30, Cx37, Cx40, Cx43, ERK, AKT, phospho (p)-ERK and (p)-AKT (Santa Cruz Biotechnology Inc., Santa Cruz, CA) at 1:1,000 dilution (4 °C, overnight), which were further incubated in peroxidase-conjugated secondary antibody (Sigma-Aldrich, St. Louis, MO) at a 1:1,000 dilution for 1 hour at room temperature. The signal was developed with an ECL system (GE Healthcare Life Sciences, Chalfont, UK). After intensity normalization to its respective β-actin, the ratio of phosphorylated over total protein expression was calculated with Image J software.
Quantitative real-time RT-PCR (qRT-PCR)
Total RNA was extracted from carotid tissues (n=6 per group) with TRIzol™ Plus RNA Purification Kit (Thermo Fisher Scientific, Inc., Rockford, IL, USA). cDNA Reverse Transcription Kit (Applied Biosystems, Foster City, CA, USA) was utilized to reverse-transcribed cDNA from 1 µg RNA. SYBR Green master mix (Roche, Mannheim, Germany) was adopted to indicate the amplification efficiency, and the reaction procedures were as follows: 95 °C for 10 min, 40 cycles of 95 °C for 15 s, and 60 °C for 1 min. Interest genes expressions were normalized to β-actin mRNA expression. Primer sequences were listed: β-actin, forward primer 5'-CCCGCGAGTACAACCTTCT-3', reverse primer 5'-CGTCATCCATGGCGAACT-3'; CX30, 5'-ATCTTCCGCATCCTGGTG-3', reverse primer 5'-AGTGACAGAGTGGCAGGTCA-3'; CX37, forward primer 5'-CGGTCGTCCCCTCTACCT-3', reverse primer 5'-GTCGAGTGCTCCTGGACCT-3'; CX40, forward primer 5'- AAGGCTTAGTTAGCATCACAGAAAG-3', reverse primer 5'-TGGACCCCAGAGTTCAATG-3'; CX43, forward primer 5'-GAGCCTGAACTCTCATTTTTCC-3', reverse primer 5'-CCATGTCTGGGCACCTCT-3'.
Statistical analysis
All experiments were quantitatively analyzed with GraphPad Prism 6, and results were reported as the mean ± SEM and analyzed by Student’s t-test or one-way ANOVA analysis followed by a Tukey’s post hoc test. P<0.05 was considered to be statistically significant.
Results
Temporal expression of Cxs
Both BI and NI rats kept healthy after the surgery without significant weight loss during the whole period of this investigation. Every 6 rats of the two groups were sacrificed at 6 hours, 24 hours, 7 days, and 14 days post the surgery for Cx30, Cx37, Cx40, and Cx43 assessments. Western blot results indicated that Cx37 showed decreased expression pattern as time passed and Cx43 showed increased expression trend, while there was no significant difference in Cx30 and Cx40 (Figure 1A,B) (P<0.01 or P<0.001). In accordance with this, the relative mRNA expression of Cx30, Cx37, Cx40, and Cx43 also showed the same expression trend (Figure 1C). All of these indicated that among the four Cxs detected, only Cx37 and Cx43 were differentials expressed during the whole process after BI.
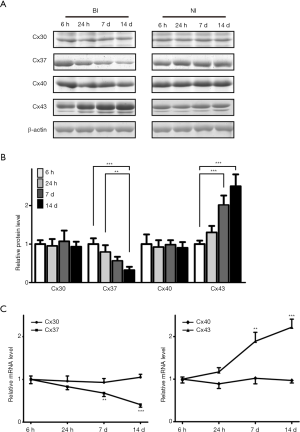
BI-induced neointima formation
According to the results of Cxs expression, neointima formation determined by H&E staining was observed 14 days after BI (6 rats in each group). There was no development of IH could be observed in rats received sham surgery, and significantly increased neointimal formation could be easily identified in the BI group (Figure 2A). Quantitative morphometry estimation exhibited notable increases in the intimal area (Figure 2B, P<0.01). Nevertheless, there was no significant difference in the media areas between BI group and NI group (Figure 2C). The luminal areas were decreased in the BI group compared with the NI group (Figure 2D, P<0.01), while a significant increase could be found in intima-to-media (I/M) ratio between the two groups (Figure 2E, P<0.01).
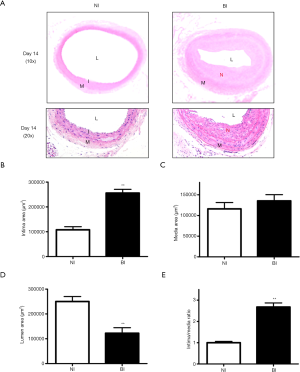
Up-regulated ERK and Akt expression
Mitogen-activated protein kinase (MAPK, also known as ERK) pathway and PI3K/Akt pathway are testified to be essential in neointima formation (12,13). In this investigation, total ERK, p-ERK and p-Akt showed up-regulated expression in BI rats (Figure 3A), and quantification of the Akt and ERK phosphorylation levels also showed up-regulated expression when compared with NI rats (Figure 3B, P<0.01). Administration with Akt-inhibitor MIK2206 could significantly down-regulate the expression of Cx43 (Figure 3C,D, P<0.01) and up-regulate the expression of Cx37 (Figure 3C,E, P<0.01). Meanwhile, ERK-inhibitor U0216 could also significantly down-regulate the expression of Cx43 (Figure 3C,D, P<0.01) and up-regulate the expression of Cx37 (Figure 3C,E, P<0.001). Taken together, these results indicated that the MAPK and Akt pathway could regulate the expression of Cx37 and Cx43.
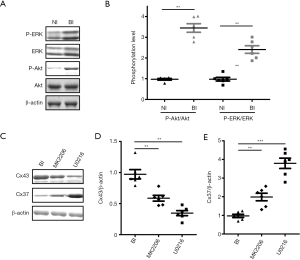
ERK and Akt mediated neointima formation and cell proliferation
Neointima formation assayed by H&E staining was utilized to determine the treatment effect of Akt-inhibitor and ERK inhibitor. When compared with BI rats, MK2206 (P<0.05) and U0126 (P<0.01) could significantly attenuate the neointima formation indicated by the I/M ratio (Figure 4A,B). MK2206 and U0126 could remarkably down-regulate PCNA expression in carotid arteries of BI rat (Figure 4C), which was further indicated by quantitative analysis of PCNA positive area in the media (Figure 4D) and intima area (Figure 4E) over the total carotid area. All of these indicated that ERK and Akt mediated balloon-injury induced neointima formation and associated cell proliferation.
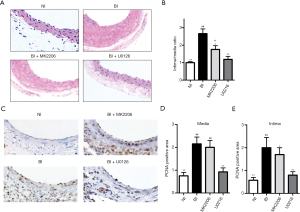
Discussion
Of the 21 identified Cxs, vascular ECs primarily express Cx37, Cx40, and Cx43 (14,15), all of which will facilitate the formation of gap junction channels and hemichannels. Gap junction channels, composed of two docked hemichannels oligomerized from six connexin molecules, can directly connect the cytoplasm of adjacent VSMCs and ECs and facilitate the propagation of chemical and electrical signals, which is essential for the function of the blood vessels (16,17).
Alterations in Cxs expression, localization, phosphorylation status, and gap junction properties are thought to contribute to cardiovascular dysregulation (18-20). It is Cx37, not Cx40, that is associated with aging and cardiac disease, which can serve as predictive markers for prognosis of post-stenting complications in patients with atherosclerosis (21). Up-regulation of connexin43 gap junctions between smooth muscle cells after balloon catheter injury in the rat carotid artery is observed (22). In contrast, reduced connexin43 expression could limit neointima formation after balloon distension injury in hypercholesterolemic mice (23). It is worth noting that Cx43 is induced in VSMCs during coronary arteriogenesis (24,25).
Cx43 over-expression in human primary VSMCs can increase P-Akt levels (26). In this investigation, both Cx37 and Cx43, not Cx40 are found to be involved in the BI-induced IH, which is further testified to be regulated by MAPK and Akt pathway. It is testified that MAPK cascade can be activated by mechanical injury-induced growth factors release (27), which can phosphorylate connexin to be involved in the regulation of assembly, disassembly, trafficking, and degradation, as well as the gating of gap junction channels (28,29). While the role of Akt in the process of IH is less discussed. This investigation also demonstrates that ERK-inhibitor U0216 is more effective than Akt-inhibitor MIK2206 to attenuate IH, although detailed mechanism still needs further decipher.
Different expression patterns of Cxs in diverse tissue suggest that there might be molecular movement specificity through gap junctions. It is worth noting that Cx40/Cx43 expression ratio can influence heteromeric/heterotypic gap junction channel properties, such as selectivity for dye movement, electric signaling, and second messenger coupling (30). Besides, phosphorylation of connexin proteins can regulate the junctional permeability (31,32). Whether co-expression or mutually expression of Cx37 and Cx43 or phosphorylation modulation is involved in BI-induced IH needs further investigation.
Although anti-platelet drugs, drug-eluting stent implantation, anti-thrombotic drugs, and brachytherapy have been utilized to overcome restenosis after PCI, none of them has shown long-term benefit (33,34). The quiescent VSMCs’ re-entry into the cell cycle, proliferation, and migration are hallmark biological events in IH process, which is thought to be regulated by the gap junction communication between VSMCs and ECs. So, it is necessary to deepen the research on the regulation of Cxs.
Although the detailed mechanism involved in the BI-induced IH remains unclear, this investigation suggests that ERK and Akt mediated down-regulated Cx37 and up-regulated Cx43 are involved.
Conclusions
ERK-inhibitor U0216 and Akt-inhibitor MIK2206 can ameliorate BI-induced IH via up-regulation of Cx37 and down-regulation of Cx43.
Acknowledgments
Funding: The study was supported by the Wenzhou Science and Technology Project in Zhejiang Province (Y20180669).
Footnote
Reporting Checklist: The authors present the study following the ARRIVE reporting checklist. Available at http://dx.doi.org/10.21037/cdt-20-345
Data Sharing Statement: Available at http://dx.doi.org/10.21037/cdt-20-345
Peer Review File: Available at http://dx.doi.org/10.21037/cdt-20-345
Conflicts of Interest: All authors have completed the ICMJE uniform disclosure form (available at http://dx.doi.org/10.21037/cdt-20-345). The authors have no conflicts of interest to declare.
Ethical Statement: The authors are accountable for all aspects of the work in ensuring that questions related to the accuracy or integrity of any part of the work are appropriately investigated and resolved. All experimental procedures and animal feeding were approved by the Animal Care and Use Committee of the First Affiliated Hospital of Wenzhou Medical University (#WZYKDX-311), in compliance with institutional guidelines of Wenzhou Medical University for the care and use of animals.
Open Access Statement: This is an Open Access article distributed in accordance with the Creative Commons Attribution-NonCommercial-NoDerivs 4.0 International License (CC BY-NC-ND 4.0), which permits the non-commercial replication and distribution of the article with the strict proviso that no changes or edits are made and the original work is properly cited (including links to both the formal publication through the relevant DOI and the license). See: https://creativecommons.org/licenses/by-nc-nd/4.0/.
References
- Xu K, Al-Ani MK, Pan X, et al. Plant-Derived Products for Treatment of Vascular Intima Hyperplasia Selectively Inhibit Vascular Smooth Muscle Cell Functions. Evid Based Complement Alternat Med 2018;2018:3549312.
- Tesfamariam B. Bioresorbable Scaffold-Based Controlled Drug Delivery for Restenosis. J Cardiovasc Transl Res 2019;12:193-203. [Crossref] [PubMed]
- Zhang M, Zhang Z, Xie X, et al. Xenobiotic pregnane X receptor promotes neointimal formation in balloon‐injured rat carotid arteries. J Cell Physiol 2019;234:4342-51. [Crossref] [PubMed]
- Sandow SL, Robin LW, Beth D, et al. Expression of homocellular and heterocellular gap junctions in hamster arterioles and feed arteries. Cardiovasc Res 2003;60:643-53. [Crossref] [PubMed]
- Isakson BE, Ramos SI, Duling BR. Ca2+ and inositol 1, 4, 5-trisphosphate–mediated signaling across the myoendothelial junction. Circ Res 2007;100:246-54. [Crossref] [PubMed]
- Davies PF, Shi C, Depaola N, et al. Hemodynamics and the focal origin of atherosclerosis: a spatial approach to endothelial structure, gene expression, and function. Ann NY Acad Sci 2001;947:7-16; discussion 16-7. [Crossref] [PubMed]
- Kwak BR, Mulhaupt F, Veillard N, et al. Altered pattern of vascular connexin expression in atherosclerotic plaques. Arterioscler Thromb Vasc Biol 2002;22:225-30. [Crossref] [PubMed]
- Pfenniger A, Chanson M, Kwak BR. Connexins in atherosclerosis. BBA-Biomembranes 2013;1828:157-66.
- Tulis DA. Rat carotid artery balloon injury model. Methods Mol Med 2007;139:1-30. [Crossref] [PubMed]
- Clowes AW, Clowes MM. Kinetics of cellular proliferation after arterial injury. IV. Heparin inhibits rat smooth muscle mitogenesis and migration. Circ Res 1986;58:839-45. [Crossref] [PubMed]
- Ahnstedt H, Mostajeran M, Blixt FW, et al. U0126 attenuates cerebral vasoconstriction and improves long-term neurologic outcome after stroke in female rats. J Cereb Blood Flow Metab 2015;35:454-60. [Crossref] [PubMed]
- Fang H, Yang S, Luo Y, et al. Notoginsenoside R1 inhibits vascular smooth muscle cell proliferation, migration and neointimal hyperplasia through PI3K/Akt signaling. Sci Rep 2018;8:7595. [Crossref] [PubMed]
- Zhou J, Du T, Li B, et al. Crosstalk Between MAPK/ERK and PI3K/AKT Signal Pathways During Brain Ischemia/Reperfusion. ASN Neuro 2015;7:1759091415602463. [Crossref] [PubMed]
- Brisset AC, Isakson BE, Kwak BR. Connexins in vascular physiology and pathology. Antioxid Redox Signal 2009;11:267-82. [Crossref] [PubMed]
- Inai T, Shibata Y. Heterogeneous expression of endothelial connexin (Cx) 37, Cx40, and Cx43 in rat large veins. Anat Sci Int 2009;84:237-45. [Crossref] [PubMed]
- Wang N, De Vuyst E, Ponsaerts R, et al. Selective inhibition of Cx43 hemichannels by Gap19 and its impact on;myocardial ischemia/reperfusion injury. Basic Res Cardiol 2013;108:309. [Crossref] [PubMed]
- Kotini M, Barriga EH, Leslie J, et al. Gap junction protein Connexin-43 is a direct transcriptional regulator of N-cadherin in vivo. Nat Commun 2018;9:3846. [Crossref] [PubMed]
- Danik SB, Fangyu L, Jie Z, et al. Modulation of cardiac gap junction expression and arrhythmic susceptibility. Circ Res 2004;95:1035-41. [Crossref] [PubMed]
- Severs NJ, Bruce AF, Dupont E, et al. Remodelling of gap junctions and connexin expression in diseased myocardium. Cardiovasc Res 2008;80:9-19. [Crossref] [PubMed]
- Solan JL, Lampe PD. Spatio-temporal regulation of connexin43 phosphorylation and gap junction dynamics. Biochim Biophys Acta Biomembr 2018;1860:83-90.
- Kozlov KL, Bolotov II, Lin'kova NS, et al. Expression of Signal Molecules in Culture of Human Endothelial Cells in Atherosclerosis and Restenosis. Bull Exp Biol Med 2017;163:566-9. [Crossref] [PubMed]
- Yeh HI, Lupu F, Dupont E, et al. Upregulation of connexin43 gap junctions between smooth muscle cells after balloon catheter injury in the rat carotid artery. Arterioscler Thromb Vasc Biol 1997;17:3174-84. [Crossref] [PubMed]
- Chadjichristos CE, Matter CM, Roth I, et al. Reduced connexin43 expression limits neointima formation after balloon distension injury in hypercholesterolemic mice. Circulation 2006;113:2835-43. [Crossref] [PubMed]
- Cai WJ, Koltai S, Kocsis E, et al. Connexin37, not Cx40 and Cx43, is Induced in Vascular Smooth Muscle Cells During Coronary Arteriogenesis. J Mol Cell Cardiol 2001;33:957-67. [Crossref] [PubMed]
- Johnstone S, Isakson B, Locke D. Biological and biophysical properties of vascular connexin channels. Int Rev Cell Mol Biol 2009;278:69-118. [Crossref] [PubMed]
- Allagnat F, Dubuis C, Lambelet M, et al. Connexin37 reduces smooth muscle cell proliferation and intimal hyperplasia in a mouse model of carotid artery ligation. Cardiovasc Res 2017;113:805-16. [Crossref] [PubMed]
- Hu Y, Cheng L, Hochleitner BW, et al. Activation of mitogen-activated protein kinases (ERK/JNK) and AP-1 transcription factor in rat carotid arteries after balloon injury. Arterioscler Thromb Vasc Biol 1997;17:2808-16. [Crossref] [PubMed]
- Lampe PD, Lau AF. Regulation of Gap Junctions by Phosphorylation of Connexins. Arch Biochem Biophys 2000;384:205-15. [Crossref] [PubMed]
- Freitas-Andrade M, Wang N, Bechberger JF, et al. Targeting MAPK phosphorylation of Connexin43 provides neuroprotection in stroke. J Exp Med 2019;216:916-35. [Crossref] [PubMed]
- Cottrell GT, Wu Y, Burt JM. Cx40 and Cx43 expression ratio influences heteromeric/ heterotypic gap junction channel properties. Am J Physiol Cell Physiol 2002;282:1469-82. [Crossref] [PubMed]
- Darren L, Jade L, Harris AL. Lipid rafts prepared by different methods contain different connexin channels, but gap junctions are not lipid rafts. Biochemistry 2005;44:13027-42. [Crossref] [PubMed]
- Xie H, Laird DW, Chang TH, et al. A mitosis-specific phosphorylation of the gap junction protein connexin43 in human vascular cells: biochemical characterization and localization. J Cell Biol 1997;137:203-10. [Crossref] [PubMed]
- Shammas NW. Restenosis after lower extremity interventions: current status and future directions. J Endovasc Ther 2009;16 Suppl 1:I170. [Crossref] [PubMed]
- Marcos EG, Da Fonseca AC, Hofma SH. Bridging therapy for early surgery in patients on dual antiplatelet therapy after drug-eluting stent implantation. Neth Heart J 2011;19:412-7. [Crossref] [PubMed]